Abstract
Curculio sikkimensis (Heller) (Coleoptera: Curculionidae) is a serious pest of the Japanese chestnut, Castanea crenata, because the larvae feed inside seeds. Chestnut seeds are usually harvested before the larvae leave them, meaning it is unlikely that the weevil is based in orchards. Instead, adults from neighboring woodlands likely invade orchards to oviposit. In this study, I examined host plant use and the course of development of Cu. sikkimensis in mixed oak and chestnut coppice stands to improve our understanding of weevil infestations in chestnut orchards. Females oviposited into seeds of three Quercus species and Ca. crenata, whose seed maturation times differed, over several months. The phenology of the weevil’s oviposition most closely tracked that of Quercus serrata seed maturation. Mature larvae left the seeds and burrowed into the soil, where they overwintered—in some cases for up to three winters because of prolonged diapause. Adults emerged over the course of 1 month and survived for about 2 months; such traits seem to enable this weevil to reproduce for several months. Thus, Cu. sikkimensis uses several host plants in coppice stands, and its oviposition phenology suggests that it is more dependent on Q. serrata than on Ca. crenata.
Similar content being viewed by others
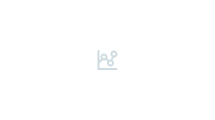
Avoid common mistakes on your manuscript.
Introduction
Curculio sikkimensis (Heller) (Coleoptera: Curculionidae) is an important pest of the Japanese chestnut, Castanea crenata Sieb. et Zucc. (Kimura 1971; Okabe and Takaeda 1993). This weevil is widely distributed in Japan, Korea, China, and India (Hayashi et al. 1984). Adults lay eggs into chestnut seeds, inside which the larvae develop. Several studies have investigated the infestation of chestnut and timing of oviposition of Cu. sikkimensis to determine the suitable timing for spraying pesticides in orchards (Kanazaki and Ii 2008; Kimura 1971; Nakagaki and Yanagibashi 1985; Takamura 1971). So far, however, little is known about the how the reproductive phenology and seasonal life cycle of this weevil are related to weevil damage to chestnut seeds. Larvae of Cu. sikkimensis continue to develop in chestnut seeds for several weeks after seed fall, and mature larvae leave the seeds and burrow into the soil, where they overwinter (Nakagaki and Yanagibashi 1985). After overwintering, pupation and adult eclosion occur in the soil, and adults emerge from the soil from summer to autumn (Higaki 2005; Okabe and Takaeda 1993).
In appropriately managed orchards, chestnut seeds are usually harvested soon after seed fall, so chestnut seeds housing Cu. sikkimensis larvae are harvested before the larvae exit them. This leaves little possibility for adult emergence of the chestnut weevil in appropriately managed orchards. Chestnut is a major crop grown in mountainous regions of Japan (Motoki 2008), and therefore, chestnut orchards are often surrounded by woodlands. Curculio sikkimensis is known to use seeds of several species of Quercus in addition to chestnuts as hosts (Fukumoto and Kajimura 1999; Higaki 2016; Maeto and Ozaki 2003; Ueda 2000). Thus, adult weevils that oviposit in chestnut orchards likely originate in the neighboring forests.
To clarify an ecological basis for the efficient control of Cu. sikkimensis, in addition to research based in chestnut orchards, detailed study of the weevil in nearby woodlands is also necessary. Previous studies, however, have not focused on ecological aspects of Cu. sikkimensis, such as host plant use and the seasonal life cycle in woodlands. In other Curculio species, adult females actively select seeds that have reached a developmental stage suitable for oviposition (Espelta et al. 2009; Harris 1976; Higaki 2016). Such specific timing of oviposition during seed growth also has been reported for Cu. sikkimensis in chestnut cultivars (Okabe and Takaeda 1993). It is possible that this weevil uses seeds of several host plants with different seed maturation times sequentially over a long period. However, life-cycle traits such as timing of adult emergence from soil and adult longevity have not been studied in detail.
Under both field and laboratory conditions, adult emergence of Cu. sikkimensis is spread over several years owing to prolonged diapause in the larval stage (Higaki 2005, 2006; Higaki and Toyama 2012; Maeto and Ozaki 2003). However, the seasonal timing of weevil development such as pupation and adult eclosion in soil was examined only under laboratory conditions (Higaki 2005). Thus, more research is needed on the weevil’s course of development and the timing in woodland soils.
In this study, I examined host plant use and the course of development of Cu. sikkimensis in mixed oak and chestnut coppice stands to improve our understanding of weevil infestations in chestnut orchards. First, to assess the use of several host plants by the weevil, I examined weevil infestation of seeds of several host plants with different seed maturation times, and then evaluated the degree of synchronization of weevil oviposition with the seed maturation of hosts. Second, to reveal the course of weevil development, I investigated the seasonal timing of development in soil, variability in life-cycle duration, and adult longevity.
