Abstract
This study examines the influence of lactic acid fermentation on iridoid and polyphenolic compounds in unripe Cornus mas L. fruits. Fermentation was carried out at room temperature over 6 weeks. Eight versions of the fermentation process were conducted: with inoculation by microflora isolated from black olives, Lb. brevis 18 M, Lb. casei 0919, Lb. paracasei 920, Lb. rhamnosus 0900, Lb. rhamnosus 0908, and spontaneous fermentation with and without summer savory (Satureja hortensis). The polyphenolic compounds, iridoids and antioxidant activities were examined in the products obtained. After fermentation, the polyphenols detected were: gallic acid and one of its derivatives, protocatechuic and ellagic acids, neochlorogenic and chlorogenic acids, and a chlorogenic acid derivative. Loganic acid and cornuside were found to be present in both the fermented fruits and the brines. The tested microorganisms produced varying amounts of lactic acid and had varying degrees of impact on the content of biologically active compounds. Lb. rhamnosus 908 proved to be the most effective strain. Unripe cornelian cherries fermented with probiotic strains could be used as an innovative pro-health product.
Similar content being viewed by others
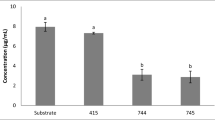
Explore related subjects
Discover the latest articles, news and stories from top researchers in related subjects.Avoid common mistakes on your manuscript.
Introduction
The cornelian cherry (Cornus mas L.) belongs to the family Cornaceae. It is a wild plant that grows in Asia and Europe. It ranges in size from a shrub to a small tree of about 7–8 m in height. The fruits are suitable for fresh consumption or can be processed to produce syrups, juices, jams, spirits and other traditional products (Kucharska 2012; Sokół-Łętowska et al. 2014). Since ancient times, the unripe fruits of Cornus mas L. have also been pickled as olive substitutes. In Poland, pickled cornelian cherries were very popular in the nineteenth century, and are now manufactured by Arboretum in Bolestraszyce as a traditional product (Kucharska et al. 2011; Kucharska 2012). Kucharska et al. (2011) studied the composition of fermented fruits made with the addition of pure cultures of lactic acid bacteria (LAB) Lactobacillus casei and Lactobacillus acidophilus, with and without the addition of various spices. These authors observed that both strains and herbs affected the quality of the finished product. However, they did not study the effect of fermentation on the content of individual active compounds in cornelian cherries, such as polyphenols or iridoids.
Phenolic compounds are important constituents of food products of plant origin. These compounds are directly related to sensory characteristics of foods such as flavour, astringency and colour. In addition, due mainly due to their antioxidant activities, phenolic compounds have chemopreventive activities against carcinogenesis and mutagenesis, so their presence in the diet can have health benefits.
Iridoids are a little-tested class of bioactive compounds which occur in plant and animal material. They are a type of cyclopentane monoterpene, with a basic skeleton composed of a cyclopentane ring coupled with a heterocyclic pyran ring. The subclass of secoiridoids has cleaved bonds in the cyclopentane ring between carbon atoms C-7 and C-8. Secoiridoids are bitter and deter herbivores. They occur predominantly in the form of glycosides and less frequently as non-sugar iridoids, for example in the form of esters, acetals and hemiacetals (Dewick 2009). Depending on their structure, they may exhibit antibiotic, anti-inflammatory or hypertensive activities. Secoiridoids are found mainly in leaves, but are also present in young stems and very rarely in fruits. Fruits of the genera Cornus are one of only a few in which iridoids occur (Kucharska 2012; Deng et al. 2013).
Lactic acid fermentation, an ancient method of preservation, is today valued primarily as a “natural” process, used to increase the shelf life of various products. For fermented milk and meat products, instead of spontaneous lactic acid fermentation, selected starter cultures are now commonly used to control the process (Settanni and Moschetti 2014). These strains can be derived from the human gastrointestinal tract and exhibit probiotic properties. Currently, most probiotic products belong to dairy product groups, but lactose intolerance and the cholesterol content may deter consumers. Therefore, there is growing interest in the possibility of using fruits and vegetables for the preparation of probiotic products, with industry so far focusing on fermentation of cucumbers, cabbages and olives using LAB. Different lactic acid bacteria require different amino acids and vitamins for growth, and it has been found that some probiotic strains have the ability to grow in fruit matrices. Consumer trends with respect to food choices are changing, due to increasing awareness of the link between diet and health. In Europe, the largest segment of the functional food market comprises foods fortified with probiotics, prebiotics and synbiotics.
