Abstract
In this study, drying of grape (Vitis vinifera) in isothermal and non-isothermal conditions has been done with the newly improved proportional integral derivative (PID) system. The average energy efficiency has been calculated in the processes in which the grapes are dried is 53.4% in the isothermal PID system, 59.7% in the non-isothermal PID system, and 30.5% in the tray dryer (forced convection). To maximum exergy efficiency in the tray dryer, the experimental optimization is made according to the response surface methodology (RSM). In the RSM design, the results have been evaluated by working at different airflow rates (1.5 m/s, 2.2 m/s, 2.9 m/s) and different temperatures (298 K, 308 K, and 318 K). In natural conditions, the drying of grapes took approximately 8 days in the sun and 11 days in the shade. A new shrinkage model has been improved based on the transformation rate, considering the drying behavior of grape grains. The consistency of the obtained model equation with the experimental data has been determined with the help of statistical analysis (R2 0.9987, SST 0.0098). Moreover, when the diffusion behavior of grapes has been investigated, it is determined that both temperature and airflow rate increase the effective diffusion coefficient in the tray dryer. The maximum effective diffusion coefficient in the tray dryer is 2.11·109 m2/s at a temperature of 318 K and an airflow rate of 2.9 m/s.
Highlights
• According to the exergy results, the efficiency has been found to be maximum in the non-isothermal drying performed with the new improved system.
• A new model has been improved based on the conversion rate of shrinkage diameter for grape grains during drying.
• To obtain maximum efficiency in the tray dryer, the experimental design of the airflow rate and temperature has been evaluated by RSM optimization.
•The effective diffusion coefficient of grape has been compared in the tray dryer under the different conditions.
Graphical abstract

Similar content being viewed by others
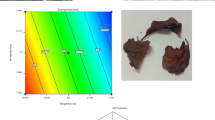
Avoid common mistakes on your manuscript.
1 Introduction
Many natural and artificial processes related to the drying of foods have been developed in the literature. Natural processes are generally designed using solar energy and the drying process is carried out without losing the nutritional value of foods. Using Fick’s law, the effective diffusion values of the air have been found between 1.93·10−11 and 9.16·10−11 m2/s in which grape leather in different thicknesses was used for sun drying [1]. After the food is harvested, it is requested that the moisture in its structure be preserved by drying in the sun should drop below 20% [2]. The most efficient conditions for sun drying in an economical method are that the air is dry, breezy, and at a minimum temperature of 86 °C [3]. This process can contribute to the sun exposure of nutrients, removing most of the water from them, and thus their preservation. However, since it has been observed that drying by exposure to direct sunlight has many disadvantages, systems have been developed for indirectly sun drying and drying food [4]. Systems developed using direct, indirect, and mixed methods in solar-powered dryers are becoming widespread [5]. In indirect drying, the food to be dried is exposed to the open air for several days to reach the desired moisture content. In the indirect solar drying method, the energy gained by the system is used to heat the flowing air for the product to be dried [6]. To improve energy efficiency in food drying technologies, hybrid systems have been developed to increase energy efficiency [7].
Advances in drying technologies have enabled foods to be preserved without losing their nutritional value by economical methods [8]. Controlling the drying process is very important to increase the safety, quality, and efficiency of the food and to minimize the production cost [9]. Zeolites have been used in the food industry to increase drying efficiency. When used in the dehumidified air-drying process with zeolite, 10–18% more efficient energy results were obtained between 52 and 70 °C compared to conventional dryers [10].
In a study, the effect of drying methods on essential oil ratios of fresh lemongrass (2.86%), oven-dried (2.45%), shade-dried (2.12%), and sun-dried (2.10%) samples were compared. Lemongrass has been dried in the sun (36 h), in the shade (48 h), and in the oven (7 h) at 45 ° C with three different drying methods [11]. In another investigating of the effect of drying efficiency on essential oil ratio, Satureja Hortensis plant was dried using three different drying methods (sun, shade, and oven drying at 45 °C). GC-MS analysis showed that there was no significant difference in oil yield of the shade-dried (0.94%), sun-dried (0.87%), and oven-dried (1.06%) samples [12].