Materials and methods
Study site
This study was conducted in five coppice stands (total area of about 11,000 m2) and a row of roadside trees, all at the Institute of Fruit Tree and Tea Science, National Agriculture and Food Research Organization, Tsukuba, Ibaraki Prefecture, central Japan (36°03′N, 140°08′E, 20 m a.s.l.). Four of the coppice stands consisted mainly of deciduous oaks (Quercus serrata Murray, Quercus acutissima Carruthers), evergreen oak (Quercus myrsinifolia Blume), Japanese chestnut (Ca. crenata), and Japanese red pine (Pinus densiflora Sieb. et Zucc.), and the other was mainly Ca. crenata. The row of roadside trees consisted mainly of Q. myrsinifolia.
Collection of fallen seeds
To assess the seasonal patterns of seed production in the stands, I collected mature seeds that fell from the trees from August to January in 2002–2003 and 2004–2005. During that period, seeds that fell from 53 Ca. crenata trees onto mowed ground were collected every day. Seeds were collected every day from vinyl sheets (5.4 × 3.6 m) laid beneath 7 Q. serrata trees in 2002 (4 sheets) and 12 Q. serrata trees in 2004 (8 sheets) and from beneath 5 Q. myrsinifolia trees (2 sheets in each year) in 2002–2003 and 2004–2005. Seeds that fell from 8 Q. acutissima trees in mid-to-late September were collected at intervals of 5–7 days in 2002, and all seeds that fell from 5 other Q. acutissima trees were collected every day in 2004.
To evaluate weevil infestation and timing of larval emergence from seeds, chestnut seeds were stored in baskets (44 × 33 × 27 cm) covered with polyethylene sheeting on trays and acorns were spread on 5-mm-mesh nylon netting suspended inside covered plastic containers (10.5 cm in height, 15 cm in diameter) at ambient temperature. Weevil larvae that emerged from chestnuts dropped through the basket onto the tray, and those from acorns dropped through the netting into the bottom of the container. Larvae were collected daily. In 2002–2003, the number of larvae that emerged from seeds during a 5- or 6-day period were counted. In 2004–2005, the number of larvae that emerged from seeds that fell during a 5- or 6-day period were counted. To compare the numbers of larval exit holes among hosts, I counted exit holes in seeds of all three species collected in 2004–2005 in the following February or April.
To compare seed sizes among hosts, I measured the fresh seed weight of Q. serrata and Q. myrsinifolia collected in 2004 and that of Ca. crenata collected in 2015 on an electronic balance (AT201, Mettler-Toledo International Inc., Greifensee, Switzerland).
Body size of adults derived from each host
To compare body size of adults derived from different hosts, larvae that emerged from seeds of Ca. crenata, Q. serrata, or Q. myrsinifolia in 2002 were placed in separate glass cylinders (9 cm in height, 9 cm in diameter) filled with moist garden soil made of coconut fiber (P-8L, Iris Ohyama Inc., Sendai, Japan) to a depth of about 8 cm, with 25 larvae in each. Larvae that emerged from seeds of Q. acutissima were kept in plastic containers (10.5 cm in height, 15 cm in diameter) filled with the same soil to a depth of about 9 cm, with 70 larvae in each. All larvae were housed at ambient temperature. The soil was watered when the surface became dry. Adults that emerged from the soil were collected daily in 2003, and each was weighed on the electric balance on the day of emergence.
Development in a coppice stand
To examine the seasonal timing of weevil development in soil, larvae of Cu. sikkimensis were stored under field conditions. Six 50-L pots (36 cm in height, 45 cm top in diameter) were buried in the ground in a coppice stand. Each pot was filled with field soil to a depth of about 30 cm. To prevent entry of mammals and entomophagous insects and the escape of Cu. sikkimensis adults, the top and hole at the bottom of each pot were covered with 1-mm mesh wire gauze.
First, to assess the timing of adult emergence from soil and variability in life-cycle duration, I released larvae that emerged from chestnuts in early October 2001 into four pots, 300 larvae in each, on 6 October 2001. The larvae burrowed into the soil within a day. Larval density was determined according to Ihara et al. (2003); in that study, most larvae (about 87%) survived for at least 6 months. Therefore, I judged that serious interference among larvae would not occur at such a larval density. Adult emergence from the soil was checked daily from May to October for 5 years.