The addition of probiotic bacteria to fruits and vegetables which already contain large amounts of bioactive substances may bring additional health benefits (Blana et al. 2014). In recent years, substantial research has been undertaken to clarify the technological properties of LAB that can be used to obtain functional food from olives (Abriouel et al. 2012; Argyri et al. 2013; Bautista-Gallego et al. 2013). The ability of LAB to colonize the surface of olives and form biofilms during fermentation has opened new possibilities for using olives as biological carriers of probiotic microorganisms (Sisto and Lavermicocca 2012, Blana et al. 2014). To the best knowledge, of the authors no previous studies have been made of the influence of lactic acid bacteria on iridoids (loganic acid and cornuside).
The aim of this work is to evaluate changes to polyphenols and iridoids caused by selected lactic acid strains in order to determine their potential for the development of new starter cultures for a functional food, green fermented cornelian cherry. The effect of the strains on the polyphenols, iridoids, residual sugars and organic acids was determined in the fermented medium.
Materials and methods
Chemicals
The study was conducted using 2,2′-azino-bis(3-ethyl-benzothiazoline-6-sulphonic acid) diammonium salt (ABTS), 2,2-diphenyl-1-picrylhydrazyl (DPPH), 6-hydroxy-2,5,7,8-tetramethylchroman-2-carboxylic acid (Trolox); 2,4,6-tri(2-pyridyl)-s65 triazine (TPTZ), dimethyl sulfoxide (DMSO) and Folin-Ciocalteu reagent (F–C) purchased from Sigma-Aldrich (Poznan, Poland), as well as acetonitrile, formic acid and methanol supplied by Sigma–Aldrich (Steinheim, Germany). Acetonitrile for LC–MS was purchased from POCh (Gliwice, Poland). Loganic acid, cornuside, ellagic acid, protocatechuic acid and chlorogenic acids were purchased from Extrasynthese (Lyon Nord, France). Gallic, tartaric, malic, quinic, citric, shikimic, lactic and acetic acids were procured from Sigma-Aldrich (St. Louis, MO, USA).
Plant material
Unripe Cornus mas L. fruits were collected in the last week of July from bushes and trees growing in Zadzim (central Poland, 18º50′E, 51º46′N) and transported to the laboratory. The fruits were washed, selected and divided into samples weighing approximately 0.5 kg.
Bacterial strains
The research material comprised five strains of Lactobacillus sp. Four of the investigated strains were isolated from the feces of healthy infants at the Institute of Fermentation Technology and Microbiology, Lodz, Poland and one (Lb. brevis 18 M—Lb18 M) was obtained from vegetables at the University of Warmia and Mazury in Olsztyn, Poland.
Lactobacillus casei ŁOCK 0919 (Lc919), Lactobacillus rhamnosus ŁOCK 0908 (Lr908) and Lactobacillus rhamnosus ŁOCK 0900 (Lr900) possess full probiotic documentation (approved as being probiotic under WHO criteria) and are licensed (P-382760, P-382761, P-382762) (Aleksandrzak-Piekarczyk et al. 2013; Koryszewska-Baginska et al. 2013, 2014). They are deposited at the Ludwik Hirszfeld Institute of Immunology and Experimental Therapy, Polish Academy of Sciences, Wroclaw, Poland, under the following accession numbers: B/00019, B/00020, B/00021. The strain Lb. paracasei 920 (Lp920) is covered by patent application P-396640 (Bulletin of the Patent Office, Strains of lactic acid bacteria Lactobacillus paracasei). Strains obtained in the form of lyophilizates were passaged twice in MRS (de Man, Rogosa, Sharpe) broth.
Fermentation conditions
The fruits were blanched with the addition of savory at 85 °C for five minutes in 5% NaCl solution and then fermented without savory (ws) but with the addition of pure cultures of lactic acid bacteria (Lb18 M, Lc919, Lp920, Lr900, Lr908) or microbiota isolated from commercially-available fermented black olives (mBO). Samples subjected to spontaneous fermentation (wos) were used for comparison. The final concentration of the inoculum in brine was around 104 CFU/mL. The fermentations were carried out in triplicate at room temperature, over six weeks.
Microbiological analysis
Samples of the products were prepared according to ISO 6887. Total mesophilic count (TMC) was determined on plate count agar (PCA, Merck) following incubation at 30 °C for 96 h. Lactic acid bacteria (LAB) were quantified on MRS agar (Merck) following incubation at 30 °C for 72 h under anaerobic conditions (Gas-Pack System, BBL, Becton–Dickinson, Franklin Lakes, NJ USA). DRBC agar (Merck) was used for the yeast and mould count. The lowest detection limit of these enumeration techniques is 10 CFU/g. Three samples were analysed of each product.
