Abstract
Osteocalcin is an abundant, highly conserved bone specific protein and the serum levels of OC have been used as a biochemical marker of bone turnover. The genetic variation of certain candidate genes impacts osteocalcin levels in the postmenopausal period and may predispose some women to high bone turnover. To identify the genes influencing variation in serum OC levels, we investigated the polymorphisms of Vitamin D, Estrogen α, Parathyroid and Collagen Type I alpha Receptor genes and its association with bone turnover evaluated by serum osteocalcin in postmenopausal women from south India. The polymerase chain reaction-restriction fragment length polymorphism strategy was used to detect the polymorphisms at all the four gene receptors (i.e., for VDR, ERα, PTH and COLIA1) in 300 postmenopausal women from South India. Serum osteocalcin levels were measured by immunoassay (ELISA).The serum osteocalcin levels for the Apa I polymorphisms showed varied results, in which, subjects in the control group with “GG” genotype and the osteopenic group with “TT” genotype of the ApaI polymorphism had a significantly higher serum osteocalcin concentration (p < 0.05). The BstBI-AA group in controls had a significantly higher level of serum osteocalcin, this suggests a higher state of bone turnover in the AA genotype. The outcome of this study proposes the probability of a small impact of the VDR- ApaI (GG) genotype, the VDR-TaqI (TT) genotype and the (AA) genotype of the PTH-BstBI polymorphism indicating a higher rate of bone turnover in the healthy postmenopausal women.
Graphical Abstract

Similar content being viewed by others
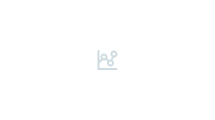
Avoid common mistakes on your manuscript.
Introduction
Osteoporosis is a typical metabolic bone disorder with a strong hereditary impact. It is portrayed by decline in bone mass and defects in bone tissue which weaken bone strength and lead to increased risk of bone fragility [14, 15]. Osteoporosis inflicts 33% of women and one out of eight men beyond the age of 50 [17]. In adolescence there is a high pace of bone turnover in which formation surpasses resorption. In young adulthood, formation and resorption are in exact equilibrium, but with aging there is an overall deficit of bone. Susceptibility to osteoporosis results from a wide range of genetic variations and their interactions with environmental factors [23]. Bone biomarkers produced from the bone remodeling measure includes bone development biomarkers, bone resorption biomarkers and regulators of bone turnover. Distinguishing of bone metabolism has been explored with the biomarkers of catalysts, proteins and by-products during the bone remodeling process [5]. Diverse biomarkers are as of now available for explicit and sensitive evaluation of the pace of bone development and bone resorption. These biomarkers are important to give the early assessment of osteoporosis when the BMD assessment does not offer adequate information to make the conclusion [20]. Osteocalcin has regularly been utilized as a serum marker for bone formation and act in the bone matrix to control mineralization. Osteocalcin may likewise be engaged with the enrollment and differentiation of osteoclasts. Morrison and collaborators observed that a typical allelic variation in a gene encoding the vitamin D receptor had a strong affiliation to bone mineral density and bone turnover estimated as serum osteocalcin [18]. Recognizing genetic risk factors for osteoporosis depends upon previous information, for example, the known association of a specific gene in aspects of osteoporosis, e.g., bone metabolism. This gene is then referred to as a "candidate gene". In this way, polymorphisms must be identified in the candidate gene that leads to contrasts in the functional levels of the encoded protein. Sequence analysis of a "candidate" osteoporosis gene in various individuals will recognize sequence variants, yet additionally a few databases are currently accessible that contain this data (e.g., NCBI dbSNP, HapMap, 1000Genomes, and a few more specialized databases). Some DNA sequence variations will be simply polymorphic (unknown polymorphisms), while others will have functional consequences for the activity of the protein encoded (functional polymorphisms). Clearly, it relies upon the gene and the kind of polymorphisms in the population. Polymorphisms of interest are normally first tested in population- based or case- control "association studies", to assess the phenotype of interest at the population level. Testing of individual candidate genes to what their contribution is to osteoporosis risk is a valid analysis. Once that has been established, the interaction or multiplicative impacts of a few genes will be examined and finally, gene-environment interactions can be contemplated. Linkage and genetic mapping studies are generally performed for analyzing complex traits and disease. There are two principle subtypes of linkage analysis: parametric (determining a model of inheritance in a family) and nonparametric (no inheritance model) [21]. The most recent strategy has been more generally utilized for examination of complex traits. Numerous genome-wide linkage studies have been performed on Bone Mineral Density (BMD) and other related phenotypes of osteoporosis [24], however even in huge meta-analysis, linkage studies