Abstract
Acute hyperglycemia (HG) exacerbates reperfusion injury and aggravates tissue plasminogen activator (tPA)-induced hemorrhagic transformation (HT). Previous experimental hyperglycemic stroke studies employed very high blood glucose levels and exclusively used suture occlusion model to induce ischemia. Only few studies evaluated HG in embolic stroke and mostly involving the use of 10-fold higher dose of tPA than that is used in patients. However, the interaction between acute HG and low (human) dose tPA in different experimental models of stroke has never been reported. We first tested the impact of the severity of acute HG on stroke outcome. Building upon our findings, we then compared the impact of mild acute HG on neurovascular injury in rats subjected to suture or thromboembolic occlusion with and without low dose tPA. We assessed cerebral blood flow, neurobehavioral outcomes, infarction, hemorrhage, and edema. tPA did not change the infarct size in either control or hyperglycemic animals when compared to no tPA groups. HG increased HT and worsened functional outcomes in both suture and embolic occlusion models. The combination of HG and tPA exacerbated the vascular injury and worsened the neurological deficits more than each individual treatment in both models. Our findings show that the interaction between HG and even low dose tPA has detrimental effects on the cerebrovasculature and functional outcomes independent of the method of reperfusion.
Similar content being viewed by others
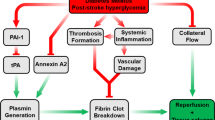
Avoid common mistakes on your manuscript.
Introduction
Almost 40 % of acute ischemic stroke (AIS) patients present with admission hyperglycemia (HG), an independent predictor of poor outcomes in AIS [1, 2]. Restoration of cerebral blood flow (CBF) to the ischemic brain is paramount for the salvation of the ischemic penumbra. However, reperfusion itself can induce brain injury through increased oxidative stress and inflammation. Moreover, HG exacerbates the reperfusion injury [3–6]. As recently reviewed, experimental models using various methods to induce hyperglycemia in different species recapitulated the clinical findings and showed that HG increases infarct size, brain swelling, hemorrhagic transformation (HT), blood–brain barrier (BBB) disruption, and neurological deficits [7–12]. However, most of these preclinical studies employed very high blood glucose levels and used a mix of diabetes and acute HG models. Given that patients that have HG with no prior history of diabetes suffer the least favorable clinical outcomes, in a recent study we compared the neurovascular injury after ischemic stroke in acute hyperglycemia and diabetes models. We reported that even a mild and acute elevation in blood glucose increases vascular injury and worsens functional outcome [9]. Since these past studies, including ours, almost exclusively used a suture occlusion model to induce stroke, which promptly restores CBF to the ischemic area, a direct comparison of the impact of the method of reperfusion on acute hyperglycemic stroke injury and outcome remained to be determined.
Tissue plasminogen activator (tPA) is the only FDA-approved therapy for AIS patients. Yet, its use in the clinical setting is limited due to its narrow therapeutic window and increased risk of cerebral bleeding, which is more profound under hyperglycemic conditions [13–15]. In spite of the importance of tPA in the clinical setting, only a handful of studies involved tPA in experimental hyperglycemic models. Moreover, due to the differences in human and rodent fibrinolytic system, current embolic stroke models use 10 times greater dose of tPA than that is used in patients [7, 16–20]. Thus, in this translational study using suture and thromboembolic occlusion of middle cerebral artery (MCA), with and without human dose tPA in the hyperglycemic setting, we tested the hypothesis that even acute mild HG worsens the neurovascular injury and stroke outcomes irrespective of the method of reperfusion and that the use of tPA amplifies this injury.
Materials and Methods
Animal Models
The animals were housed at the Georgia Regents University animal care facility, which is approved by the American Association for Accreditation of Laboratory Animal Care. This study was conducted in accordance with the National Institute of Health guidelines for the care and use of animals in research, and all protocols were approved by the institutional animal care and use committee.
Study 1
To determine the effect of the severity of hyperglycemia, male Wistar rats (Harlan Laboratories Inc., Indianapolis, IN) were assigned to three different groups: (1) control/normoglycemic (NG) (90–120 mg/dl), (2) mild HG (BG 140–200 mg/dl), and (3) severe HG (BG 240–350 mg/dl). Animals from all groups were subjected to 90 min MCA occlusion (MCAO) by the suture occlusion method and up to 24 h reperfusion. Thirty percent and 60 % glucose solutions were used to achieve blood glucose levels of 140–200 and 240–350 mg/dl, respectively. Acute HG was achieved by 2 ml intraperitoneal (IP) injection of glucose solution 15 min before MCAO. A second dose was given just after the stroke surgery to maintain HG through 90 min of ischemia. Blood glucose was measured from the tail vein using a glucometer (Freestyle, Alameda, CA). Plasma osmolality was measured using advanced micro-osmometer, model 3300 (Advanced instruments, INC., Norwood, MA).
Study 2
To determine the effect of tPA and method of reperfusion in hyperglycemic stroke, control (NG) or mild HG (140–200 mg/dl) male Wistar rats were subjected to either suture or thromboembolic occlusion of the MCA. Animals were randomized to receive either tPA (Cathflo Activase (Alteplase), Genentech) or vehicle (water for injection) of equal volume. tPA (1 mg/kg) was intravenously infused over 20 min through the jugular vein 2 h after induction of ischemia to rats with either suture or thromboembolic occlusion.
