Abstract
ABO blood groups have been proposed to influence malaria parasite infection and disease severity in individuals residing in different geographical areas. In Thailand, genetic polymorphisms of blood groups and susceptibility to malaria infection have rarely been investigated. The aim of this study was to assess the genotype frequencies of ABO and Duffy blood groups and susceptibility to malaria infection in two populations residing in malaria-endemic areas of Thailand. 1100 malaria samples and an identical number of samples from healthy subjects were collected from Thai-Malaysian and Thai-Myanmar areas. Genotyping of ABO and Duffy blood groups was performed by sequence specific primer-polymerase chain reaction. The distribution of ABO and Duffy blood groups was similar in malaria-positive and negative subjects. Blood group O was prevalent in both populations followed by blood group B (BO genotype) and A (AO genotype), respectively. In Plasmodium falciparum infections, blood group A frequency was significantly higher in Thai-Malaysian samples (P = 0.042) whereas blood group B frequency was significantly higher in Thai-Myanmar samples (P = 0.022). FY*A/*A frequency was significantly higher in Plasmodium vivax infection (P = 0.036) while FY*A/*B frequency was significantly higher in healthy subjects (P = 0.005). The different ABO blood group frequencies in the two populations may contribute to susceptibility to P. falciparum infection and the high prevalence of FY*A/*A can confer a risk of P. vivax infection. Further research in various ethnic groups is needed to clarify the association between blood groups and pathogenesis of malaria.
Similar content being viewed by others
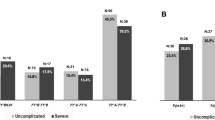
Avoid common mistakes on your manuscript.
Introduction
Malaria is an infectious disease which caused around 409,000 deaths in 2019 (WHO 2020). In Thailand, Plasmodium vivax and P. falciparum are the main causes of malaria infections (Ministry of Public Health 2020; Baum et al. 2015). Most of the malaria cases occur in rural areas or near forest covered regions along international borders such as Thai-Myanmar, Thai-Cambodian and Thai Malaysian (Rungsihirunrat et al. 2008; Thway et al. 2018). Although malaria can be completely cured, some patients develop severe symptoms which depend on several factors (Andrade and Barral-Netto 2011). Susceptibility to Plasmodium infection and pathogenesis of malaria result from a combination of parasite, mosquito carrier, environment and host factors such as blood groups (Andrade and Barral-Netto 2011; Laishram et al. 2012). A blood group is defined by a red blood cell antigen with different characteristics controlled by a series of genes (Mitra et al. 2014). The ABO and Duffy blood groups are important in many biomedical fields including malariology (Mattos and Moreira 2004; Martins et al. 2017).
Several studies from different geographical areas reported associations between the ABO blood group and malaria but with contradicting results (Gupta and Chowdhuri 1980; Panda et al. 2011; Kuesap and Na-Bangchang 2018; Tekeste and Petros 2010; Pathirana et al. 2005; Zhang et al. 2017; Bayoumi et al. 1986). The ABO blood group is associated with malaria severity with the O blood group protective against severe malaria since it lacks the receptors for the parasite rosetting ligands and the A and B blood groups susceptible to malaria infection (Gupta and Chowdhuri 1980; Panda et al. 2011; Kuesap and Na-Bangchang 2018; Tekeste and Petros 2010; Pathirana et al. 2005). Nevertheless, some studies reported no significant relationship between the ABO blood groups and malaria (Zhang et al. 2017; Bayoumi et al. 1986; Degarege et al. 2019). While an association of the ABO blood group in infection with P. falciparum has been reported such data is limited for infection with P. vivax (Resende et al. 2017; Gayathri et al. 2013).
Duffy blood group and susceptibility to P. vivax infection has been well-documented. The antigen of this blood group, Duffy antigen receptor for chemokines (DARC), serves as a receptor for P. vivax parasites. Homozygous Duffy-negative individuals are naturally resistant to P. vivax invasion while heterozygous Duffy-negative individuals are susceptible to P. vivax infections (Miller et al. 1976, 1977). There is only limited data concerning the association between Duffy blood group and P. vivax infection in Thailand (Pimpat et al. 2016).
The aim of this study was to investigate the phenotype and genotype frequencies of ABO and Duffy blood groups in malaria patients residing in endemic areas of Thailand. The obtained results will assist in personal risk protection and rational design of antimalarial drugs to overcome the problems relating to treatment and control of malaria.
Materials and methods
Ethical consideration
Ethical approval for the study was obtained from the Ethics Committee of Thammasat University (COA No.090/2561) and National Blood Centre, Thai Red Cross Society (COA No. NBC 4/2019).