Second, to clarify the timing of pupation and adult eclosion in soil, I placed another group of larvae that emerged from chestnuts in early October 2001 individually into wells (1.7 cm depth, 1.6 cm diameter) of 24-well tissue-culture plates (Multiwell, BD Falcon, Becton Dickinson and Co., Franklin Lakes, NJ, USA) filled with moist garden soil (as above, ~ 7 mm deep), with one larva per well. The plates were held in covered plastic containers (24 × 18 × 8.5 cm) with a plastic vessel filled with distilled water inside each to maintain the relative humidity at close to 100%. One container with five tissue-culture plates was placed in each of two pots at a suitable depth (15–25 cm); previous studies reported that the vertical position of Cu. sikkimensis larvae in soil ranges from ca. 10–25 cm, although soil hardness may influence the depth to which larvae dig (Ihara et al. 2003; Okabe and Takaeda 1993). From 10 May to 31 October in 2002, the containers were dug out every 10 or 11 days, pupation and adult eclosion were assessed, and the containers were reburied. The larvae that remained in diapause on 31 October were considered to be in prolonged diapause.
Rearing adult weevils
To assess the adult longevity of Cu. sikkimensis, adults were reared on commercial diet made for adults of dynastid and lucanid beetles (Mega jelly, Mitani Co. Ltd., Kasumigaura, Japan). Larvae that emerged from seeds of Ca. crenata in 1999 were kept in glass cylinders filled with moist garden soil, held at 5 °C for 120 days, and then transferred to 20 °C. The adults that emerged from the soil were sexed and then reared individually in glass cylinders (9 cm high, 9 cm diameter) on the jelly diet (22 g), which was replaced every 4 days. Another group of adults were reared without diet to compare adult lifespan between feeding and non-feeding conditions. All adults were given water-soaked cotton wool and kept individually to avoid copulation and oviposition. Survival of the adults was assessed until all adults died.
Statistical analysis
Scheffe’s test following one-way ANOVA was used to compare mean seed weights among Ca. crenata, Q. serrata, and Q. myrsinifolia. The Steel–Dwass test was used for multiple comparison of number of larval exit holes per seed among Ca. crenata, Q. serrata, and Q. myrsinifolia. Two-way ANOVA was used to examine the effects of host plant and sex on fresh weights of adult weevils derived from of each host, as well as the effects of food and sex on adult longevity. When there was a significant interaction between two factors, simple main effects of one variable within each level of the other variable were examined by Scheffe’s test or t test. The above statistical analyses were conducted in BellCurve for Excel (Social Survey Research Information Co., Ltd., Tokyo, Japan), which is add-in statistical software.
I estimated the phenology of the amount of weevil oviposition activity to evaluate how well it synchronizes with the phenology of host seeds. I assumed that the oviposition activity of adult Cu. sikkimensis for a specified host species is proportional to the total oviposition activity at that time and also proportional to the total number of seeds of the host species at that time, using the following notation.
-
\({\lambda }_{t}\) = total amount of oviposition activity of adult Cu. sikkimensis at time t,
-
\({x}_{\mathrm{c}t}, {x}_{\mathrm{s}t}, {x}_{\mathrm{m}t}\) = numbers of available seeds of Ca. crenata, Q. serrata, and Q. myrsinifolia, respectively, at time t,
-
\({y}_{\mathrm{c}t}, {y}_{\mathrm{s}t}, {y}_{\mathrm{m}t}\) = observed numbers of attacks on seeds of Ca. crenata, Q. serrata, and Q. myrsinifolia, respectively, at time t,
-
\({w}_{\mathrm{s}},{w}_{\mathrm{m}}\) = relative degrees of preference for seeds of Q. serrata and Q. myrsinifolia, respectively, measured as the quantities relative to that of Ca. crenata.
The observed number of attacks follows a Poisson distribution for a given intensity of oviposition activity. Therefore, the likelihood of observing \({y}_{\mathrm{c}t}\), \({y}_{\mathrm{s}t}\), and \({y}_{\mathrm{m}t}\) at time t, which is denoted by \({L}_{t}\), is given by:
The maximum likelihood estimate (\({\widehat{\lambda }}_{t}\)) of the total amount of oviposition activity at time t is given by solving \(\partial \mathrm{log}\left({L}_{t}\right)/\partial {\lambda }_{t}=0\):
where a hat indicates the estimate.
Let \({\mu }_{t}\) be the probability density function (PDF) of the total amount of oviposition activity, which is estimated by:
where k is the number of observation periods.
