Abstract
Economic growth and rising food consumption in the world have led to increased production of organic wastes due to an important intensification of the agricultural production systems. The analysis of macro elements (nitrogen [N], phosphorus [P], and potassium [K]) in organic wastes reveals that the economic loss is significant with the intensification of the agricultural system. The good management of those wastes would be an effective means of organic matter restoration through carbon restitution to the depleted soils through organic amendments. Composting is a type of waste processing that has gained increasing acceptance over the years. As a rule, the process consists of the natural biological decomposition of organic waste components and involves diverse species of microorganisms. Those organic residues could reconstitute soils and be an important fertilization backup. Composts prepared from different organic wastes differ in their quality and stability, which further depends upon the composition of raw material used for the compost production. Compost quality is closely related to its stability and maturity. The wide variety of chemical and biological variations that occur during composting, and the range of methods suggested in literature, has made it difficult to agree on methods for the practical assessment of maturity. A literature review of the main start-up, monitoring and maturity parameters are discussed concerning different raw materials used and different composting methods practiced.
Similar content being viewed by others
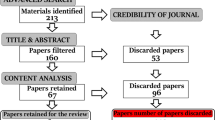
Explore related subjects
Discover the latest articles, news and stories from top researchers in related subjects.Avoid common mistakes on your manuscript.
Introduction
Composting is a biological process (hygienic transformation of organic wastes in a homogeneous and plant available material), that occurs under aerobic conditions (presence of oxygen), with adequate moisture and temperature. Composting can be interpreted as the sum of complex metabolic processes performed by different microorganisms that, in the presence of oxygen, use nitrogen (N) and carbon (C) available to produce their own biomass. In this process, additionally, the microorganisms generate heat and a solid substrate, with less carbon and nitrogen, but more stable, which is called compost (Roman et al. 2015). The composting process is similar to the natural processes of mineralization and humification occurring after incorporating organic residues into cultivated soils. Antizar-Ladislao et al. (2006) specify that composting accelerates the biological aerobic transformation of organic matter involving the formation of humic substances and generating a stable product called compost. Composting is the direct result of the action of a diversity of microbial populations under aerobic conditions (Sharma et al. 1997). Different communities of microorganisms mainly, bacteria, fungi, and protozoa are actively and successively implied in composting process with variable intensity depending on the temperature, moisture content, C/N ratio and nature of fresh organic materials (Mustin 1987; Tuomela 2000; Hassen et al. 2001).
There are two main phases in composting process. The first is characterized by microbial activity leading to the decomposition of most of the biodegradable material and the stability of the organic residue. The second is characterized by converting a portion of the organic material remaining in humic substances (Adani et al. 1999). The humification is indicated as the main factor in improving the quality of compost because of the importance of the humic substances in the ecology, soil fertility, and soil structure (Chen and Aviad 1990; Chen et al. 1994). Kaiser (1983) described the two phases as follows:
-
Decomposition phase which involves all the three stages (mesophile, thermophile and cooling) in which breaking both simple and complex organic matter occurs;
-
Humification phase which corresponds to the maturation phase characterized by the reorganization of the organic matter in stable molecules.
For the mesophile stage, the pile temperature slowly increases from ambient temperature to reach the average temperature of this stage, which is about 40 °C. The pH decreases due to the organic acids released from the carbohydrates and lipids degraded by microorganisms (Kaiser 1983; Mustin 1987; Tuomela 2000). Starting from 40 °C, mesophile microorganisms are gradually replaced by thermophile microorganisms (bacteria, fungi and actinomycetes). The thermophile stage is characterized by a temperature usually between 50 and 60 °C. This stage involves many thermo-tolerant and thermophilic fungi, pH rises because the microorganisms degrade proteins and release ammonia. Above 60 °C, the degradation of organic matter slow down, and after 70 °C, only the enzymes released in the previous stage, still contribute to the degradation. During cooling stage, the material tends to stability, and the mesophile organisms are re-established.
The maturation is carried out at ambient temperature, under the mesophilic microorganisms (bacteria and fungi) (Makan et al. 2013). Micro and macro fauna appear in this phase. Antagonism and predation relationships develop between organisms. Antibiotics are synthesized in appreciable quantities. Finally, the release of heat and the weight loss remains low (Vobrkov et al. 2016). El Fels et al. (2014) stated that during the maturation phase, there are secondary reactions of polymerization and condensation, which lead to the formation of humus with humic acids, are particularly resistant to degradation. The composted material matures when:
-
Do not heat up more after turning,
-
Do not become anaerobic during storage,
-
Do not take nitrogen to the soil after its amendment.
In this phase, a part of the nitrogen from protein becomes resistant to microbial degradation by its incorporation into humic acids (Roman et al. 2015).
There are various composting parameters in the literature such as: carbon-nitrogen ratio (C/N), microbial activity, germination index, cation exchange capacity (CEC), humic substances content, compost concentration of water soluble carbon (WSC), dissolved organic matter, ratios of NH4 +—N and NO3 −—N, WSC/TN, and WSC/organic-N (Azim et al. 2017). Most of them are well studied, but there are new monitoring parameters that have been recently developed in order to describe better the exact level of biodegradation. No single parameter is widely accepted, and thus a combination of tests is likely to be needed.
This review discusses the main start up parameters, monitoring parameters, and quality test of compost. A suggested index of maturity with regard to spectral analysis of compost are also discussed.
Composting parameters
The growing number of papers discussing more issues on composting parameters proves that there is obviously a need for more information on the various composting parameters, which may not be abundant but are very important to the biochemical processes and for assessing the compost quality. Most of the studied parameters, concern compost monitoring, and compost quality at the end of the process. Fewer studies discuss start-up parameters (C/N ratio, moisture content and particle size of fresh materials). Composting parameters can consequently, be split into three categories as shown in Fig. 1.
Start-up parameters
Effective composting can be achieved by providing optimal start up conditions for decomposers. These include proper nutrient balance, moisture content, and aeration. Among these, the nutrient balance is expressed as carbon to nitrogen ratio (C/N ratio). A certain amount of moisture is also essential for composting, since the main site of microbial activity is in the thin water-film on the surface of particles (Choi 1999).
C/N ratio
The microorganisms, such as bacteria or fungi, are known to utilize about 30 parts of cellulose for each part of nitrogen in the breakdown process (Choi 1999). Proportions of C and N in composting materials have particular importance. Carbon serving both, as a source of energy and elemental component for microorganisms and nitrogen is essential for the synthesis of amino acids, proteins and nucleic acids. During the active phases of aerobic fermentation, the microorganisms consume 15 to 30 times more carbon than nitrogen (Mustin 1987). In Fig. 2, Mustin (1987) has verified that the time of composting become longer as far as the initial C/N ratio is higher. Due to the recalcitrant carbon, which is difficult to degrade, compost of shrub and wood (also in Fig. 2) takes longer time to be mature (18 months) than a compost of household waste (with initial C/N of 30) that matures in 7 months.
Evolution of C/N ratios of several organic wastes during composting (Mustin 1987)
The carbon to nitrogen (C/N) ratio is one of the important factors affecting the composting process as well as the properties of the end-product (Kumar et al. 2010). In general, the optimal C/N ratios in composting of most materials have been reported to vary from 25 to 30 (Choi 1999). In the literature, the ideal C/N ratio is around 30 to ensure the carbon energy intake while enabling rapid growth of microorganisms (Golouke 1991). During composting experiments on municipal green waste (mixed or not with treated sludge), it was revealed that the optimal value of C/N ratio was 25 in the starting material; higher values have slowed the rate of decomposition while lower values increased N losses (De Bertoldi et al. 1983). If the value of the initial C/N ratio is greater than 35, the microorganisms must pass through many life cycles to oxidize excess of carbon. In this case, the aerobic fermentation rate is controlled by the availability of nitrogen. However, recent studies have shown that composting can be carried out effectively at a lower C/N of 15. Composting at low initial C/N ratios will reduce the requirement of bulking agent for adjusting the initial C/N ratio of a food waste composting mixture (Kumar et al. 2010).