Analysis of sugars and organic acids
Glucose, fructose and organic acids were analysed using a Finnigan Surveyor HPLC system (Thermo Fisher Scientific Inc, Waltham, MA, USA) equipped with an autosampler, a refractive index detector (Finnigan Surveyor-RI Plus), a diode array detector (Finnigan Surveyor-PDA Plus) and ChromQuest 5.0 chromatography software (Thermo Fisher Scientific Inc, Waltham, MA, USA). Separation was performed on an Aminex HPX87H column (300 × 7.8 mm, Bio-Rad, Hercules, CA, USA) protected by a guard column. The elution conditions were as follows: 0.6 mL/min flow rate; oven temperature 60 °C; solvent 5 mmol sulphuric acid following absorbance at 210 nm and RI detection.
Extraction of iridoids and phenolic acids for HPLC analysis
After fermentation, cornelian cherry (C. mas L.) fruits were pitted and homogenized. Ten millilitres of 80% aqueous methanol, acidified with 1% of HCl were added to 5 g of homogenate. The samples were sonicated (Sonic 6D, Polsonic, Warsaw, Poland) twice for 10 min. The extracts were then filtered under a vacuum using a Schott funnel. The precipitates were collected into 50 mL flasks. The extract was centrifuged at 19,000 g for 15 min at 4 °C and filtered using a 0.45 mm filter before HPLC analysis. Three parallel extracts were prepared from each cultivar.
Quantification of iridoids and phenolic acids by HPLC
Analysis of iridoids and phenolic acids was performed according to the method described by Kucharska et al. (2015). HPLC analysis was performed using a Dionex (Sunnyvale, CA, USA) system equipped with an Ultimate 3000 diode array detector model, an LPG-3400A quaternary pump, an EWPS-3000SI autosampler and a TCC-3000SD thermostated column compartment, controlled by Chromeleon v 6.8 software. An Atlantis T3 (250 mm × 4.6 mm i.d., 5 µm) column (Waters, Ireland) and an Atlantis T3 (20 mm x 4.6 mm i.d, 5 µm) guard column (Waters, Ireland) were used. The mobile phase was composed of solvent A (4.5% formic acid, v/v) and solvent B (acetonitrile). The elution system was as follows: 0–1 min 5% B, 1–37 min 5–25% B, 37–42 min 25–100% B, 42–47 min 100% B, 47–50 min, 100–5% B, 50–55 min 5% B. The flow rate of the mobile phase was 1.0 mL/min and the injection volume was 20 µL. The column was operated at 30 °C. Iridoids were detected at 245 nm, ellagic and protocatechuic acids at 254 nm, gallic acid and its derivative at 280 nm and chlorogenic acids and their derivatives at 320 nm. Calibration curves were prepared for loganic acid, gallic acid and its derivative as gallic acid, and for chlorogenic acids and their derivatives as chlorogenic acid. The results were expressed as mg/100 g fresh weight (fw). The calibration curves were obtained using the external standard method with six levels of concentration of standard mixtures and three injections per level. Chromatogram peak areas for 245 nm (loganic acid) and 320 nm (chlorogenic acid) were plotted against the known concentrations (0.02–0.3 mg/mL for loganic acid (Extrasynthese, 99% purity) of the standard solutions to establish the calibration equations. Linear regression equations were calculated using the least-squares method.
Analysis of antioxidant activity
The content of substances which reacted with Folin–Ciocalteu (FC) reagent (SRFC) was determined using the Folin–Ciocalteu method, as described in Sokół-Łętowska et al. (2014).
An ABTS assay was performed according to the method developed by Re et al. (1999). The DPPH radical scavenging activity of the samples was determined according to the method set out by Yen and Chen (1995). Antioxidant activity was measured using FRAP according to the method described by Benzie and Strain (1996). All analyses were performed in triplicate. Measurements were made using a Shimadzu UV-2401 UV–Vis spectrophotometer (Kyoto, Japan). Standard curves were prepared using different concentrations of Trolox. The results were expressed as Trolox-equivalent (TE) values (µmol TE/1 ml brine), defined as the amount of Trolox (in µmol) that exhibited the same antioxidant activity as 1 ml of brine.
Statistical analysis
All measurements were performed in triplicate and the results presented as mean values ± standard deviations (SD). Using STATISTICA 10 PL software (StatSoft, Krakow, Poland), the standard deviation was determined and an ANOVA test conducted, assuming a significance level of 0.05.
Results
Microbiology
The total number of mesophilic bacteria in the tested samples reached 104–105 CFU/ml (Table 1). The numbers of mesophilic bacteria were statistically higher in brine fermented with the strains Lc919, Lb18M, Lr900 and Lr908 as compared to the controls. There were no statistically significant differences between the number of mesophilic bacteria and the number of LAB, except in the case of product obtained with Lb. rhamnosus 900. In that case, the number of LAB was slightly higher, which may indicate better recovery of this strain in the MRS broth. The results indicate that lactic acid bacteria predominated among the bacterial microbiota in the product. The largest numbers of LAB, at 106, were observed in the samples fermented with Lb. rhamnosus 900 and 908. The amount of yeast was the lowest in brine fermented with mixed microbiota isolated from black olives. The largest amount of yeast was found in samples Lr908, Lb18M and Lr900.