To calculate the amount of oviposition activity, the degrees of preference for seeds of Q. serrata and Q. myrsinifolia were estimated by considering the following characteristics of a PDF. An observed PDF generally suffers the influence of sampling errors. Various statistical methods have been proposed to estimate the true PDF by smoothing the observed PDF (e.g., Simonoff 1996). I adopted a discrete version of the smoothing spline method for estimating PDF as follows. The slope of the PDF should not abruptly change with increasing time. That is, the slope of the PDF of adjacent periods, \(\left({\mu }_{t+1}-{\mu }_{t}\right)\) and \(\left({\mu }_{t}-{\mu }_{t-1}\right)\), should not be much different if the true PDF consists of smooth curves. Hence, the sum of squared difference (SSD) between adjacent slopes is minimized as
I applied the above estimation procedure to the data obtained in 2004. The numbers of fallen seeds of Ca. crenata, Q. serrata, and Q. myrsinifolia collected in each 5- or 6-day period were used as \({x}_{\mathrm{c}t}\), \({x}_{\mathrm{s}t}\), \({x}_{\mathrm{m}t}\), respectively. The numbers of larvae exited from these seeds were used as \({y}_{\mathrm{c}t}\), \({y}_{\mathrm{s}t}\), and \({y}_{\mathrm{m}t}\), respectively. Then, I estimated the total amount of oviposition activity at time t (\({\lambda }_{t}\)) and the degrees of preference of seeds (\({w}_{\mathrm{s}}\) and \({w}_{\mathrm{m}}\)) by minimizing the quantity of SSD using Eq. (4) after substituting Eqs. (2) and (3) for Eq. (4).
Results
Host range of Curculio sikkimensis at the study site
Larvae of Cu. sikkimensis emerged from seeds of all four tree species examined in this study. Seeds of Ca. crenata, Q. serrata, and Q. myrsinifolia hosted only Cu. sikkimensis, whereas Q. acutissima seeds hosted some Cu. sikkimensis but mainly Curculio robustus (Roelofs) (92.7% in the 2002 cohort, n = 141; 87.5% in the 2004 cohort, n = 80). Because of the difficulty of species identification at the larval stage, Q. acutissima is excluded from the results shown below.
The fresh seed weight (mean ± SE [g], range, sample size) decreased in the order Ca. crenata (6.06 ± 0.06, 0.84–23.36, n = 2953) > Q. serrata (1.73 ± 0.01, 0.55–3.52, n = 1566) > Q. myrsinifolia (1.16 ± 0.01, 0.56–1.93, n = 693) (Scheffe’s test: all p < 0.001). Numbers of larval exit holes per seed (mean ± SE, range, sample size) decreased in the order Ca. crenata (1.53 ± 0.03, 1–5, n = 750) > Q. serrata (1.26 ± 0.01, 1–4, n = 2190) > Q. myrsinifolia (1.08 ± 0.01, 1–3, n = 846) (Steel–Dwass test: all p < 0.001).
Seasonal timing of seed fall and emergence of weevil larvae from seeds
In 2002, seed fall started first in Ca. crenata (late August to mid October), then in Q. serrata (early October to early November), and finally in Q. myrsinifolia (mid October to mid January) (Fig. 1). Larval emergence from seeds followed the same order, although larval emergence from Q. myrsinifolia spanned a very long period, from November to April. In the comparison of larval emergence from seeds that fell at different times within each host species, larvae exited earlier from seeds that fell earlier (Fig. 2).
Patterns of seed fall in 2004 were similar to those in 2002 (Fig. 3). Seeds of Ca. crenata fell from late August to mid October, and the most larvae exited from seeds that fell after the peak of seed fall. Seeds of Q. serrata fell from late September to early November, and larvae exited from seeds that fell throughout the period of seed fall. The number of Q. serrata seeds that fell during each 5- or 6-day period changed synchronously with the number of larvae that emerged from them. Seeds of Q. myrsinifolia fell from mid October to early December, and most larvae emerged from seeds that fell early.