Preparation of Blood Clots
The method of clot preparation is the same as we previously reported [21, 22] but with minor modifications for rats to further increase the stability and strength of the freshly prepared blood clots. Briefly, arterial blood was withdrawn from a donor rat in a syringe prefilled with human fibrinogen (10 mg/ml). The blood was immediately pushed into 20 cm polyethylene (PE)-50 tubes. The tubes were kept for 4–6 h at room temperature and then at 4 °C for 24 h. The PE-50 tubes containing the clots were cut into 5 cm long pieces and the formed clots were pushed out into a petri dish containing sterile water for injection and allowed to wash for 15 min. Blood clots were then transferred to a petri dish containing PBS and left for up to 6 h for retraction at room temperature and then washed with PE-10 tubes. A single piece (3–4 cm long) of clot was withdrawn in a PE-10 catheter and used to induce MCAO.
Stroke Surgery
Focal cerebral ischemia was performed as previously described [23]. Briefly, 90 min MCAO was performed under 2 % isoflurane anesthesia followed by up to 24 h reperfusion. A midline cervical incision was made to expose the common carotid artery. The external carotid artery (ECA) was separated, ligated, and cauterized. An arteriotomy was performed on the ECA stump. A rounded-tip 3-0 monofilament nylon suture (prepared carefully under microscope with high magnification power to ensure uniformity) was inserted into the ECA stump and advanced through the internal carotid artery to occlude the origin of MCA. The occlusion suture was secured with one-silk suture at the stump of ECA, and the incision was closed. After 90 min, animals were re-anesthetized, and the occlusion suture was removed to allow reperfusion. For the embolic model, the surgical procedure remained the same as in suture model except that instead of the monofilament suture, a PE-10 catheter containing the clot was inserted through the ECA stump to deliver the clot to the origin of the MCA and produce occlusion. The clot was gently injected with 100 μl sterile saline. Scanning laser Doppler (Pim-3, Perimed, ST) was used to confirm a similar degree of drop in CBF among groups. The percent drop in CBF after stroke was determined by comparing to baseline [24]. To monitor the ability of tPA to resolve the blood clots and restore blood flow, CBF was measured 30, 60, and 120 min after tPA administration in animals subjected to thromboembolic occlusion. Animals with reduction in CBF less than 40 % or more than 75 % from baseline are excluded.
Evaluation of Infarct Size, Edema, HT, and Hemoglobin Content
At 24 h, and just before sacrifice, animals were put into deep sleep using isoflurane and subjected to intracardiac perfusion with ice cold PBS to flush out blood from cerebral vessels. Brains were isolated and sliced into seven coronal sections marked A to G (+6.7 to −8.8 mm from the bregma). The infarct size was measured after staining the brain slices with 2,3,5-triphenyl tetrazolium chloride (TTC) as previously described [23]. Edema was calculated as a percent (%) increase in the ischemic hemisphere vs. the contralateral hemisphere. The stained brain sections are then separated into ipsilateral and contralateral hemispheres, snap frozen, and kept at −80 °C for biochemical assays. Following brain homogenization, hemoglobin (Hb) content was measured with Quanti-Chrom kit (BioAssay Systems, Hayward, CA), 2007) and reported as excess hemoglobin (mg/g protein) in the ischemic hemisphere normalized to sham animals. A blinded investigator scored macroscopic HT in brain slices B to E using a four-point rubric (0—no hemorrhage; 1—dispersed individual petechiae; 2—confluent petechiae; 3—small diffuse hemorrhage or hematoma; 4—large diffuse hemorrhage or hematoma) and the total score for each animal was reported [25].
Evaluation of Neurobehavioral and Functional Outcomes
Neurobehavioral tests were assessed, recorded, and scored in a blinded fashion as previously described [26]. Briefly, animals were handled for 5–7 days prior to behavior testing in rooms where behavior testing is to be carried out. Neurobehavioral evaluation involved Bederson’s score, beam walk, and grip strength tests. Behavior testing was performed before stroke and at 24 h reperfusion, just before sacrifice. Bederson’s score for each rat was obtained by using three parameters which include (a) observation of no circling scored as 2, partial circling scored as 1, continuous circling scored as 0; (b) hindlimb retraction; and (c) forelimb flexion scored as 1 or 0 according to animal’s ability to immediately replace the limb upon pulling or not, respectively. The resistance to push is also measured and scored as 1 or 0, depending on whether the animal is able to resist pushing or not. Maximum score of 7 is given to a normal rat. Beam walking ability is graded based on a seven-point scale method previously described [25]. Total composite neurological score out of 14 composed of sum of the Bederson’s score and the scores obtained from the beam walking test. Forelimb grip strength was measured with a standard grip strength meter (Columbus Instrument, Columbus, OH).