Fermentation
Table 2 shows the concentration of organic acids and sugar consumption in the fermented unripe cornelian cherry brine.
After fermentation, most of the samples differed in terms of their concentrations of glucose and fructose. These ranged from 0.10 for the ws sample to 0.65 g/100 mL for spontaneous fermentation (wos) for glucose content and from 1.03 to 1.56 g/100 mL for fructose content. The lower glucose content indicates that this sugar was utilized by the bacteria.
On the basis of chromatographic assays, the presence of six acids was revealed in the tested samples. Four were compounds present in the raw material (malic, tartaric, quinic and shikimic acids). The other two were products of lactic acid fermentation (lactic and acetic acids). Of the acids, malic acid showed the highest concentrations in the tested samples. The malic acid content reached 833–1224 mg/100 mL and in most cases did not differ significantly from the control sample (ws). Shikimic, quinic and tartatic acids occurred at levels of 30–86 mg/100 mL, tr-50 mg/100 mL and 6–11 mg/100 mL, respectively.
Lactic acid was found to be the major metabolite in all the samples, with implications both for the preservative qualities of the fermented products and their sensorial characteristics.
The lowest amounts of lactic acid were observed in samples fermented using the spontaneous process (wos) and microbiota from black olives. The highest content of lactic acid was found in the control sample (ws), at 174 mg/100 mL. The quantity of lactic acid in the other samples ranged from 93 to 113 mg/100 mL, with the highest levels in the samples inoculated with Lb. rhamnosus 900 and 908. Smaller amounts of acetic acid were also observed in all the investigated samples. The amounts of this acid varied quite considerably, ranging from 3.7 to 16.7 calculated as milligrams of acetic acid per 100 mL of brine. As with lactic acid, the highest content of acetic acid was found in the control sample (ws). The correlation between glucose and lactic acid contents was calculated as −0.91 using a Pearson correlation coefficient (r).
Polyphenols in fermented fruits and brines
Included among the compounds detected in the fruits and brine were hydroxybenzoic acids: gallic acid and one of its derivatives, protocatechuic acid and ellagic acid. The following hydroxycinnamic acids were also present: neochlorogenic acid, chlorogenic acid and a chlorogenic acid derivative (Table 3). Gallic acid and its derivative were the main products in all of the tested samples. The concentration of gallic acid in the fruits ranged from 3.01 (for CFLc919) to 11.45 mg/100 g (CFBO). That of its derivative ranged from 2.21 (CFLc919) to 9.58 mg/100 g (CFws). In almost all cases (except for samples fermented with the addition of Lp920 and mBO), the gallic acid content was lower than that in the control sample (CFws). The content of ellagic acid ranged from 0.47 (CFLc919) to 2.55 mg/100 g (CFLp 920). Of the hydroxycinnamic acid derivatives, the predominant compound was chlorogenic acid. Its content ranged from 1.42 (CFLc919) to 5.97 (CFLr908) mg/100 g.
As with gallic acid, the amount of chlorogenic acid was lower relative to the control sample (CFws), except in the cases of CFLr908 and CFBO. Neochlorogenic acid and the chlorogenic acid derivative were present in lower amounts: from 0.54 (CFLc919) to 1.85 (CFLr908) and from 0.52 (CFLc919) to 1.91 mg/100 g (CFmBO), respectively. The total amount of polyphenols in fruits determined using HPLC ranged from 8.56 to 30.51 mg/100 g. The lowest levels of these compounds (approx. 3 times lower than in the CFws sample) were clearly observed in the samples fermented with Lc919, whereas the highest levels were found in samples fermented with microbiota from black olives. The total concentration of polyphenolic compounds in brine analysed using HPLC was less varied and ranged from 18.45 (CBLr900) to 25.36 mg/100 g (CBmBO). The polyphenol content was lower in all of the samples fermented with probiotic bacteria as compared to the control sample (CBws). Gallic acid, its derivative and chlorogenic acid predominated in terms of the content of individual compounds in the brine.
Based on these analyses, it may be concluded that a significant part of the phenolic acids were transferred to brine, although the total phenols content was from 2 to 3 times higher in the fruits than in the brine.
