Abstract
To investigate the relationship between the bamboo including its nutrients and microbiome, and gut microbiome of giant panda, different bamboo species were provided for feeding the giant pandas. Nutrients of bamboo were examined, and the bamboo microbiome and gut microbiome of giant panda were investigated using high throughput sequencing. The results revealed that the composition of gut microbiome in giant panda varied among groups with different bamboo diet. Except for fat and hemicellulose, cellulose, lignin, carbohydrate, protein, flavone and tannin were significantly different between different bamboo species (p < 0.05). PCoA showed that the composition of bamboo microbiome varied by different species. Significant differences in richness and diversity of bacteria were observed between different bamboo (p < 0.05), as well as the abundance of the main bacteria and fungi at phylum and genus level (Kruskal-Wallis, LDA > 4). Interestingly, the content of lignin and cellulose in bamboo was positively associated with the relative abundance of Streptococcus and Clostridium in gut of giant panda, respectively (p < 0.05). The diversity of bamboo bacteria was positively correlated with that of gut bacteria in giant panda (p < 0.05). Significant associations of certain bacteria and fungi between bamboo and gut of giant panda (p < 0.05) were found. 36 bacterial and 54 fungal genera were both shared between bamboo and the gut of giant panda in all groups. Our results demonstrated that the gut microbial community varied between giant pandas fed on different bamboo species revealing that bamboo nutrients and microbiome affect gut microbiome of giant panda. Our findings contributed to the deeper understanding of dietary or environmental impact on gut microbiome of giant panda.
Similar content being viewed by others
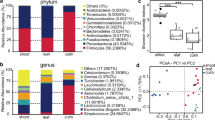
Avoid common mistakes on your manuscript.
1 Introduction
The gastrointestinal tract normally contains an immensely complex ecology of microorganisms, collectively called the gut microbiome (Bäckhed et al. 2004; Zoetendal et al. 2012). Gut microbiome plays a fundamental role on host immune system, metabolic processes and development of diseases (Nicholson et al. 2012; Tremaroli and Backhed 2012). Particularly, the gut microbiome of herbivores play a critical and essential role in facilitating the digestion and fermentation of dietary fibers (Simpson and Campbell 2015; Williams et al. 2013). Changes in gut microbiome composition has been frequently associated with diseases (Claesson et al. 2012). Over the past decades, researchers have established a link between the alteration of gut microbiome and various diseases (Scott et al. 2013; Simpson and Campbell 2015) in human (Jain et al. 2018), brown bear (Persson et al. 2001), monkey (Su et al) and wood mice (Maurice et al. 2015). Early study about human intestinal microbiota from children characterized by a modern western diet and a rural diet indicated that high fiber content in diet lead to the high abundance of gut microbiome for cellulose and xylan hydrolysis (Carlotta et al. 2010). Similar result revealed the components of diet nutrition could influence human gut microbiome remarkably (Zhernakova et al. 2016). Meanwhile, oral probiotics or microbial agent could restore the composition of gut microbiome, causing influence on the physiology and health of the host (Kuss et al. 2011; Sarı et al. 2010; Siggers et al. 2008). Food-derived microbiota consumed by seals could transiently colonize the gut and contribute to the observed differences in the gut microbiome (Nelson et al. 2013).
The giant panda (Ailuropoda melanoleuca) is one of the world’s recognizable endangered species, and well known for its specific diet (Li et al. 2010). Although it belongs to Carnivora order, the giant panda is a vegetarian taking bamboo as its principal staple food (Hu et al. 2017). Wild giant pandas forage on different bamboo species and different parts of bamboo at different times of the year (Schaller et al. 1985). Coincidently, Wu et al. found gut microbiome of wild giant panda varied by seasons due to different contents of nutrition in different parts of bamboo (Wu et al. 2017). Besides, accumulate evidences revealed microbiome in environment could shape the composition of host gut microbiome profoundly (Nelson et al. 2013; Schmidt et al. 2019). Bamboo is an important environmental factor for giant pandas, and both its nutrients and microbiomes could be varied in different species (Helander et al. 2013; Zhou et al. 2017). However, little was known about the associations between bamboo nutrition, microbiome and gut microbiome of giant panda. Thus, in our study we chose four different species of bamboo, including Pleioblastus amarus, Phyllostachys nidularia, Fargesia robusta and Bashania fangiana, to feed adult giant pandas, examined gut microbiome variation and analyzed its associations between bamboo nutrition and microbiome.
2 Material and methods
2.1 Experimental design and sampling
A total of 20 healthy captive adult giant pandas housed more than 3 months in the China Conservation and Research Center for the Giant Panda (CCRCGP) were chosen for experiment from November to December. Twenty non-relative giant pandas in our study were housed individually without contact with each other during the study. All experimental giant pandas received no antibiotics or dietary supplements from September to December. The daily intake and health status of giant pandas were monitored everyday by veterinarians during the 2-month feeding trial. The giant pandas were randomly assigned to four groups, and each group included 3 males and 2 females. All of giant pandas took bamboo Chimonobambusa qundrangularis as staple food from 1st Aug to 19th Oct., except six giant pandas from 1st Aug. to 20th Oct.. Subsequently, four different bamboo stems as staple diet were provided for feeding giant panda in each group after 21st Oct., including P. amarus (grows at an altitude of 700 m to 900 m, B1 group), P. nidularia (grows at an altitude of 800 m to1600 m, B2 group), F. robusta (grow at altitude of 1600 m to 2600 m, B3 group) and B. fangiana (grows at an altitude of 2600 m to 3500 m, B4 group). According the bamboo species, the four giant panda groups were as follows: P. amarus as diet (F1), P. nidularia as diet (F2), F. robusta as diet (F3) and B. fangiana as diet (F4). The giant pandas were put on the treatment diets about 10 days ahead of the study to avoid any complications from diet shifts during the study. Besides, 1000 g panda cakes and 200 g apples were also provided for each giant panda every day. Fresh fecal samples from each giant panda were first sampled on 1st Nov., and the bamboo preparing for feeding giant pandas were also collected the day before. Subsequently, fresh feces were collected from each giant panda in every 15 days (day 15, 30, 45 and 60), and the corresponding bamboo samples were also taken simultaneously. Five subsamples of fresh feces or bamboo preparing for feeding that from the same group at the same day were mixed into a composite sample. Finally, five composite fecal and bamboo samples were both obtained in each group. All samples were aseptically collected in sterilized plastic bags and kept cold during transport from the sampling sites to the laboratory.