In a study for seaweed, the performance of a sun dryer designed with natural convection was compared with drying in the shade. Solar drying was done in 6 days (20.45% moisture) and shade drying in 9 days (3.78% moisture). Both error functions and correlation coefficients have been calculated by statistical analysis using the kinetic models of Linear, Lewis, Henderson, and Pabis [13]. The drying behavior of Vernonia amygdalina leaves was investigated by drying in the open sun with shade and drying kinetics were modeled by statistical approaches. According to the Midilli and Kucuk models, V. amygdalina leaves dried in open sun and shade were calculated of R values 0.99951 and 0.99981, RMSE values 0.00243 and 0.00253, and chi-square values 0.000511 and 0.000428, respectively. In addition, the effective diffusion coefficient was calculated to be 26.58·10−10 m2/s in open sun drying and 52.77·10−11 m2/s in shade drying [14]. It has been observed that drying the leaves in the shade gives better results as photochemical effects cause deformation in leaf morphology in open sun drying [14, 15].
In recent years, exergy analysis and optimization of systems have been carried out in drying processes [16]. Freeze-drying was investigated in a study that analyzed the exergy losses in the drying process and created the mathematical model and evaluated the distribution of exergy losses. According to the results, when the temperature of the cooling source was reduced from −75 to −25 °C, the energy loss per unit mass of the system concerning the moist basis decreased from 1409 to 604 kJ [17]. Using the first and second principles of thermodynamics, the useful energy used during drying can be estimated. The main purpose of the drying process is to evaluate the optimum conditions in minimum time and minimum energy [18]. The efficiency and performance of the drying systems should be evaluated according to the results obtained from the exergy analysis. Hence, it has been observed that exergy can play an important role in ensuring sustainable and economical energy use [19].
In this research, different drying techniques were used for the use of the grape type (V. vinifera) grown in the Eastern Anatolia region. The drying behavior of the grapes dried in sun, shade, tray dryer, and PID system were investigated under isothermal and non-isothermal conditions. Exergy analysis can be performed easily with the new PID system, and it is ensured that the grapes have been dried in a short time, with minimum cost and high efficiency.
Moreover, both a local product (grape) has been introduced and the drying techniques of the product with different processes are compared. Since the shelf life of grape, which has a large commercial market, can be extended by drying, efficient, and economical methods have been evaluated. In this research, the applicability is very high because the experimental studies are suitable for real conditions.
2 Materials and methods
2.1 Materials
The grape type (Fig. 1), scientific name Vitis vinifera, grown in the eastern Anatolia region was collected in Arapgir town (Malatya) and prepared for drying. The local grape (V. vinifera) grown in Elazığ and Malatya regions (Turkey) is used especially in the wine factory and food sector. The annual average harvest of grapes, which is one of the important livelihoods of the region, is close to 110 thousand tons (Turkish Statistical Institute, 2020).
2.2 Methods used in the study
The grape harvest in autumn has been collected and prepared for drying. The drying kinetics of the grapes has been investigated in the sun, shade, tray dryer, isothermal, and non-isothermal systems. Firstly, the drying behavior of the grapes in the natural sun environment has been investigated. On average, the entire surface (360°) of grape grains of a certain size was dried at equal time intervals for about 2 weeks. Under laboratory conditions, it has been dried in the shade for 16 days without exposure to direct sunlight. In the newly improved system, the drying process was carried out by isothermal and non-isothermal conditions at certain temperatures. The grape has been dried under isothermal conditions at the temperature of 344 K until it lost 80% of its initial mass. The initial moisture of the grapes (based on dry mass) is approximately 7% to 7.5% at room temperature. Besides, grape-drying kinetics were studied under non-isothermal conditions in the PID system with a certain temperature increase rate. This drying process, which started at 293 K, was carried out gradually with a heating rate of 0.15 K/min. The shrinkage behavior of the grape has been modeled with the newly developed equation in isothermal and non-isothermal conditions considering the volumetric and superficial shape changes in the