Data Analysis
Data are presented as mean ± SD. Data were examined for outlying observations and nonparametric analyses were used when needed. Area under the curve was determined for blood glucose from baseline to 24 h post-ischemia and for CBF as a percent of baseline using NCSS 2007 (NCSS, LLC, Kaysville, UT). The rCBF values post-reperfusion were compared to pre-reperfusion values using a paired t test. The effects of mild and severe hyperglycemia were determined using a one-way ANOVA with three groups (control, mild HG, and severe HG). An exact Kruskal-Wallis test was used to analyze HT due to outlying values in the HG groups. The effect of hyperglycemia and tPA treatment was assessed using a 2 HG (no vs. yes) by 2 tPA (no vs. yes) ANOVA where a significant interaction would indicate a differential effect of tPA on stroke outcomes dependent on HG status. SAS© 9.3 (SAS, Inc., Cary, NC) was used for all analyses. Statistical significance was determined at alpha < 0.05 and a Tukey’s post-hoc test was used to compare means from significant ANOVAs.
Results
The Impact of the Severity of Hyperglycemia on Neurovascular Injury and Functional Outcomes
Administration of 30 and 60 % glucose solutions achieved graded blood glucose levels of 140–200 and 240–350 mg/dl (Fig. 1a), respectively. Plasma osmolality was 309 ± 3.2, 310 ± 1.9, and 313 ± 2 mosmol for the control, mild HG, and severe HG groups, respectively. There was no significant difference in plasma osmolality between groups. The mortality was 10–15 % in each group. The drop in CBF after occlusion was almost the same among the different animal groups (Fig. 1b). Mild elevation in blood glucose (140–200 mg/dl) did not increase the infarct size when compared to control animals. However, severe HG (240–350 mg/dl) caused a significant increase in the infarct size (Fig. 1c).
a The hyperglycemia model. Administration of 30 and 60 % glucose solutions successfully achieved blood glucose levels of 140–200 mg/dl (mild HG) and 240–350 mg/dl (severe HG), respectively. Acute HG was maintained through 90 min of ischemia. b The percent drop in cerebral blood flow from baseline was almost the same among the different animal groups. c Mild HG did not affect the infarct size; however, severe HG significantly increased the infarct size when compared to control and mild HG animals. N = 5–7/group. *p < 0.05 vs. control, #p < 0.05 vs. mild HG
Edema in the brain, HT, and Hb content were used to assess the vascular injury. Although mild elevation in BG did not increase the infarct size, it significantly increased bleeding in the brain when compared to control animals. All levels of HG, whether mild or severe, significantly increased the edema in the brain, macroscopic HT, and Hb content in the ischemic hemispheres (Fig. 2a–c).
All levels of HG whether mild or severe significantly exacerbated the cerebrovascular injury in the brain. a Representative images of HT patterns are shown on top. HT index, shown at the bottom as a measure of macroscopic HT occurrence, demonstrates increased HT in hyperglycemic animals. b HG significantly increased Hb content in the ischemic hemisphere which is used as a measure of the severity of bleeding. c HG also increased edema in the brain. *p < 0.05 vs. control
Mild and severe levels of acute HG significantly reduced the grip strength and exacerbated the neurological deficits when compared to control normoglycemic animals (Fig. 3a, b).
Mild HG significantly exacerbated the neurological deficits and worsened the functional outcomes as indicated by a composite score and b grip strength. Severe HG caused even worse deterioration compared to mild HG. Lower composite score indicates greater neurological deficits. *p < 0.05 vs. control, #p < 0.05 vs. mild HG
The Impact of the Combination of Acute HG and tPA in Suture and Thromboembolic Models of Hyperglycemic Reperfusion on Neurovascular Injury and Functional Outcomes
Since mild HG exacerbated HT and edema and worsened functional outcomes, the next set of studies used this level of HG to assess the impact of tPA and the method of reperfusion. Administration of tPA did not affect blood glucose levels in different stroke models (data not shown). There was no difference in percent drop in CBF measured immediately either before reperfusion in the suture group or before tPA administration in the embolic group (Fig. 4a, b). In the thromboembolic model, laser Doppler monitoring showed that the first remarkable reperfusion was 30 to 60 min after tPA administration and achieved only 40 to 60 % increase in CBF and this level persisted after tPA reperfusion up till sacrifice (Fig. 4b).
a The percent drop in CBF at 90 min occlusion (just before reperfusion) is almost the same among the different animal groups subjected to suture occlusion. b The drop in CBF at 2 h in rats subjected to thromboembolic occlusion was almost the same among tPA treated and non-treated groups. The use of low dose tPA (1 mg/kg) was able to slightly and gradually restore the blood flow through a period of 2 h. *p < 0.05 vs. pre-tPA and vehicle
tPA treatment did not affect the infarct size in either normoglycemic or hyperglycemic animals when compared to their non-treated counterparts, whether these animals were subjected to suture or embolic occlusion (Fig. 5a, b). The administration of tPA slightly but not significantly increased the HT in control animals. However, the combination of HG with tPA significantly exacerbated the cerebrovascular injury and HT in the brain in both suture and embolic occlusion models (Fig. 6a, b). The interaction between tPA and HG led to a synergistic effect in exacerbating HT in animals subjected to embolic stroke (interaction p value = 0.029, Fig. 6b). The intracerebral bleeding was further confirmed by assessing the Hb content in the ischemic hemispheres. tPA administration increased the Hb content in the ischemic hemispheres in both normoglycemic and hyperglycemic animals irrespective of the method of reperfusion (Fig. 6c, d). tPA administration also increased edema in both suture and thromboembolic occlusion animal models (Fig. 6e, f).