2.2 Chemical analysis of bamboo samples
The fresh bamboo stem samples were dried at 70 °C. The dried samples were ground to fine powder with a high-speed universal disintegrator, and sieved through a 0.45-mm-mesh sieve before analysis. Protein content was measured using Kjeldah method (Bremner et al. 1996). Fat content was determined by Soxhlet extraction method (International AOAC 1995). The content of water soluble carbohydrate was measured based on the Anthrone method (Rondel et al. 2013). Additionally, the concentrations of hemicellulose, cellulose, and lignin were measured according to Van Soest method (Van Soest and Mcqueen 1973). All measurements were performed five times.
2.3 DNA extraction and amplicon sequencing
50 g of each fresh feces or 50 g of bamboo samples were pretreated following the method as previously described (Xue et al. 2015). Total microbial genomic DNA was extracted using the MoBio Power Fecal DNA Isolation Kit (MoBio Laboratories, Inc., Carlsbad, CA) according to the manufacturer’s instructions. Fungal DNA was extracted using E.Z.N.A.™ Fungal DNA Mini Kit (OMEGA Bio-tek, Norcross, USA) according to the manufacturer’s instructions. Successful DNA isolation was confirmed by agar gel electrophoresis. As previously described, bacterial amplicon libraries were prepared by amplifying the V4 region of 16S rRNA and fungal amplicon libraries were prepared by amplifying the ITS1 region (Huang et al. 2015). Sequencing was performed using a 250-bp paired-end sequencing protocol on the Illumina MiSeq 2500 platform (Illumina, San Diego, USA). The extraction kits and reagents in this study were used as negative (blank) controls, and no contaminant sequences were detected.
2.4 Data analysis
Paired-end reads were merged using FLASH (V1.2.7), and quality filtering of reads were performed via QIIME (V1.9.1) (Caporaso et al. 2011). Chimera sequences were removed using the UCHIME algorithm (Edgar et al. 2011; Haas et al. 2011). Tags were assigned to each sample with unique barcodes. Operational Taxonomic Units (OTUs) were clustered using UPARSE (V7.0.1001) using a 97% similarity threshold (Edgar 2013). Sequences for each OTU were referenced against the SILVA (Quast et al. 2013) and UNITE (Koljalg et al. 2013) databases to assign taxonomic classification.
Alpha diversity (Chao1 and Shannon index) were calculated with QIIME. ANOVA was used to determine the differences in alpha-diversity between groups, which were graphed using GraphPad Prism 7. Principal coordinate analysis (PCoA) based on Bray-Curtis distances were used to assess differences in bamboo or gut microbiome between individuals. The PCoA plot was graphed through ape and ggplot2 packages of R (V2.15.3). PERMANOVA was used to test for differences, and a p value less than 0.05 was considered significant. Linear Discriminant Analysis (LDA) coupled with effect size measurement (LEfSe) based on the Kruskal-Wallis sum-rank test was utilized to analyze the differences in the microbiome profile between groups, and performed using galaxy platform (http://huttenhower.sph.harvard.edu/galaxy/root) to estimate the effect size of each feature with a normalized relative abundance matrix. The Spearman analysis was used to assess the correlation between bamboo (nutrients and microbiome) and giant panda (gut microbiome), and the Network was graphed by Cytoscape (3.6.0). In addition, Kruskal-Wallis and Mann-Whitney analysis were used to determine the differences in physicochemical indexes of bamboo between different species. The Bonferroni correction was used for multiple comparisons.
3 Results
3.1 Bacterial community in gut of giant panda fed with different bamboo species
For 16S rRNA gene sequencing, the library from each fecal sample was sequenced at a minimum depth of 40,000 reads. After quality filtering and assembly, 1,349,155 high-quality sequences were obtained, and 1972 OTUs were yielded at 97% sequence similarity. The Good’s coverage of the five composite fecal samples ranged from 96.21 to 97.65%. The PCoA based on Bray-Curtis showed that fecal samples from the same group clustered together (Fig. 1a). However, no significant differences of richness (Chao1 index) and diversity (Shannon index) were observed between four groups (p > 0.05, ANOVA). Richness of F4 group was the highest, and F2 group was the lowest (Supplementary Fig. 1a). Diversity of F3 group was the highest, and F2 group was the lowest (Supplementary Fig. 1b).
The bacterial communities in the gut of giant panda with different species of bamboo diet. (a) Principal Coordinate Analysis (PCoA) of bacterial community structures of F1, F2, F3 and F4 groups. (b) The composition of bacterial community in F1, F2, F3 and F4 groups at the phylum level (the top 10 in each group). (c) The composition of bacterial community in F1, F2, F3 and F4 groups at the genus level (the top 10). (d) Bacterial taxa significantly differentiated among the F1, F2, F3 and F4 groups, identified by linear discriminant analysis coupled with effect size (LEfSe) using the default parameters. (F1, Pleioblastus amarus as diet; F2, Phyllostachys nidularia as diet; F3, Fargesia robusta as diet; F4, Bashania fangiana as diet. Red symbols represent samples of F1. Blue represent samples of F2. Green represent samples of F3. Orange represent samples of F4)
As shown in Fig. 1 and Supplementary Table1, Firmicutes (74.3%) and Proteobacteria (24.3%) were the major phyla among four groups. The dominant phylum in F1group was Proteobacteria (72.3%), which was significantly higher than other groups (2.1%, 12.6% and 10.2% in F2, F3 and F4 group, respectively) (Kruskal-Wallis test, LDA > 4). Firmicutes was the most dominant phylum in F2 (97.3%), F3 (86.5%) and F4 (86.8%) group respectively. The relative abundance of Firmicutes was abundant in F2 group (97.3%) (Kruskal-Wallis test, LDA > 4).
At the genus level, Escherichia (62.3%), Clostridium (8.6%) and Streptococcus (6.2%) were the top three genera in F1 group (Fig. 1c). Escherichia and Enterococcus was significantly higher in F1 group (62.3% and 2.1%) than other groups (0.6% and 0.01% in F2, 3.9% and 0.04% in F3, 1.0% and 0.0% in F4) (Kruskal-Wallis test, LDA > 4) (Fig. 1d). Streptococcus (89.2%) was significantly abundant (Kruskal-Wallis test, LDA > 4) and the dominant genus in F2 group, followed by Leuconostoc (4.1%) and Escherichia (0.6%). Leuconostoc (31.6%), Streptococcus (20.8%) and Clostridium (17.9%) were the top three genera in F3 group. Besides, Leuconostoc and Turicibacter was significantly abundant in F3 group (31.6% and 8.6%) than other groups (3.5% and 0.3% in F1, 4.1% and 0.03% in F2, 0.01% and 3.9% in F4) (Kruskal-Wallis test, LDA > 4). Clostridium (71.7%), Pseudomonas (5.3%) and Turicibacter (3.9%) were the top three genera in F4 group, and Clostridium significantly abundant than other groups (Kruskal-Wallis test, LDA > 4).
