Abstract
Clostridium perfringens can rarely cause severe systemic infections, usually from an abdominal source, associated with massive hemolysis, which is usually fatal. Hemolytic anemia and acute renal injury resulting from toxin action are critical for the development of multiple organ dysfunction syndrome (MODs), making this condition a real emergency, requiring multispecialty skills and aggressive multimodal therapies. We herein describe a case of septic shock from acute cholecystitis with massive hemolysis caused by C. perfringens in a 55 year-old man that was successfully treated with early blood purification and continuous renal replacement therapy (CRRT) along with antibiotic therapy and surgery. The effect of the enormous amount of toxins produced by Clostridium which elicit a strong cytokine response and the damage caused by the hemolysis products are the main pathogenetic mechanisms of this rare but lethal clinical entity. The main goal of treatment is to remove toxins from plasma, block toxin action, and further production by achieving bacterial killing with antimicrobial agents and controlling the infectious focus, remove waste products and prevent or limit multiorgan damage. Blood purification techniques play an important role due to a strong pathophysiological rationale, as they can remove toxins and cytokines as well as cell-free products from plasma and also replace renal function. Although this condition is rare and robust data are lacking, blood purification techniques for C. perfringens-induced massive hemolysis are promising and should be further explored.
Similar content being viewed by others
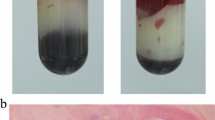
Avoid common mistakes on your manuscript.
Introduction
Clostridium perfringens is a Gram-positive anaerobic spore-forming rod found in soil, sewage and food and is part of the resident flora of human intestinal and genital tracts [1, 2]. Its protean role in human disease is well recognized: besides being the second cause of bacterial food poisoning, it can cause non-foodborne diarrhea and enteritis, necrotizing enterocolitis in fragile populations, asymptomatic bacteremia, and it is the major cause of gas gangrene (Clostridial myonecrosis); in addition, in the course of clostridial sepsis massive intravascular hemolysis (MIH) occurs in 7–15% of cases and results in high fatality, making it one of the most severe clinical presentation of bacteremia [1, 2].
The pathogenicity and virulence of C. perfringens are mainly due to its rapid doubling time (about 7 min) and huge toxin production, which induce both direct cellular damages (mostly to red blood cells—RBCs—with free Hb and cellular waste products release on plasma) and cytokine storm; all these factors account for the tumultuous clinical evolution of more severe C. perfringens infections, i.e., gas gangrene, necrotizing enterocolitis, and septic shock with MIH [1,2,3]. A multidisciplinary approach is mandatory: early source control, antibiotic therapy, and resuscitative measures aim to counteract multiple aspects of toxin production and action, prevent further toxin production, and eliminate the focus of infection; adjunctive therapies such as hyperbaric oxygen therapy (HBOT), plasma exchange, antitoxin drugs, and anti-IL6 monoclonal antibodies (tocilizumab) have been proposed [1,2,3]. Blood purification techniques are increasingly used in sepsis, especially in cases with high cytokine burden and are a promising option in this setting [2,3,4,5,6].
In this article, we describe a case of C. perfringens with massive hemolysis due to acute cholecystitis that was successfully treated with blood purification during continuous renal replacement therapy (CRRT), surgery, and antimicrobials. We analyze the effects of extracorporeal blood purification techniques on toxin removal, immune modulation, and protection against hemolysis damage.
Case report
A 55 year-old obese man with a history of kidney stones and recurrent urinary infections was admitted to the Emergency Department (ED) because of syncope and a three-day history of hyperpyrexia and chest pain. On arrival, he appeared agitated, jaundiced, febrile (40 °C), tachypneic, tachycardic, and hypotensive; on physical examination, there was diffuse abdominal tenderness and positive Murphy sign; bladder catheterization yielded scarce blackish urine. Initial chemistry tests, though distorted by excessive hemolysis, showed acute renal and hepatic failure, metabolic acidosis, coagulation abnormalities, blood smear revealed spherocytes and ghost cells (Table 1). Bedside ultrasound showed signs of hypovolemia and acute cholecystitis; at contrast computerized tomography (CT) scan, we found acute lithiasic cholecystitis with surrounding multiple liver abscesses.