Iridoids in fruits and brine
Iridoids were another group of compounds examined in the research. Loganic acid and cornuside were each found to be present in both the fruits and in the brine (Table 4). The loganic acid content was higher and ranged from 15.52 (CFLc919) to 130.23 mg/100 g (CFLr 908) in the fruits and from 17.39 (CBLc919) to 115.61 mg/100 g (CBws) in the brine. Cornuside occurred in the fruits in quantities from 2.15 to 15.69 mg/100 g, but was not found in two samples of brine (CBLr900, CBLp920). This compound reached 2.69 mg/100 g in the remaining sample (CBLr908). As in the case of polyphenols, the content of iridoids in the fruits was lowest for samples fermented with Lc919 and highest for samples fermented with Lr908.
The content of loganic acid in the fruits was comparable to that in the brine. The cornuside content was much lower than in the fruits, and its presence was observed in only three cases.
Antioxidant capacity of unripe fermented fruits and brines
Four different assays (reaction with FC reagent, the ABTS radical cation assay, the DPPH radical assay and ferric reducing antioxidant power FRAP) were used to evaluate the antioxidant properties of the fruits and brines (Table 5).
The largest amounts of substances which reacted with FC were observed in fruit samples fermented with the Lr908 strain. The concentration of these compounds in CFmBO was significantly lower than in the control sample (CFws).
The ABTS•+ values were significantly higher, statistically, in fruits fermented with microbiota from black olives as compared to the controls. Large amounts of this radical were also observed in samples fermented with Lb. rhamnosus 908, but these were not statistically different from the control.
The highest values from the DPPH and FRAP assays were measured in fruit samples fermented with the addition of Lr908. The highest values for SRFC, DPPH, ABTS and FRAP assays were observed in the control brine samples and the brine samples with the addition of microbiota from black olives. The lowest were measured in the brine samples inoculated with bacteria Lr900 and Lc919. In the brine samples fermented with probiotic bacteria, the highest antioxidant activity was observed in the samples inoculated with Lr908.
The correlation between the bioactive compound contents and the antioxidant properties of the samples was determined using a Pearson correlation coefficient (r) (Table 6). Higher correlations were observed between antioxidant activities and the amount of iridoids as compared to polyphenols in the fruits, as characterized by HPLC.
Discussion
This study has cleared demonstrated that lactic acid bacteria of different origins can have an effect on polyphenols, iridoids, residual sugars and organic acids in fermented green cornelian cherries. Moreover, it has opened the prospect of a new probiotic functional product with high iridoid compound content.
The microorganisms were present in the samples at levels ranging from 105 to 106 CFU/mL. The fruits selected for fermentation had less sugar and more polyphenols compared to ripe fruits (Kucharska et al. 2011).
Fermented unripe cornelian cherries were described as “false olives” in old Polish literature (Kucharska et al. 2011), so it is worth comparing their fermentation to that of olives. Landete et al. (2008) studied the degradation of phenolic compounds by lactic acid bacteria in olives. Protocatechuic acid found in fermented olives was metabolized by Lb. plantarum strains.
Arbequina table olives are processed as “naturally green olives” and harvested just before “turning colour”, so they provide a good point of comparison to the cherries used in this study.
In an investigation into the influence of fruit ripeness on the microbial processing of Arbequina table olives, Hurtado et al. (2009) report that the growth of lactic acid bacteria, as opposed to Enterobacteriaceae, was delayed in green olives compared to black and “turning colour” olives. The low survival rates of the bacteria in the present study may be due partially to the environment, in which there are phenolic acids and short-chain organic acids such as malic, tartaric or shikimic acids. LAB can be partially inhibited by the presence of phenolic compounds during the preparation of olives which are not flooded with NaOH (lye) (Ruiz-Barba et al. 1993). The role of yeasts then becomes more important (Arroyo-López et al. 2012). Yeasts can synthesize vitamins, amino acids and other compounds vital for the growth of Lactobacillus, which requires a more demanding medium for growth (Arroyo-López et al. 2012). Yeasts can affect the flavour of table olives and are considered spoilage microorganisms during olive storage and packaging (Arroyo-López et al. 2008).
Lactic acid was found to be the main biochemical product of fermentation in all samples. Both the organic acids and sugars were measured in the brine. However, the results of Blana et al. (2014) suggested that changes in the organic acids and sugars in the brine reflected changes in the fruits. The authors observed a gradual loss of lactic acid in samples subjected to spontaneous fermentation with 8% brine, until its complete disappearance. In the present study, the lowest lactic acid content was also observed in samples in which spontaneous fermentation had occurred. In the samples, the number of LAB was also relatively low, and there were predominantly yeasts which could utilize the acid produced as a readily-available source of carbon.