Study population and sample collection
Study sites were malaria clinics or hospitals in malaria-endemic areas from the Thai-Myanmar (Tak and Kanchanaburi provinces; western area) and Thai-Malaysian (Yala provinces; southern area) borders. Male and female participants were 18–60 years old and lived in the investigated endemic areas. In total, 2200 samples were included in this study. 1100 positive malarial samples consisting of genomic DNA (gDNA) of confirmed malaria from a previous collection (2012–2017) and EDTA blood samples or finger prick dried blood spots (2018–2019) were collected from malaria patients at the study sites. Detection of malaria infection was initially by Giemsa-stained thick and thin blood films (Tangpukdee et al. 2009) and confirmed by nested polymerase chain reaction (nested PCR) that used Plasmodium genus-specific primers for the initial PCR (round 1) amplifications and species-specific primers for the second (round 2) amplifications (Singh et al. 1999). 1100 healthy subject samples or prevalent data, non-malaria subjects, were obtained from uninfected people who lived in the same area close to the study sites or from the Regional Blood Centre, Thai Red Cross Society. Non-malaria subjects were screened from donors who had no history of malaria infection by face-to-face interviews. All samples were included in ABO blood group determination. Two hundred samples from P. vivax infected patients and an identical number of healthy subject samples from western (Tak province) and southern (Yala province) areas were included in Duffy blood group genotyping.
Blood group determination
Standard agglutination method (serological method) was used to determine the phenotypes of positive controls for ABO and Duffy blood groups by using commercial antisera (National Blood Centre, Bangkok, Thailand). DNA samples with known phenotypes and genotypes were included as controls, i.e., A, B, O, and AB for ABO genotyping and Fy(a + b −), Fy(a + b +), Fy(a − b +), and Fy(a − b −) for Duffy genotyping.
DNA extraction
Genomic DNA was extracted from blood samples using a QIAamp DNA mini kit (Qiagen, California, USA) according to the manufacturer’s instruction (QIAGEN Supplementary Protocol) and stored at − 20 °C until used.
DNA amplifications
ABO blood group genotyping
ABO blood group was determined by sequence specific primer-polymerase chain reaction (PCR-SSP). Four reaction systems were used to identify A, B, and O blood groups based on three different single nucleotide polymorphisms (SNPs) at position 261, 796, and 803, respectively (Muro et al. 2012). Individual ABO blood group genotyping included four PCR reaction systems. Reaction system 1 detected the A and B alleles which have G at position 261. Reaction system 2 detected the O allele that has a deletion at position 261. Reaction system 3 detected the B allele which has A at position 796 and reaction system 4 detected the A and O alleles which have G at position 803 (Muro et al. 2012). The primers and reaction conditions used for amplification of the four reaction systems were according to the previously described method with some modifications (Muro et al. 2012). Each reaction sample had a volume of 10 µl and contained, 1X Taq buffer with KCl (Thermo Scientific, Massachusetts, USA), 3 mM MgCl2 (Thermo Scientific), 0.4 mM dNTPs (Bioline, London, United Kingdom), 0.3 µM reaction system 2 primers and 0.2 µM reaction systems 1, 3 and 4 primers (Sigma, Lowa, USA), and 0.05 U Taq polymerase (Thermo Scientific). The PCR conditions for amplification were as follows: initial denaturation at 95 °C for 5 min, followed by 35 cycles of 95 °C for 30 s, annealing at 67 °C (reaction systems 1 and 3), 53 °C (reaction system 2), 55 °C (reaction system 4) for 30 s and extension at 72 °C for 30 s, and then final extension at 72 °C for 5 min. The amplified PCR products were stained with Ultra Power™ DNA safe dye (Gellex International Co., Ltd., Machida, Tokyo, Japan), separated by 2% agarose gel electrophoresis at 100 V for 40 min and visualized under UV illumination.