3.2 Fungal community in gut of giant panda fed with different bamboo species
For ITS ribosomal DNA sequencing, the library from each fecal sample was sequenced at a minimum depth of 30,000 reads. After quality filtering and assembly, 1,765,921 high-quality sequences were obtained, and 3925 OTUs were yielded at 97% sequence similarity. The Good’s coverage of the five composite fecal samples ranged from 95.60 to 96.72%. As shown in Supplementary Fig. 2, richness and diversity of F3 group was the highest, while F1 group was the lowest. The PCoA based on Bray-Curtis showed that samples from the same group clustered together (Supplementary Fig. 2c). However, no significant differences in richness and diversity were observed among four groups (p > 0.05, ANOVA).
Ascomycota (54.5%) and Basidiomycota (39.8%) were the dominant phyla among four groups. LDA analysis showed that Ascomycota and Basidiomycota were significantly higher in F4 group (73.2%) and F1 group (83.4%), respectively (Kruskal-Wallis test, LDA > 4) (Supplementary Fig. 2). At the genus level, Cryptococcus (51.0%), Cystofilobasidium (9.6%) and Candida (3.4%) were the top three genera in F1 group, and the relative abundance of Cryptococcus was significantly higher than other groups (Kruskal-Wallis test, LDA > 4). Candida (41.6%), Cystofilobasidium (5.9%) and Cryptococcus (5.0%) were the top three genera in F2 group. Candida and Rhodotorula (41.6% and 1.0%) in F2 group had significantly higher relative abundance than other groups (Kruskal-Wallis test, LDA > 4). Mrakiella (13.5%), Barnettozyma (13.0%) and Cryptococcus (8.8%) were the top three genera in F3 group. Meanwhile, the relative abundance of Mrakiella (13.5%) was significantly higher than other groups (Kruskal-Wallis test, LDA > 4). Calycina (11.7%), Cystofilobasidium (10.9%) and Mrakiella (4.6%) were the top three genera in F4 group.
3.3 Nutritional content of different bamboo species
Regardless of bamboo species, cellulose (44.4%–59.2%), hemicellulose (6.1%–25.1%) and lignin (9.2%–26.1%) were the main components of bamboo. However, the content of soluble carbohydrate (sugar) (0.6%–1.9%), protein (1.2%–3.8%), fat (0.1%–0.3%), flavone (0.03%–0.1%) and tannin (0.0002% - 0.0006%) were lower. Significant differences in the content of nutrients were found between different bamboo species (Fig. 2). Specifically, the content of cellulose, flavone, carbohydrate and tannin were significantly higher in B3 group (p < 0.05, Kruskal-Wallis and Mann-Whitney). Lignin content was significantly higher in B2 group (p < 0.05, Kruskal-Wallis and Mann-Whitney). Protein content was significantly higher in B4 group \ (p < 0.05, Kruskal-Wallis and Mann-Whitney). There was no significant difference in the content of fat and hemicellulose between different bamboo (p > 0.05, Kruskal-Wallis and Mann-Whitney).
3.4 The associations between bamboo nutrients and gut microbiome of giant panda
The associations between bamboo nutrients and gut microbiome of giant pandas were analyzed via Spearman correlation analysis. Statistically significant associations between bamboo nutrients and gut microbiome of giant pandas were confirmed (p < 0.05, Spearman) (Fig. 3). For bacterial communities, the content of carbohydrate was negatively associated with the diversity of gut microbiome (p < 0.05, Spearman) (Supplementary Table 2). At the phylum level, protein content was positively associated with the abundance of Firmicutes, while negatively associated with Proteobacteria (p < 0.05, Spearman) (Supplementary Table 3). At the genus level (the top 10 genera in each group), lignin content was positively associated with the abundance of Streptococcus (p < 0.05, Spearman). The content of flavone was positively associated with the abundance of Leuconostoc and Pedobacter (p < 0.05, Spearman). Cellulose content was positively associated with the abundance of Clostridium (p < 0.05, Spearman). Fat content was negatively associated with Rhodanobacter (p < 0.05, Spearman), and hemicellulose was negatively with Epulopiscium, Paenibacillus and SMB53 (p < 0.05, Spearman).
For fungal communities, no significant associations were found between bamboo nutrients and richness and diversity of gut microbiome (p > 0.05, Spearman) (Supplementary Table 2). At the phylum level, the content of protein and flavone were positively associated with the abundance of Ascomycota, while negatively with Basidiomycota (p < 0.05, Spearman) (Supplementary Table 3). At the genus level (the top 10 of genera in each group), protein content was positively associated with the abundance of Microdochium (p < 0.05, Spearman). Flavone content was positively associated with the abundance of Phoma, and fat was negatively associated with Cryptococcus (p < 0.05, Spearman).
3.5 Bacterial community of different bamboo
For 16S rRNA gene sequencing, the library from each bamboo sample was sequenced at a minimum depth of 30,000 reads. Totally, 1,023,750 high-quality sequences were obtained, and 5227 OTUs were yielded at 97% sequence similarity. The Good’s coverage of the five composite samples ranged from 95.12 to 96.73%. Notably, significant differences in richness and diversity were observed between different bamboo species (p < 0.05, ANOVA) (Fig. 4). Furthermore, the PCoA also showed that samples from the same bamboo clustered together. Richness of B1 and B4 group were significantly higher than B2 group (p < 0.05, ANOVA). Diversity of B1 group was significantly higher than B2 group (p < 0.05, ANOVA).