When the initial C/N ratio is high during aerobic fermentation phase, organic substrates quickly lose their carbon (metabolized and released as carbon dioxide) than their nitrogen (metabolized and lost as a small part of volatile nitrogen). Therefore, the C/N ratio decreases slowly during composting of high recalcitrant materiel. For example, Fuangworawong (2008) found that several months were necessary in order to obtain matured coir pith compost even though aeration was provided. It is necessary then to correct it in order to promote composting. This correction can take the form of addition of sludge, manure, seaweed or other nitrogen rich substrates. Table 1 indicates some raw materials used for correction in order to constitute a balanced C/N ratio of 30. There is a high variability between the raw materials concerning their organic matter content that depends on the component from which the material was made of. Materials with high lignin and cellulosic content (trunk, stems sawdust…) have high C/N ratio, due to the complexity of carbon chains. Raw materials with high C/N ratio are very difficult to be composted and could take over 10 months to be decomposed (Yulipriyanto 2001).
In contrast, if the C/N ratio is low, nitrogen losses to the atmosphere are relatively higher. The work of Tiquia and Tam (2000) on the composting of chicken litter showed a C/N ratio of 20 resulted in a loss of nitrogen through volatilization of NH3 at the beginning of composting. The C/N ratio of microorganisms is about 10 and this value is theoretically the best for their metabolism. Nevertheless, this low value can lead to a loss of nitrogen through volatilization of ammonia due to high values of pH and high temperature.
Tripetchkul et al. (2012) studied also the effect of initial C/N ratios (20; 25 and 30) on the C/N ratio evolution. A decrease in C/N ratio was observed in all treatments (Fig. 3), which implies an increasing degree of organic matter humification as long as the process is taking place. The C/N ratio of the three piles decreased substantially until 21 days of post composting and became comparatively stable. The compost piles containing 30:1 and 25:1 C/N ratio yielded a more rapid decrease in C/N ratio than that observed with the 20 C/N ratio formulation. Such evolution matched well with the significant reduction in organic matter indicating a rapid biodegradation rate. Further, initial C/N ratios posed significant influence on the composting performance assessed in terms of temperature evolution, OM degradation, and TN loss. Kumar et al. (2010) have studied the co-composting of food waste and green waste at low initial carbon to nitrogen (C/N) ratios. The results indicate that the optimal moisture content for co-composting of food waste and green waste is 60%, and the substrate at a C/N ratio of 19.6 can be decomposed effectively to reduce 33% of total volatile solids (TVS) in 12 days. In addition, the compost passes the standard germination index of white radish seed indicating that it can be used as soil amendment.
C/N ratio evolution under different initial C/N ratios of coir pith composting (Tripetchkul et al. 2012)
The C/N ratio is a crucial indicator both as a start-up and quality parameter. In fact, the amendment of a material with low C/N ratio, which has not successfully completed the composting process, can lead to phytotoxicity. It is caused by the conversion of ammonium (in hot and humid conditions) into ammonia, creating a toxic environment for plant growth, and resulting in odours. Similarly, unfinished compost contains unstable volatile chemicals such as organic acids that are toxic to seeds and plants. In the other hand, the amendment of a materiel with high C/N ratio can lead a biological block of nitrogen, also known as “nitrogen starvation”. It occurs in materials that are far richer in carbon than in nitrogen. When applied to soil, microorganisms quickly use de C present in the material increasing the consumption of N and exhausting the reserves of N (Roman et al. 2015). Thus, the quality of the produced compost depends largely on the level of start-up C/N ratio and the quality of its constituents within the mixture (Azim et al. 2014).
Moisture
Moisture is a parameter closely related to microorganisms, because, like all living beings, they use water to transport nutrients and energy elements through the cell membrane (Roman et al. 2015). Stabilization of optimum moisture during composting is relatively difficult, particularly for composting in an open environment. Practically, this problem is often solved by monitoring the temperature that informs well the best moment to turn; moisten, and/or ventilate the pile (Tiquia and Tam, 1998). Low initial moisture values (less than 30%) can lead to rapid dehydration of compost that pauses the biological process, and provide physically stable but biologically unstable compost (De Bertoldi et al. 1983). In contrast, high humidity values (more than 80%) generate anaerobic conditions in the compost. It is therefore important to determine the best values of moisture for composting process (Yulipriyanto 2001). In Fig. 4, Razmjoo et al. (2015) found that the moisture between 45 and 50% is an optimum range for the composting process. When moisture is less than 30%, the bacterial activities will be limited and that above 65% will decrease the porosity of the compost resulting in an anaerobic growth and unpleasant odour emissions. Variation of the moisture content in monitored windrows had normal trends starting from 46 to 51% in the 1st month and lowering to 42–47% at the end of the third month operation. However, the moisture content of the first windrow was the lowest due to the higher paper content of its raw materials. Manually water spraying on the first windrow during the third month caused its humidity to be increased. Nevertheless, humidity ranges appeared to be ideal for the composting process in this study.
Moisture content evolution of municipal solid waste composting plant (Razmjoo et al. 2015)
An organic waste pile prior to composting presents water both, in the form of a liquid film around the organic particles (solid fraction), and in the pore space (in the form of water vapour). These pore spaces have an essential function for the airflow in the compost pile (Kumar et al. 2010). The optimal rate of humidity for a given substrate is determined by the percentage of pore space that does not obstruct the flow of air required for the activity of microorganisms. Experience has shown that, for most mixtures, the initial moisture content should be between 40 and 65%. The optimal values of pore space in the pile are between 30 and 36% for an efficient aeration in a composting process (Rynk 1992, Mustin 1987). During composting, the water content tends to increase due to release of metabolic water by microorganisms that break down organic matter in the presence of oxygen. The water content decreases under the combined action of the rise in temperature and forced ventilation, leading to its loss as a water vapour. In addition, the optimum moisture content varies and depends essentially on the physical structure and the particle size change during composting and thus, moisture and aeration are closely related (Gigliotti et al. 2012).
There is no universally applicable optimum moisture for composting materials. This is because each material has its unique physical, chemical and biological characteristics, and these affect the relationship between moisture and its corollary factors water availability, particle size, porosity, and permeability (Makan et al. 2013). However, the quality of municipal solid wastes compost rely on additional trace element analysis and physical parameters (Woodbury and Breslin 1992).
Porosity, bulk density, and oxygen content
The bulk density of compost is a measurement of the mass of material within a given volume. The density of compost also influences the mechanical properties such as strength, porosity and ease of compaction. Typical dry bulk densities are in the range 100 to 400 kg.m−3 whereas wet bulk densities are typically 500 to 900 kg.m−3 (Agnew and Leonard 2003). Higher values of bulk density imply an increase in mass and a decrease of porosity and air volume. On the contrary, very low wet bulk density can indicate excessive substrate aeration and, indirectly, a drop in the available water fraction (Nappi and Barberis 1993).
The porosity (pore space) of the pile in composting, must allow degradation under aerobic conditions. Thus, the porosity is positively correlated to the airflow. In order to maintain the aerobic condition inside the compost pile, a minimum rate of 5% O2 in pore space is required, while anaerobic condition occurs with less than 1% of O2 (Mustin 1987). Anaerobic compartments may also appear during composting in some less aerated part of the pile (Finstein et al. 1999).