Duffy blood group genotyping
Duffy blood group was determined by PCR-SSP. Two reaction systems were used to identify FY*A and FY*B alleles based on SNPs in exon 2 from G to A at position 125 (Olsson et al. 1998). The primers and reaction conditions used for amplification of FY*A and FY*B alleles were according to the previously described method with some modifications (Olsson et al. 1998). Co-amplification of the human growth hormone (HGH) gene as internal control was performed as previously described (Olsson et al. 1998; Chen and Flegel 2005). Briefly, the 10 µl PCR reaction mixture comprised 1X Taq buffer with KCl (Thermo Scientific), 2 mM MgCl2 (Thermo Scientific), 0.4 mM dNTPs (Bioline), 0.3 µM primers (Sigma), and 0.05 U Taq polymerase (Thermo Scientific). The PCR conditions were as follows: initial denaturation at 96 °C for 5 min, followed by 10 cycles of 94 °C for 20 s, annealing at 69 °C for 1 min, 20 cycles of 94 °C for 30 s, 64 °C for 1 min, 72 °C for 1 min then final extension at 72 °C for 5 min. The amplified PCR product was stained with Ultra Power™ DNA safe dye (Gellex International Co., Ltd.), separated by 1.5% agarose gel electrophoresis at 100 V for 40 min and visualized under UV illumination.
The FY*X allele was identified by PCR-SSP. PCR was performed for samples which had negative results for the FY*B allele. The reaction systems were based on SNPs in exon 2 from C to T at position 265 (Palacajornsuk et al 2015). The primers and reaction conditions used for amplification of FY*X allele were according to the previously described method with some modifications (Palacajornsuk et al. 2015). HGH gene was used as an internal control by co-amplification with FY*X allele (Nathalang et al. 2015). Briefly, the 10 µl PCR reaction mixture comprised 1X Taq buffer with KCl (Thermo Scientific), 1.5 mM MgCl2 (Thermo Scientific), 0.2 mM dNTPs (Bioline), 0.4 µM FYB-FW and FYX-RV primers (Sigma), 0.2 µM HGH-F and HGH-R primers (Sigma), and 0.1 U Taq polymerase (Thermo Scientific). The PCR conditions were as follows: denaturation at 95 °C for 1 min followed by 30 cycles of 95 °C for 30 s, 67 °C for 30 s, extension at 72ºC for 45 s, and final extension at 72ºC for 5 min. The PCR product was stained with Ultra Power™ DNA safe dye (Gellex International Co., Ltd.), separated by 1.2% agarose gel electrophoresis at 100 V for 40 min and visualized under UV illumination.
Data analysis
Statistical analysis was performed using the SPSS statistical package (version 15.0 SPSS Inc., Chicago, Illinois, USA). The frequencies of malaria species, ABO blood group and Duffy blood group were summarized as percentages (%). Differences between groups were analysed using the Chi-square test (χ2). Statistical significance level was set at α = 0.05.
Results
The 1100 positive malaria samples analysed in this study consisted of 200 samples from P. falciparum patients and 900 samples from P. vivax patients. In terms of site, 260 samples were from the western areas (Tak 171 and Kanchanaburi 89 samples) and 840 samples from the southern area. The 1100 samples from healthy, non-malaria subjects consisted of 200 samples from the western areas and 900 samples from the southern area.
ABO blood group
ABO blood group in malaria patients and healthy subjects
The phenotypic frequencies of ABO blood groups in malaria infected samples and healthy subjects are presented in Table 1. The most prevalent ABO blood group in malaria patients was O (37.1%) and the least was AB (7.6%). A similar trend was found in healthy subjects. The O blood group was also most prevalent in P. falciparum (32.0%) and P. vivax (38.2%) patients. The frequency of blood group A was significantly higher in P. falciparum patients (P = 0.044), while no significant difference was found for all blood groups in P. vivax infection.
The genotype of ABO blood group in malaria patients
A representative agarose gel showing analysis of the ABO genotypes after PCR-SSP is shown in Fig. 1. The genotype frequencies of ABO blood groups in malaria infected samples are shown in Table 2. Six ABO genotypes (AA, AO, BB, BO, OO, and AB) were observed. Of these, OO (32%) had the highest frequency in P. falciparum infections, followed by BO (23.0%), AO (22.5%), AB (8.5%), BB (7.5%), and AA (6.5%) genotypes. The same pattern was found in P. vivax infections. The OO genotype was the predominant ABO blood group in patients infected with P. falciparum (32.0%) and P. vivax (38.2%). This finding was eventually correlated to the higher frequencies of AO and BO genotypes compared to the homozygous AA and BB genotypes. Although, the genotype pattern was not indicate significant p value difference between the two species of infection, AA and BB genotype frequencies in P. falciparum infection were almost 1.6 and 1.8 times higher than in P. vivax infection.