The bacterial communities among different bamboo species (*0.01 < p < 0.05, **p < 0.01). (a) The variation of bacterial richness among different bamboos. (b) The variation of bacterial diversity among different bamboos. (c) Principal Coordinate Analysis (PCoA) of bacterial community structures of different bamboo species. (d) Differences in bacterial phyla structures of different bamboo species. (e) Differences in bacterial genera structures of different bamboo species. (f) Bacterial taxa significantly differentiated among different bamboo species, identified by linear discriminant analysis coupled with effect size (LEfSe) using the default parameters(B1: Pleioblastus amarus, B2: Phyllostachys nidularia, B3: Fargesia robusta and B4: Bashania fangiana. Red symbols represent samples of B1, Blue represent samples of B2, Green represent samples of B3, Orange represent samples of B4)
As shown in Fig. 4, Proteobacteria (53.5% in B1, 92.3% in B2, 83.4% in B3 and 82.5% in B4) was the most prevalent phylum regardless of bamboo species, and significantly higher in B2 group (92.3%) (Kruskal-Wallis test, LDA > 4). Most remainder sequences of B1 group belonged to phyla Firmicutes (16.6%), Acidobacteria (10.8%) and Planctomycetes (6.2%). At the genus level, the composition of main genera varied by bamboo species. Acinetobacter (11.3%), Exiguobacterium (9.6%) and Dyella (4.3%) were the top three genera in B1 group. Besides, the abundance of Acinetobacter (11.3%), Exiguobacterium (9.6%), Dyella (4.3%), Bryocella (4.2%), Lactococcus (3.2%) and Singulisphaera (3.0%) were significantly higher than other groups (Kruskal-Wallis test, LDA > 4). Pseudomonas (33.3%), Methylobacterium (9.4%) and Acinetobacter (8.9%) were top three genera in B2 group. Pseudomonas, Methylobacterium and Pantoea were significantly higher in B2 group (33.3%, 9.4% and 8.8%) (Kruskal-Wallis test, LDA > 4). Pseudomonas (20.0%), Acidiphilium (5.8%) and Methylobacterium (3.7%) were top three genera in B3 group, and Acidiphilium was significantly higher than other groups (Kruskal-Wallis test, LDA > 4) (Fig. 4e, f). Pseudomonas (18.4%), Acidiphilium (4.4%) and Granulicella (3.2%) were top three genera in B4 group. Notably, Granulicella in B4 (3.2%) had significantly higher abundance than other groups (Kruskal-Wallis test, LDA > 4) (Fig. 4f).
3.6 The associations between bamboo bacteria and gut bacteria of giant panda
There were 72 genera both shared between B1 and F1, 56 genera between B2 and F2, 63 genera between B3 and F3, and 70 genera between B4 and F4. Totally, 36 mutual genera were found in four matched groups, accounted for 16.2%–20.8% in the gut of giant panda and 12.8%–16.4% of bamboo (Supplementary Tables 4, and 5). For example, Pseudomonas (18.7% in bamboo and 1.5% in giant panda), Acinetobacter (6.0% in bamboo and 0.8% in giant panda), Methylobacterium (3.6% in bamboo and 0.02% in giant panda), Pantoea (2.5% in bamboo and 0.03% in giant panda), Stenotrophomonas (2.2% in bamboo and 0.04% in giant panda), Sphingomonas (1.7% in bamboo and 0.02% in giant panda), Hymenobacter (1.1% in bamboo and 0.01% in giant panda) and Streptococcus (0.01% in bamboo and 29.7% in giant panda) were both found in bamboo and the gut of giant panda. Particularly, the relative abundance of Acinetobacter in bamboo was positively associated with that of Acinetobacter in gut of giant panda (p < 0.05, Spearman). Nevertheless, Pseudomonas in bamboo had negative association with Pseudomonas in gut of giant panda (p < 0.05, Spearman).
The diversity of bamboo bacteria was positively correlated with that of gut bacterial community in giant panda (p < 0.05, Spearman). Significant associations of the relative abundance between bamboo and gut bacteria in giant panda were also found (p < 0.05, Spearman). At the genus level (the top 10 genera in bamboo and giant panda group), there were 24 significant associations (p < 0.05, Spearman) between bamboo and gut of giant panda (Fig. 5).
3.7 Fungal microbial community of different bamboo species
For ITS ribosomal DNA sequencing, the library from each bamboo sample was sequenced at a minimum depth of 25,000 reads. 848,925 fungal high-quality sequences were obtained, and 2102 OTUs were yielded at 97% sequence similarity. The Good’s coverage of the five composite samples ranged from 94.80 to 95.27%. As shown in Supplementary Fig. 3, there were no significant differences of richness and diversity between different bamboo species (p > 0.05, ANOVA). However, the PCoA showed that samples from the same group clustered together.
Ascomycota (72.8%) was the most dominant phylum, followed by Basidiomycota (11.9%) regardless of bamboo species. LDA analysis showed that Ascomycota and Basidiomycota were higher in B1 group (81.8%), and B4 group (24.9%), respectively (Kruskal-Wallis test, LDA > 4) (Supplementary Fig. 3). At the genus level, Shiraia (12.0%), Ceramothyrium (11.7%) and Cryptococcus (8.6%) were the top three genera in B1 group. The relative abundance of Shiraia and Ceramothyrium in B1 (12.0% and 11.7%) were significantly higher other groups (Kruskal-Wallis test, LDA > 4). Ramichloridium (22.6%), Cryptococcus (4.1%) and Zymoseptoria (1.0%) were the top three genera in B2 group, and Ramichloridium was significantly higher than other groups (Kruskal–Wallis test, LDA > 4). Rachicladosporium (8.9%), Bacidia (6.8%) and Cryptococcus (6.2%) were the top three genera in B3 group. Rachicladosporium, Bacidia, Rhinocladiella, Libertella and Strelitziana were significantly higner in B3 (8.9%, 6.8%, 5.4%, 2.4% and 2.0%) than other groups (Kruskal-Wallis test, LDA > 4). Cryptococcus (22.8%), Mycosphaerella (2.6%) and Libertella (1.2%) were the top two genera in B4 group, and Cryptococcus and Mycosphaerella were significantly higher than other groups (Kruskal-Wallis test, LDA > 4).
3.8 The associations between bamboo fungi and gut fungi of giant panda
There were 73 genera shared between B1 and F1, 99 genera between B2 and F2, 113 genera between B3 and F3, and 116 genera between B4 and F4. Totally, 54 mutual genera were found in four matched groups, accounted for 9.8%–15.5% in the gut of giant panda and 26.7%–42.5% of bamboo (Supplementary Tables 6 and 7). For example, Cryptococcus (16.3% in bamboo and 10.4% in giant panda), Mrakiella (4.7% in bamboo and 0.01% in giant panda), Ramichloridium (0.03% in bamboo and 7.9% in giant panda), Shiraia (0.7% in bamboo and 3.2% in giant panda), Ceramothyrium (0.01% in bamboo and 3.0% in giant panda) and Rhinocladiella (0.3% in bamboo and 1.8% in giant panda) were both found in bamboo and the gut of giant panda. Especially, the relative abundance of Cephalosporium in bamboo was positively associated with that of Cephalosporium in gut of giant panda (p < 0.05, Spearman).
No significant associations were found between fungi in bamboo and gut of giant panda for the diversity and at phylum level (p > 0.05, Spearman). However, 32 significant associations between the fungi in bamboo and gut of giant panda at genus level were found (top 10 genera, p < 0.05, Spearman) (Fig. 6).