didn't yield any genome-wide significant loci for BMD [12], perhaps in light of the fact that basic variations controlling BMD have modest impacts which are hard to be distinguished by traditional linkage investigations [31]. Given the failure of linkage studies, researchers turned their focus to candidate gene investigations. However, results frequently gave off an impression of being non-replicative, most likely because of the statistical power, sample size, lack of standardized phenotype and genotype, limited number of gene variants evaluated and challenges in matching with cases and controls. Essentially, GWASs have distinguished numerous genome-wide significant loci. The significant advantage of GWASs over candidate gene investigations for common diseases is that they may lead to the identification of new susceptible genes and pathways. The VDR gene encodes the vitamin D receptor (Fig. 1) which plays a crucial role in calcium homeostasis and bone metabolism. Vitamin D is essential for calcium absorption, and its receptor, VDR, mediates its effects. Numerous studies have investigated the association between VDR polymorphisms (ApaI, TaqI, BsmI) and bone mineral density (BMD) or osteoporosis risk. These polymorphisms have been linked to variations in VDR activity and its impact on calcium absorption and bone turnover. For example, the "BsmI B allele" has been associated with lower BMD and increased osteoporosis risk in Caucasian populations [17]. Estrogen plays a crucial role in bone health by inhibiting bone resorption. Polymorphisms in the estrogen type I alpha gene, particularly PvuII and XbaI, may affect estrogen receptor function. Research has examined the association between estrogen receptor gene polymorphisms and osteoporosis risk. Variants like PvuII and XbaI have been linked to differences in estrogen receptor expression and function, potentially impacting bone density and fracture risk [27]. The parathyroid hormone (PTH) is crucial for maintaining calcium levels in the blood and bone remodeling. Polymorphisms in genes related to PTH regulation may influence bone metabolism. Some studies have explored the association between parathyroid gene polymorphisms and bone health. The BstBI polymorphism in the parathyroid gene has been studied for its potential influence on PTH levels and, consequently, bone density [2]. Collagen type I is a major component of bone matrix, and mutations or polymorphisms in the COL1A1 gene can affect collagen production, leading to bone disorders. The COL1A1 gene has been extensively studied in the context of osteoporosis and bone diseases. Polymorphisms like the SpI polymorphism have been associated with variations in bone density and fracture risk in some populations [30]. In summary, these five genes were chosen for testing in osteoporosis and bone metabolism studies because of their biological relevance to bone health and the existing body of research indicating potential associations between specific gene variants (polymorphisms) and bone-related phenotypes, such as bone mineral density, fracture risk, and calcium homeostasis. Prior studies have provided valuable insights into how these genetic variations may contribute to the development and progression of osteoporosis, making them important candidates for further investigation in clinical and genetic studies [19]. The aim of the current study was to examine the polymorphisms of the vitamin D receptor (VDR), estrogen receptor (ER) and parathyroid and collagen type I receptor gene polymorphisms in association with biochemical markers of bone turnover evaluated by serum osteocalcin in postmenopausal women from south India.
Methods
Study subjects
The present case–control study consisted of randomly selected 300 South Indian women in the post menopausal age group who belong to the south Indian population. A standard questionnaire was used to collect information regarding age, medical conditions; medications used risk factors, family history of osteoporosis together with dietary and lifestyle habit. The subjects who had various endocrinological disorders such as hyperthyroidism and hypo- and hyperparathyroidism, chronic disorders of the liver and kidney and other skeletal diseases (Paget’s disease, osteogenesis imperfecta and rheumatoid arthritis) along with few other subjects using medications that were known to affect bone density and metabolism (such as calcium supplements, corticosteroids, anticonvulsants, hormone replacement therapy and heparin) or those with an unusual gynaecological history such as bilateral oophorectomy, irregular cycles or premature menopause before the age of 40 were excluded from this study. The stratified random sampling method was used in this study. The selection of the study group being restricted only to postmenopausal women, all of whom were to be without any of the above mentioned major risk factors posed a limiting factor. Thus, reducing the sample size to achieve 80% statistical power. Hong et al. [11], suggested that the dominant model required the smallest sample size (90 cases and 90 controls) to achieve 80% power when compared to other genetic models, under the following assumptions of 5% disease prevalence, 5% MAF, complete LD (D' = 1), 1:1 case-to-control ratio, and 5% type I error rate (α) and odds ratios of heterozygotes at 2 and odds ratios of rare homozygotes at 3.