HG and tPA augment vascular injury. a Mild acute HG significantly increased the macroscopic HT in animals subjected to suture occlusion compared to control. b In animals with embolic stroke, mild HG showed a trend (p value = 0.075 vs. control) but not a significant increase in macroscopic HT when compared to control. The combination of HG and tPA exacerbated the injury and showed a significant increase in HT more than each alone in both reperfusion models. The interaction between HG and tPA potentiated the HT in animals subjected to embolic stroke (λp = 0.029, interaction between HG and tPA, b). Mild HG and tPA individually have significantly increased the Hb content in the ischemic hemispheres compared to control animals in both c suture and d embolic occlusion models. The combination of HG and tPA worsened the bleeding and significantly increased the Hb content when compared to each of them alone, independent of the model of reperfusion. Mild HG significantly increased edema in the brains of animals subjected to either e suture or f embolic occlusion. The combination of HG and tPA significantly increased edema when compared to each alone in animals with embolic (f) but not suture occlusion (e). *p < 0.05 vs. groups receiving vehicle (no tPA) either control or HG, #p < 0.05 vs. control (NG) receiving vehicle (no HG, no tPA), ¥p < 0.05 vs. control treated with tPA alone (no HG). In b, λp < 0.05, and lambda indicates the significant interaction between HG and tPA that significantly potentiated the HT in rats subjected to embolic stroke
tPA significantly reduced the grip strength and neurological scores resulting in worse functional outcomes in normoglycemic and hyperglycemic animals compared to untreated animals in both models of reperfusion. However, the combination of tPA and HG significantly exacerbated the neurological deficits compared to each alone (Fig. 7a–d). The neurological deterioration showed a similar pattern in both suture and embolic occlusion models (Fig. 7a–d).
HG and tPA worsen functional outcomes. Both HG and tPA significantly worsened the neurological deficits and reduced the grip strength in suture (a, c) and embolic (b, d) occlusion models. The combination of HG and tPA further exacerbated the injury and worsened the outcome more than each alone in both models of reperfusion. *p < 0.05 vs. groups receiving vehicle (no tPA) either control or HG, #p < 0.05 vs. control receiving vehicle (no HG, no tPA), ¥p < 0.05 vs. control treated with tPA alone (no HG)
Discussion
One goal of this preclinical study was to bridge the critical gap between clinical observations and experimental studies in acute hyperglycemic stroke with regard to blood glucose levels and use of tPA. Accordingly, this study was designed to address the following points: (1) the impact of the severity of acute HG on stroke outcomes using clinically relevant blood glucose levels; (2) the impact of the interaction between acute HG and tPA on neurovascular injury and functional outcomes in different experimental stroke models that mimic the clinical scenario; and (3) establishing a novel approach for studying the hyperglycemia reperfusion injury in an embolic stroke model with human tPA dose.
Clinically, HG is an independent predictor of poor functional outcomes for AIS patients. The TOAST trial showed that higher admission blood glucose was associated with worse clinical outcomes in patients with non-lacunar stroke [27]. Admission HG was shown to be an independent predictor of poor neurological outcomes and increased rate of symptomatic intracranial hemorrhage (ICH) in the NINDS and ATLANTIS trials [28, 29]. The ECASS II trial also showed that HG persisting for 24 h or more is an independent predictor of poor clinical outcomes, ICH, and death in AIS patients [30, 31]. Moreover, HG aggravates tPA-induced cerebral hemorrhage in AIS patients [14, 15, 29]. Accordingly, several clinical trials were conducted to examine the safety, feasibility, and efficacy of lowering blood glucose for hyperglycemic AIS patients. The Treatment of HG in Ischemic Stroke (THIS) and the Glucose Regulation in Stroke Patients (GRASP) trials showed that, in these cohorts, blood glucose levels range between 160 and 260 mg/dl at admission and they targeted blood glucose levels of less than 130 and 110 mg/dl, respectively [32, 33]. Based on the results of these feasibility studies, the Stroke Hyperglycemia Insulin Network Effort (SHINE) trial is currently recruiting patients to examine the efficacy and safety of blood glucose lowering in AIS patients targeting blood glucose of 80–130 mg/dl [34]. However, evidence from clinical trials including The Normoglycemia in Intensive Care Evaluation-Survival Using Glucose Algorithm Regulation (NICE-SUGAR) and the Action to Control Cardiovascular Risk in Diabetes (ACCORD) trials showed that intensive blood glucose lowering was associated with worse outcome and increased mortality [35, 36]. The incidence of hypoglycemia (<60 mg/dl) was the major cause of adverse events and increased mortality. Blood glucose target <180 mg/dl resulted in less mortality than did a target of 80–108 mg/dl [35]. The UK Glucose Insulin in Stroke Trial (GIST-UK) showed that tight glycemic control did neither improve the mortality nor the neurologic impairment at 90 days in hyperglycemic AIS patients but the study was terminated early due to slow recruitment [37]. These past studies demonstrate the challenges in the field and emphasizes the importance of the cautious and conservative control of blood glucose in AIS patients.