4 Discussion
Diet conversion could affect gut microbiome of giant panda in diversity and composition (Zhang et al. 2018a), as well as in humans (Bäckhed et al. 2015), pig (Frese et al. 2015), mice (Hildebrandt et al. 2009) and rainbow trout (Michl et al. 2017). Bamboo as a staple food for giant pandas has high fiber (cellulose, lignin and hemicellulose) and low carbohydrate, fat, flavone and tannin food (Wang et al. 2017). The content of cellulose, lignin, carbohydrate, flavone and tannin strongly altered by bamboo species in our study. No significant differences in content of fat and hemicellulose were found between different bamboo species, indicating that they may be more stable in bamboo. Vegetable fat was important for growth, which could provide the calories, vitamins and essential fatty acids (Akinoso et al. 2012). Zhang et al. demonstrated that giant panda lives on hemicellulose in bamboo with the contribution of gut microbiota in gut of giant panda (Zhang et al. 2018c). Hence, we hypothesized that fat and hemicellulose in bamboo may be crucial for growth and development of giant panda.
Previous studies showed that nutrient of diet could shape gut microbiome in human (Carlotta et al. 2010) and mice (Daniel et al. 2014). In our study, a substantial effect of bamboo species variation on gut microbiome of giant panda was observed, and significant correlations were found (p < 0.05, Spearman). As previously described, seasonal variation in nutrient utilization shapes gut microbiome structure and function of giant pandas (Wu et al. 2017). Herein, we found carbohydrate decreased the diversity of gut bacterial communities in giant panda. Similar result was reported in human that a higher content of carbohydrates in the diet was associated with lower microbiome diversity (Zhernakova et al. 2016). This may due to synergistic growth was absent in carbohydrate medium, but common in lignocellulose medium (Deng and Wang 2016). In addition, Firmicutes and Proteobacteria were dominant phyla in gut of giant pandas (Shengzhi et al. 2018, Wei et al. 2015, Xue et al. 2015, Zhang et al. 2018), indicating their roles in utilization of lignocellulose for giant pandas (Lifeng et al. 2011, Shengzhi et al. 2018).
Moreover, many significant associations between bamboo nutrients and gut bacteria at genera level were observed. The content of cellulose was positively associated with Clostridium (p < 0.05, Spearman), which also supported that conclusion that Clostridium could help giant panda digest cellulose (Lifeng et al. 2011).In our study, Streptococcus was dominant in gut of giant panda and positively associated with lignin content (p < 0.05, Spearman). As we know, breakage in the lignin structure is necessary for opening the structure of the lignocellulosic materials so that the cellulose fibrils become exposed, and giant panda can utilize the cellulose and hemicellulose of bamboo (Fang and Jr 2016). Streptococcus was also the common mucosal-associated taxa found associated in the gut of giant panda (Williams et al. 2013). The thick layer of mucus in gut of giant pandas could protect their stomachs from the bamboo splinters and aid the intake of high-fiber diet (Montagne et al. 2003). Streptococcus probably aids in the digestion of lignin and is considered an example of gut microbiota adaptation to highly fibrous bamboo diet.
We found bamboo nutrients had no significant associations with richness and diversity of gut fungi in giant panda (p > 0.05, Spearman). We also speculated gut bacterial communities may contribute more than fungal communities to bamboo utilization for giant panda (Zhang et al. 2018). As previously described, the most dominant fungal phylum in giant panda was Ascomycota, followed by Basidiomycota (Tun et al. 2014; Zhang et al. 2018). In giant panda vaginas, Chen et al. demonstrated Basidiomycota and Ascomycota were also the dominant phyla (Chen et al. 2018). In our study, we found that the content of flavone and protein in bamboo was positively associated with the abundance of Ascomycota in giant panda, but negatively associated with the Basidiomycota (p < 0.05). Flavonoid in bamboo could be also associated with reproductive hormone levels of giant pandas, and the total flavonoids of bamboo may play a distinct role in the reproductive success of giant pandas (Liu et al. 2019). Thus, we speculated the flavonoids in bamboo may be related with reproduction of giant panda, which might possess a sensitive adaptation to phytoestrogens from bamboo diet.
Interestingly, genus Microdochium belonged to phylum Ascomycota, and the variation of the abundance of Microdochium within four groups was in accord with Ascomycota. The content of bamboo protein was positively correlated with Microdochium in giant panda (p < 0.05, Spearman), revealed that protein in bamboo can influence the relative abundance of intestinal fungi Microdochium in giant panda. It should be noted that Microdochium was important plant fungi (Aveskamp and Gruyter 2008; Liu et al. 2016), and both found in bamboo and in the gut of giant panda with a high relative abundance in our study.
The microbial species more exposure to host, and more likely to persist in the host gut (Schmidt et al. 2019). Whether oral probiotic or fecal transplants technology reflected microbiome in diet could influence the composition of gut microbiome in humans (Borody and Alexander 2012; Kuss et al. 2011). Changes in habitat may influence endophytic fungi of different bamboo species in giant panda diet (Helander et al. 2013), and we found the composition of bamboo microbiome varied by bamboo species. Many mutual microbes were shared between bamboo and giant panda, and significant associations between bamboo microbiome and gut microbiome of giant panda were observed in our study, reflecting the gut microbial community appears to be influenced by intake of different bamboo harboring different composition of microbes. Similar findings had been shown in insects acquiring microbiome from the inhabit soil (Hannula et al. 2019).
For bacterial communities, we found giant panda eating bamboo with higher diversity of bacteria had higher diversity of gut bacteria (p < 0.05, Spearman). The diet associated microbes represent a source of potential colonists for host (Bolnick et al. 2014). Bamboo with high diversity of microbiome may be benefit for improving diversity of gut microbiome in giant panda, conferring higher resilience (Larsen and Claassen 2018). In our study, Proteobacteria was the most dominant phylum in bamboo. It was also the majority of bamboo root endophytic bacteria, and the major phylum in bamboo soil (Han et al. 2009). Coincidently, Proteobacteria was the prevalent phylum in the gut of captive and wild giant pandas (Guo et al. 2018; Wei et al. 2015; Zhang et al. 2018). Pseudomonas belonged to Proteobacteria and were shared between bamboo and giant panda, and had positive correlation with Streptococcus in gut of giant panda (p < 0.05, Spearman). Pseudomonas was the main genus in bamboo, and Streptococcus was the major genus in giant pandas (Xue et al. 2015). It worthy noted that Pseudomonas was found be involved in metabolism of lignin (Jiménez et al. 2015), while lignin of bamboo had positive association with Streptococcus (p < 0.05, Spearman). We hypothesized the genes involved in lignin digestion may transfer horizontally, and further research was needed to confirm the findings herein. We also observed Pseudomonas in bamboo had negative correlation with Escherichia in gut of giant panda (p < 0.05, Spearman). E. coli is a common opportunistic pathogen and it could cause gut infections in giant pandas (Zhou et al. 2017). Thus, this negative association may be benefit for keeping the health of giant panda, and we speculated that microbiome of bamboo may be one channel for giant panda to stable gut microbiome (Mueller et al. 2015).