Synchronization of weevil oviposition with the seed maturation of hosts
Figure 4a illustrates the observed phenology of the numbers of emerged larvae per fallen seed of Ca. crenata, Q. serrata, and Q. myrsinifolia (i.e., \({y}_{\mathrm{c}t}/{x}_{\mathrm{c}t}\), \({y}_{\mathrm{s}t}/{x}_{\mathrm{s}t}\), and \({y}_{\mathrm{m}t}/{x}_{\mathrm{m}t}\), respectively), which was calculated for each 5- or 6-day period. Figure 4b compares PDFs between the estimated phenology of the total amount of oviposition activity, \({\widehat{\mu }}_{t}\), and the seed phenology of the three species: \({x}_{\mathrm{c}t}/\left(\sum {x}_{\mathrm{c}t}\right)\), \({x}_{\mathrm{s}t}/\left(\sum {x}_{\mathrm{s}t}\right)\), and \({x}_{\mathrm{m}t}/\left(\sum {x}_{\mathrm{m}t}\right)\). The mean period of the oviposition PDF is 10.6, whereas the mean periods of the seed PDFs are 6.5 for Ca. crenata, 11.6 for Q. serrata, and 16.8 for Q. myrsinifolia. Thus, the total amount of oviposition activity seems to be most closely synchronized with the seed phenology of Q. serrata. Figure 4b also supports this conclusion visually. When the preference for Ca. crenata was defined as 1.00, however, the degrees of preference were estimated to be \({w}_{\mathrm{s}}=0.48\) for Q. serrata and \({w}_{\mathrm{m}}=1.75\) for Q. myrsinifolia. That is, the weevil’s preference for Q. myrsinifolia was estimated to be larger than that for Ca. crenata and Q. serrata.
(A) Seasonal change in the number of Curculio sikkimensis larvae per seed of three host species (Castanea crenata, Quercus serrata, and Quercus myrsinifolia) in 2004, which is shown for each 5- or 6-day period within which the sample was collected. (B) Comparison between the probability density functions (PDFs) of the estimated total amount of oviposition in 2004 (\({\widehat{\mu }}_{t}\)) and the PDF of the amount of available seeds of the three host species. The quantity is shown as the probability density for each 5- or 6-day period within which the sample was collected
Body size of adults derived from each host
The mean body weight of adults was affected by host plant, sex, and their interaction (two-way ANOVA: all p < 0.001). Adult body weight (mean ± SE [mg], sample size) decreased in the order of Ca. crenata (male, 31.6 ± 0.6, n = 52; female, 41.9 ± 0.7, n = 85) > Q. serrata (male, 24.3 ± 0.8, n = 35; female, 28.7 ± 1.1, n = 40) > Q. myrsinifolia (male, 20.6 ± 0.6, n = 56; female, 23.4 ± 0.8, n = 79), and was larger in those that developed in larger host seeds (Scheffe’s test: male, Ca. crenata vs. Q. serrate, p < 0.001; Ca. crenata vs. Q. myrsinifolia, p < 0.001; Q. serrata vs. Q. myrsinifolia, p < 0.01; female, all p < 0.001). Females were significantly larger than males within each host species (t test: Ca. crenata, p < 0.001; Q. serrata, p < 0.01; Q. myrsinifolia, p < 0.01), and the difference tended to be greater in those that developed in larger host seeds.
Seasonal timing of development and prolonged diapause of Curculio sikkimensis in a coppice stand
Larvae buried in tissue-culture plates in pots in a coppice stand in 2001 pupated from early June to early August in the next year, most from late June to mid July (Fig. 5). Adult eclosion occurred from early July to late August and peaked from mid July to early August. About 27% of larvae did not resume development in 2002 and seemed to enter prolonged diapause. Larvae released directly into pots in 2001 emerged as adults on the soil surface a month after adult eclosion of larvae buried in the tissue-culture plates (Fig. 5), beginning in early August but mainly from mid August to early September. The rate of adult emergence for larvae that were released directly into pots was 71.7% after the first winter, 28.0% after the second, and 0.3% after the third (n = 293). No adult emerged from soil after the fourth or later winter.
Weevil adult longevity after emergence from soil
Mean longevities (mean ± SE [days], range, sample size) were much longer in fed adults (male, 56.3 ± 6.1, 21–95, n = 15; female, 57.8 ± 5.5, 20–108, n = 24) than in unfed adults (male, 8.6 ± 0.8, 6–19, n = 17; female, 14.0 ± 1.2, 4–25, n = 22) (two-way ANOVA: food condition, p < 0.001; sex, p = 0.42; interaction, p = 0.64). Three of the fed adults survived for more than 100 days.
Discussion
Understanding the use of host plants and life-cycle traits is clearly important for analysis of Cu. sikkimensis damage to chestnut seeds. The present study revealed details about the weevil’s use of several host plants with different seed maturation times and its seasonal development in mixed oak and chestnut coppice stands. Curculio sikkimensis oviposition phenology most closely tracked the phenology of Q. serrata seed maturation; however, in terms of food quality, Ca. crenata is likely to be the best host for the weevil.