Some authors have reported the presence of hydrolysable tannins, derivatives of gallic and ellagic acids in raw materials from the Cornaceae family (Hatano et al. 1990; Kucharska 2012). Kucharska (2012) observed the presence of phenolic acids from the group of benzoic and hydroxycinnamic acid derivatives in ripe C. mas fruits. The author identified gallic acid and its dimer ellagic acid from the group of hydroxybenzoic acids and their derivatives, and chlorogenic acid from the group of hydroxycinnamic acid derivatives, among others. The gallic acid content in the fruits ranged from 0.50 mg/100 g to 17.96 mg/100 g and was 7.96 mg/100 g on average, whereas the ellagic acid content ranged from 0.48 to 1.42 mg/100 g. These quantities are comparable with those obtained in the present study. The tested acids form high molecular tannins, which are decomposed by hydrolysis into smaller particles, which in turn may be degraded by various factors, such as enzymes in the microorganisms.
Gallic acid is biologically active and exhibits antibacterial, antifungal and anti-inflammatory activities. Therefore, its presence is positive from a health standpoint.
The products obtained through lactic acid fermentation with probiotic bacteria also contained a gallic acid derivative. A compound with the same retention time and a similar spectrum was identified in cornelian cherry tinctures by Kucharska (2012).
The chlorogenic acid content in the ripe fruits of different varieties of C. mas ranged from 0.68 mg/100 g to 3.62 mg/100 g. The fruits examined by Kucharska contained a high concentration of p-coumaroylhexoside, which was not detected in this study. The higher content of chlorogenic acid and the presence of neochlorogenic acid and its derivative could be due to the degree of fruit ripeness and changes that may occur in this group of compounds as a result of lactic acid fermentation.
The metabolism of polyphenolic compounds by lactobacilli is still a subject of research. Most studies have focused on Lactobacillus plantarum. There are very few publications on Lb. brevis (Curiel et al. 2010; Landete et al. 2010) and even fewer on Lb. casei (Rivas-Sendra et al. 2011) or Lb. rhamnosus (Marazza et al. 2009). In a review of the literature, no publications were found on Lb. paracasei.
A study by Curiel et al. (2010) on several strains of Lb. brevis isolated from different environments demonstrated the possibility of degrading phenolic acids in foods. The metabolism of phenolic acids was similar for all the tested strains. Of the tested cinnamic acids, only p-coumaric, ferulic and caffeic acids were metabolized by Lb. brevis strains. Decarboxylation yielded the corresponding vinyl derivatives. As in the case of Lb. plantarum, Lb. brevis modified, of the benzoic acids, only gallic acid and protocatechuic acid, to catechol and pyrogallol respectively.
According to Landete et al. (2010), phenolic acid decarboxylase (PAD) is the enzyme involved in the metabolism of phenolic acids by Lb. brevis. An analysis of the available genomic sequences of Lb. casei did not show the presence of this gene (Rivas-Sendra et al. 2011).
Heterofermentative lactic acid bacteria such as Lb. curvatus PE5, Weisella spp., Lb. brevis, Lb. fermentum and Leuconostoc mesenteroides were examined by Filannino et al. (2014). These authors reduced caffeic, p-coumaric and ferulic acids to dihydrocaffeic, phloretic and dihydroferulic acids or to the corresponding vinyl and then ethyl derivatives.
Most phenolic compounds can exert an inhibitory effect on LAB growth. This inhibition activity seems to be related to alterations to cytoplasmic membranes and in the cell wall (Campos et al. 2009).
Gallic acid and catechin have been reported to have an inhibitory effect on Lb. plantarum in wine (Landete et al. 2007). On the other hand, some studies suggest that gallic acid can stimulate the growth and malolactic activity of certain strains of lactic acid bacteria (Alberto et al. 2001).
Hydroxycinnamic acids (particularly p-coumaric acid) are known to inhibit the growth of a variety of microorganisms, including wine-spoilage strains of Lb. collinoides and Lb. brevis (Stead 1994). Phenolic compounds have been found to cause significant inhibition of growth in Lb. casei BL23, with p-coumaric acid the most active compound in this respect (Rivas-Sendra et al. 2011).
Four iridoids have been identified in the fruits of the European species of cornelian cherry (C. mas L.), including loganic acid and cornuside, as well as sweroside and loganin, present in trace amounts (Kucharska 2012; Kucharska et al. 2015; Deng et al. 2013). Loganic acid and its methyl derivative loganin are classified as glycosidic iridoids. Cornuside, a compound which influences the bitterness of some fruits, is a secoiridoid glycoside and a combination of secologanin and gallic acid (Dewick 2009).