For fungal communities, no significant associations were found between bamboo and in gut of giant panda for the diversity and at phylum level (p > 0.05, Spearman). Ascomycota and Basidiomycota were dominant phyla of bamboo and in the gut of giant panda (Tun et al. 2014; Zhou et al. 2017). The dominant phyla in human (Christian et al. 2013), dog (Stefanie et al. 2011), soil (Dang et al. 2017) and near-surface atmosphere (Bowers et al. 2013) was also the Ascomycota and Basidiomycota, suggesting that they might be better in colonising a wide range of environments. At the genus level, some genera were shared between bamboo and giant panda, including Candida, Ramichloridium, Devriesia and Mrakiella. Interestingly, Candida was also found in vagina of giant panda, and positively associated with diets high in carbohydrates (Chen et al. 2018; Christian et al. 2013). Ramichloridium had positively correlation with Candida in gut of giant panda(p < 0.05, Spearman), but little was known about Ramichloridium (Arzanlou et al. 2007). We speculated Ramichloridium may synergy with Candida to utilize carbohydrates. Meanwhile, yeast strains Mrakiella were isolated from cold habitats, like superficial and deep sediments, ice cores and meltwaters (Branda et al. 2010). Previous study found the trail of giant panda and bamboo in high elevational areas with −14 °C lower temperature (Liu et al. 2015; Schaller et al. 1985), reflecting microbes on bamboo could be one source of the gut microbiome in giant panda (Mueller et al. 2015; Schmidt et al. 2019).
5 Conclusions
Overall, our study illustrated the relationship between bamboo nutrients and microbiome and the gut microbiome of giant pandas. The composition of gut microbiome in giant panda varied among groups with different bamboo diet. The content of cellulose, lignin, carbohydrate, protein, flavone and tannin were significantly different between different bamboo species (p < 0.05). Significant differences in richness and diversity of bacteria were observed between different bamboo (p < 0.05), as well as the abundance of the main bacteria and fungi (Kruskal-Wallis, LDA > 4). The diversity of bamboo bacteria was positively correlated with that of gut bacteria in giant panda (p < 0.05). Significant associations of certain bacteria and fungi were found between bamboo and gut of giant panda (p < 0.05). 36 bacterial and 54 fungal genera were both shared between bamboo and the gut of giant panda in all groups. Our results revealed that the gut microbial community varied between giant pandas that fed on different bamboo species, revealing that bamboo nutrients and microbiome could affect gut microbiome of giant panda.
Understanding of how bamboo nutrition and microbial composition influence gut microbiome of the giant pandas may be valuable in increasing the effectiveness of the captive breeding programs for giant pandas. Our study further complemented researches on the factors which influence gut microbiome of giant panda, and could help improve the survival rates of giant pandas. Up to now, there is little data regarding the function of these gut microbiomes. Thus, we suggested that further work is needed to understand microbial functions and bamboo microbiome’s effects on gut microbiome, especially those microbes with higher relative abundance and significant associations.
References
Akinoso R, Aboaba S, Olajide W (2012) Optimization of roasting temperature and time during oil extraction from orange ( Citrus sinensis ) seeds: a response surface methodology approach. Afr J Food Agric Nutri Develop 11(6):5300–5317
Arzanlou M, Groenewald JZ, Gams W, Braun U, Shin HD, Crous PW (2007) Phylogenetic and morphotaxonomic revision of Ramichloridium and allied genera. Stud Mycol 58:57–93
Aveskamp MM, Gruyter CPW (2008) Biology and recent developments in the systematics of Phoma, a complex genus of major quarantine significance. Fungal Divers 31:1–18
Bäckhed F, Ding H, Wang T, Hooper LV, Gou YK, Nagy A et al (2004) The gut microbiome as an environmental factor that regulates fat storage. Proc Natl Acad Sci U S A 101:15718–15723
Bäckhed F, Roswall J, Peng Y, Feng Q, Jia H, Kovatchevadatchary P et al (2015) Dynamics and stabilization of the human gut microbiome during the first year of life. Cell Host Microbe 17:690–703
Bolnick DI, Snowberg LK, Hirsch PE, Lauber CL, Rob K, Gregory J, C et al (2014) Individuals' diet diversity influences gut microbial diversity in two freshwater fish (threespine stickleback and Eurasian perch). Ecol Lett 17:979–987
Borody TJ, Alexander K (2012) Fecal microbiome transplantation and emerging applications. Nat Rev Gastroenterol Hepatol 9:88–96
Bowers RM, Nicholas C, Emerson JB, Christine W, Hannigan MP, Noah F (2013) Seasonal variability in bacterial and fungal diversity of the near-surface atmosphere. Environ Sci Tech 47:12097
Branda E, Turchetti B, Diolaiuti G, Pecci M, Smiraglia C, Buzzini P (2010) Yeast and yeast-like diversity in the southernmost glacier of Europe (Calderone glacier, Apennines, Italy). FEMS Microbiol Ecol 72:354–369
Bremner JM, Sparks DL, Page AL, Helmke PA, Loeppert RH, Soltanpour PN et al (1996) Nitrogen - total. In: Sparks D et al (eds) In methods ofsoil analysis. Part 3: chemical methods, Madison, Soil ScienceSociety of America and American Society of Agronomy, pp 1085–1123
Caporaso JG, Lauber CL, Walters WA, Berg-Lyons D, Lozupone CA, Turnbaugh PJ, Fierer N, Knight RJ (2011) Global patterns of 16S rRNA diversity at a depth of millions of sequences per sample. Proc Natl Acad Sci USA 108:4156–4122
Carlotta DF, Duccio C, Monica DP, Matteo R, Jean Baptiste P, Sebastien M et al (2010) Impact of diet in shaping gut microbiome revealed by a comparative study in children from Europe and rural Africa. Proc Natl Acad Sci U S A 107:14691–14696