Curculio sikkimensis used three Quercus species in addition to Ca. crenata as hosts. The pattern of larval emergence from seeds followed the timing of seed fall, being earliest in Ca. crenata and latest in Q. myrsinifolia (Fig. 1). Within each host species, larvae emerged later from seeds that fell later (Fig. 2). These facts suggest that Cu. sikkimensis females select seeds that have reached a developmental stage that is suitable for oviposition and use several host plants with different seed maturation times sequentially over a long period. Such specific timing of oviposition during seed growth has also been reported in Cu. sikkimensis in chestnut cultivars (Okabe and Takaeda 1993), Curculio caryae in pecan (Harris 1976), and Curculio elephas, Curculio glandium, and Cu. robustus in oak (Espelta et al. 2009; Higaki 2016).
Most larvae that developed in seeds of Ca. crenata and Q. serrata emerged by December (Fig. 1). In contrast, fewer than half of the larvae that developed in seeds of Q. myrsinifolia emerged by then, and emergence from this host continued until the next April. It seems likely that most individuals did not receive sufficient temperature to complete larval development within the year because of the late timing of oviposition in Q. myrsinifolia seeds. Alternatively, it is possible that seed quality affected larval development. Seeds of Q. myrsinifolia remain dormant until the following spring, whereas those of Q. serrata germinate soon after falling (Higaki, unpublished data). Therefore, Cu. sikkimensis larvae can use reserves stored by Q. myrsinifolia seeds over a longer time. Adult emergence of larvae developed in Q. myrsinifolia seeds occurred in the next summer, irrespective of the time of larval emergence from seeds (Higaki, unpublished data). Therefore, Cu. sikkimensis larvae that emerged from seeds late in the season would still have a good chance of reproduction.
Few larvae exited from Ca. crenata seeds that fell early, with most exiting from seeds that fell in the middle to late period of seed fall (Fig. 3). This pattern is similar to previous reports that Cu. sikkimensis rarely attacks seeds of early-maturing cultivars and frequently attacks those of late-maturing cultivars in chestnut orchards (Nakagaki and Yanagibashi 1985; Takamura 1971). In Q. serrata, infested seeds were present throughout the seed fall period, and the number of seeds fallen and number of larvae emerged from them changed synchronously (Fig. 3). In Q. myrsinifolia, most larvae emerged from seeds that fell early, whereas few emerged from seeds that fell later. In the analysis of how well weevil oviposition synchronizes with the phenology of host species, the mean period of the oviposition PDF is nearest to the mean period of the seed PDF for Q. serrata (Fig. 4b). Thus, despite the weevil being known as a serious chestnut pest, Cu. sikkimensis oviposition phenology most closely tracks the phenology of Q. serrata seed maturation.
However, more larvae developed per seed and adult body size was larger in groups that developed in larger host seeds, in the order of Ca. crenata > Q. serrata > Q. myrsinifolia. The increase of adult body size when larvae developed in larger host seeds was more evident in females than in males. In the European chestnut weevil Cu. elephas, larger larvae have a higher likelihood of survival and larger adults have a higher potential fecundity (Desouhant et al. 2000). Likewise, large adults of Cu. sikkimensis may have an advantage in reproductive performance such as oviposition. Thus, in terms of food quality, Ca. crenata is likely to be the best host for Cu. sikkimensis among the three species.
On the other hand, the degree of host preference showed a different trend from oviposition phenology and food quality and was estimated to be larger for Q. myrsinifolia than for Ca. crenata and Q. serrata. Further studies of host plant suitability for Cu. sikkimensis are needed to explain why this weevil synchronizes its oviposition with Q. serrata seed maturation more closely than with that of Ca. crenata and Q. myrsinifolia. Such studies should evaluate quality of seeds as hosts for larval development and quantify acorn traits such as seed mass, shell thickness, and tannin, lipid, and protein contents (Bogdziewicz et al. 2018).
As reported in many Quercus species (Crawley and Long 1995; Maeto and Ozaki 2003; Sork et al. 1993), seed production by Q. serrata, Q. acutissima, and Q. myrsinifolia varies considerably from year to year in coppice stands (Higaki 2016; Higaki unpublished data). If Cu. sikkimensis is dependent on Quercus seeds, then the weevil’s population size may be considerably affected by masting in Quercus species. This and previous studies (Higaki 2005, 2006; Higaki and Toyama 2012; Maeto and Ozaki 2003) show that Cu. sikkimensis enters prolonged diapause in the larval stage and that adult emergence is spread over several years. Thus, prolonged diapause in Cu. sikkimensis may serve as a bet-hedging tactic that protects against irregular and unpredictable seed production in its host plants, as reported in other Curculio species (Higaki 2016; Maeto and Ozaki 2003; Menu and Debouzie 1993). To understand the relationship between seed production by host trees and predation by Cu. sikkimensis, it will be necessary to examine interannual variations in seed production, rates of infestation by weevils, and the life-history traits of the weevil.