While past preclinical studies provided very valuable information on the impact and mechanisms by which HG worsens stroke outcomes, they used hyperglycemia models with much higher blood glucose levels (350–500 mg/dl) than those observed in patients [7, 8, 19, 38]. To address this gap, in the current study we used graded blood glucose levels that mimic the clinical scenario. In this study, we found that mild elevation in blood glucose increased edema and HT without increasing the infarct size. This reflects the sensitivity and the vulnerability of the vasculature to acute yet subtle changes in blood glucose and also reflects the role the vasculature plays in contributing to worse functional outcomes when there is no further neuronal injury.
Our findings provide evidence that the severity of acute HG is a determinant of functional outcome. Severe HG significantly increased the infarct size compared to control and mild HG groups, and this was associated with worse functional outcomes. The major cause of this damage may be attributed to the increase in generation of reactive oxygen species (superoxide and peroxynitrite) and inflammatory cytokines (TNF alpha and interleukins) [1, 4, 6, 8, 39, 40]. Another possibility may be increased osmolality. Bhardwaj et al. showed that continuous infusion of hypertonic saline for 22 h increased plasma osmolality and this was associated with an increase in the infarct volume after transient focal ischemia in rats [41]. However, we did not observe a significant difference in plasma osmolality in the current study which may be due to the small bolus dose of glucose solution that we used to induce acute HG.
As recently reviewed, almost all the previous experimental studies in acute hyperglycemic stroke research used the suture occlusion model to induce stroke [7]. However, this model allows abrupt reperfusion and prompt restoration of CBF to the ischemic tissue, which may exacerbate the damage. Moreover, it does not mimic what happens in ischemic stroke patients where the ischemia is due to occlusion of cerebral vessels by blood clots, which further increases risk of microemboli formation and entrapment. In this translational study, we used both suture and thromboembolic occlusion models to recapitulate the clinical condition. Our findings show that the infarct size is greater in the embolic model (most likely due to longer occlusion). However, both models cause a similar degree of HT and neurological deficit in acute HG, in spite of the shorter occlusion time achieved with the suture model. This is most probably due to the prompt reperfusion that takes place in the suture model and flushes the ischemic tissue with a massive and sudden flow of reactive oxygen species and inflammatory cytokines. We recently reported that embolic stroke causes less HT in a diabetic model as compared to suture occlusion and suggested that gradual reperfusion that occurs in embolic stroke may have lessened the injury [22]. Current results raise the possibility that under diabetic conditions compensatory changes may limit the vascular injury.
Although the interaction between HG and tPA has been shown to be detrimental to AIS patients, very few experimental studies were conducted to study this. The limited experimental studies with the embolic model used streptozotocin-induced diabetes, not acute hyperglycemia, with very high blood glucose levels. Based on early studies which reported differences in rat’s fibrinolytic system [42], these studies used a 10-fold higher dose of tPA (10 mg/kg) than that what is used in ischemic stroke patients and reported increased intracerebral hemorrhage [16–19]. Recently, several groups used low dose of tPA in combination with other drugs in order to improve the outcomes and at the same time avoid the adverse effects of high dose tPA. The combination of low dose tPA (2.5 mg/kg) with annexin A2 (5 mg/kg) in rats subjected to embolic stroke significantly improved CBF, reduced brain infarction and HT [43]. A higher dosage regimen of 5 mg/kg tPA/10 mg/kg annexin A2 was able to improve the long-term neurological outcomes 1 month post-stroke [44]. These studies were conducted in normal animals. In the current study, we used a low dose of tPA (1 mg/kg), which is almost the human dose. We hypothesized that tPA treatment will reduce infarct and improve outcomes in the control (NG) animals and expected greater HT and neurological deficits in the HG group. However, we did not see a significant difference in infarct size in animals receiving tPA as compared to vehicle group. This is most probably because the tPA-induced reperfusion was not sufficient to limit the infarct expansion even in the control group. Prolonged monitoring of CBF demonstrated that the low dose tPA given at 2 h post-occlusion was not able to fully restore CBF which may have contributed to this finding. Past studies reported lower [45] or similar infarct volumes [46–48] as we observed in this study. In the current study, tPA increased bleeding and worsened functional outcome. Lapergue et al. also reported increased HT after tPA treatment in an embolic model but they did not report any functional outcomes [47]. Several other studies showed increased BBB permeability and there was no effect of tPA on functional outcomes as compared to vehicle-treated groups [46, 49]. While we do not have a full explanation of poor outcomes in the control group treated with tPA, there is a significant increase in bleeding which may be responsible for poor outcomes. We also measured behavioral outcomes at 24 h while injury is still evolving. Late time points may be needed to address this concern.
The rationale to use tPA in the suture model was to assess the effects of tPA on neuronal injury and HT irrespective of its recanalization capability. Our results show that infarct size is similar between vehicle- and tPA-treated animals suggesting that “low dose” tPA does not have additional effects on cell death (core formation) as previously reported [45, 47]. These past studies either did not report HT and functional outcomes or reported HT without functional outcomes. In the current study, we show that tPA increased HT and worsened the outcome emphasizing the role of vascular injury on functional outcomes. There are also studies which showed reduction in infarct size with tPA. Berny-Lang et al. showed that tPA (2.5 mg/kg) given early during MCAO (15 min after inducing ischemia) reduced infarct size in mice but not rats with suture occlusion. However, they did not report the infarct size when they gave tPA 2 h after reperfusion [50]. Kilic et al. also reported reduction in ischemic injury in mice when treated with tPA 10 mg/kg during early MCAO [51]. These differences in outcomes may be due to the difference in dose and timing of tPA treatment.