Example of an Ultra PowerTM DNA safe dye stained 2.0% agarose gel electrophoresis of PCR-SSP products for ABO genotyping. Lanes 1–4 = A (AO), lanes 5–8 = B (BO), lanes 9–12 = B (BB), lanes 13–16 = O (OO). Reaction system 1 (lanes 1, 5, 9) resulted in a 79 bp product for A and B alleles detection, reaction system 2 (lanes 2, 6, 14) in a 74 bp product for O allele detection, reaction system 3 (lanes 7, 11) in a 109 bp product for B allele detection and reaction system 4 (lanes 4, 8, 16) in a 104 bp product for A and O alleles detection. M = 25 bp ladder marker (Bioline, London, United Kingdom)
The association of ABO blood groups and malaria infection in different endemic areas
Table 3 shows the comparison between ABO blood group and malaria infection in the two endemic areas. The A (38.7%) and B (35.5%) blood groups showed the highest frequencies in P. falciparum infected patients from Thai-Malaysian and Thai-Myanmar areas, respectively. Their frequencies were statistically significantly different in these areas with P = 0.042 and P = 0.022, respectively (Table 3). The O blood group was dominant in P. vivax infected patients from Thai-Malaysian (37.2%) and Thai-Myanmar areas (45.1%).
Duffy blood group
A representative agarose gel showing analysis of the Duffy genotypes after PCR-SSP is shown in Fig. 2. FY*A/*A (83.5%, 75.0%) was the predominant Duffy blood group followed by FY*A/*B (13.0%, 24.0%), and FY*B/*B (3.5%, 1.0%) in both P. vivax infected patients and healthy subjects (Table 4). The FY*Null/*Null blood group, Fy(a–b–) phenotype, was not found in any study participant. The frequency of FY*A/*A was significantly higher in P. vivax infected patients than in healthy subjects (P = 0.036) whereas frequency of FY*A/*B was significantly higher in healthy subjects than in P. vivax infection (P = 0.005). Frequency of FY*B/*B was higher in P. vivax infected patients than in healthy subjects but not significantly different. The mutation of the FY*X gene at nucleotide position 265 was not found in 167 FY*B negative samples.
Example of an Ultra PowerTM DNA safe dye stained 1.5% agarose gel electrophoresis of PCR-SSP products for Duffy genotyping. Lanes 1, 2, 9–10 = FY*A/*B, lanes 3, 4, 7–8 = FY*A/*A, lanes 5, 6 = FY*B/*B. Reaction system 1: lanes 1, 3, 7, 9 show a 711 bp product for FY*A alleles detection whereas lane 5 is negative for FY*A alleles detection. Reaction system 2: lanes 2, 6, 10 show a 711 bp product for FY*B alleles detection whereas lanes 4, 8 are negative for FY*B alleles detection. M = 100 bp ladder marker (Thermo Scientific, Massachusetts, USA). Internal control: 434 bp PCR product of Human Growth Hormone gene
Discussions
This study revealed a high prevalence of the O blood group in healthy individuals and in malaria patients in the studied Thai population. These results were similar to a study in East China in which the O blood group was the most common blood group among malaria cases (Zhang et al. 2017), severe and non-severe falciparum malaria anemia children in Kenya (Ahmed et al. 2020), and that of uncomplicated falciparum cases in Odisha, India (Panda et al. 2011). Furthermore, the results also agreed with a P. vivax study in South India (Gayathri et al. 2013).
Significant differences in the genotype frequencies of the ABO blood group were not observed between P. falciparum and P. vivax infections but the percentage of patients homozygous for AA and BB in P. falciparum infection was higher than in P. vivax infection. This may suggest an association of A and B antigen dosage with P. falciparum infection. A previous study reported that the O blood group could protect against higher parasitemia in P. falciparum infection (Migot-Nabias et al. 2000). Furthermore, different ethnic populations in different areas were also an important factor associated with P. falciparum infection (Migot-Nabias et al. 2000).
It has been proposed that ethnic differences contribute to variation and severity of diseases (Tekeste and Petros 2010; Rowe et al. 2007). In this study, the association of ABO blood group and malaria infection in different endemic areas of Thailand was investigated. Along the Thailand and Myanmar border (western area) is an ethnic diverse area with a population composed of many ethnic groups such as Thai-Karen and Thai-Myanmar while along the border with Malaysia (southern area) the population are Thai-Malays. In P. falciparum infection, the prevalence of the A blood group was significantly higher in samples from the southern area (38.7%, n = 24) than the western area (24.6%, n = 34) of Thailand (P = 0.042), whereas, the prevalence of the B blood group was significantly higher in the western area (35.5%, n = 49) than the southern area (19.3%, n = 12) (P = 0.022). Contradictory results were observed in a study in Ethiopia that found common O blood groups in different minor ethnic groups (Tekeste and Petros 2010; Zerihun et al. 2011). However, a study in Awash, Metehara and Ziway areas in Ethiopia found high prevalence of A blood group in severe malaria cases whereas high prevalence of the O blood group was found in uncomplicated P. falciparum infection and healthy controls (Tekeste and Petros 2010).