Kucharska (2012) noted the presence of loganic acid in Polish varieties of cornelian cherry. Loganic acid is a dominant iridoid, which amounts to between 88 and 96% of the total iridoids in ripe cornelian cherry fruits, depending on the variety (Kucharska 2012; Kucharska et al. 2015). Kucharska (2012) was also the first to identify the presence of cornuside in cornelian cherry fruits. The concentration of cornuside was approximately ten times lower than that of loganic acid. The ratios between these compounds were similar in our products for the control samples, Lb. brevis 18 M and for the sample with the addition of microbiota from black olives. The antioxidant activity of cornelian cherry products was investigated using DPPH, FRAP and ABTS methods. Many reports have indicated a close linear correlation between the content of total polyphenols determined by reaction with FC and antioxidant activity determined using ABTS or FRAP. These results are the effect of the similarity of these methods, based on the reaction of oxidation and reduction, rather than reliable comparative measures of the amount of polyphenols and antioxidant activity.
Conclusion
The use of lactic acid bacteria which are able to survive while fermenting unripe cornelian cherry fruits may expand the list of food products involving probiotics and improve the nutritional and dietary value of fermented foods. Such fermented products may be an alternative way to complement gut microbiota, especially for those who do not tolerate lactose and milk proteins, and in whom the presence and development of probiotic bacteria in the gastrointestinal tract may have been disturbed. This study has shown that the choice of strain significantly affects the amount of lactic acid produced and the quality of the product. The results indicate that the human strain Lb. rhamnosus 908 could be used as both a starter and a probiotic culture, allowing the fermentation process to be controlled and yielding a final functional product with probiotic properties. Further research should be focus on the metabolism of loganic acid and other compounds by Lb. rhamnosus bacteria.
References
Abriouel H, Benomar N, Cobo A, Caballero N, Fernández Fuentes MA, Pérez-Pulido R, Gálvez A (2012) Characterization of lactic acid bacteria from naturally fermented Manzanilla Aloreña green table olives. Food Microbiol 32:308–316
Alberto MR, Farías ME, Manca de Nadra MC (2001) Effect of gallic acid and catechin on Lactobacillus hilgardii 5w growth and metabolism. J Agric Food Chem 49:4359–4363
Aleksandrzak-Piekarczyk T, Koryszewska-Baginska A, Bardowski J (2013) Genome sequence of the probiotic strain Lactobacillus rhamnosus (Formerly Lactobacillus casei) LOCK900. Genome Announc 1(4):5
Argyri A, Zoumpopoulou G, Karatzas KA, Tsakalidou E, Nychas G-JE, Panagou EZ, Tassou CC (2013) Selection of potential probiotic lactic acid bacteria from fermented olives by in vitro tests. Food Microbiol 33:282–291
Arroyo-López FN, Querol A, Bautista-Gallego J, Garrido-Fernández A (2008) Role of yeasts in table olive production. Int J Food Microbiol 128:189–196
Arroyo-López FN, Romero-Gil V, Bautista-Gallego J, Rodríguez-Gómez F, Jiménez-Díaz R, García-García P, Querol A, Garrido-Fernández A (2012) Yeasts in table olive processing: Desirable or spoilage microorganisms? Int J Food Microbiol 160:42–49
Bautista-Gallego J, Arroyo-López FN, Rantsiou K, Jiménez-Díaz R, Garrido-Fernández A, Cocolin L (2013) Screening of lactic acid bacteria isolated from fermented table olives with probiotic potential. Food Res Int 50:135–142
Benzie IEF, Strain JJ (1996) The ferric reducing ability of plasma (FRAP) as a measure of “antioxidant power”. The FRAP assay. Anal Biochem 239:70–76
Blana VA, Grounta A, Tassou CC, Nychas G-JE, Panagou EZ (2014) Inoculated fermentation of green olives with potential probiotic Lactobacillus pentosus and Lactobacillus plantarum starter cultures isolated from industrially fermented olives. Food Microbiol 38:208–218
Campos FM, Couto JA, Figuereido AR, Toth IV, Rangel AOSS, Hogg TA (2009) Cell membrane damage induced by phenolic acids on wine lactic acid bacteria. Int J Food Microbiol 135:144–151
Curiel JA, Rodriguez H, Landete JM, de las Rives B, Munoz R (2010) Ability of Lactobacillus brevis strains to degrade food phenolic acids. Food Chem 120:225–229
Deng S, West BJ, Jarakae Jensen C (2013) UPLC-TOF-MS characterization and identification of bioactive iridoids in Cornus mas fruit. J Anal Methods Chem. doi:10.1155/2013/710972