structure. Besides, parameters affecting the drying behavior of the grape with forced convection (tray dryer) were determined using the response surface methodology (RSM). According to the conditions determined in the RSM method, an experimental study plan (Table 1) was made with airflow velocities between 1.5 and 2.9 m/s and temperature between 318 and 298 K. In the drying system shown in Fig. 2, the electricity consumed (kWh), the voltage (V) supplied to the system, the power (kW) of the system, and the approximate insulation losses can be calculated. In the PID system, high sensitivity temperature and mass losses can be measured, electrical energy entering the system, insulation, and other losses can be approximately calculated. A certain amount of sample (1 g) can be taken and the temperature can be easily distributed on all surfaces to the conductive and porous cell. The PID system reactor has a total diameter of 19.5 cm and a height of 21.5 cm and is made of perlite-reinforced insulated mortar (5 cm thick), rock wool (1.5 cm thick), and aluminum plate (1 mm thick). The drying cell was placed in the cylindrical space (approximately inner diameter = 6 cm) in the inner center of the reactor. The cylindrical drying cell has a diameter of 3.5 cm, a height of 3 cm, and a porous side surface area (about d = 1–5 mm). The drying cell made of galvanized steel plate (1 mm thick) is placed in the center of the system reactor. In this system, temperatures can be controlled very precisely with the help of thermocouples. For thermogravimetric measurements, the foot of the drying cell only comes into contact with the sensitive mass scale (0.001 g). Temperature control can operate with 0.1 K precision in the programmable PID system and the data can be transferred to the computer.
2.3 Exergy analysis
Exergy analysis of grapes can be performed under isothermal and non-isothermal conditions in the PID system. Exergy efficiency can be determined since the energy entering the system, the energy lost from the system, and the energy consumed can be calculated approximately. In the process according to the second law of thermodynamics, the exergy changes and efficiency analyzed at steady state are given in Eq. 1 and 2 [20,21,22,23]. In the equations below, the characteristics of the air entering (in) the system and the air leaving (out) the system are indicated. Also, temperature (T), exergy (Ex), and specific heat capacity (Cp) have been expressed with symbols in the equations.
The exergy input and exergy output of the air in the tray dryer is given in Eq. (3) and (4) [24].
2.4 Effective diffusion coefficient
In Eq. (5) and (6), Fick’s diffusion model improved for solids with spherical geometry, and the simplified inference by making the necessary assumptions are shown, respectively [25, 26].
In Eq. (7), the change of effective diffusivity with temperature is shown by the Arrhenius relationship [27].
2.5 Shrinkage model
The volume of the spherical structure was obtained by scanning through the three-dimensional printer before and after drying. Shrinkage diameter (DR) has been determined in the new improved model with the obtained results. Since the grains shrink and move away from the spherical geometry after drying, their volumes were calculated with the help of a three-dimensional scanner. When the experimental data were compared with the model equation with the help of statistical analysis, R2=0.9987, SST=0.0098 (total sum of squares), and χ2=0.025 (chi-square) values were found. In Eq. (8) and Eq. (9), α is the conversion ratio, β is the spherical shrinkage coefficient, M is the mass of the sample, and ξ is the specific correction factor. In addition, some symbols indicate that is the initial (i), the final (f), and any time (t) in the models.
The experimental data have been recorded by measuring the average diameter of the grape before and after the drying process. These calculations were repeated for five different grape grains and the theoretically developed model was confirmed in the equation. With statistical analysis, correlation coefficients, R2 values, error functions were evaluated, and experimental and theoretical results were compared. To determine the exact spherical shrinkage diameter in grape grains, all surfaces must be at equal temperature during drying. Non-linear regression analysis is needed to evaluate the parameters of the selected model. Since the moisture permeability of the waxy skin of the grape is very low, drying the grains is a slow and energy-intensive process. Shrinkage during drying plays an important role in determining the accuracy of the drying pattern. The experimental conditions should be optimized by examining the drying kinetics, moisture diffusion, quality change, and shrinkage behavior [28,29,30,31,32,33].