These physical characteristics of the compost mixture can interact with high moisture levels to reduce oxygen transport. Small particle sizes reduce the number of large pores and increase the likelihood that oxygen will need to diffuse a long way through small pores. The shape, size, and structure of particles affects how they settle, with tight packing arrangements increasing the bulk density and reducing the air filled porosity (free air space). Compaction (caused mechanically or by the weight by overburden in tall piles) encourages tight packing as well. Supposing that a pile of compost has an initial air filled porosity of 30%, and the volume of a pile is reduced by 10% through compaction and size reduction after the first pass of a windrow turner. Assuming constant moisture and solids content, the air filled porosity would be reduced from 30 to 20%, with a 33% reduction, and the apparent diffusion coefficient will be reduced by 56%. This significant change in oxygen diffusion might easily pass unnoticed in the windrow itself, where volume reductions of greater than 50% are common, and 80% is not unheard of with an initially loose material like autumn leaves. To the extent that natural or forced convection is important in oxygen transport, small diameter pores can cause problems even if total air filled porosity is maintained. The increased frictional resistance from the walls in small pores reduces any mass flow of oxygen that would otherwise occur. In addition, the air channels in a composting matrix are not a bundle of continuous straight tubes, but twist and bend in tortuous path, with many dead ends and narrow passages. These factors further reduce oxygen transport in ways that are difficult to quantify (Papendick and Campbell, 1981).
The oxygen content depends on the porosity of the compost pile; therefore, attention must be given to the size, the shape of the organic particles and the amount of water present in the pores (and thus the moisture of the pile). Moreover, the CO2 concentration should not exceed 15%; this concentration corresponds to approximately 6% oxygen. The oxygen demand is very high during the initial decomposition phase (15–20%) because of the rapid development of the microorganisms. After that, the oxygen demand, decreases during the stabilization of the compost (5 to 10%) and even more during the maturation phase (Mustin 1987).
Khater (2015) have conducted an experiment to study the physical and chemical properties of compost made of different raw material with different proportions. Fig. 5 “A” reveals the relationship between the bulk density and the total organic matter. It decreases from 655 to 420 kg m−3 when the total organic matter increased from 28.6 to 41.2%. The bulk density of compost is negatively correlated with the compost total organic matter (R 2 = −0.89). Fig. 5 “B” indicates that the porosity of compost decreased from 72.47 to 60.69% when the bulk density increased from 420 to 655 kg m−3. These results further indicate that the porosity of compost increased from 60.7 to 72.5% with the moisture content increasing from 25.6 to 32.1% (Fig. 5 “C”). The porosity is negatively correlated to bulk density (R 2 = 0.93) and positively correlated to moisture content of compost (R 2 = 0.60). Those results are in conformity with the findings of Ahn et al. (2008) who suggested that the increasing porosity makes available water flux in both forms as water vapour nor liquid form.
Physical properties (bulk density, moisture content, and porosity) of the different types of compost (Khater 2015)
Monitoring parameters
Since composting is a biological process carried out by microorganisms, parameters affecting their growth and reproduction should be taken into account. These factors include temperature, pH, C/N ratio, oxygen, or aeration and the substrate moisture. Externally, the composting process largely depends on environmental conditions; the method used, raw materials, and other elements, so that some parameters may vary. However, they must be under constant surveillance to always be within an optimal range (Roman et al. 2015). The main parameters and their optimal ranges are listed below.
Temperature
Temperature is a critical parameter for composting. High temperatures have often been considered as a necessary condition for compost sanitation. Optimal temperatures are those that achieve the desired objectives: sanitation, rapid degradation, water evaporation, and humification. High temperatures should be avoided, since they slow the biological activity and cause undesirable chemical modifications of the organic matter. Low temperatures as well are undesirable because they do not achieve the remediation objectives (Mustin 1987).
High temperatures are attributed to the biological activity, the heat being generated by the lysis of carbon bonds in the biodegradation process. The heat flux is then an indicator of the successful composting. It can be regulated by aeration (removal of heat by ventilation or turning). More generally, the generation of metabolic heat, temperature, ventilation, and humidity are the four interacting factors during the composting process (Strom 1985). The experiments of McKinley and Vestal (1985) on municipal waste composting, shows that temperature is the main factor affecting the microbial metabolism. The optimum temperature for the composting of the substrate is below 55 °C, and the microbial activity is at its highest level when the temperature of municipal waste composting is below 58 °C. However, composting temperature must be maintained at a level that ensures the reduction of pathogens, which is usually 55 °C for 3 to 5 days. Monitoring of the temperature is highly required to insure the removal of pathogens, while keeping the microbial community in optimal conditions.
For rapid composting, high temperatures for longer periods should be avoided. A first thermophile stage may be useful to neutralize the heat-sensitive pathogens. After this step, it is preferable to reduce the temperature to levels that permit the development of eumycetes and actinomycetes, main decomposers of long chain polymers, cellulose and lignin. Optimum temperatures vary from 45 to 55 °C (De Bertoldi et al. 1983). Parr et al. (1994) has explained in Fig. 6, the theoretical evolution of the pile temperature during composting. Curve 1 indicates that conditions of moisture, temperature, C/N ratio, and aeration are at optimum levels for rapid aerobic, thermophile composting. Within several days, the internal pile temperatures increase rapidly from the mesophile (20 to 40 °C) into the thermophile (>40 °C) stage, after which the temperature begins to decline as the indigenous microorganisms deplete available nutrients. Curve 2 indicates what might happen when certain parameters are deficient or outside their optimum range, which would limit the growth and activity of microorganisms and adversely affect the desired time-temperature transition for successful composting.
Temperature evolution during composting (Parr et al. 1994)
pH
The composting process is relatively indifferent to pH, within the limits commonly found in mixtures of organic materials. Its importance is especially sensitive for substrates rich in nitrogen, where high values favour the conversion of nitrogen bound to organic compounds and ammonia nitrogen volatilization as ammonia (Rynk 1992). According to De Bertoldi et al. (1983), organic material can be composted in a broad pH range (3–11). Optimal values are between 5.5 and 8. Values around neutrality are optimal for microorganisms’ development. However, fungi are more tolerant to distant neutral pH than bacteria.
The pH may decrease in the first stage of composting, due to the organic acids releasing during the decomposition of simple organic substrates, and volatilization of the initial ammonia. Thereafter, the disappearance of easily degradable organic materials and mineralization leading to an increase in pH (McKinley and Vestal 1985). At the end of composting, it is also possible to end in an acidic pH due to H+ ions released during nitrification (Fang and Wong 1999). In reality, the changes in the pH depend significantly on the raw materials and any additives component of the initial mixture as reported by Eklind and Kirchmann (2000) in the case of compost made from waste wood and paper. It also depends on the conditions of airflow of composting, for effective ventilation, for example, allowing a good degradation of the organic material and resulting in a higher final pH (Ferrer 2001). Temperature also plays a role in the evolution of pH, favouring ammonia volatilization.
The pH changes several times during composting. Generally, there are four phases (Poincelot 1972) (Fig. 7):
-
Acid-genesis Phase (I): the pH decreases, flora produces a lot of CO2 and organic acids early in the thermophile phase,
-
Alkalization Phase (II): with increasing pH, bacterial hydrolysis of protein and organic nitrogen produces ammonia,
-
pH stabilization phase (III): The C/N ratio decreases, the reactions become slower. Ammonia is lost by volatilization (especially with a pH > 8), and nitrogen is used by microbes to synthetize new humic compounds,
-
Stable Phase (IV): near to neutral pH, the compost is being in maturation. This stability is due to the slow reactions and influence of humus buffer.
pH evolution curve during composting (Poincelot 1972)
Tripetchkul et al. (2012) studied the influence of low C/N ratio on naturally aerated composting of high lignocellulosic materials, particularly coir pith, under several C/N ratios. Concerning pH evolution, Fig. 8 shows an increase in pH of all compost piles at the beginning of composting, especially during the thermophilic phase. It was due likely to the metabolic degradation of organic acids and the ammonification process that take place during organic matter degradation. After the 14th day, the pH values of all piles significantly declined which is likely to be a consequence of phenolic compound production and organic acid production during biodegradation of lignocellulose in the compost piles.