Estimation of serum osteocalcin
Blood samples were obtained using standard venipuncture technique for DNA processing and measurement of blood chemistries. Serum was separated from clotted blood by centrifugation and then stored at − 80 °C until assayed. Serum concentrations of intact OC were measured in duplicate with enzyme-linked immunosorbent assay (ELISA) using the Human osteocalcin/Bone gla protein (OT/BGP) ELISA kit (Bioassay Technology Laboratory, China), in a micro titer strip well format using monoclonal antibody directed against distinct epitopes of human osteocalcin.
SNP genotyping
DNA was isolated for PCR amplification from the whole blood samples by non-enzymatic salting-out method [24]. The following polymorphisms of rs7975232, rs731236, rs1544410, rs2234693, rs9340799, rs6254 and rs1800012 was genotyped for all the subjects using PCR- restriction fragment length polymorphism (RFLP), as previously described by us in our earlier work [6].
Statistical analysis
All the analysis were carried out using the statistical package of Graph Pad Prism version 8. The association of genotype with serum osteocalcin was evaluated by analysis of variance (one-way ANOVA). Multiple linear regression analyses were performed to examine the interaction effects on the levels of serum osteocalcin variations among the SNPs. The subjects were divided into three groups based on their genotypes and each group was coded as “0”, “1” and “2” respectively. The code “0” represents the homozygous genotype for the reference genotype, the code “1” represents the heterozygous genotype and code “2” represents the homozygous genotype for the variant allele. The p value < 0.05 was selected to define statistical significance.
Results
Baseline characteristics of the study subjects
The distribution of serum osteocalcin levels in the postmenopausal women is given in Fig. 2. The serum OC concentrations were measured in 300 subjects, ranging in age from 45 to 88 years. Values ranged from 2.20 ng/mL, the lower limit of detection of this assay, to 44.50 ng/mL. Mean values were 15.1 ± 7.8 ng/mL and 31.6 ± 9.8 ng/mL in healthy and osteoporotic postmenopausal women, respectively. The distribution of serum OC according to age is shown in Table 1. The highest mean of serum osteocalcin level was found among the age group 65–70 years (27.70 ± 13.3). The Anthropometric Characteristics of the Study Subjects are given in Table 2.
Association of VDR genotypes with serum osteocalcin levels
Analysis of the relationship between RFLPs and serum osteocalcin is shown in Table 3. The serum osteocalcin levels did not show any significant differences in their concentrations in subjects with different genotypes for Bsm I polymorphisms. However, osteocalcin levels for the Apa I polymorphisms showed varied results, in which, subjects in the control group with “GG” genotype and the osteopenic group with “TT” genotype of the ApaI polymorphism had a significantly higher serum osteocalcin concentration (p < 0.05). Similarly, for TaqI polymorphism, controls with wild type genotype “TT” shows significantly elevated levels (p < 0.05) than the osteopenic and the osteoporotic groups with no significant variations. It was observed that, among all three groups the subjects who were osteoporotic exhibited elevated concentrations of serum osteocalcin. There was no significant difference in the serum OC levels among the different genotypes. The combined genotypes for VDR- ApaI-Taq-BsmI were analyzed for the association with serum osteocalcin levels. Among the combined genotypes only 26 groups were used for the analysis and the following six combined genotype groups GGCCAA, GGTCAA, GGTCGG, GGTTAG, GGTTGG, TGTTGG was not included in the calculations since the number was less than 5 in each group. The genotype “GGTTAA” was not observed in this study population. There were no significant association found between bone mineral density and serum osteocalcin with the combined genotypes. The combination genotype TTTCAA had the highest mean osteocalcin levels (31.0 ± 13.7) as seen in Table 4. In combination these genotypic differences are likely to affect VDR protein levels of function depending on the cell type, developmental stage and activation status.
Association of ERα 1 genotypes with serum osteocalcin levels
The mean levels of osteocalcin in control, osteopenic and osteoporotic postmenopausal women are classified according to the genotypes in Table 3. There was no significant difference in the osteocalcin levels across the PvuII (controls TT = 13.5 ± 7.1, TC = 16.5 ± 8.7, CC = 26.5 ± 6.2; p = 0.3, osteopenic TT = 26.5 ± 6.2, TC = 26.3 ± 8.0, CC = 24.2 ± 8.3; p = 0.5, osteoporotic TT = 30.5 ± 7.6, TC = 30.8 ± 10.6, CC = 34.1 ± 10.4; p = 0.3) and XbaI polymorphisms (controls AA = 15.5 ± 8.1, AG = 14.4 ± 7.0, GG = 15.8 ± 8.7; p = 0.7, osteopenic AA = 24.8 ± 5.6, AG = 26.3 ± 9.0, GG = 26.7 ± 6.9; p = 0.5, osteoporotic AA = 32.6 ± 8.7, AG = 30.1 ± 11.8, GG = 25.7 ± 6.4; p = 0.07). The genotype combination did not have any significant association with serum osetocalin levels. The combined genotype TTAG showed (Table 5) the lowest level of OC (20.6 ± 8.7 ng/mL) whereas genotype CCAA revealed women with a higher serum osteocalcin level (28 ± 12.1 ng/mL).