3 Result and discussion
3.1 Sun drying
Sun drying of food with traditional methods is the oldest and most economical method. However, modified systems using solar energy have been developed to dry foods effectively and in a short time without losing their nutritional value [34, 35]. In this research, the drying behavior of grape grains was investigated in direct sunlight in the open air. In Fig. 3, the drying curve of the grapes is shown according to the average day and night temperature values of the air. When the drying curve is examined, it is determined that the slope of the curve decreases in both days and slows down very much after the sixth day. The decrease in moisture loss after the sixth day of drying in the sun is because the grape grains gradually reduce on the surface and near the surface. In the drying conditions where both heat transfer and the mass transfer took place, the moisture in the center of the grains occurred with a slower mass transfer rate. In other words, after about the sixth day, the drying behavior of grapes can be expressed as a decreasing speed region [34,35,36].
3.2 Shade drying
In Fig. 4, the curve of drying in the shade without exposure to direct sunlight in the laboratory is given. Drying speed in shade drying conditions is slow and since direct sunlight has no harmful effects, both shrinkage and nutritional values of grapes are affected less [31].
3.3 Isothermal and non-isothermal drying with the PID system
The grape was dried at 344 K under isothermal conditions with the improved PID drying system. In non-isothermal conditions, starting from room temperature, the drying process was carried out in the PID system with a temperature increase of 0.15 K/min. It can be seen in Fig. 5 that the drying rate of grapes under isothermal conditions is higher than that of non-isothermal drying in Fig. 6. Also, the drying time of grapes in the isothermal condition is faster than drying in the non-isothermal condition. According to the results obtained from the exergy analysis in the PID system, the exergy efficiency in the isothermal drying was approximately 53.4%, while it was 59.7% in non-isothermal conditions. Since the drying temperature of the grapes is both high and constant under isothermal conditions, the maximum drying rate and minimum drying time were realized. In non-isothermal drying, on the other hand, starting from room temperature, a gradual increase in temperature was achieved with the help of the PID system. Since the temperature in the isothermal is reached after about 3 h, the mass transfer rate is lower than isothermal drying with the effect of temperature.
3.4 Response surface methodology
In this study, an experimental design was made with statistical regression according to RSM method and optimum conditions were determined according to response functions. In experimental design, it is necessary to determine the effective parameters to find optimum results with a small number of experiments. The response functions of these parameters in the process are evaluated statistically in a fast, reliable way, and a good solution is reached. According to the RSM method, the most appropriate results are obtained in the most economical, short time, and with maximum efficiency by consuming less energy [37].
Experimental design, regression modeling, and optimization processes were evaluated using RSM with the help of statistical analysis. Statistical analysis was performed with RSM to obtain the minimum number of experiments and maximum effective efficiency. In the experiments, optimum results were obtained by evaluating the response functions of the independent variables. First, the independent variables and their range of change were defined, and then the experimental results obtained were entered into the RSM as a response function. The results were evaluated by statistical analysis and both optimum experimental conditions and optimum mixing ratios were determined [38, 39]. RSM model in Fig. 7 shows the drying time results found in the tray dryer for different temperatures and airflow rates.
In the verification experiments, the airflow rate was 2.2 m/s and the temperature was taken as 308 K, and it was repeated five times. According to the experimental data, drying times were found to be approximately 291, 293, 291, 295, and 290 min. In the RSM method, the results have been expressed in the lowest 288.897 min. and the highest 295.126 min. at 95% confidence interval. With the statistical analysis (standard deviation, 2.0826), it was seen that the experimental data and the theoretical results were consistent.
When the RSM results in Table 2 are compared with the models suggested in the tray dryer, it is determined by statistical analysis that the experimental data are more compatible with natural log.
In Eq. 10, the drying time (tdrying: min.), drying temperature (T: K), and airflow rate (v: m/s) have been expressed in the RSM model equation.
According to RSM results, optimum experimental conditions are 2.2 m/s air velocity, 308 K temperature, and 306 min. 51 seconds drying time.
RSM results in Fig. 8, the distribution of the experimental data and the model data were statistically compared. The data obtained in the experimental study plan in RSM design according to the central composite method were evaluated by statistical analysis. In the central region, the distribution of errors, large deviations, and small deviations have been determined, and the harmony between the actual and estimated values is evaluated. According to the statistical parameters, the significance (p-value = 0.0001) is small, the R2 value is close to 1, and the error functions such as SST are close to 0 expresses that the reliability between the experimental and the theoretical model [39,40,41,42].