pH evolution of three composts with different initial C/N ratio (Tripetchkul et al. 2012)
Organic carbon
Organic carbon is one of the main constituents of composted organic wastes. Total carbon consists of the total organic carbon (TOC) and inorganic carbon in the form of carbonates and bicarbonates. TOC generally represents more than 90% of total carbon in composts (Navarro et al. 1993). Raw green wastes contain 20 to 30% of TOC (Riffaldi et al. 1986; Vallini et al. 1993), 25 to 50% for household waste (Avnimelech et al. 1996) and 30 to 40% in sludge (Diaz-Burgos et al. 1993; Ayuso et al. 1996; Bernal et al. 1998). The TOC content decreases during composting because of microorganisms degradation of organic substances necessary for their metabolism, leading to their mineralization as carbon dioxide (CO2). The possible presence of anaerobic sites in the compost pile may result in methane (CH4) emissions associated with fermentative metabolism (He et al. 2000). According to Beck-Friis et al. (2003), the emission of methane, occurs only during the thermophile phase, representing less than 2% of the initial TOC in the case of a poorly ventilated compost pile. Volatile fatty acids, found in young composts can also be released during composting. They appear in the case of an incomplete oxidation of organic carbon and therefore reflect an anaerobic metabolism. They are present only in the mesophile phase of composting, represent less than 10% of the TOC, and contribute to decrease the pH (Michel and Reddy 1998; Beck-Friis et al. 2003).
Nitrogen
During composting, organic nitrogen of waste is mineralized mainly into ammonium (NH4 +) and nitrate (NO3 −) when nitrification is achieved. Part of this mineral nitrogen is reincorporated into the active microbial metabolism during composting, some is incorporated into organic matter compost in their humification, and part is released in the form of inorganic nitrogen matrix (Larsen and McCartney 2000). At the end of composting, mineralization process become predominant, and an increase in the content of NO3 − is frequently observed (Sanchez-Monedero et al. 2001). Therefore, an increase in total nitrogen concentration is generally observed in the matured compost.
However nitrogen losses are possible, either by nitrate leaching in the case of unprotected composts piles under rainy season, or by volatilization of ammonia (NH3), and nitrous oxide (N2O). NH3 emissions that represent the major losses are closely correlated to the pH and the presence of volatile fatty acids. They take place during the intensive phase of degradation. Under optimal aeration condition, the pH rise causes a transformation of NH4 + into a volatile nitrogen (NH3). On the other hand, the limited ventilation causes an increase in the content of volatile fatty acids, resulting in a decrease of the pH, and locking of nitrogen as NH4 + (Michel and Reddy 1998). The total nitrogen is generally 1 to 4% of the total dry weight of compost, and is composed of at least 10% of mineral nitrogen (Hirai et al. 1986, Willson 1989, Kapetanios et al. 1993, Canet and Pomares 1995, Bernal et al. 1998, Brinton and Evans 2000).
Quality parameters
Definition
The quality of compost is difficult to define and remains an elusive concept (Lasaridi et al. 2006). The stability and maturity of the compost is essential for optimum use as an amendment and nutrient source for plants (Amir 2005). Francou (2003) considers that the term of maturity is often ambiguous although frequently cited in the literature. In most articles, the stability and maturity are either defined only implicitly but is not defined at all.
Two separate approaches coexist to describe the quality of compost:
-
The first is based on the notion of transformation of an initial instable organic matter into a stable organic on at the end of composting. The degree of stability of the compost is estimated by the biodegradability of organic materials and their humification status (Bernal et al. 1998, Chen 2003, Grigatti 2004, Castaldi et al. 2005, Adani et al. 2006).
-
The second approach considers the effects of compost on plants. The degree of maturity, in this case, linked to the absence of damage to plants due to the use of compost. Indeed, Tiquia et al. (1997) explain that the amendment of immature compost in a soil has negative effects on germination, growth and development of plants. In this context, the best indicator of maturity of compost remains its phytotoxicity. Tiquia and Tam (1998) and Alburquerque et al. (2006) confirm in several studies of this issue, that stable compost does not necessarily mean that it is mature as it can still have an inhibitory or phytotoxic effect on plant growth.
Empirical methods
The practice of composting has been known for a long time. Quite a lot of empirical methods have allowed users to determine the maturity of composts well before the rise of modern science. The sensory approach allows, for example, to judge on maturity (Iglesias-Jiménez and Pérez-García 1991, Mbuligwe et al. 2002). Ripe compost comprises the following features:
-
Does not emit ammonia odour;
-
Temperature is low even if the compost is moist and returned;
-
Granular, dark woods and pleasant odour;
-
Distinguishable with regard to the raw material.
However, these simple and rapid methods must be supplemented by more precise laboratory analyses (Charnay 2005).
Conventional physicochemical characteristics
Most of studies on compost maturity are based on the evolution of the physic-chemical parameters such as pH, C/N ratio, organic matter content, humification ratio, cation exchange capacity (CEC) (Albrecht 2007).
pH
The pH was one of the first chemical indicators of the compost maturity and has been used in many studies (Albrecht 2007). Iglesias-Jiménez and Pérez-García (1991) and Cayuela et al. (2006) have observed an increase in pH during composting. According to Avnimelech et al. (1996), the acid pH levels are characteristic of immature compost while mature composts have pH between 7 and 9.
Cation exchange capacity (CEC)
Humic compounds have a high capacity to adsorb positively charged ions, which are then easily exchanged with other cations on the same adsorption sites. This ability, referred to as “cation exchange capacity” (CEC), tends to increase during composting as organic materials are humified and carboxyl and phenolic functional groups are formed (Wichuk and McCartney 2013). Iglesias-Jiménez and Pérez-García (1991) recommend that a CEC greater than 60 meq.100 g−1 of organic matter is necessary to consider compost as mature.
C/N ratio
The C/N ratio (organic carbon/organic nitrogen) decreases during composting. This parameter is commonly measured to assess the maturity of compost (Albrecht 2007). Iglesias-Jiménez and Pérez-García (1991) estimate that a ratio of less than 20 and even 15 characterizes mature composts. Chefetz et al. (1996) and Namkoong et al. (1999) explain that compost characterized by a ratio of 10 to 15 can be considered stable although the final ratio depends significantly on the initial materials used. The C/N ratio in compost is comparable to the C/N ratio of humic soil, which is close to 10 (Charnay 2005).
NO3 −/NH4 + ratio
The appearance of nitrates in compost can be a maturity indicator. Nitrifying microorganisms induce a decrease of ammonium content (NH4 +) and an increase in nitrate (NO3 −) production. Some authors thus use the NO3 −/NH4 + ratio as an indicator of compost maturity (Albrecht 2007). However, according to Francou (2003), this ratio is slightly used and the results are contradictory.
Humification ratio (HA/FA)
The humification processes have led many authors to study the humified organic matter or humic substances. The chemical fractioning of organic matter in humin, humic, and fulvic acids has led some authors to develop maturity indicators. Several studies showed a significant increase of humic acid (HA) fulvic acid (FA) ratio (HA/FA) during composting (Veeken et al. 2000, Jouraiphy et al. 2005, Huang et al. 2006). In fact, the composting process involves the formation of fulvic acids (FA) as an intermediate step in the formation of humic acids (HA) and, finally, insoluble, non-phytotoxic humic substances. As a result, the fraction of humic compounds making up the total dry matter or total OM is expected to increase to a nearly constant value as compost matures (Wichuk and McCartney 2013). Results found in the literature are quite consistent with values less than 1 for immature composts, and greater than 1 or 3 for mature composts.
Biological activities
Measurements of respiration (BOD = Biological oxygen demand)
The respiration measures are based on the respiratory activity of microorganisms in the compost. Immature compost is characterized by a significant rate of O2 demand and CO2 production. The intense microbial activity causes a high biodegradability of the substrates and thus a significant oxygen demand in comparison with a mature compost, more stable, and less active (Bernal et al. 1998; and Lasaridi and Stentiford 1998). Grigatti et al. (2011) showed that the measurement of the OUR (oxygen uptake rate) by means of a standardized test proved to be a valid tool to describe the stabilization level variation during the composting process.