Conclusion and Limitations
Up to date, tPA remains to be the only therapeutic option for AIS patients. Given that almost 50 % of stroke patients present with HG, which aggravates the tPA-induced cerebral hemorrhage, there is a pressing need for more preclinical studies in which important clinical questions can be addressed. For example, clinical studies suggest that women benefit more from tPA therapy as compared to men [52]. While the use of only healthy, male young animals and assessment of only short-term outcomes remain to be limitations of the current study, to the best of our knowledge, this is the first study investigating the interaction between tPA and acute HG in a clinically relevant model of embolic stroke. This translational study is also the first to compare the effects of acute HG in different models of reperfusion injury.
References
Kruyt ND, Biessels GJ, Devries JH, Roos YB. Hyperglycemia in acute ischemic stroke: pathophysiology and clinical management. Nat Rev Neurol. 2010;6(3):145–55.
Capes SE, Hunt D, Malmberg K, Pathak P, Gerstein HC. Stress hyperglycemia and prognosis of stroke in nondiabetic and diabetic patients: a systematic overview. Stroke. 2001;32(10):2426–32.
Quast MJ, Wei J, Huang NC, Brunder DG, Sell SL, Gonzalez JM, et al. Perfusion deficit parallels exacerbation of cerebral ischemia/reperfusion injury in hyperglycemic rats. J Cereb Blood Flow Metab. 1997;17(5):553–9.
Yip PK, He YY, Hsu CY, Garg N, Marangos P, Hogan EL. Effect of plasma glucose on infarct size in focal cerebral ischemia-reperfusion. Neurology. 1991;41(6):899–905.
Suh SW, Shin BS, Ma H, Van Hoecke M, Brennan AM, Yenari MA, et al. Glucose and NADPH oxidase drive neuronal superoxide formation in stroke. Ann Neurol. 2008;64(6):654–63.
Wei J, Huang NC, Quast MJ. Hydroxyl radical formation in hyperglycemic rats during middle cerebral artery occlusion/reperfusion. Free Radic Biol Med. 1997;23(7):986–95.
Hafez S, Coucha M, Bruno A, Fagan SC, Ergul A. Hyperglycemia, acute ischemic stroke, and throhrombolytic therapy. Transl Stroke Res. 2014;5(4):442–53.
Kamada H, Yu F, Nito C, Chan PH. Influence of hyperglycemia on oxidative stress and matrix metalloproteinase-9 activation after focal cerebral ischemia/reperfusion in rats: relation to blood-brain barrier dysfunction. Stroke. 2007;38(3):1044–9.
Elgebaly MM, Ogbi S, Li W, Mezzetti EM, Prakash R, Johnson MH, et al. Neurovascular injury in acute hyperglycemia and diabetes: a comparative analysis in experimental stroke. Transl Stroke Res. 2011;2(3):391–8.
Cipolla MJ, Huang Q, Sweet JG. Inhibition of protein kinase Cbeta reverses increased blood-brain barrier permeability during hyperglycemic stroke and prevents edema formation in vivo. Stroke. 2011;42(11):3252–7.
Xing Y, Hua Y, Keep RF, Xi G. Effects of deferoxamine on brain injury after transient focal cerebral ischemia in rats with hyperglycemia. Brain Res. 2009;1291:113–21.
Kumari R, Willing LB, Patel SD, Krady JK, Zavadoski WJ, Gibbs EM, et al. The PPAR-gamma agonist, darglitazone, restores acute inflammatory responses to cerebral hypoxia-ischemia in the diabetic ob/ob mouse. J Cereb Blood Flow Metab. 2010;30(2):352–60.
Lees KR, Bluhmki E, von Kummer R, Brott TG, Toni D, Grotta JC, et al. Time to treatment with intravenous alteplase and outcome in stroke: an updated pooled analysis of ECASS, ATLANTIS, NINDS, and EPITHET trials. Lancet. 2010;375(9727):1695–703.
Poppe AY, Majumdar SR, Jeerakathil T, Ghali W, Buchan AM, Hill MD. Admission hyperglycemia predicts a worse outcome in stroke patients treated with intravenous thrombolysis. Diabetes Care. 2009;32(4):617–22.
Alvarez-Sabin J, Molina CA, Montaner J, Arenillas JF, Huertas R, Ribo M, et al. Effects of admission hyperglycemia on stroke outcome in reperfused tissue plasminogen activator-treated patients. Stroke. 2003;34(5):1235–41.
Ning R, Chopp M, Yan T, Zacharek A, Zhang C, Roberts C, et al. Tissue plasminogen activator treatment of stroke in type-1 diabetes rats. Neuroscience. 2012;222:326–32.
Fan X, Lo EH, Wang X. Effects of minocycline plus tissue plasminogen activator combination therapy after focal embolic stroke in type 1 diabetic rats. Stroke. 2013;44(3):745–52.