3.5 Exergy analysis results
Exergy efficiency has come to the fore in the drying of food, as in every field. The energy values entering, leaving, and lost in the system during the drying of grapes directly affect the exergy efficiency. It has been observed that the energy costs of processes with very low exergy efficiency are also very high [43,44,45,46,47,48]. In this study, exergy analysis of grapes, electrical energy (kWh) entering the proportional integral derivative (PID) system have been controlled. The lost energy in the laboratory conditions is calculated approximately by examining the controlled (3 times) experiments without drying in the system. The energy consumed in the drying process can be calculated approximately after removing the energy lost from the system from the energy entering the system. However, it is understood that most of the energy used during drying is given back to the environment. While calculating the exergy efficiency, all these parameters and system losses were taken into consideration and exergy analysis was made. The approximate energy efficiency for the processes in which the grapes are dried has been calculated as 53.4% in the isothermal system, 59.7% in the non-isothermal system, and 30.5% in the tray dryer with forced convection [21].
3.6 Effective diffusion coefficient results
The effective diffusion coefficient was calculated in the range of 1×10−10 to 7×10−8 in other studies in which the drying process of grapes with convective air was performed. In convective drying, the type of grape, the drying surface (thin or thick skin), and the speed and temperature of the air directly affect the diffusion coefficient. Effective diffusion coefficient values calculated for the grape grain at the different temperatures and airflow rates are given in Table 3. Average effective diffusion coefficients were determined for each grape grain in spherical shape in the constant drying region under certain conditions. The spherical grape grains prepared for the drying process have been separated from their stems, cleaned, and placed in the drying system and the data were recorded. Effective diffusion coefficients of grape grains of approximately the same size have been calculated with the help of the models in Eq. (6) and (7) [49, 50]. Convective air-dried, the grape grains in a shorter time at high speeds and temperatures. It is also seen in the experimental work plan in Table 1 that the increase in airflow velocity and temperature decreases the drying time. The decrease in the drying time also means an increase in the diffusion rate. Besides, when Table 3 is examined carefully, it can be understood that the convective airflow velocity reduces the drying time more effectively than the temperature. When Table 3 is examined, it is seen that both temperature and airflow rate increase the diffusion coefficient. The highest effective diffusion coefficient has been calculated as 2.11×10−9 m2/s at 318 K temperature and 2.9 m/s airflow velocity.
4 Conclusion
In this study, exergy efficiency has been made by comparing different dryers and drying mechanisms for the drying grapes. It has been observed that grape grains dried naturally (sun and shade) take a long time. The energy losses in the drying processes have been evaluated and the method with the highest exergy efficiency is determined.
The average energy efficiency for grapes dried by different methods is found at 53.4% in the isothermal PID system, 59.7% in the non-isothermal PID system, and 30.5% in the tray dryer.
The suitability of the newly improved model for the grapes in the tray dryer to the experimental data (R2 0.9987, SST 0.0098) has been determined with the help of statistical analysis. Also, it has been determined that the effective diffusion coefficient in the tray dryer (forced convection) increases with both temperature and airflow rate. The highest effective diffusion coefficient is calculated as 2.11×109 m2/s at 318 K temperature and 2.9 m/s air flow rate.
Heating of the air, sending it to the system at high speed, discharging it from the system in a very short time, and insulation losses can cause large energy consumption (kWh). However, it was preferred in the tray dryer due to its low drying time and drying temperature.
Since the volatile components in the grape are gradually diffused from the surface by natural convection, the PID system has become advantageous because the color change, shrinkage, and deformation in the structure are less. Drying of grapes under natural conditions took about a week in the sun and 11 days in the shade.