Enzymatic activity
The important enzymes involved in the composting process include cellulase, hemicellulase, phenoloxidase, proteases, lipases, phosphatases, and their activities change depending on the degree of decomposition of organic matter. Thus, high levels of protease activity, lipase, and cellulase were measured during the active phase of composting (Mondini et al. 2004; Goyal et al. 2005). However, composting is made from very different organic substrates and many processes; Mondini et al. (2004) consider that the establishment of threshold values of enzyme activities as a maturity index is extremely difficult.
Self-heating test
The intense activity of microorganisms in immature composts results in heat production during the degradation of the simplest and most accessible compounds. Thus, it is possible to measure the degree of decomposition of the organic material in a compost by measuring the temperature rise after moistening. Several studies have demonstrated the relevance of this test to assess the maturity of composts (Brinton et al. 1995; Leifeld et al. 2001).
Solvita® test
Based on the mineralization of the carbon and the volatilization of ammonia, there is a maturity test promoted under the test name of Solvita® (Woods Research® Management, USA). This test is performed on the compost whose moisture is adjusted to a level corresponding to the optimal microbial activity. It combines an estimation of carbon mineralization and an estimation of ammonia volatilization compost placed in a sealed bottle of 200 ml, with two coloured indicators, which refer to a global index. A number greater than 6 on a scale of 1 to 8 characterizes a cured compost (Francou 2003). This latest test is used on platforms in Denmark and the USA. It is the subject of recent and rare publications, which revealed its relevance especially for composts with advanced stability (Brinton and Evans 2000). On the other hand, it is less correlated with self-heating test (Brinton and Evans 2000). In addition to the stability evaluated by released CO2, potential phytotoxicity associated with the presence of ammonium is estimated by volatilized ammonia (Changa et al. 2003).
Francou (2003) gave in Tables 2 and 3, respectively, different methods for assessing the maturity of composts, and relevant quality parameters and has given intervals with thresholds.
Succession of nematode populations
Steel et al. (2010) found that at the beginning of the composting process (thermophilic phase), immediately after the heat peak, the nematode population is primarily built by bacterial feeding enrichment opportunists (Rhabditidae, Panagrolaimidae, Diplogastridae) followed by the bacterial-feeding general opportunists (Cephalobidae) and the fungal-feeding general opportunists (Aphelenchoididae). Thereafter, during the cooling and maturation stage, the bacterial-feeding-predator opportunistic nematodes (Mononchoides sp.) became dominant. Finally, at the most mature stage, the fungal-feeding Anguinidae (mainly Ditylenchus filimus) were present. The fungivorous/bacterivorous ratio (f/b ratio), increase as the compost becomes more mature (ranging from zero to 11.90). Based on these results, the f/b ratio could be suggested as potential tool to assess compost maturity.
Overall index of composting development (OICD)
The wide range of maturity parameters, complexity, and simplicity of certain analysis methods, but their reliability and precision, makes the estimation of compost maturity a delicate task in which no parameter is used distinctly. Goyal et al. (2005) and Boulter-Bitzer et al. (2006) suggested a combination of different parameters. Consequently, Albrecht et al. (2009) proposed a combination as an Overall Index of Composting Development (OICD) that takes into account all the information of 14 physical and chemical parameters using the PCA (principal component analysis). The OICD was calibrated with the results of near infrared spectrophotometry (NIRS) technology and the chemical and biological data of the analysed samples. Thus, NIRS can replace conventional methods of analysis of the compost maturity, and even predict OICD that can be used to assess accurately, efficiently, and quickly the maturity of composts.
Greenhouse effect
The contribution of agricultural sector to climate change by greenhouse gas (GHG) emissions is becoming better understood. At the same time, the potential of agriculture to contribute to the reduction of the GHG emission was largely neglected in the past. A little known fact is that it has been estimated that more than twice as much carbon is retained in the soil as organic matter, as in vegetation or the atmosphere (Batjes 1996). In the context of the GHG reduction, soil incorporation of products containing stabilized organic matter seems to be a reasonable solution. Indeed, compost, compared to untreated material (sewage sludge) increases the carbon stabilization (Albrecht 2007). Favoino and Hogg (2008) found that the greenhouse gas perspective, evaluations led in the past neglected the important positive effects of composting and compost application on:
-
1.
Improving organic carbon soil reserves;
-
2.
Its effects on improving the absorption of nutrients;
-
3.
The high energy consumption avoided for the production of equivalent chemical fertilizers;
-
4.
The avoided release nitrous oxide from fertilizer by allowing the release of nutrients from organic amendments.
Based on the average of the recalcitrant carbon contents of major types of compost, and the annual production, Houot (2002) evaluates to 1 million tons of carbon storage potential in the soil. The development of composting and compost amendment having a stabilized organic matter increases this potential. The use of low-stabilized organic matter has the opposite effect of increasing soil microbial activity and thus the return to the atmosphere of carbon as a carbon dioxide (Ginting et al. 2003).
The potential GHG impact of composting of different raw material was evaluated with a review of literature focusing on the avoided release of methane (CH4). The option of organic waste management took into account composting raw materials instead of being burying them directly in the soil or their surface lagooning. Moreover, GHG emissions during composting were higher for nitrogen rich materials and high moisture content. This finding may be adjusted by the use of raw materials with a high C/N ratio and lower moisture contents. Studies have confirmed that composting in addition to the environmental benefits associated with this practice can be an effective way to reduce greenhouse gas emissions of a range of organic waste materials (Brown and Subler 2007).
Conclusion
The literature survey showed that there are many parameters that can be considered for start-up, monitoring and quality of compost. However, it becomes challenging when extrapolating the process into field and industrial level. Nowadays, gas and moisture sensors become to be used in monitoring by large composting companies, but farmers and small composters cannot afford the device and the analytical cost. Therefore, scientists have a great responsibility to perform simple field test for monitoring the composting process. Furthermore, the start-up parameters could be gathered in a database and then given for both initial C/N and moisture calculation. Spectral infrared analysis (FTIR and NIRS) start to be adapted to assess the maturity of the produced compost, but there is a consistent work to calibrate the method in order to fit to every composted organic waste. Cress test and phytotoxicity test remain affordable maturity tests for field and laboratory testing. According to literature, initial C/N (25–30), initial moisture (50–60%) and O2 content in pore space (15%) are required to start a significant thermophile phase. However, those values can change in order to accelerate (decreasing initial C/N and increasing initial O2 content in pore space) the process or lowering it by reversing the initial conditions. Composting is an exciting global process to turn wastes into resources, but it should be locally adapted taking into consideration the cost, the nature of the waste and the environmental impact of the produced compost amended to the soil.`
References
Adani F, Genevini P, Tambone F (1995) A new index of organic matter stability. Compost Science & Utilization 3(2):25–37
Adani F, Genevini P, Gasperi F, Tambone F (1999) Composting and humification. Compost Sci Util 7(1):24–33
Adani F, Ubbiali C, Generini P (2006) The determination of biological stability of composts using the dynamic respiration index: the results of experience after two years. Waste Manag 26(1):41–48
Agnew J, Leonard J (2003) The physical properties of compost. Compost Sci Util 11(3):238–264
Ahn H, Richard T, Glanville T (2008) Laboratory determination of compost physical parameters for modeling of airflow characteristics. Waste Manag 28(3):660–670
Albrecht R (2007) Co-compostage de boues de station d’épuration et de déchets verts : Nouvelle méthodologie du suivi des transformations de la matière organique. Dissertation, Faculté des Sciences et Technique St Jérôme, L’Université Paul Cézanne, Marseille, France, p. 189
Albrecht R, Joffre R, Petit J, Terrom G, Périssol C (2009) Calibration of chemical and biological changes in co-composting of biowastes using near-infrared spectroscopy. Environ Sci Technol Lett 43(3):804–811
Alburquerque J, Gonzálvez J, García D, Cegarra J (2006) Measuring detoxification and maturity in compost made from “Alperujo”, the solid by-product of extracting olive oil by the two-phase centrifugation system. Chemosphere 64(3):470–477
Amir S (2005) Contribution à la valorisation de boues de stations d’épuration par compostage : devenir des micropolluants métalliques et organiques et bilan humique du compost, Thèse de Doctorat, Ecole Nationale Supérieure Agronomie, Institut National Polytechnique (ENSAT-INP), Toulouse, France, p. 341
Antizar-Ladislao B, Lopez-Real J, Beck A (2006) Investigation of organic matter dynamics during in-vessel composting of an aged coal–tar contaminated soil using fluorescence excitation–emission spectroscopy. Chemosphere 64(5):839–847
Avnimelech Y, Bruner M, Ezrony I, Sela R, Kochba M (1996) Stability indexes for municipal solid waste compost. Compost Sci Util 4(2):13–20
Ayuso M, Pascual J, García C, Hernández T (1996) Evaluation of urban wastes for agricultural use. Soil Sci Plant Nutr 42(1):105–111
Azim K, Ouyihya K, Amellouk A, Perissol C, Thami-Alami I and Soudi B (2014) Dynamic composting optimization through C/N ratio variation as a startup parameter. In: Rahmann, G. and Aksoy, U. (Eds.) Building Organic Bridges, Johann Heinrich von Thünen-Institut, Braunschweig, Germany, 3, Thuenen Report, no. 20, pp. 787–790. http://orgprints.org/23554/1/23554_MM.pdf (Accessed 20 February 2017).