Association between PTH- BstBI genotypes with serum osteocalcin levels
The levels of serum osteocalcin a turnover marker examined, the levels of serum osteocalcin in controls were statistically higher in the PTH–GG group than in the PTH–GA and AA PTH; (GG = 17.9 ± 9.4, GA = 12.3 ± 5.6, AA = 16.1 ± 7.5; p = 0.007*). The mean levels of osteocalcin among the genotype groups in osteopenic and osteoporotic postmenopausal women did not have significant difference: (osteopenic GG = 25 ± 5.4, GA = 26.1 ± 8.4, AA = 26.8 ± 8.5; p = 0.6, osteoporotic GG = 31.6 ± 11.5, GA = 30 ± 8.7, AA = 33.2 ± 9.2; p = 0.4) as given in Table 3. Although there was no significant difference in the serum levels of osteocalcin among the three genotype groups in the osteopenic and osteoporotic women, the PTH-AA group in controls had a significantly higher level of serum osteocalcin, this suggests a higher state of bone turnover in the AA genotype [16].
Association between collagen type I alpha 1-Sp1 polymorphism genotypes with serum osteocalcin levels
The mean levels of serum osteocalcin did not have significant differences among the genotype for the Collagen Type I gene receptor as given in Table 3 for the study groups; (controls GG = 15 ± 8, GT = 14 ± 7; p = 0.3, osteopenic GG = 26 ± 7, GT = 25 ± 7; p = 0.6, osteoporotic GG = 31 ± 9, GT = 32 ± 10; p = 0.5).
Gene-to-gene interactions of genotypes with respect to osteocalcin by multiple linear regression
The results of multiple linear regression analysis in postmenopausal women with respect to the gene interaction model among the VDR, ER α1, PTH and COLIA1 genotypes for serum osteocalcin observed a significant interaction between TaqI × BsmI (Adjusted R2 = 0.831, p = 0.04*). No other interaction models were found to be significant as seen in Table 6.
Discussion
The subjects for this study comprised of South Indian postmenopausal women, to evaluate the polymorphisms of the VDR, ER α1, PTH and COLIA1 genes in association with serum osteocalcin levels. Osteocalcin comprises 25% of the non-collagenous matrix proteins and is manufactured by the bone cells [1, 19]. To determine whether allelic variation in the VDR receptor gene is the cause of the variation in serum osteocalcin levels. Three polymorphisms namely ApaI, TaqI and BsmI was investigated among the 300 postmenopausal women and the results revealed an increased level of serum osteocalcin in healthy postmenopausal women for ApaI (GG) and TaqI (TT) polymorphisms. Meanwhile, the ApaI (TT) genotype shows a fundamentally raised degree of serum osteocalcin in osteopenic women. However, the mean serum osteocalcin level was significantly higher in the group of postmenopausal osteoporotic women than in control women. These differences may indicate a higher rate of bone turnover in healthy postmenopausal women [12]. Such differences in serum osteocalcin concentrations in healthy and osteoporotic women were not found to be consistent (Table 3). This may be due to the small sample size or the absence of age matching between the allelic subgroups. In a recent report the TT of TaqI genotype was associated with low levels of OC in overweight and obesity type 2 diabetes mellitus subjects [25]. In contrast to the present study, the findings of the Sheehan et al. [28]suggests that in healthy Irish adults with the tt VDR genotype have higher rates of bone turnover than those with Tt or TT VDR genotypes and, therefore, may have a higher risk of low osteocalcin.