Chen D, Li C, Feng L, Zhang Z, Zhang H, Cheng G, Li D, Zhang G, Wang H, Chen Y, Feng M, Wang C, Wu H, Deng L, Ming H, Yang X (2018) Analysis of the influence of living environment and age on vaginal fungal microbiome in giant pandas (Ailuropoda melanoleuca) by high throughput sequencing. Microb Pathog 115:280–286
Christian H, Serena D, Stephanie G, Jun C, Hongzhe L, W. G D et al (2013) Archaea and Fungi of the human gut microbiome: correlations with diet and bacterial residents. PLoS One 8:e66019
Claesson MJ, Jeffery IB, Conde S, Power SE, O'Connor EM, Cusack S, Harris HM, Coakley M, Lakshminarayanan B, O'Sullivan O, Fitzgerald GF, Deane J, O'Connor M, Harnedy N, O'Connor K, O'Mahony D, van Sinderen D, Wallace M, Brennan L, Stanton C, Marchesi JR, Fitzgerald AP, Shanahan F, Hill C, Ross RP, O'Toole PW (2012) Gut microbiome composition correlates with diet and health in the elderly. Nature 488:178–184
Dang P, Yu X, Le H, Liu J, Shen Z, Zhao Z (2017) Effects of stand age and soil properties on soil bacterial and fungal community composition in Chinese pine plantations on the loess plateau. PLoS One 12:e0186501
Daniel H, Gholami AM, Berry D, Desmarchelier C, Hahne H, Loh G, Mondot S, Lepage P, Rothballer M, Walker A, Böhm C, Wenning M, Wagner M, Blaut M, Schmitt-Kopplin P, Kuster B, Haller D, Clavel T (2014) High-fat diet alters gut microbiome physiology in mice. ISME J 8:295–308
Deng YJ, Wang SY (2016) Synergistic growth in bacteria depends on substrate complexity. J Microbiol 54:23–30
Edgar RC (2013) UPARSE: highly accurate. OTU sequences from microbial amplicon reads. Nat Meth 10(10):996–998
Edgar RC, Haas BJ, Clemente JC, Quince C, Knight R (2011) UCHIME improves sensitivity and speed of chimera detection. Bioinformatics 27(16):2194–2200
Fang Z, Smith RL Jr (2016). Production of biofuels and chemicals from lignin
Frese S. A, Parker K, Calvert C. C, Mills D. A (2015). Diet shapes the gut microbiome of pigs during nursing and weaning. Microbiome,3,1(2015-07-01)3: 28
Guo M, Chen J, Li Q, Fu Y, Fan G, Ma J et al (2018) Dynamics of gut microbiome in Giant panda cubs reveal transitional microbes and pathways in early life. Front Microbiol 9:3138
Haas BJ, Gevers D, Earl AM, Feldgarden M, Ward DV, Giannoukos G, Ciulla D, Tabbaa D, Highlander SK, Sodergren E, Methé B, DeSantis T, Human Microbiome Consortium, Petrosino JF, Knight R, Birren BW (2011) Chimeric 16S rRNA sequence formation and detection in sanger and 454-pyrosequenced PCR amplicons. Genome Res 21:494–504
Han J, Xia D, Li L, Lei S, Kai Y, Zhang L (2009) Diversity of Culturable Bacteria isolated from root domains of Moso bamboo (Phyllostachys edulis). Microb Ecol 58:363–373
Hannula SE, Zhu F, Heinen R (2019) Foliar-feeding insects acquire microbiomes from the soil rather than the host plant. Nat Commun 10:1254
Helander M, Huitu O, Sieber TN, Jia J, Niemelä P, Saikkonen K (2013) Endophytic fungi and silica content of different bamboo species in giant panda diet. Symbiosis 61:13–22
Hildebrandt M. A, Hoffmann C, Sherrill-Mix S. A, Keilbaugh S. A, Hamady M, Chen Y. Y et al (2009). High-fat diet determines the composition of the murine gut microbiome independently of obesity. Gastroenterology137: 1716-1724.e1711-1712
Hu Y, Wu Q, Ma S, Ma T, Shan L, Wang X, Nie Y, Ning Z, Yan L, Xiu Y, Wei F (2017) Comparative genomics reveals convergent evolution between the bamboo-eating giant and red pandas. Proc Natl Acad Sci U S A 114:1081–1086
Huang X, Liu L, Wen T, Zhu R, Zhang J, Cai Z (2015) Illumina MiSeq investigations on the changes of microbial community in the Fusarium oxysporum f.sp. cubense infected soil during and after reductive soil disinfestation. Microbiol Res 181:33–42
International AOAC (1995). Official methods of analysis of AOAC international, 16th edition. Volume 1
Jain A, Xin HL, Wei NC (2018) Similarities and differences in gut microbiome composition correlate with dietary patterns of Indian and Chinese adults. AMB Express 8:104
Jiménez DJ, Chavesmoreno D, Elsas JDV (2015) Unveiling the metabolic potential of two soil-derived microbial consortia selected on wheat straw. Sci Rep 5:1–17
Koljalg U, Nilsson RH, Abarenkov K, Tedersoo L, Taylor AF, Bahram M et al (2013) Towards a unified paradigm for sequence-based identification of fungi. Mol Ecol 22:5271–5277
Kuss SK, Best GT, Etheredge CA, Pruijssers AJ, Frierson JM, Hooper LV, Dermody TS, Pfeiffer JK (2011) Intestinal microbiome promote enteric virus replication and systemic pathogenesis. Science 334:249–252
Larsen OFA, Claassen E (2018) The mechanistic link between health and gut microbiome diversity. Sci Rep 8:2183
Li R, Fan W, Tian G, Zhu H, He L, Cai J et al (2010) The sequence and de novo assembly of the Giant panda genome. Nature 463:311
Lifeng Z, Qi W, Jiayin D, Shanning Z, Fuwen W (2011) Evidence of cellulose metabolism by the giant panda gut microbiome. Proc Natl Acad Sci U S A 108:17714–17719
Liu X, Wang T, Wang T, Skidmore AK, Songer M (2015) How do two giant panda populations adapt to their habitats in the Qinling and Qionglai Mountains, China. Environ Sci Pollut Res 22:1175–1185
Liu Y, Zachow C, Raaijmakers JM, De BI (2016) Elucidating the diversity of aquatic Microdochium and Trichoderma species and their activity against the fish pathogen Saprolegnia diclina. Int J Mol Sci 17:140
Liu H, Zhang C, Liu Y, Duan H (2019) Total flavonoid contents in bamboo diets and reproductive hormones in captive pandas: exploring the potential effects on the female giant panda (Ailuropoda melanoleuca). Conservation physiology7:coy068
Maurice CF, Knowles SC, Ladau J, Pollard KS, Fenton A, Pedersen AB, Turnbaugh PJ (2015) Marked seasonal variation in the wild mouse gut microbiome. ISME J 9:2423–2434
Michl SC, Ratten JM, Beyer M, Hasler M, Laroche J, Schulz C (2017) The malleable gut microbiome of juvenile rainbow trout (Oncorhynchus mykiss): diet-dependent shifts of bacterial community structures. PLoS One 12:e0177735