Azim K, Komenane S, Soudi B (2017) Agro-environmental assessment of composting plants in southwestern of Morocco (Souss-Massa region). Int J Recycl Org Waste Agricult. doi:10.1007/s40093-017- 0157-7
Batjes NH (1996) Total carbon and nitrogen in the soils of the world. Eur J Soil Sci 47:151–163
Beck-Friis B, Smars S, Jönsson H, Eklind Y, Kirchmann H (2003) Composting of source-separated household organics at different oxygen levels: gaining an understanding of the emission dynamics. Compost Sci Util 11(1):41–50
Bernal M, Navarro A, Sánchez-Monedero M, Roig A, Cegarra J (1998) Influence of sewage sludge compost stability and maturity on carbon and nitrogen mineralization in soil. Soil Biol Biochem 30(3):305–313
Bidlingmaier W (1993) Odour emissions from composting plants. Compost Sci Util 1(4):64–68
Boulter-Bitzer J, Trevors J, Boland G (2006) A polyphasic approach for assessing maturity and stability in compost intended for suppression of plant pathogens. Appl Soil Ecol 34(1):65–81
Brinton WF and Evans E (2000) Plant Performance in Relation to Oxygen Depletion, CO2-Rate and Volatile Fatty Acids in Container Media Composts of Varying Maturity. In: Insam H, Riddech N and Klammer S (eds) Microbiology of Composting, XII edn. Springer, pp 335–343.
Brinton WF, Evans E, Droffner ML, Brinton RB (1995) Standardized test for evaluation of compost self- heating. Biocycle 36:64–69
Brown S, Subler S (2007) Composting and greenhouse gas emissions: a Producer’s perspective. Biocycle 48(3):37–41 https://www.biocycle.net/2007/03/23/composting-and-greenhouse-gas-emissions-a-producersperspective/ (Accessed 06 February 2017
Canet R, Pomares F (1995) Changes in physical, chemical and physico-chemical parameters during the composting of municipal solid wastes in two plants in Valencia. Bioresour Technol 51(2–3):259–264
Castaldi P, Alberti G, Merella R, Melis P (2005) Study of the organic matter evolution during municipal solid waste composting aimed at identifying suitable parameters for the evaluation of compost maturity. Waste Manag 25(2):209–213
Cayuela M, Sánchez-Monedero M, Roig A (2006) Evaluation of two different aeration systems for composting two-phase olive mill wastes. Process Biochem 41(3):616–623
Changa C, Wang P, Watson M, Hoitink H, Michel F (2003) Assessment of the reliability of a commercial maturity test kit for composted manures. Compost Sci Util 11(2):125–143
Charnay F (2005) Compostage des déchets urbains dans les Pays en Développement. Elaboration d’une démarche méthodologique pour une production pérenne de compost. Dissertation, University of Limoges, p. 277
Chefetz B, Hatcher P, Hadar Y, Chen Y (1996) Chemical and biological characterization of organic matter during composting of municipal solid waste. J Environ Qual 25(4):776
Chen Y (2003) Nuclear magnetic resonance, infra-red and pyrolysis: application of spectroscopic methodologies to maturity determination of composts. Compost Sci Util 11(2):152–168
Chen Y and Aviad T (1990) Effect of humic substances on plant growth. In MacCarthy P, Clapp CE, Malcolm RL, Bloom PR et al. (eds) Humic substances in soil and crop sciences: Selected readings, American Society of Agronomy, Madison, WI), pp 161–186.
Chen Y, Magen H, Riov J (1994) Humic substances originating from rapidly decomposition organic matter: properties and effects on plant growth. In: Senesi N, Miano TM (eds) Humic substances in the global environment and implication on human Health. Elsevier Science B.V, London, pp 427–445
Choi K (1999) Optimal operating parameters in the composting of swine manure with wastepaper. J Environ Sci Health 34(6):975–987. doi:10.1080/03601239909373240
De Bertoldi M, Vallini G, Pera A (1983) The biology of composting: a review. Waste Manag Res 1(1):157–176
Deiana S, Gessa C, Manunza B, Rausa R, Seeber R (1990) Analytical and spectroscopic characterization of humic acids extracted from sewage sludge, manure, and worm compost. Soil Sci 150(1):419–424
Diaz-Burgos M, Ceccanti B, Polo A (1993) Monitoring biochemical activity during sewage sludge composting. Biol Fertil Soils 16(2):145–150
Eklind Y, Kirchmann H (2000) Composting and storage of organic household waste with different litter amendments. I: carbon turnover. Bioresour Technol 74(2):115–124
El Fels L, Lemee L, Ambles A, Hafidi M (2014) Identification and biotransformation of lignin compounds during co-composting of sewage sludge-palm tree waste using pyrolysis-GC/MS. Int Biodeterior Biodegradation 92:26–35
Fang M, Wong J (1999) Effects of lime amendment on availability of heavy metals and maturation in sewage sludge composting. Environ Pollut 106(1):83–89
Favoino E, Hogg D (2008) The potential role of compost in reducing greenhouse gases. Waste Manag Res 26(1):61–69
FCQAO (1994) Methods book for the analysis of compost. Compost information N°230. BGK ed. https://www.kompost.de/fileadmin/docs/shop/Grundlagen_GS/Methods_Book_2002.pdf (Accessed 23 February 2017)
Ferrer J (2001) Agronomic use of biotechnologically processed grape wastes. Bioresour Technol 76(1):39–44
Finstein MS, Strom PF, Hogan JA, Cowan RM (1999) Composting on Mars or the moon: I. Comparative evaluation of process design alternatives. Life Support Biosph Sci 6(3):169–179
Forster J, Zech W, Wurdinger E (1993) Comparison of chemical and microbiological methods for the characterization of the maturity of composts from contrasting sources. Biol Fertil Soils 16(2):93–99
Francou C (2003) Stabilisation de la matière organique au cours du compostage de déchets urbains : Influence de la nature des déchets et du procédé de compostage – Recherche d’indicateurs pertinents. Dissertation, Institut national agronomique Paris- Grignon, p. 289
Fuangworawong P (2008) Influence of Nitrogen Sources, Molasses Addition and Aeration Schemes on Composting of Coir Pith. Dissertation, King Mongkut’s University of Technology Thonburi.