The association of ER α genotypes and serum osteocalcin levels was also assessed in this study. Osteocalcin being a distinct bone turnover marker assuming a significant part in bone redesigning raises the likelihood that the ESR genotypes characterized by XbaI and PvuII might be of importance in deciding the variability of bone rebuilding and the level of reaction to retreatment by estrogen. Our results did not show any significant association between genotypes and serum osteocalcin. However Rapuri et al. [23] reported that the change in bone remodelling markers was significantly higher in women with CC or GG genotypes. Sowers et al. [24] reports that women homozygous for the ER α gene variant without an XbaI restriction site (AA) and PvuII restriction site (TT) had significantly lower osteocalcin levels. Estrogen receptor 1 plays a significant part in the support of the skeletal framework which has been demonstrated in trial mice, from which the gene was erased from the particular bone cells and their precursors. Absence of the estrogen receptor in osteoblast precursor cells affected the periosteum, while their lack in differentiated osteoblasts, osteoclasts, and osteocytes occur in reduced cancellous bone mass [3]. In the assessments on ERα gene XbaI polymorphism and COL1A1gene Sp1 polymorphism, it was accounted for that there was no differentiation as far as normal OC levels, genotype, and allele frequencies among the groups. A review in elderly women by Koren et al. [27] reports that the Px haplotype of the ERα gene receptor and the “s” allele of the COLIA1 sp1 polymorphism might be engaged with causing the phenotypic articulation of higher circulating levels of PTH and higher bone turnover, which, thus, may prompt bone loss.
The degree of osteocalcin as turnover marker was also analyzed in PTH- BstBI polymorphism among the controls, osteopenic and osteoporotic postmenopausal women. It was noticed that the osteocalcin levels within the controls was statistically higher in the PTH–AA group than in the PTH– GA and GG groups (Table 3). However, the degree of osteocalcin was not significantly different between the genotypes for the osteopenic and osteoporotic women. Similar results were observed with no association was observed in Arab postmenopausal women with serum levels of osteocalcin and PTHR1 gene polymorphisms [27]. Although there was no significant difference in the serum levels of osteocalcin among the three genotype groups in the osteopenic and osteoporotic women, the PTH-AA group in controls had a significantly higher level of serum osteocalcin, this suggests a higher state of bone turnover in the AA genotype among the control group.
This study also demonstrated a significant association of SNP combination TaqI (rs731236) T > C and BsmI (rs1544410) A > G in the VDR gene with serum osteocalcin (Table 6). Marcia et al. [36] reported on the polymorphisms in VDR, COLIA1, osteocalcin and osteonectin genes which were related with change in BMD with time and the serum markers of osteocalcin. In any case, they revealed a significant gene- gene collaboration impact with the ER genotypes and the VDR BsmI genotype. The biological and functional proof would be needed to confirm the intriguing impact of polymorphisms on the circulating levels of osteocalcin. Additionally, to the best of our knowledge we were the first to demonstrate the influence of gene–gene interactions in VDR, ER α1, PTH and COLIA1 genes on the circulating levels of serum osteocalcin in postmenopausal women. To further investigate the genetic interactions between these genes, the number of the studied SNPs should be increased and confirmed in the larger cohorts.
Serum OC levels are viewed as heritable in different populaces like the Mexican American populace [22], Australian [16] and United Kingdom [8]. How the hereditary determinants of serum OC may be or in which pathways they could act isn’t clear. For example, at younger ages serum OC could transcendently reflect skeletal improvement and serum OC levels may be emphatically impacted by genes related with bone formation. Nonetheless, as ages increases, serum OC may transcendently reflect bone turnover rates and OC levels might be strongly impacted by genes influencing osteoblast or osteoclast function. There are no evident candidate genes that could impact bone turnover. A few affiliation investigations of serum OC levels and gene variations have been performed to identify candidate genes, including type I collagen alpha 1 [7], the estrogen receptor a gene [10, 16] and the Vitamin D receptor gene and reported to have no association accounted for in this population. This study reports no association among age and BMI to any of these gene variations in our study. It is conceivable that the overall impacts of various genes on phenotypic variation differ among populaces, and results acquired from the south Indian population may not be generalized to other population. Many cross-sectional information in a large group of postmenopausal women showed that serum osteocalcin increases at the menopause and consequently declines with increasing age [4, 35, 37]. The high levels of osteocalcin in the postmenopausal women are credited to the lack of estrogen, an observation which associates to similar reports [10], which demonstrate a strong correlation between the estrogen inadequacy and the raised osteocalcin levels. The low estrogen levels at the menopause leads to a lower absorption of calcium, bringing about low serum calcium concentrations and a higher osteoclastic resorption of the bone, thus increasing bone turnover, which in turn contributes as risk factors of osteoporosis. Serum osteocalcin is a accurate marker of the bone turnover, especially when the resorption and bone formation are coupled. It is also considered as a specific marker of bone development when the formation and resorption are uncoupled [34].