References
Maskan A, Kaya S, Maskan M (2002) Hot air and sun drying of grape leather (pestil). J Food Eng 54(1):81–88. https://doi.org/10.1016/S0260-8774(01)00188-1
Green MG, Schwarz D (2001) Solar drying technology for food preservation. Energy 49(0):1–8
Ahmed N, Singh J, Chauhan H, Gupta P, Anjum A, Kour H (2013) Different drying methods: their applications and recent advances. Int J Food Nut Safety 4(1):34–42 www.ModernScientificPress.com/Journals/IJFNS.aspx
Guiné RPF (2018) The drying of foods and its effect on the physical-chemical, sensorial and nutritional properties. ETP Int J Food Eng 4(2):93–100. https://doi.org/10.18178/ijfe.4.2.93-100
El-Sebaii AA, Shalaby SM (2012) Solar drying of agricultural products: a review. Renew Sust Energ Rev 16(1):37–43. https://doi.org/10.1016/j.rser.2011.07.134
Maisnam D, Rasane P, Dey A, Kaur S, Sarma C (2017) Recent advances in conventional drying of foods : a review recent advances in conventional drying of foods. J Food Technol Pres 1(1):25–34
Menon A, Stojceska V, Tassou SA (2020) A systematic review on the recent advances of the energy efficiency improvements in non-conventional food drying technologies. Trends Food Sci Technol 100(June 2019):67–76. https://doi.org/10.1016/j.tifs.2020.03.014
Ratti C (2001) Hot air and freeze-drying of high-value foods: a review. J Food Eng 49(4):311–319. https://doi.org/10.1016/S0260-8774(00)00228-4
Sun Q, Zhang M, Mujumdar AS (2019) Recent developments of artificial intelligence in drying of fresh food: a review. Crit Rev Food Sci Nutr 59(14):2258–2275. https://doi.org/10.1080/10408398.2018.1446900
Djaeni M, Bartels P, Sanders J, van Straten G, van Boxtel AJB (2007) Process integration for food drying with air dehumidified by zeolites. Dry Technol 25(1):225–239. https://doi.org/10.1080/07373930601161096
Mohamed Hanaa AR, Sallam YI, El-Leithy AS, Aly SE (2012) Lemongrass (Cymbopogon citratus) essential oil as affected by drying methods. Ann Agric Sci 57(2):113–116. https://doi.org/10.1016/j.aoas.2012.08.004
Sefidkon F, Abbasi K, Khaniki GB (2006) Influence of drying and extraction methods on yield and chemical composition of the essential oil of Satureja hortensis. Food Chem 99(1):19–23. https://doi.org/10.1016/j.foodchem.2005.07.026
Phang H, Chu C, Kumaresan S, Rahman M, Yasir S (2015) Preliminary study of seaweed drying under a shade and in a natural draft solar dryer. Int J Sci Eng 8(1):10–14. https://doi.org/10.12777/ijse.8.1.10-14
Alara OR, Abdurahman NH, Abdul Mudalip SK, Olalere OA (2018) Mathematical modeling of thin layer drying using open sun and shade of Vernonia amygdalina leaves. Agric Nat Resourc 52(1):53–58. https://doi.org/10.1016/j.anres.2018.05.013
Omidbaigi R, Sefidkon F, Kazemi F (2004) Influence of drying methods on the essential oil content and composition of Roman chamomile. Flav Fragrance J 19(3):196–198. https://doi.org/10.1002/ffj.1340
Aghbashlo M, Mobli H, Rafiee S, Madadlou A (2013) A review on exergy analysis of drying processes and systems. Renew Sust Energ Rev 22:1–22. https://doi.org/10.1016/j.rser.2013.01.015
Liu Y, Zhao Y, Feng X (2008) Exergy analysis for a freeze-drying process. Appl Therm Eng 28(7):675–690. https://doi.org/10.1016/j.applthermaleng.2007.06.004
Boulemtafes-Boukadoum A, Benzaoui A (2011) Energy and exergy analysis of solar drying process of mint. Energy Procedia 6:583–591. https://doi.org/10.1016/j.egypro.2011.05.067
Dincer I (2011) Exergy as a potential tool for sustainable drying systems. Sustain Cities Soc 1(2):91–96. https://doi.org/10.1016/j.scs.2011.04.001