Dewick PM (2009) Medicinal natural products. A biosynthetic approach. Wiley, New York
Filannino P, Gobbetti M, de Angelis M, Di Cagno R (2014) Hydroxycinnamic acids used as external acceptors of electrons: an energetic advantage for strictly hetero-fermentative lactic acid bacteria. Appl Environ Microbiol 80:7574–7582
Hatano T, Yasuhara T, Abe R, Okuda T (1990) A galloylated monoterpene glucoside and a dimeric hydrolysable tannin from Cornus officinalis. Phytochemistry 29:2975–2978
Hurtado A, Reguant C, Bordons A, Rozes N (2009) Influence of fruit ripeness and salt concentration on the microbial processing of Arbequina table olives. Food Microbiol 26:827–833
Koryszewska-Baginska A, Aleksandrzak-Piekarczyk T, Bardowski J (2013) Complete genome sequence of the probiotic strain Lactobacillus casei (Formerly Lactobacillus paracasei) LOCK919. Genome Announc 1(5):26
Koryszewska-Baginska A, Bardowski J, Aleksandrzak-Piekarczyk T (2014) Genome Sequence of the Probiotic Strain Lactobacillus rhamnosus (Formerly Lactobacillus casei) LOCK908. Genome Announc 2(1):20
Kucharska AZ (2012) Active compounds of cornelian cherry fruit (Cornus mas L.). Wydawnictwo Uniwersytetu Przyrodniczego, Wrocław
Kucharska AZ, Piórecki N, Sokół-Łętowska A, Żarowska B (2011) Characteristics of chemical composition and antioxidant properties of cornelian cherry fruit fermented in brine. Zeszyty Problemowe Postępów Nauk Rolniczych 566:125–133
Kucharska AZ, Szumny A, Sokół-Łętowska A, Piórecki N, Klymenko SV (2015) Iridoids and anthocyanins in cornelian cherry (Cornus mas L.) cultivars. J Food Compos Anal 40:95–102
Landete JM, Rodrıguez H, de las Rives B, Munoz R (2007) High added-value antioxidants obtained from the degradation of wine phenolics by Lactobacillus plantarum. J Food Prot 70:2670–2675
Landete JM, Curiel JA, Rodriguez H, de las Rives B, Munoz R (2008) Study of the inhibitory activity of phenolic compounds found in olive products and their degradation by Lactobacillus plantarum strains. Food Chem 107:320–326
Landete JM, Rodrıguez H, Curiel JA, de las Rives B, Munoz R (2010) Gene cloning, expression, and characterization of phenolic acid decarboxylase from Lactobacillus brevis RM84. J Ind Microbiol Biotechnol 37:617–624
Marazza JA, Garro MS, de Giori GS (2009) Aglycone production by Lactobacillus rhamnosus CRL981 during soymilk fermentation. Food Microbiol 26:333–339
Re R, Pellegrini N, Poteggente A, Pannala A, Yang M, Rice-Evans C (1999) Antioxidant activity applying an improved ABTS radical cation decolorization assay. Free Radic Biol Med 26:1321–1327
Rivas-Sendra A, Landete JM, Alcantara C, Zuniga M (2011) Response of Lactobacillus casei BL23 to phenolic compounds. J Appl Microbiol 111:1473–1481
Ruiz-Barba JL, Brenes-Balbuena M, Jiménez-Díaz R, García-García P, Garrido Fernández A (1993) Inhibition of Lactobacillus plantarum by polyphenols extracted from two different kind of olive brine. J Appl Bacteriol 74:15–19
Settanni L, Moschetti G (2014) New trends in technology and identity of traditional dairy and fermented meat production processes. Trends Food Sci Technol 37:51–58
Sisto A, Lavermicocca P (2012) Suitability of a probiotic Lactobacillus paracasei strain as a starter culture in olive fermentation and development of the innovative patented product “probiotic table olives”. Front Microbiol. doi:10.3389/fmicb.2012.00174
Sokół-Łętowska A, Kucharska AZ, Wińska K, Szumny A, Nawirska-Olszańska A, Mizgier P, Wyspiańska D (2014) Composition and antioxidant activity of red fruit liqueurs. Food Chem 157:533–539
Stead D (1994) The effect of chlorogenic, gallic and quinic acids on the growth of spoilage strains of Lactobacillus collinoides and Lactobacillus brevis. Lett Appl Microbiol 18:112–114
Yen GC, Chen HY (1995) Antioxidant activity of various tea extracts in relation to their antimutagenicity. J Agric Food Chem 43:27–32
Author information
Authors and Affiliations
Corresponding author
Rights and permissions
About this article
Cite this article
Czyżowska, A., Kucharska, A.Z., Nowak, A. et al. Suitability of the probiotic lactic acid bacteria strains as the starter cultures in unripe cornelian cherry (Cornus mas L.) fermentation. J Food Sci Technol 54, 2936–2946 (2017). https://doi.org/10.1007/s13197-017-2732-3
Revised:
Accepted:
Published:
Issue Date:
DOI: https://doi.org/10.1007/s13197-017-2732-3