Fan X, Ning M, Lo EH, Wang X. Early insulin glycemic control combined with tPA thrombolysis reduces acute brain tissue damages in a focal embolic stroke model of diabetic rats. Stroke. 2013;44(1):255–9.
Fan X, Qiu J, Yu Z, Dai H, Singhal AB, Lo EH, et al. A rat model of studying tissue-type plasminogen activator thrombolysis in ischemic stroke with diabetes. Stroke. 2012;43(2):567–70.
Won SJ, Tang XN, Suh SW, Yenari MA, Swanson RA. Hyperglycemia promotes tissue plasminogen activator-induced hemorrhage by increasing superoxide production. Ann Neurol. 2011;70(4):583–90.
Hoda MN, Li W, Ahmad A, Ogbi S, Zemskova MA, Johnson MH, et al. Sex-independent neuroprotection with minocycline after experimental thromboembolic stroke. Exp Transl Stroke Med. 2011;3(1):16.
Li W, Qu Z, Prakash R, Chung C, Ma H, Hoda MN, et al. Comparative analysis of the neurovascular injury and functional outcomes in experimental stroke models in diabetic Goto-Kakizaki rats. Brain Res. 2013;1541:106–14.
Ergul A, Elgebaly MM, Middlemore ML, Li W, Elewa H, Switzer JA, et al. Increased hemorrhagic transformation and altered infarct size and localization after experimental stroke in a rat model type 2 diabetes. BMC Neurol. 2007;7:33.
Guan W, Kozak A, El-Remessy A, Johnson M, Pillai B, Fagan S. Acute treatment with candesartan reduces early injury after permanent middle cerebral artery occlusion. Transl Stroke Res. 2011;2(2):179–85.
Kelly-Cobbs AI, Prakash R, Li W, Pillai B, Hafez S, Coucha M, et al. Targets of vascular protection in acute ischemic stroke differ in type 2 diabetes. Am J Physiol Heart Circ Physiol. 2013;304(6):H806–15.
Prakash R, Li W, Qu Z, Johnson MA, Fagan SC, Ergul A. Vascularization pattern after ischemic stroke is different in control versus diabetic rats: relevance to stroke recovery. Stroke. 2013;44(10):2875–82.
Bruno A, Biller J, Adams Jr HP, Clarke WR, Woolson RF, Williams LS, et al. Acute blood glucose level and outcome from ischemic stroke. Trial of ORG 10172 in Acute Stroke Treatment (TOAST) Investigators. Neurology. 1999;52(2):280–4.
Clark WM, Albers GW, Madden KP, Hamilton S. The rtPA (alteplase) 0- to 6-hour acute stroke trial, part A (A0276g): results of a double-blind, placebo-controlled, multicenter study. Thromblytic therapy in acute ischemic stroke study investigators. Stroke. 2000;31(4):811–6.
Bruno A, Levine SR, Frankel MR, Brott TG, Lin Y, Tilley BC, et al. Admission glucose level and clinical outcomes in the NINDS rt-PA Stroke Trial. Neurology. 2002;59(5):669–74.
Yong M, Kaste M. Dynamic of hyperglycemia as a predictor of stroke outcome in the ECASS-II trial. Stroke. 2008;39(10):2749–55.
Piironen K, Putaala J, Rosso C, Samson Y. Glucose and acute stroke: evidence for an interlude. Stroke. 2012;43(3):898–902.
Bruno A, Kent TA, Coull BM, Shankar RR, Saha C, Becker KJ, et al. Treatment of hyperglycemia in ischemic stroke (THIS): a randomized pilot trial. Stroke. 2008;39(2):384–9.
Johnston KC, Hall CE, Kissela BM, Bleck TP, Conaway MR. Glucose regulation in acute stroke patients (GRASP) trial: a randomized pilot trial. Stroke. 2009;40(12):3804–9.
Bruno A, Durkalski VL, Hall CE, Juneja R, Barsan WG, Janis S, et al. The stroke hyperglycemia insulin network effort (SHINE) trial protocol: a randomized, blinded, efficacy trial of standard vs. intensive hyperglycemia management in acute stroke. Int J. Stroke. 2014;9(2):246–51.
Finfer S, Chittock DR, Su SY, Blair D, Foster D, Dhingra V, et al. Intensive versus conventional glucose control in critically ill patients. N Engl J Med. 2009;360(13):1283–97.
Gerstein HC, Miller ME, Byington RP, Goff Jr DC, Bigger JT, Buse JB, et al. Effects of intensive glucose lowering in type 2 diabetes. N Engl J Med. 2008;358(24):2545–59.
Gray CS, Hildreth AJ, Sandercock PA, O'Connell JE, Johnston DE, Cartlidge NE, et al. Glucose-potassium-insulin infusions in the management of post-stroke hyperglycaemia: the UK Glucose Insulin in Stroke Trial (GIST-UK). Lancet Neurol. 2007;6(5):397–406.
Siemkowicz E, Hansen AJ, Gjedde A. Hyperglycemic ischemia of rat brain: the effect of post-ischemic insulin on metabolic rate. Brain Res. 1982;243(2):386–90.