Tagnamas Z, Lamsyehe H, Moussaoui H, Bahammou Y, Kouhila M, Idlimam A, Lamharrar A (2021) Energy and exergy analyses of carob pulp drying system based on a solar collector. Renew Energy 163:495–503. https://doi.org/10.1016/j.renene.2020.09.011
Dolgun EC, Karaca G, Aktaş M (2020) Performance analysis of infrared film drying of grape pomace using energy and exergy methodology. Int Commun Heat Mass Transf 118(August):104827. https://doi.org/10.1016/j.icheatmasstransfer.2020.104827
DOLGUN GK, AKTAŞ M, Can DOLGUN, E. (2021) Infrared convective drying of walnut with energy-exergy perspective. J Food Eng 306(December 2020):110638. https://doi.org/10.1016/j.jfoodeng.2021.110638
Midilli A, Kucuk H (2003) Energy and exergy analyses of solar drying process of pistachio. Energy 28(6):539–556. https://doi.org/10.1016/S0360-5442(02)00158-5
Yiotis AG, Boudouvis AG, Stubos AK, Tsimpanogiannis IN, Yortsos YC (2003) Effect of liquid films on the isothermal drying of porous media. Phys Rev E Stat Phys Plasmas Fluids Relat Interdiscip Topics 68(3):4. https://doi.org/10.1103/PhysRevE.68.037303
Srikiatden J, Roberts JS (2006) Measuring moisture diffusivity of potato and carrot (core and cortex) during convective hot air and isothermal drying. J Food Eng 74(1):143–152. https://doi.org/10.1016/j.jfoodeng.2005.02.026
Panda D, Supriya B, Kharaghani A, Tsotsas E, Surasani VK (2020) Lattice Boltzmann simulations for micro-macro interactions during isothermal drying of bundle of capillaries. Chem Eng Sci 220:1–18. https://doi.org/10.1016/j.ces.2020.115634
Metzger T, Irawan A, Tsotsas E (2007) Isothermal drying of pore networks: influence of friction for different pore structures. Dry Technol 25(1):49–57. https://doi.org/10.1080/07373930601152640
Adiletta G, Russo P, Senadeera W, Di Matteo M (2016) Drying characteristics and quality of grape under physical pretreatment. J Food Eng 172:9–18. https://doi.org/10.1016/j.jfoodeng.2015.06.031
Behroozi Khazaei N, Tavakoli T, Ghassemian H, Khoshtaghaza MH, Banakar A (2013) Applied machine vision and artificial neural network for modeling and controlling of the grape drying process. Comput Electron Agric 98:205–213. https://doi.org/10.1016/j.compag.2013.08.010
Senadeera W, Adilettta G, Di Matteo M, Russo P (2014) Drying kinetics, quality changes, and shrinkage of two grape varieties of Italy. Appl Mech Mater 553:362–366. https://doi.org/10.4028/www.scientific.net/AMM.553.362
Esmaiili M, Sotudeh-Gharebagh R, Cronin K, Mousavi MAE, Rezazadeh G (2007) Grape drying: a review. Food Rev Int 23(3):257–280. https://doi.org/10.1080/87559120701418335
Mayor L, Sereno AM (2004) Modelling shrinkage during convective drying of food materials: a review. J Food Eng 61(3):373–386. https://doi.org/10.1016/S0260-8774(03)00144-4
Mia MM (2013) Archi and of Archi of 3(2):829–837
Fadhel A, Kooli S, Farhat A, Bellghith A (2005) Study of the solar drying of grapes by three different processes. Desalination 185(1–3):535–541. https://doi.org/10.1016/j.desal.2005.05.012
Essalhi H, Benchrifa M, Tadili R, Bargach MN (2018) Experimental and theoretical analysis of drying grapes under an indirect solar dryer and in open sun. Innov Food Sci Emerg Technol 49(August):58–64. https://doi.org/10.1016/j.ifset.2018.08.002
Riva M, Peri C (1986) Kinetics of sun and air drying of different varieties of seedless grapes. Int J Food Sci Technol 21(5):199–208. https://doi.org/10.1111/j.1365-2621.1986.tb00441.x
Aghbashlo M, Kianmehr MH, Nazghelichi T, Rafiee S (2011) Optimization of an artificial neural network topology for predicting drying kinetics of carrot cubes using combined response surface and genetic algorithm. Dry Technol 29(7):770–779. https://doi.org/10.1080/07373937.2010.538819