Multiple blood cultures (BCs) were collected at admission (BacT/Alert®Virtuo blood culture system bioMérieux, France). Four hours after incubation, Gram staining revealed Gram-positive bacilli, which were subsequently identified as Clostridium perfringens by matrix-assisted laser desorption/ionization time-Of-flight mass spectrometry (MALDI-TOF, bioMérieux, Marcy l’Etoile, France). After sample collection, antibiotic treatment was started with piperacillin/tazobactam, clindamycin, and fosfomycin. IV methylprednisolone 1 mg/kg was added to control overwhelming hemolysis.
Due to rapidly worsening conditions, the patient was intubated and placed on vasopressor support. Continuous veno-venous hemodiafiltration (CVVHDF) with highly absorptive filter oXiris® (Baxter, Meyzieu, France on CRRT platform Prismax®, Baxter, USA) was started at effluent dose 70 ml/kg/h on regional citrate anticoagulation (Prisma citrate® 18.0 Gambro, Italy), (Table 2). Laparotomy cholecystectomy and bladder fossa debridement were performed 36 h after admission once hemodynamic stability and control of hemolysis were achieved (Fig. 1); peritoneal fluid and bile cultures were examined for aerobic and anaerobic pathogens according to laboratory-defined standard procedures: Clostridium perfringens was isolated from peritoneal fluid cultures, while bile culture was negative.
Clindamycin and fosfomycin were suspended on second and fifth postoperative day, respectively; after 72 h of blood purification, renal support was switched to standard continuous venovenous hemofiltration (CVVH): a standard AN69 membrane (ST150set on CRRT platform Prismax®, Baxter USA) was employed, with effluent dose 25 ml/kg/h, post-dilution replacement fluid 800 ml/h, regional citrate anticoagulation (Table 2). CVVH was continued for another 25 days, with weekly therapeutic drug monitoring (TDM) of piperacillin/tazobactam.
On day 20, because of fever recurrence and bladder fossa abscess, the patient underwent percutaneous drainage of residual perihepatic fluid collections; blood cultures grew toti-S Escherichia coli, and culture of drainage fluid yielded toti-S E. Coli and fosfomycin-resistant Klebsiella pneumoniae. The patient was discharged after 58 days with mild residual renal dysfunction (CrCl 36 ml/min); at 3 months, he had fully recovered.
Discussion
We have reported a case of massive hemolysis and septic shock due to Clostridium perfringens that was successfully treated with a multimodal approach including, in addition to antimicrobial therapy and surgical control of the infectious focus, and high-volume renal replacement therapy associated with cytokine absorption and toxins removal.
C. perfringens MIH is a rare (4.9 cases/100,000 per year worldwide) but rapidly fatal condition, with a 70–100% mortality rate, that has not decreased over the last decades [1,2,3]. C. perfringens sepsis has been found to be more prevalent in older comorbid patients: patients were mostly males in their seventies and suffering from diabetes, solid neoplasms or hematologic malignancies, liver and biliary tract diseases, and renal failure. Hepatobiliary and genital tracts are the most common source of infection, followed by intestinal tract infections, trauma, and instrumentation (e.g., endoscopic digestive procedures, abdominal surgery, cesarean section, abortion) [1, 2].
C. Perfringens’ main pathogenic action is toxin-mediated: up to 20 different toxinotypes are identified, referring to the predominant toxin production (α-toxin—C. perfringens alpha toxin CPA, β-toxin—CPB, ε-toxin—ETX, ι-toxin—ITX, enterotoxin—CPE, θ toxin—perfringolysin PFO, necrotic enteritis B-like toxin—NetB); all types produce CPA, and nearly all produce PFO, depicting their crucial role in pathogenic effects and virulence; in addition, the role of many other molecules, namely extracellular degradative enzymes, is still under investigation [2, 4, 5]. Alpha toxin (CPA) is a phospholipase C that destroys RBC membrane phospholipids and causes spherocytosis with subsequent hemolysis; it also exerts a negative inotropic effect, an inhibitory effect on neutrophil migration, and a vasoconstrictor effect, creating an anaerobic milieu in which clostridia can further replicate [2, 7,8,9]. PFO has recently emerged as a cause of major pathological damage associated with C. perfringens infection: it is a cholesterol-dependent cytolysin that binds biological membranes via a cholesterol-mediated pathway and causes the formation of pores on the membrane surface, leading to leakage of intracellular contents. PFO induces tumor necrosis factor-α (TNF-α), IL-5, IL-6, and IL-8 production more strongly than CPA, it exerts direct cytotoxicity in synergy with CPA and inhibits macrophage migration into affected tissues [2, 7, 8, 10].