In conclusion it is noticed that genetic factors are most likely not a significant determinant in explaining the variation of the circulating serum osteocalcin levels in postmenopausal women. The outcome of this study proposes the probability of a small impact of the VDR-ApaI (GG) genotype, the VDR-TaqI (TT) genotype and the (AA) genotype of the PTH-BstBI polymorphism indicating a higher rate of bone turnover in the healthy postmenopausal women. This impact, if present, would be an important clinical risk factor for osteoporosis after menopause. In contrast, our previous study in the same population supports the significant role of VDR-BsmI (rs1544410) and PTH-BstBI (rs6254) polymorphisms and its significant allelic association with bone mineral density either individually or in different combinations pertaining to osteoporosis susceptibility among postmenopausal women. The genetic effects on premenopausal bone turnover, peak bone mass and environmental mechanisms will probably play a significant part in mediating postmenopausal bone turnover. The high rate of bone turnover after menopause is probably responsible for the increased rate of bone loss, our results indicate that genetic factors regulate bone development within a group of normal subjects and demonstrate strongly that more focus ought to be coordinated towards the regulation of bone turnover and in understanding the molecular mechanisms underlying the genetic impact on postmenopausal osteoporosis.
Availability of data and materials
The datasets generated during and/or analyzed during the current study are available from the corresponding author on reasonable request.
Code availability
Not applicable.
References
Abdi S, Almiman AA, Ansari MGA, Alnaami AM, Mohammed AK, Aljohani NJ, Alenad A, Alghamdi A, Alokail MS, Al-Daghri NM. PTHR1 genetic polymorphisms are associated with osteoporosis among postmenopausal Arab women. BioMed Res Int. 2021. https://doi.org/10.1155/2021/2993761.
Abdi S, Almiman AA, Ansari MGA, Alnaami AM, Mohammed AK, Aljohani NJ, Alenad A, Alghamdi A, Alokail MS, Al-Daghri NM. PTHR1 genetic polymorphisms are associated with osteoporosis among postmenopausal arab women. Biomed Res Int. 2021;2021:2993761. https://doi.org/10.1155/2021/2993761.
Almeida M, Iyer S, Martin-Millan M, Bartell SM, Han L, Ambrogini E, Onal M, Xiong J, Weinstein RS, Jilka RL, O’Brien CA, Manolagas SC. Estrogen receptor-α signaling in osteoblast progenitors stimulates cortical bone accrual. J Clin Investig. 2013;123(1):394–404. https://doi.org/10.1172/JCI65910.
Aloubaidy R. Osteocalcin level and its association with vitamin D receptor gene polymorphisms (TaqI and ApaI) in Iraqi obese type 2 diabetes mellitus. Int J Sci Res (IJSR). 2017;6:1599–604.
Eastell R, Hannon RA. Biomarkers of bone health and osteoporosis risk. Proc Nutr Soc. 2008;67(2):157–62.
Fernandez C, Tennyson J, Priscilla AS. Osteoporosis and its association with vitamin D receptor, oestrogen α receptor, parathyroid receptor and collagen type I alpha receptor gene polymorphisms with bone mineral density: a pilot study from south Indian postmenopausal women of Tamil Nadu. Biochem Genet. 2022. https://doi.org/10.1007/s10528-022-10197-5.
Garnero P, Borel O, Grant SF, Ralston SH, Delmas PD. Collagen Ia1 Sp1 polymorphism, bone mass, and bone turnover in healthy French premeno pausal women: the OFELY study. J Bone Miner Res. 1998;13(813–817):37.
Garnero P, Borel O, Sornay-Rendu E, Arlot ME, Delmas PD. Vitamin D receptor gene polymorphisms are not related to bone turnover, rate of bone loss, and bone mass in postmenopausal women: the OFELY Study. J Bone Miner Res. 1996;11:827–34.
Griesmacher A, Peichl P, Pointinger P. Biochemical markers in menopausal women. Scand J Clin Lab Investig. 1997;227:64–72.
Han KO, Moon IG, Kang YS, Chung HY, Min HK, Han IK. Nonassociation of estrogen receptor genotypes with bone mineral density and estrogen responsiveness to hormone replacement therapy in Korean postmenopausal women. J Clin Endocrinol Metab. 1997;82:991–5.
Hong EP, Park JW. Sample size and statistical power calculation in genetic association studies. Genom Inform. 2012;10(2):117–22.