Ghafoor K, Park J, Choi YH (2010) Optimization of supercritical fluid extraction of bioactive compounds from grape (Vitis labrusca B.) peel by using response surface methodology. Innov Food Sci Emerg Technol 11(3):485–490. https://doi.org/10.1016/j.ifset.2010.01.013
Ravula SR, Munagala SR, Arepally D, Ravula PR, Golla S (2017) Mathematical modelling and estimation of effective moisture diffusivity and activation energy, exergy analysis of thin layer drying of pineapple. J Exp Biol Agric Sci 5(2):390–401. https://doi.org/10.18006/2017.5(3).392.401
Wang G, Deng Y, Xu X, He X, Zhao Y, Zou Y, Liu Z, Yue J (2016) Optimization of air jet impingement drying of okara using response surface methodology. Food Control 59:743–749. https://doi.org/10.1016/j.foodcont.2015.06.047
Panwar NL, Kaushik SC, Kothari S (2012) A review on energy and exergy analysis of solar drying systems. Renew Sust Energ Rev 16(5):2812–2819. https://doi.org/10.1016/j.rser.2012.02.053
Domínguez-Perles R, Teixeira AI, Rosa E, Barros AI (2014) Assessment of (poly)phenols in grape (Vitis vinifera L.) stems by using food/pharma industry compatible solvents and response surface methodology. Food Chem 164:339–346. https://doi.org/10.1016/j.foodchem.2014.05.020
Ghafoor K (2009) Optimization of ultrasound assisted extraction of phenolic compounds and antioxidants from grape peel through response surface methodology. J Korean Soc Appl Biol Chem 52(3):295–300. https://doi.org/10.3839/jksabc.2009.052
Karacabey E, Mazza G (2010) Optimization of antioxidant activity of grape cane extracts using response surface methodology. Food Chem 119(1):343–348. https://doi.org/10.1016/j.foodchem.2009.06.029
Chandramohan VP (2020) Convective drying of food materials: an overview with fundamental aspect, recent developments, and summary. Heat Transf - Asian Res September. https://doi.org/10.1002/htj.21662
Corzo O, Bracho N, Alvarez C (2008) Water effective diffusion coefficient of mango slices at different maturity stages during air drying. J Food Eng 87(4):479–484. https://doi.org/10.1016/j.jfoodeng.2007.12.025
Ahmet Tütüncü M, Labuza TP (1996) Effect of geometry on the effective moisture transfer diffusion coefficient. J Food Eng 30(3–4):433–447. https://doi.org/10.1016/s0260-8774(96)00028-3
Golestani R, Raisi A, Aroujalian A (2013) Mathematical modeling on air drying of apples considering shrinkage and variable diffusion coefficient. Dry Technol 31(1):40–51. https://doi.org/10.1080/07373937.2012.714826
Noshad M, Ghasemi P (2020) Influence of freezing pretreatments on kinetics of convective air-drying and quality of grapes. Food Biosci 38(September):100763. https://doi.org/10.1016/j.fbio.2020.100763
Miraei Ashtiani SH, Rafiee M, Mohebi Morad M, Khojastehpour M, Khani MR, Rohani A, Shokri B, Martynenko A (2020) Impact of gliding arc plasma pretreatment on drying efficiency and physicochemical properties of grape. Innov Food Sci Emerg Technol 63(March):102381. https://doi.org/10.1016/j.ifset.2020.102381
Author information
Authors and Affiliations
Corresponding author
Additional information
Publisher’s note
Springer Nature remains neutral with regard to jurisdictional claims in published maps and institutional affiliations.
Rights and permissions
About this article
Cite this article
Aydoğmuş, E., Demirpolat, A.B. & Arslanoğlu, H. Isothermal and non-isothermal drying behavior for grape (Vitis vinifera) by new improved system: exergy analysis, RSM, and modeling. Biomass Conv. Bioref. 12, 527–536 (2022). https://doi.org/10.1007/s13399-021-02034-3
Received:
Revised:
Accepted:
Published:
Issue Date:
DOI: https://doi.org/10.1007/s13399-021-02034-3