Initial symptoms and signs are unspecific but rapidly worsening: impaired consciousness, severe pain (localized in the back, abdomen chest), hematuria, tachypnea, and respiratory failure; MODs rapidly ensue and can hinder any further diagnostic investigation [2, 9,10,11]. However, some laboratory features are quite characteristic: red coloration of plasma after centrifugation, anemia, altered values of chemistry analytes (liver function tests, LDH, creatine kinase, electrolytes), anisocytosis, spherocytosis, and ghost cells at blood smear can help differentiate MIH from other infectious (malaria, bartonellosis, and babesiosis) and non-infectious hemolytic conditions (sickle cell anemia, paroxysmal nocturnal hemoglobinuria, glucose 6-phosphate dehydrogenase deficiency with precipitating fever, and toxics); automatic signaling of hemolysis from laboratory analyzers usually triggers further tests such as blood smear [1,2,3, 12,13,14]. Traditional microbiological diagnosis (culture of blood, urine, and surgical samples) has slow turnaround times, although preliminary identification by Gram stain in positive blood samples offers valuable information; newer rapid identification techniques (i.e., MALDI-TOF) and culture-independent methods (multiplex polymerase chain reaction—PCR) are a promising field to explore as they become more available and affordable [2, 15, 16].
Prompt antimicrobial treatment is of utmost importance, as combination regimens of ureidopenicillin plus clindamycin are associated with improved survival: clindamycin rapidly suppresses toxin activity and achieves bacterial killing, but it must be used only in combination due to increasing rates of resistance; a rational empiric regimen of ureidopenicillin plus clindamycin is associated with improved survival compared to other combination regimens or penicillin alone [21]. Clostridia are usually susceptible to β-lactam combination agents, metronidazole, and tigecycline. Although it has no anti-anaerobe activity, fosfomycin may initially be added to broaden the spectrum, as half of C. perfringens infections are polymicrobial [2, 17]. When feasible, Source control (surgery or interventional procedures) is another positive prognostic factor, even in more severe patients [1, 2]. According to various clinical experiences, early extracorporeal renal replacement therapy and blood purification techniques may be useful in managing this peculiar condition [1, 2].
Extracorporeal blood purification techniques (EBPT) are now an established adjunctive therapy for septic shock and have a strong therapeutic rationale: early after infection, pathogen-associated molecular patterns (PAMPs) are recognized by host cells via specific receptors (mostly TLR), activating innate immunity and triggering production of a variety of pro- and anti-inflammatory cytokines; damaged cells release DAMPs (damage-associated molecular patterns), and perpetuate a loop that generates further damages and organ dysfunction; removal of these mediators by EBPT can lead to stabilization of hemodynamic function, organ protection, and immune modulation [2, 18]. Although not endorsed in the latest Surviving Sepsis Campaign (SSC) guidelines, evidence of the efficacy and safety of such therapies is accumulating. Technologies and devices are evolving, and data on improvement of hemodynamics and vasopressor- and ventilator-free days in intensive care unit (ICU) patients, if not yet improved survival rates, are compelling [19, 21].
Toxins, along with PAMPs, cytokines, and DAMPs can be removed by hemodiafiltration or absorption because of their low molecular weight relative to the pore size of the hemofilters and currently used CRRT membranes. The cut-off of the membranes is about 35–40 kDa, a dimension that allows filtering molecules of small-medium weight by convection, preserving those of larger dimensions (such as albumin characterized by a molecular weight of 70 kDa): in particular, CPA and PFO have a molecular weight of 42.5 kDa and 53 kDa, respectively, placing them above the cut-off of standard membrane pores, but hemofilters can absorb and retain larger molecules [4, 6, 8]. Finally, regarding direct effects on the hemolysis products, CRRT techniques could have a role because of their ability to filter molecules of small-medium size: once released in plasma, Hb exists in a dynamic equilibrium of tetramer and αβ-subunit heterodimers with a dimension of 32 kDa, so hemofilters could eliminate them. Furthermore, renal replacement techniques can also serve as immune-modulatory treatment, facilitating the clearance of waste products and inflammatory cytokines [20].