Larvae reared individually in tissue-culture plates buried in pots in the coppice stand eclosed as adults mainly from early July to mid August (Fig. 5). Those released directly into pots emerged on the soil surface a month later. These results show that Cu. sikkimensis adults remained in the soil for a month before emergence, as also reported in Cu. robustus (Higaki 2016). Adult emergence dates of Cu. sikkimensis were spread over a month in the coppice stand (Fig. 5), as reported in Cu. elephas by Menu (1993), who suggested that this extended period allows the weevil to respond to the annual variability of chestnut phenology. The extension of adult emergence dates also seems to be beneficial for Cu. sikkimensis using several host plants with different seed maturation times.
Curculio sikkimensis larvae emerged later from seeds that fell later, and the beginning of larval emergence varied from early October to early December (Fig. 2), suggesting that the oviposition period continues for about 2 months. Rearing experiments on a chestnut tree indicated that adult longevity of Cu. elephas was about 26–30 days (Menu and Debouzie 1993). In this study, adult lifespan was about 2 months, and several individuals survived for more than 100 days when adults were reared on jelly diet. Thus, Cu. sikkimensis adults have high potential longevity. In addition to the extension of adult emergence dates, a long adult lifespan seems to enable this weevil to use seeds of several hosts over a long period. In woodlands, Cu. sikkimensis adults are known to feed on small immature acorns and willow twigs (Maeto 1993). Further experiments should be conducted by feeding other natural foods such as immature acorns.
My findings indicate that Cu. sikkimensis uses seeds of several host plants with different seed maturation times in coppice stands, and the phenology of its oviposition most closely tracks the phenology of Q. serrata seed maturation. This weevil is more dependent on Q. serrata than on Ca. crenata, and its population size seems to be considerably affected by seed production of Quercus species. Because chestnut orchards in Japan are often surrounded by woodlands, the forest composition should be taken into account in any analysis of damage to chestnut caused by Cu. sikkimensis. Factors influencing population dynamics of this weevil such as masting of host plants, characteristics of the prolonged larval diapause, and natural enemies should be examined. To reveal how this weevil moves from woodland to orchard and the process of chestnut infestation, it is important to examine its mobility, host location, and reproductive traits.
Change history
15 May 2021
A Correction to this paper has been published: https://doi.org/10.1007/s13355-021-00745-7
References
Bogdziewicz M, Bonal R, Espelta JM, Kalemba EM, Steele MA, Zwolak R (2018) Invasive oaks escape pre-dispersal insect seed predation and trap enemies in their seeds. Integr Zool 13:228–237. https://doi.org/10.1111/1749-4877.12285
Crawley MJ, Long CR (1995) Alternate bearing, predator satiation and seedling recruitment in Quercus robur L. J Ecol 83:683–696. https://doi.org/10.2307/2261636
Desouhant E, Debouzie D, Ploye H, Menu F (2000) Clutch size manipulations in the chestnut weevil, Curculio elephas: fitness of oviposition strategies. Oecologia 122:493–499. https://doi.org/10.1007/s004420050971
Espelta JM, Bonal R, Sánchez-Humanes B (2009) Pre-dispersal acorn predation in mixed oak forests: interspecific differences are driven by the interplay among seed phenology, seed size and predator size. J Ecol 97:1416–1423. https://doi.org/10.1111/j.1365-2745.2009.01564.x
Fukumoto H, Kajimura H (1999) Seed-insect fauna of pre-dispersal acorns and acorn seasonal fall patterns of Quercus variabilis and Q. serrata in central Japan. Entomol Sci 2:197–203
Harris MK (1976) Pecan weevil adult emergence, onset of oviposition and larval emergence from the nut as affected by the phenology of the pecan. J Econ Entomol 69:167–170. https://doi.org/10.1093/jee/69.2.167
Hayashi M, Morimoto K, Kimoto S (1984) The Coleoptera of Japan in Color, vol 4. Hoikusha, Osaka (in Japanese)
Higaki M (2005) Effect of temperature on the termination of prolonged larval diapause in the chestnut weevil Curculio sikkimensis (Coleoptera: Curculionidae). J Insect Physiol 51:1352–1358. https://doi.org/10.1016/j.jinsphys.2005.08.006