Ioannidis JP, Ng MY, Sham PC, Zintzaras E, Lewis CM, Deng HW, Econs MJ, Karasik D, Devoto M, Kammerer CM, Spector T, Andrew T, Cupples LA, Duncan EL, Foroud T, Kiel DP, Koller D, Langdahl B, Mitchell BD, Peacock M, Ralston SH. Meta-analysis of genome-wide scans provides evidence for sex- and site-specific regulation of bone mass. J Bone Miner Res Off J Am Soc Bone Miner Res. 2007;22(2):173–83.
Kalaiselvi VS, Prabhu K, Ramesh M, Venkatesan V. The association of serum osteocalcin with the bone mineral density in postmenopausal women. J Clin Diagn Res JCDR. 2013;7(5):814–6. https://doi.org/10.7860/JCDR/2013/5370.2946.
Kamel HK. Postmenopausal osteoporosis: etiology, current diagnostic strategies, and nonprescription interventions. J Manag Care Pharmacy JMCP. 2006;12(6 Suppl A):S4–28.
Kanis JA, Melton LJ 3rd, Christiansen C, Johnston CC, Khaltaev N. The diagnosis of osteoporosis. J Bone Miner Res Off J Am Soc Bone Miner Res. 1994;9(8):1137–41.
Kelly PJ, Hopper JL, Macaskill GT, Pocock NA, Sambrook PN, Eisman JA. Genetic factors in bone turnover. J Clin Endocrinol Metab. 1991;72:808–13.
Kobayashi S, Inoue S, Hosoi T, Ouchi Y, Shiraki M, Orimo H. Association of bone mineral density with polymorphism of the estrogen receptor gene. J Bone Miner Res. 1996;11(306–311):38.
Li WF, Hou SX, Yu B, Li MM, Férec C, Chen JM. Genetics of osteoporosis: accelerating pace in gene identification and validation. Hum Genet. 2010;127(3):249–85.
Lian JB, Friedman PA. The vitamin K-dependent synthesis of gamma-carboxyglutamic acid by bone microsomes. J Biol Chem. 1978;253(19):6623–6. https://doi.org/10.1016/S0021-9258(17)37956-5.
Liao J, Qin Q, Zhou Y, et al. Vitamin D receptor Bsm I polymorphism and osteoporosis risk in postmenopausal women: a meta-analysis from 42 studies. Genes Nutr. 2020;15:20. https://doi.org/10.1186/s12263-020-00679-9.
Liu L, Webster TJ. In situ sensor advancements for osteoporosis prevention, diagnosis, and treatment. Curr Osteoporos Rep. 2016;14(6):386–95.
Mitchell BD, Cole SA, Bauer RL, Iturria SJ, Rodriguez EA, Blangero J, MacCluer JW, Hixson JE. Genes influencing variation in serum osteocalcin concentrations are linked to markers on chromosomes 16q and 20q. J Clin Endocrinol Metab. 2000;85(4):1362–6. https://doi.org/10.1210/jcem.85.4.6571.
Morrison NA, Yeoman R, Kelly PJ, Eisman JA. Contribution of trans-acting factor alleles to normal physiological variability: vitamin D receptor gene polymorphism and circulating osteocalcin. Proc Natl Acad Sci USA. 1992;89(15):6665–9. https://doi.org/10.1073/pnas.89.15.6665.
Nishimoto SK, Price PA. Secretion of the vitamin K-dependent protein of bone by rat osteosarcoma cells. Evidence for an intracellular precursor. J Biol Chem. 1980;255(14):6579–83. https://doi.org/10.1016/S0021-9258(18)43608-3.
Ralston SH, Uitterlinden AG. Genetics of osteoporosis. Endocr Rev. 2010;31(5):629-662.4.
Rapuri PB, Gallagher JC, Knezetic JA, Haynatzka V. Estrogen receptor alpha gene polymorphisms are associated with changes in bone remodeling markers and treatment response to estrogen. Maturitas. 2006;53(4):371–9. https://doi.org/10.1016/j.maturitas.2005.07.007.
Rivera-Leon EA, Palmeros-Sanchez B, Llamas-Covarrubias IM, Fernandez S, Armendariz-Borunda J, Gonzalez-Hita M, Bastidas-Ramirez BE, Zepeda-Moreno A, Sanchez-Enriquez S. Vitamin-D receptor gene polymorphisms (TaqI and ApaI) and circulating osteocalcin in type 2 diabetic patients and healthy subjects. Endokrynol Pol. 2015;66(4):329–33. https://doi.org/10.5603/EP.2015.0042.
Saoji R, Desai M, Das RS, Das TK, Khatkhatay MI. Estrogen receptor α and β gene polymorphism in relation to bone mineral density and lipid profile in Northeast Indian women. Gene. 2019;710:202–9. https://doi.org/10.1016/j.gene.2019.05.060.