In our case, we used the oXiris® system: this modified AN69ST membrane consists of multi-layer AN69 (polyacrylonitrile) grafted with a highly positively charged polycationic polymer and subsequently heparin-coated to reduce thrombogenesis and improve the lifespan end efficiency of the filter. The device has a high adsorptive affinity for peptides and proteins through ionic binding and hydrophilic interactions and can simultaneously provide renal replacement therapy, endotoxin removal, and cytokines absorption [2, 21].
Extracorporeal blood purification techniques (EBPT) are now an established adjunctive therapy for septic shock and have a strong therapeutic rationale. Currently, several devices with cytokine removal and immune modulation aims are available, though favorable data regarding improvement of hemodynamics and survival are based on limited studies and specific devices can lack removal of specific molecules [4, 6]. At present, no large studies have explored the role of oXiris® in septic shock and in general to demonstrate a survival benefit of blood purification treatments, the reason why SSC guidelines cannot recommend hemoperfusion techniques and express against polymyxin B albeit with a low-grade recommendation, but experts could not make recommendations on other blood purification techniques [5, 11, 12].
Antimicrobial therapy is crucial in C. perfringens sepsis: it can significantly reduce mortality, and it is well-known that hemofilter membranes can retain several antibiotics, namely hydrophilic ones like β-lactams. Currently, no definite guidelines exist for antibiotic dosage adjustments during CRRT treatments. Consequently, regular TDM is a useful tool for guiding antimicrobial dose variations for evolving septic patients with acute kidney injury (AKI) [5, 8, 13, 15].
The renal replacement technique utilized in our case, CVVHDF, also included high volumes of fluid exchange: effluent rates above 50 ml/kg/h were chosen in view of the pathophysiological features of this condition [4]. If ultrafiltration flows of about 35 mL/Kg/h (so-called standard volume hemofiltration-SVHF) are used in the routine renal function replacement setting, there’s not yet agreement in the literature on the possible use of higher flow methods (so-called high-volume hemofiltration, HVHF) in sepsis scenario, due to the risk of a proportional increase in loss of beneficial molecules such as nutrients, proteins, and antimicrobials. High-volume hemofiltration (HVHF) is defined when a convective dose > 35 mL/kg/h is used. This technique failed to demonstrate a survival benefit, even though it induced hemodynamic and organ perfusion improvements, so SSC 2021 recommended against high-volume CRRT in standard treatment of septic shock patients with AKI; nonetheless, the two techniques combined together might be useful in specific settings that are not included in large trials; and this could be the case with C. perfringens-induced hemolysis [4, 5]. Further research is warranted to define the subtypes of patients or pathogen-related syndromes that could benefit from these techniques [20].
References
Simon TG, Bradley J, Jones A, et al. Massive intravascular hemolysis from Clostridium perfringens septicemia: a review. J Intensive Care Med. 2014;29:327–33.
Suzaki A, Hayakawa S. Clinical and microbiological features of fulminant haemolysis caused by Clostridium perfringens bacteraemia: unknown pathogenesis. Microorganisms. 2023;11(4):824.
McArthur HL, Dalal BI, Kollmannsberger C. Intravascular hemolysis as a complication of Clostridium perfringens sepsis. J Clin Oncol. 2006;24(15):2387–8.
Navarro MA, McClane BA, Uzal FA. Mechanisms of action and cell death associated with Clostridium perfringens toxins. Toxins (Basel). 2018;10(5):212.
Suzaki A, Ohtani K, Komine-Aizawa S, Matsumoto A, Kamiya S, Hayakawa S. Pathogenic characterization of Clostridium perfringens strains isolated from patients with massive intravascular hemolysis. Front Microbiol. 2021;12: 713509.