Montagne L, Pluske JR, Hampson DJ (2003) A review of interactions between dietary fibre and the intestinal mucosa, and their consequences on digestive health in young non-ruminant animals [review]. Animal Feed Science & Technology 108:95–117
Mueller NT, Bakacs E, Combellick J, Grigoryan Z, Dominguez-Bello MG (2015) The infant microbiome development: mom matters. Trends Mol Med 21:109–117
Nelson TM, Rogers TL, Carlini AR, Brown MV (2013) Diet and phylogeny shape the gut microbiome of Antarctic seals: a comparison of wild and captive animals. Environ Microbiol 15:1132–1145
Nicholson JK, Elaine H, James K, Remy B, Glenn G, Wei J et al (2012) Host-gut microbiome metabolic interactions. Science 336:1262–1267
Persson IL, Wikan S, Swenson JE, Mysterud I (2001) The diet of the brown bear Ursus arctos in the Pasvik Valley northeastern Norway. Wildl Biol 7:27–37
Quast C, Pruesse E, Yilmaz P, Gerken J, Schweer T, Yarza P, Peplies J, Glöckner FO (2013) The SILVA ribosomal RNA gene database project: improved data processing and web-based tools. Nucleic Acids Res 41:D590–D596
Rondel C, Marcato-Romain CE, Girbal-Neuhauser E (2013) Development and validation of a colorimetric assay for simultaneous quantification of neutral and uronic sugars. Water Res 47:2901–2908
Sarı FN, Dizdar EA, Oguz S, Erdeve O, Uras N, Dilmen U (2010) Oral probiotics: Lactobacillus sporogenes for prevention of necrotizing enterocolitis in very low-birth weight infants: a randomized, controlled trial. Eur J Clin Nutr 86:S87–S87
Schaller GB, Hu JC, Pan WS, Zhu J (1985) The giant panda of Wolong. University of Chicago Press, Chicago
Schmidt E, Mykytczuk N, Schulte-Hostedde AI (2019) Effects of the captive and wild environment on diversity of the gut microbiome of deer mice (Peromyscus maniculatus). ISME J 13:1293–1305
Scott KP, Gratz SW, Sheridan PO, Flint HJ, Duncan SH (2013) The influence of diet on the gut microbiome. Pharmacol Res 69:52–60
Shengzhi Y, Xin G, Meng J, Zhang A, Zhou Y, Long M, Li B, Deng W, Jin L, Zhao S, Wu D, He Y, Li C, Liu S, Huang Y, Zhang H, Zou L (2018) Metagenomic analysis of bacteria, fungi,bacteriophages, and helminths in the gut of giant pandas. Front Microbiol 69:1717
Siggers R, Siggers JM, Thymann T, Molbak L, Leser T, Jensen B et al (2008) Early administration of probiotics alters bacterial colonization and limits diet-induced gut dysfunction and severity of necrotizing enterocolitis in preterm pigs. J Nutr 138:1437–1444
Simpson HL, Campbell BJ (2015) Review article: dietary fibre-microbiome interactions. Aliment Pharmacol Ther 42:158–179
Stefanie H, Dowd SE, Garcia-Mazcorro JF, Steiner JRM, Suchodolski JS (2011) Massive parallel 16S rRNA gene pyrosequencing reveals highly diverse fecal bacterial and fungal communities in healthy dogs and cats. FEMS Microbiol Ecol 76:301–310
Tremaroli V, Backhed F (2012) Functional interactions between the gut microbiome and host metabolism. Nature 71:242–249
Tun HM, Mauroo NF, Yuen CS, Ho JC, Wong MT, Leung FC (2014) Microbial diversity and evidence of novel homoacetogens in the gut of both geriatric and adult giant pandas (Ailuropoda melanoleuca). PLoS One 9:e79902
Van Soest PJ, Mcqueen RW (1973) The chemistry and estimation of fibre. Proc Nutr Soc 32:123–130
Wang H, Zhong H, Hou R, Ayala J, Liu G, Yuan S et al (2017) A diet diverse in bamboo parts is important for Giant panda ( Ailuropoda melanoleuca ) metabolism and health. Sci Rep 7:3377
Wei F, Wang X, Wu Q (2015) The giant panda gut microbiome. Trends Microbiol 23:450–452
Williams CL, Willard S, Kouba A, Sparks D, Holmes W, Falcone J, Williams CH, Brown A (2013) Dietary shifts affect the gastrointestinal microflora of the giant panda (Ailuropoda melanoleuca). J Ani Physiol Ani Nutri 97:577–585
Wu Q, Wang X, Ding Y, Hu Y, Nie Y, Wei W et al (2017) Seasonal variation in nutrient utilization shapes gut microbiome structure and function in wild giant pandas. Proceedings Biological Sciences 284:20170955
Xue Z, Zhang W, Wang L, Hou R, Zhang M, Fei L et al (2015) The bamboo-eating Giant panda harbors a carnivore-like gut microbiome, with excessive seasonal variations. mBio6:00022–00015
Zhang W, Liu W, Hou R, Zhang L, Schmitz-Esser S, Sun H et al (2018). Age-associated microbiome shows the giant panda lives on hemicelluloses, not on cellulose. ISME J 12:1319–1328
Zhernakova A, Kurilshikov A, Bonder MJ, Tigchelaar EF, Schirmer M, Vatanen T et al (2016) Population-based metagenomics analysis reveals markers for gut microbiome composition and diversity. Science 352:565
Zhou YK, Shen XY, Hou CL (2017) Diversity and antimicrobial activity of culturable fungi from fishscale bamboo ( Phyllostachys heteroclada ) in China. World Journal of Microbiology & Biotechnology 33:104
Zoetendal EG, Raes J, van den Bogert B, Arumugam M, Booijink CC, Troost FJ, Bork P, Wels M, de Vos WM, Kleerebezem M (2012) The human small intestinal microbiome is driven by rapid uptake and conversion of simple carbohydrates. ISME J 6:1415–1426
Acknowledgements
This work was supported by Open Project of SFGA on Conservation Biology of Rare Animals in the Giant Panda National Park (CCRCGP, No. KLSFGAGP2020.003), Scientific Backbone Research Project (10912-2019KYGG201530) and Postdoctoral Science Foundation of Chengdu University of Technology.
Author information
Authors and Affiliations
Corresponding authors
Additional information
Publisher’s note
Springer Nature remains neutral with regard to jurisdictional claims in published maps and institutional affiliations.
Rights and permissions
About this article
Cite this article
Jin, L., Wu, D., Li, C. et al. Bamboo nutrients and microbiome affect gut microbiome of giant panda. Symbiosis 80, 293–304 (2020). https://doi.org/10.1007/s13199-020-00673-0
Received:
Accepted:
Published:
Issue Date:
DOI: https://doi.org/10.1007/s13199-020-00673-0