Gagnon B, Simard RR, Robitaille R, Goulet M, Rioux R (1997) Effect of composts and inorganic fertilizers on spring wheat growth and N uptake. Can J Soil Sci 77:487–495
Gigliotti G, Proietti P, Said-Pullicino D, Nasini L, Pezzolla D, Rosati L, Porceddu P (2012) Cocomposting of olive husks with high moisture contents: organic matter dynamics and compost quality. Int Biodeterior Biodegradation 67:8–14
Ginting D, Kessavalou A, Eghball B, Doran J (2003) Greenhouse gas emissions and soil indicators four years after manure and compost applications. J Environ Qual 32(1):23
Golouke CG (1991) Principles of composting. In: Biocycle Guide to the Art and Science of Composting, pp. 14–37.
Goyal S, Dhull S, Kapoor K (2005) Chemical and biological changes during composting of different organic wastes and assessment of compost maturity. Bioresour Technol 96(14):1584–1591
Grigatti M (2004) Evolution of organic matter from sewage sludge and garden trimming during composting. Bioresour Technol 91(2):163–169
Grigatti M, Cavani L, Ciavatta C (2011) The evaluation of stability during the composting of different starting materials: comparison of chemical and biological parameters. Chemosphere 83(1):41–48
Harada Y, Inoko A, Tadaki M, Izawa T (1981) Maturing process of city refuse compost during piling. Soil Sci Plant Nutr 27(3):357–364
Hartz TK, Giannini C (1998) Duration of composting of yard wastes affects both physical and chemical characteristics of compost and plant growth. Hort Science 33, 7:1192–1196
Hassen A, Belguith K, Jedidi N, Cherif A, Cherif M, Boudabous A (2001) Microbial characterization during composting of municipal solid waste. Bioresour Technol 80(3):217–225
He Y, Inamori Y, Mizuochi M, Kong H, Iwami N, Sun T (2000) Measurements of N2O and CH4 from aerated composting of food waste. Sci. Total Environ 254:65–74
Helfrich P, Chefetz B, Hadar Y, Chen Y, Schnabl H (1998) A novel method for determining phytotoxicity in composts. Compost Sci Util 6(3):6–13
Hirai M, Katayama A, Kubota H (1986) Effect of compost maturity on plant growth. Biocycle 27(4):58–61
Houot S (2002) Gestion des déchets organiques hors effluents d’élevage et résidus de récoltes. In Stocker du carbone dans les sols agricoles de France. Rapport d’expertise réalisé par l’INRA à la demande du Ministère del’Ecologie et du Développement Durable, edited by INRA ed.148–152
Huang G, Wu Q, Wong J, Nagar B (2006) Transformation of organic matter during co-composting of pig manure with sawdust. Bioresour Technol 97(15):1834–1842
Iannotti D, Grebus M, Toth B, Madden L, Hoitink H (1994) Oxygen Respirometry to assess stability and maturity of composted municipal solid waste. J Environ Qual 23(6):1177
Iglesias-Jimenez E, Alvarez C (1993) Apparent availability of nitrogen in composted municipal refuse. Biol Fertil Soils 16(4):313–318
Iglesias-Jimenez E, Perez-Garcia V (1989) Evaluation of city refuse compost maturity: a review. Biol Waste 27:115–142
Iglesias-Jimenez E, Perez-Garcia V (1991) Composting of domestic refuse and sewage sludge. I. Evolution of temperature, pH, C/N ratio and cation exchange capacity. Resour Conserv Recy 6(1):45–60
Jedidi N, Van-Cleemput O, M’Hiri A (1995) Quantification des processus de minéralisation et d’organisation de l’azote dans un sol en présence d’amendements organiques. Can J of Soil Sci 75:85–91
Jouraiphy A, Amir S, El Gharous M, Revel J, Hafidi M (2005) Chemical and spectroscopic analysis of organic matter transformation during composting of sewage sludge and green plant waste. Int Biodeterior Biodegradation 56(2):101–108
Kaiser M (1983) L’analyse de la microbiologie du compost. 1ère partie. Compost information 12:9–13
Kapetanios E, Loizidou M, Valkanas G (1993) Compost production from Greek domestic refuse. Bioresour Technol 44(1):13–16
Khater E (2015) Some physical and chemical properties of compost. International Journal of Waste Resources 05(01)
Kostov O, Petkova G, Van Cleemput O (1994) Microbial indicators for sawdust and bark compost stability and humification processes. Bioresour Technol 50(3):193–200
Kumar M, Ou Y, Lin J (2010) Co-composting of green waste and food waste at low C/N ratio. Waste Manag 30(4):602–609
Larsen K, McCartney D (2000) Effect of C/N ratio on microbial activity and N retention: bench-scale study using pulp and paper Biosolids. Compost Sci Util 8(2):147–159
Lasaridi K, Stentiford E (1998) A simple respirometric technique for assessing compost stability. Water Res 32(12):3717–3723
Lasaridi K, Protopapa I, Kotsou M, Pilidis G, Manios T, Kyriacou A (2006) Quality assessment of composts in the Greek market: the need for standards and quality assurance. J Environ Manag 80(1):58–65.5
Leifeld J, Siebert S, Kögel-Knabner I (2001) Stabilization of composted organic matter after application to a humus-free Sandy Mining soil. J Environ Qual 30(2):602
Makan A, Assobhei O, Mountadar M (2013) Effect of initial moisture content on the in-vessel composting under air pressure of organic fraction of municipal solid waste in Morocco. Iranian J Environ Health Sci Eng 10(1):3
Mbuligwe S, Kassenga G, Kaseva M, Chaggu E (2002) Potential and constraints of composting domestic solid waste in developing countries: findings from a pilot study in Dar Es-salaam, Tanzania. Resour Conserv Recy 36(1):45–59
McKinley V, Vestal J (1985) Effects of different temperature regimes on microbial activity and biomass in composting municipal sewage sludge. Can J Microbiol 31(10):919–925
Michel F, Reddy C (1998) Effect of oxygenation level on yard trimmings composting rate, odor production, and compost quality in bench-scale reactors. Compost Sci Util 6(4):6–14
Mondini C, Fornasier F, Sinicco T (2004) Enzymatic activity as a parameter for the characterization of the composting process. Soil Biol Biochem 36(10):1587–1594
Morel JL (1982) L’évaluation de la maturité des composts urbains par une méthode colorimétrique. Compost Information, 10, 4–8. https://www.researchgate.net/publication/265825882_L%27evaluation_de_la_maturite_des_composts_par_une_ methode_colorimetrie (Accessed 14 February 2017).
Mustin M (1987) Le compost : La gestion de la matière organique. Francois Dubusc, Paris. p. 954
Namkoong WE, Hwang Y, Cheong JG, Choi JY (1999) A comparative evaluation of maturity parameters for food waste composting. Compost Sci Util 7:55–62
Nappi P, Barberis R (1993) Compost as growing medium: chemical, physical and biological aspects. Acta Hortic 342:249–256
Navarro A, Cegarra J, Roig A, Garcia D (1993) Relationships between organic matter and carbon contents of organic wastes. Bioresour Technol 44(3):203–207
N’Dayegamiye A, Goulet M, Laverdière M (1997) Effet à long terme d’apports d’engrais minéraux et de fumier sur les teneurs en C et en N des fractions densimétriques et des agrégats du loam limoneux Le Bras. Can J Soil Sci 77(3):351–358
Nicolardot B, Chaussod R, Morel JL, Guckert A, Benistant D, Catroux G, Germon JC (1986) Appréciation simple de la maturité des composts urbains en relation avec les effets sur la production végétale. Agronomie 6:819–827
Papendick RI and Campbell GS (1981) Theory and measurement of water potential. In: Parr JF, Gardner WR and Elliott LF (eds.) Water Potential Relations in Soil Microbiology, SSSA special publication. Wis. U.S.A. Soil Sci Soc Am J: Madison, 9:1–22
Paré T, Dinel H, Schnitzer M, Dumontet S (1998) Transformations of carbon and nitrogen during composting of animal manure. Biol Fertil Soils 26:173–178
Parr JF, Hornick SB, and Kaufman DD (1994) Use of Microbial Inoculants and Organic Fertilizers in Agricultural Production. Food and Fertilizer Technology Center for Asian and Pacific Region (Publication Database). Extension Bulletin. http://www.fftc.agnet.org/library.php?func=view&style=type&id=20110722114739 (Accessed 14 February 2017).