Sapir-Koren R, Livshits G, Kobyliansky E. Genetic effects of estrogen receptor alpha and collagen IA1 genes on the relationships of parathyroid hormone and 25 hydroxyvitamin D with bone mineral density in Caucasian women. Metab Clin Exp. 2003;52(9):1129–35.
Sheehan D, Bennett T, Cashman K. The genetics of osteoporosis: vitamin D receptor gene polymorphisms and circulating osteocalcin in healthy Irish adults. Ir J Med Sci. 2001;170:54–7. https://doi.org/10.1007/BF0316772321.
Sowers M, Jannausch ML, Liang W, Willing M. Estrogen receptorgenotypes and their association with the 10 year changesin bone mineral density and osteocalcin concentrations. J ClinEndocrinolMetab. 2004;89(2):733.
Stewart TL, Ralston SH. Role of genetic factors in the pathogenesis of osteoporosis. J Endocrinol. 2000;166(2):235–45. https://doi.org/10.1677/joe.0.1660235.
Suguna S, Kamble S, Bharatha A. Genomic DNA isolation from human whole blood samples by non-enzymatic salting out method. Int J Pharm Pharm Sci. 2014;6(6):198–9.
Trajkovic K, Perovic M, Tarasjev A, Pilipovic N, Popovic V, Kanazir S. Association of collagen type I alpha1 gene polymorphism with bone mineral density in osteoporotic women in Serbia. J Women’s Health. 2010;19(7):1299–303. https://doi.org/10.1089/jwh.2009.1698.
Willing M, Sowers M, Aron D, Clark MK, Burns T, Bunten C, Crutchfield M, D’Agostino D, Jannausch M. Bone mineral density and its change in white women: estrogen and vitamin D receptor genotypes and their interaction. J Bone Miner Res. 1998;13:695–705. https://doi.org/10.1359/jbmr.1998.13.4.695.
Wilson SG, Reed PW, Andrew T, Barber MJ, Lindersson M, Langdown M, Thompson D, Thompson E, Bailey M, Chiano M, Kleyn PW. A genome-screen of a large twin cohort reveals linkage for quantitative ultrasound of the calcaneus to 2q33–37 and 4q12–21. J Bone Miner Res. 2004;19:270–7.
Zheng HF, Spector TD, Richards JB. Insights into the genetics of osteoporosis from recent genome-wide association studies. Expert Rev Mol Med. 2011;13: e28.
Acknowledgements
The authors express their sincere thanks to Indian Council of Medical Research for funding this work. The authors also sincerely thank the Principal, Lady Doak College and the management for providing the research facilities to carry out this work. The authors would like to place on record their deepest gratitude to Dr. S. Pugalanthi Pandian, Managing Director, Pandian Advanced Medical Centre for being the clinical advisor for this study.
Funding
This work was supported by Indian Council of Medical Research (ICMR) (NO3/1/2/6/SRF/ORTHO/2018-NCD-I).
Author information
Authors and Affiliations
Contributions
CF devised and performed the experiments and data analysis; JT designed and PAS supervised and managed all studies. All the authors contributed towards writing the manuscript.
Corresponding author
Ethics declarations
Ethical approval
All procedures performed in studies involving human participants were in accordance with the ethical standards of the institutional and/or national research committee and with the 1964 Helsinki declaration and its later amendments or comparable ethical standards.
Consent to participate
Informed consent was obtained from all individual participants included in the study.
Conflict of interest
Authors declare that they have no conflict of interest.
Additional information
Corresponding Editor: Somnath Paul; Reviewers: Neerja Katiyar, Ishita Rehman, Neha Kachewar.
Publisher's Note
Springer Nature remains neutral with regard to jurisdictional claims in published maps and institutional affiliations.
Rights and permissions
Springer Nature or its licensor (e.g. a society or other partner) holds exclusive rights to this article under a publishing agreement with the author(s) or other rightsholder(s); author self-archiving of the accepted manuscript version of this article is solely governed by the terms of such publishing agreement and applicable law.
About this article
Cite this article
Freeman, C., Tennyson, J. & Priscilla, A.S. Genetic variants of vitamin D, estrogen α, parathyroid and collagen type I alpha receptor gene and its influence on circulating serum osteocalcin in postmenopausal osteoporosis: A cohort study. Nucleus (2024). https://doi.org/10.1007/s13237-023-00456-0
Received:
Accepted:
Published:
DOI: https://doi.org/10.1007/s13237-023-00456-0