Verherstraeten S, Goossens E, Valgaeren B, Pardon B, et al. Perfringolysin O: the underrated Clostridium perfringens toxin? Toxins (Basel). 2015;7(5):1702–21.
Titball RW, Naylor CE, Basak AK. The Clostridium perfringens alpha-toxin. Anaerobe. 1999;5(2):51–64.
Minneci PC, Deans KJ, Zhi H, Yuen PS, et al. Hemolysis-associated endothelial dysfunction mediated by accelerated NO inactivation by decompartmentalized oxyhemoglobin. J Clin Invest. 2005;115(12):3409–17.
Dvanajscak Z, Walker PD, Cossey LN, Messias NC, et al. Hemolysis-associated hemoglobin cast nephropathy results from a range of clinicopathologic disorders. Kidney Int. 2019;96(6):1400–7.
Smit B, van der Helm MW, Bosma M, Hudig F, Russcher H. Massive hemolysis due to Clostridium perfringens: a laboratory’s perspective. Clin Chem Lab Med. 2020;58(11):e295–7.
Rother RP, Bell L, Hillmen P, Gladwin MT. The clinical sequelae of intravascular hemolysis and extracellular plasma hemoglobin: a novel mechanism of human disease. JAMA. 2005;293(13):1653–62.
Cobo F, Pérez-Carrasco V, Martín-Hita L, García-Salcedo JA, Navarro-Marí JM. Comparative evaluation of MALDI-TOF MS and 16S rRNA gene sequencing for the identification of clinically relevant anaerobic bacteria. Crit Eval Discrepant Result Anaerob. 2023;14(82): 102754.
Mitchell SL, Simner PJ. Next-generation sequencing in clinical microbiology: are we there yet? Clin Lab Med. 2019;39(3):405–18.
Kiu R, Hall LJ. An update on the human and animal enteric pathogen Clostridium perfringens. Emerg Microbes Infect. 2018;7(1):141.
Pence MA. Antimicrobial resistance in clinically important anaerobes. Clin Microbio Newsl. 2019;41(1):1–7.
Stevens DL, Maier KA, Mitten JE. Effect of antibiotics on toxin production and viability of Clostridium perfringens. Antimicrob Agent Chemother. 1987;31(2):213–8.
Brook I. Treatment of anaerobic infection. Expert Rev Anti Infect Ther. 2007;5(6):991–1006.
De Rosa S, Marengo M, Fiorentino M, Fanelli V, Brienza N, Fiaccadori E, Grasselli G, Morabito S, Pota V, Romagnoli S, Valente F, Cantaluppi V. SIAARTI-SIN joint commission Extracorporeal blood purification therapies for sepsis-associated acute kidney injury in critically ill patients: expert opinion from the SIAARTI-SIN joint commission. J Nephrol. 2023. https://doi.org/10.1007/s40620-023-01637-5.
Evans L, Rhodes A, Alhazzani W, Antonelli M, et al. Surviving sepsis campaign: international guidelines for management of sepsis and septic shock 2021. Intensiv Care Med. 2021;47(11):1181–247.
Broman ME, Hansson F, Vincent JL, Bodelsson M. Endotoxin and cytokine reducing properties of the oXiris membrane in patients with septic shock: a randomized crossover double-blind study. PLoS ONE. 2019;14(8): e0220444.
Cucchiari D, Reverter E, Blasco M, Molina-Andujar A, et al. High cut-off membrane for in-vivo dialysis of free plasma hemoglobin in a patient with massive hemolysis. BMC Nephrol. 2018;19:250.
Funding
The authors declare that this work received no funding.
Author information
Authors and Affiliations
Corresponding author
Ethics declarations
Conflict of interest
All the authors have declared no competing interest.
Informed consent
Informed consent for storing and sharing clinical data was obtained from the patient.
Additional information
Publisher's Note
Springer Nature remains neutral with regard to jurisdictional claims in published maps and institutional affiliations.
About this article
Cite this article
Reffo, I., Domini, M., Cevolani, M. et al. Clostridium perfringens-induced massive hemolysis treatment with blood purification to target toxins: a case report. CEN Case Rep (2024). https://doi.org/10.1007/s13730-024-00857-3
Received:
Accepted:
Published:
DOI: https://doi.org/10.1007/s13730-024-00857-3