Bemeur C, Ste-Marie L, Montgomery J. Increased oxidative stress during hyperglycemic cerebral ischemia. Neurochem Int. 2007;50(7–8):890–904.
Ste-Marie L, Hazell AS, Bemeur C, Butterworth R, Montgomery J. Immunohistochemical detection of inducible nitric oxide synthase, nitrotyrosine and manganese superoxide dismutase following hyperglycemic focal cerebral ischemia. Brain Res. 2001;918(1–2):10–9.
Bhardwaj A, Harukuni I, Murphy SJ, Alkayed NJ, Crain BJ, Koehler RC, et al. Hypertonic saline worsens infarct volume after transient focal ischemia in rats. Stroke. 2000;31(7):1694–701.
Korninger C, Collen D. Studies on the specific fibrinolytic effect of human extrinsic (tissue-type) plasminogen activator in human blood and in various animal species in vitro. Thromb Haemost. 1981;46(2):561–5.
Zhu H, Fan X, Yu Z, Liu J, Murata Y, Lu J, et al. Annexin A2 combined with low-dose tPA improves thrombolytic therapy in a rat model of focal embolic stroke. J Cereb Blood Flow Metab. 2010;30(6):1137–46.
Wang X, Fan X, Yu Z, Liao Z, Zhao J, Mandeville E, et al. Effects of tissue plasminogen activator and annexin A2 combination therapy on long-term neurological outcomes of rat focal embolic stroke. Stroke. 2014;45(2):619–22.
Meng W, Wang X, Asahi M, Kano T, Asahi K, Ackerman RH, et al. Effects of tissue type plasminogen activator in embolic versus mechanical models of focal cerebral ischemia in rats. J Cereb Blood Flow Metab. 1999;19(12):1316–21.
Zhang L, Chopp M, Teng H, Ding G, Jiang Q, Yang XP, et al. Combination treatment with N-acetyl-seryl-aspartyl-lysyl-proline and tissue plasminogen activator provides potent neuroprotection in rats after stroke. Stroke. 2014;45(4):1108–14.
Lapergue B, Dang BQ, Desilles JP, Ortiz-Munoz G, Delbosc S, Loyau S, et al. High-density lipoprotein-based therapy reduces the hemorrhagic complications associated with tissue plasminogen activator treatment in experimental stroke. Stroke. 2013;44(3):699–707.
Shehadah A, Chen J, Cui Y, Zhang L, Roberts C, Lu M, et al. Combination treatment with low-dose niaspan and tissue plasminogen activator provides neuroprotection after embolic stroke in rats. J Neurol Sci. 2011;309(1–2):96–101.
Zhang L, Zhang ZG, Buller B, Jiang J, Jiang Y, Zhao D, et al. Combination treatment with velcade and low-dose tissue plasminogen activator provides potent neuroprotection in aged rats after embolic focal ischemia. Stroke. 2010;41(5):1001–7.
Berny-Lang MA, Hurst S, Tucker EI, Pelc LA, Wang RK, Hurn PD, et al. Thrombin mutant W215A/E217A treatment improves neurological outcome and reduces cerebral infarct size in a mouse model of ischemic stroke. Stroke. 2011;42(6):1736–41.
Kilic E, Hermann DM, Hossmann KA. Recombinant tissue plasminogen activator reduces infarct size after reversible thread occlusion of middle cerebral artery in mice. Neuroreport. 1999;10(1):107–11.
Kent DM, Price LL, Ringleb P, Hill MD, Selker HP. Sex-based differences in response to recombinant tissue plasminogen activator in acute ischemic stroke: a pooled analysis of randomized clinical trials. Stroke. 2005;36(1):62–5.
Acknowledgments
Adviye Ergul is a Research Career Scientist at the Charlie Norwood Veterans Affairs Medical Center in Augusta, GA. This work was supported in part by VA Merit Award (BX000347), VA Research Career Scientists Award, and NIH (R01NS083559) to Adviye Ergul; VA Merit Award (BX000891) and NIH award (NS063965) to Susan C. Fagan; and American Heart Association Predoctoral Fellowship (13PRE17090026) to Sherif Hafez. The contents do not represent the views of the Department of Veterans Affairs or the United States Government.
Compliance with Ethics Requirements
All institutional and national guidelines for the care and use of laboratory animals were followed.
Conflict of Interest
Sherif Hafez declares that he has no conflict of interest. Md Nasrul Hoda declares that he has no conflict of interest. Xinyue Guo declares that she has no conflict of interest. Maribeth H. Johnson declares that she has no conflict of interest. Susan C. Fagan declares that she has no conflict of interest. Adviye Ergul declares that she has no conflict of interest.
Author information
Authors and Affiliations
Corresponding author
Rights and permissions
About this article
Cite this article
Hafez, S., Hoda, M.N., Guo, X. et al. Comparative Analysis of Different Methods of Ischemia/Reperfusion in Hyperglycemic Stroke Outcomes: Interaction with tPA. Transl. Stroke Res. 6, 171–180 (2015). https://doi.org/10.1007/s12975-015-0391-0
Received:
Revised:
Accepted:
Published:
Issue Date:
DOI: https://doi.org/10.1007/s12975-015-0391-0