Higaki M (2006) Repeated cycles of chilling and warming effectively terminate prolonged larval diapause in the chestnut weevil, Curculio sikkimensis. J Insect Physiol 52:514–519. https://doi.org/10.1016/j.jinsphys.2006.01.011
Higaki M (2016) Prolonged diapause and seed predation by the acorn weevil, Curculio robustus, in relation to masting of the deciduous oak Quercus acutissima. Entomol Exp Appl 159:338–346. https://doi.org/10.1111/eea.12444
Higaki M, Toyama M (2012) Evidence for reversible change in intensity of prolonged diapause in the chestnut weevil Curculio sikkimensis. J Insect Physiol 58:56–60. https://doi.org/10.1016/j.jinsphys.2011.09.014
Ihara F, Toyama M, Sato T (2003) Pathogenicity of Metarhizium anisopliae to the chestnut weevil larvae under laboratory and field conditions. Appl Entomol Zool 38:461–465. https://doi.org/10.1303/aez.2003.461
Kanazaki S, Ii Y (2008) Oviposition season of chestnut weevil (Curculio sikkimensis) and its control with peach moth (Conogethes punctiferalis) on the chestnut (Castanea crenata) cultivation. Bull Ehime Fruit Tree Exp Sta 22:17–24 (in Japanese)
Kimura Y (1971) Notes on the ecology of the chestnut curculio, Curculio dentipes Roelofs (Coleoptera: Curculionidae) and the European codling moth, Laspeyresia kurokoi Amsel (Lepidoptera: Eucosmidae) and their control. Bull Osaka Pref Agric For Res Cent 8:107–112 (in Japanese)
Maeto K (1993) Acorn insects of Quercus mongolica var. grosseserrata in the Hitsujigaoka natural forest, Hokkaido— life-history of the principal species and their impacts on seed viability. Trans Mtg Hokkaido Br Jpn For Soc 41:88–90 (in Japanese)
Maeto K, Ozaki K (2003) Prolonged diapause of specialist seed-feeders makes predator satiation unstable in masting of Quercus crispula. Oecologia 137:392–398. https://doi.org/10.1007/s00442-003-1381-6
Menu F (1993) Strategies of emergence in the chestnut weevil Curculio elephas (Coleoptera: Curculionidae). Oecologia 96:383–390. https://doi.org/10.1007/BF00317509
Menu F, Debouzie D (1993) Coin-flipping plasticity and prolonged diapause in insects: example of the chestnut weevil Curculio elephas (Coleoptera: Curculionidae). Oecologia 93:367–373. https://doi.org/10.1007/BF00317880
Motoki Y (2008) Trends and new cultivation techniques of Japanese chestnut production in the global economy. Rissho University, The Quarterly Report of Economics 58:99–130 (in Japanese)
Nakagaki S, Yanagibashi Y (1985) Development of the chestnut curculio, Curculio dentipes Roelofs, and nut fruit tortrix, Cydia kurokoi Amsel in the harvesting time. Proc Kanto-Tosan Plant Protect Soc 32:203–204. https://doi.org/10.11337/ktpps1954.1985.203 (in Japanese)
Okabe N, Takaeda M (1993) Ecology and control of Curculio dentipes Roelofs in Ishikawa prefecture. Plant Prot 47:301–304 (in Japanese)
Simonoff JS (1996) Smoothing methods in statistics. Springer Verlag, New York
Sork VL, Bramble J, Sexton O (1993) Ecology of mast–fruiting in three species of North American deciduous oaks. Ecology 74:528–541. https://doi.org/10.2307/1939313
Takamura N (1971) Ecology of the chestnut weevil, Curculio sikkimensis. Bull Iwate Pref Forest Tech Center 3:21–28 (in Japanese)
Ueda A (2000) Pre- and Post-dispersal damage to the acorns of two oak species (Quercus serrata Thunb. and Q. mongolica Fischer) in a species-rich deciduous forest. J For Res 5:169–174. https://doi.org/10.1007/BF02762397
Acknowledgements
I thank Dr. Kohji Yamamura (Institute for Agro-Environmental Sciences, National Agriculture and Food Research Organization [NARO]) for his advice on statistical analysis. I also thank Ms. Mitsue Fukuda (Institute of Fruit Tree and Tea Science, NARO) for collecting chestnut seeds in 2015.
Author information
Authors and Affiliations
Corresponding author
Additional information
Publisher's Note
Springer Nature remains neutral with regard to jurisdictional claims in published maps and institutional affiliations.
The original online version of this article was revised due to the figure 4 was published incorrectly and corrected in this version.
Rights and permissions
About this article
Cite this article
Higaki, M. Use of several host plants with different seed maturation times by the chestnut weevil, Curculio sikkimensis (Coleoptera: Curculionidae), in mixed oak and chestnut coppice stands. Appl Entomol Zool 56, 345–353 (2021). https://doi.org/10.1007/s13355-021-00740-y
Received:
Accepted:
Published:
Issue Date:
DOI: https://doi.org/10.1007/s13355-021-00740-y