Pichler M, Kögel-Knabner I (2000) Chemolytic analysis of organic matter during aerobic and anaerobic treatment of minicipal solid waste. J Environ Qual 29:1337–1344
Poincelot RP (1972) Biochemistry and methodology of composting. Connecticut Experiment Station Bull, 727: 1–38. https://archive.org/details/biochemistrymeth00poin (Accessed 17 February 2017).
Provenzano M, Senesi N, Piccone G (1998) Thermal and spectroscopic characterization of composts from municipal solid wastes. Compost Sci Util 6(3):67–73
Razmjoo P, Pourzamani H, Teiri H and Hajizadeh Y (2015) Determination of an empirical formula for organic composition of mature compost produced in Isfahan-Iran composting plant in 2013. Int J Environ Health Eng 4:3. http://www.ijehe.org/text.asp?2015/4/1/3/153988 (Accessed 14 February 2017).
Riffaldi R, Levi-Minzi R, Pera A, de Bertoidi M (1986) Evaluation of compost maturity by means of chemical and microbial analyses. Waste Manag Res 4(1):387–396
Robertson F, Morgan W (1995) Mineralization of C and N in organic materials as affected by duration of composting. Australian Journal of Soil Research 33(3):511
Roletto E, Barberis R, Consiglio M, Jodice R (1985) Chemical parameters for evaluating compost maturity. Biocycle 26:46–47
Roman P, Martinez MM and Pantoja A (2015) Farmer’s Compost Handbook: Experiences in Latin America. FAO Rome. ISBN: 978–92–5-107845-7. http://www.fao.org/3/a-i3388e.pdf (Accessed 06 February 2017).
Rynk, R. 1992. On-farm composting handbook (NRAES-54). Cooperative Extension. Ithaca, N.Y. p. 186
Saharinen M (1998) Evaluation of changes in CEC during composting. Compost Sci Util 6(4):29–37
Sanchez-Monedero M, Roig A, Paredes C, Bernal M (2001) Nitrogen transformation during organic waste composting by the Rutgers system and its effects on pH, EC and maturity of the composting mixtures. Bioresour Technol 78(3):301–308
Saviozzi A, Levi-Minzi R, Riffaldi R (1988) Maturity evaluation of organic wastes. Biocycle 29:54–56
Scaglia B, Tambone F, Genevini P, Adani F (2000) Respiration index determination: dynamic and static approaches. Compost Sci U 8(2):90–98
Schumann G, Soares H, Holden C, Switzenbaum M (1993) Relationship of traditional parameters of compost stability to turfgrass quality. Environ Technol 14(3):257–263
Serra-Wittling C (1995) Valorisation de composts d’ordures ménagères en protection des cultures : Influence de l’apport de composts sur le développement des maladies d’origine tellurique et le comportement de pesticides dans un sol. Dissertation, INA-PG, p. 220
Sharma V, Canditelli M, Fortuna F, Cornacchia G (1997) Processing of urban and agro-industrial residues by aerobic composting: review. Energ Convers Manage 38(5):453–478
Steel H, De La Peña E, Fonderie P, Willekens K, Borgonie G, Bert W (2010) Nematode succession during composting and the potential of the nematode community as an indicator of compost maturity. Pedobiologia (Jena) 53(3):181–190
Strom PF (1985) Effect of temperature on bacterial species diversity in thermophilic solid-waste composting. Appl Environ Microbiol. 50:4 899–905. http://aem.asm.org/content/50/4/899.full.pdf+html (Accessed 17 February 2017).
Sugahara K, Harada Y, Inoko A (1979) Color change of city refuse during composting process. Soil Sci Plant Nutr 25(2):197–208. doi:10.1080/00380768.1979.10433160 (Accessed 17 February 2017
Thomsen M, Lassen P, Dobel S, Hansen P, Carlsen L, Mogensen B (2002) Characterisation of humic materials of different origin: a multivariate approach for quantifying the latent properties of dissolved organic matter. Chemosphere 49(10):1327–1337
Tiquia S, Tam N (1998) Elimination of phytotoxicity during co-composting of spent pig-manure sawdust litter and pig sludge. Bioresour Technol 65(1–2):43–49
Tiquia S, Tam N (2000) Fate of nitrogen during composting of chicken litter. Environ Pollut 110(3):535–541
Tiquia S, Tam N, Hodgkiss I (1997) Effects of turning frequency on composting of spent pig-manure sawdust litter. Bioresour Technol 62(1–2):37–42
Tripetchkul S, Pundee K, Koonsrisuk S, Akeprathumchai S (2012) Co-composting of coir pith and cow manure: initial C/N ratio vs physico-chemical changes. Int J Recycl Org Waste Agricult 1(1):15
Tuomela M (2000) Biodegradation of lignin in a compost environment: a review. Bioresour Technol 72(2):169–183
Vallini G, Pera A, Vadrighi M and Cecchi F (1993) Process constraints in source-collected vegetable waste composting. Water Sci Technol, 28, 2:229–236. https://www.researchgate.net/profile/Giovanni_Vallini/publication/263582162_Process_constraints_in_sourcecollected_ vegetable_waste_composting/links/53e4cd4d0cf25d674e94ee0d.pdf (Accessed 17 February 2017).
Veeken A, Nierop K, Wilde V, Hamelers B (2000) Characterisation of NaOH-extracted humic acids during composting of a biowaste. Bioresour Technol 72(1):33–41
Vobrkov S, Vaverkova M, Adamcova D (2016) Enzyme production during composting of aliphatic–aromatic copolyesters in organic wastes. Environ Eng Sci. doi:10.1089/ees.2015.044
Weppen P (2001) Process calorimetry on composting of municipal organic wastes. Biomass Bioenergy 21(4):289–299
Wichuk K, McCartney D (2013) Compost stability and maturity evaluation—a literature review. J Environ Eng Sci 8(5):601–620
Willson GB (1989) Combining raw materials for composting. BioCycle, 30:82–83. http://infohouse.p2ric.org/ref/33/32518.pdf (Accessed 17 February 2017).
Woodbury P, Breslin V (1992) Assuring compost quality: suggestions for facility managers, regulators, and researchers. Biomass Bioenergy 3(3–4):213–225
Wu L, Ma L, Martinez G (2000) Comparison of methods for evaluating stability and maturity of Biosolids compost. J Environ Qual 29(2):424
Yulipriyanto H (2001) Emission d’effluents gazeux lors du compostage de substrats organiques en relation avec l’activité microbiologique (Nitrification/Dénitrification). Dissertation, University Rennes. https://tel.archivesouvertes. fr/tel-00654701/document (Accessed 17 February 2017).
Author information
Authors and Affiliations
Corresponding author
Ethics declarations
Conflict of interest
The authors declare that they have no conflict of interest.
Rights and permissions
About this article
Cite this article
Azim, K., Soudi, B., Boukhari, S. et al. Composting parameters and compost quality: a literature review. Org. Agr. 8, 141–158 (2018). https://doi.org/10.1007/s13165-017-0180-z
Received:
Accepted:
Published:
Issue Date:
DOI: https://doi.org/10.1007/s13165-017-0180-z