Although the prevalence of the Duffy blood group among P. vivax infected patients in Thailand has been previously studied (Pimpat et al. 2016), the sample size was limited to a small cohort study with 40 samples. In this study, when compared 200 P. vivax infections and 200 healthy subjects, FY*A/*A frequency was significantly increased in P. vivax infections (p = 0.036) but FY*A/*B was significantly increased in healthy subjects (p = 0.005). These data may suggest that FY*A/*A was susceptible to P. vivax infection while FY*A/*B was protective to P. vivax infection in the study population. The predominant FY*A/*A antigen could be preferred as receptor for attachment of P. vivax to reticulocytes which accounts for its higher prevalence in the study sites consistent with the previous study in Thailand (Pimpat et al. 2016). In contrast, this result was different from a study in Brazil which stated that the susceptibility to P. vivax infection and disease severity depended on the Fyb antigen (King et al. 2011). This could be due to the ethnic variations of the study populations.
Conclusions
The study indicated that A and B blood groups are more susceptible to P. falciparum infection while O blood group are most prevalent in study population. FY*A/*A are more susceptible to P. vivax infection as compared with healthy subjects. Therefore, it is suggested to further study the association of blood group and malaria infection to clarify correlations and mechanisms of infection.
References
Ahmed JS, Guyah B, Sang’ D, Webale MK, Mufyongo NS, Munde E, Ouma C (2020) Influence of blood group, glucose-6-phosphate dehydrogenase and haemoglobin genotype on falciparum malaria in children in Vihiga highland of Western Kenya. BMC Infect Dis 20(1):487. https://doi.org/10.1186/s12879-020-05216-y
Andrade BB, Barral-Netto M (2011) Biomarkers for susceptibility to infection and disease severity in human malaria. Mem Inst Oswaldo Cruz 106:70–78. https://doi.org/10.1590/s0074-02762011000900009
Baum E, Sattabongkot J, Sirichaisinthop J, Kiattibutr K, Davies DH, Jain A, Lo E, Lee MC, Randall AZ, Molina DM, Liang X, Cui L, Felgner PL, Yan G (2015) Submicroscopic and asymptomatic Plasmodium falciparum and Plasmodium vivax infections are common in Western Thailand—molecular and serological evidence. Malar J 14:95. https://doi.org/10.1186/s12936-015-0611-9
Bayoumi RA, Bashir AH, Abdulhadi NH (1986) Resistance to falciparum malaria among adults in Central Sudan. Am J Trop Med Hyg 35(1):45–55. https://doi.org/10.4269/ajtmh.1986.35.45
Chen Q, Flegel WA (2005) Random survey for RHD alleles among D+ European persons. Transfusion 45(7):1183–1191. https://doi.org/10.1111/j.1537-2995.2005.00181.x
Degarege A, Gebrezgi MT, Beck-Sague CM, Wahlgren M, de Mattos LC, Madhivanan P (2019) Effect of ABO blood group on asymptomatic, uncomplicated and placental Plasmodium falciparum infection: systematic review and meta-analysis. BMC Infect Dis 19(1):86. https://doi.org/10.1186/s12879-019-3730-z
Gayathri B, Kumar ML, Gomathi N, Shetty J, Reethesh RP (2013) Relationship between ABO blood groups and malaria with clinical outcome in rural area of South India. Glob J Med Public Health 2(2):1–7
Gupta M, Chowdhuri AN (1980) Relationship between ABO blood groups and malaria. Bull World Health Organ 58(6):913–915
King CL, Adams JH, Xianli J, Grimberg BT, McHenry AM, Greenberg LJ, Siddiqui A, Howes RE, da Silva-Nunes M, Ferreira MU, Zimmerman PA (2011) Fy(a)/Fy(b) antigen polymorphism in human erythrocyte Duffy antigen affects susceptibility to Plasmodium vivax malaria. Proc Natl Acad Sci USA 108(50):20113–20118. https://doi.org/10.1073/pnas.1109621108
Kuesap J, Na-Bangchang K (2018) The effect of ABO blood groups, hemoglobinopathy, and Heme Oxygenase-1 polymorphisms on malaria susceptibility and severity. Korean J Parasitol 56(2):167–173. https://doi.org/10.3347/kjp.2018.56.2.167
Laishram DD, Sutton PL, Nanda N, Sharma VL, Sobti RC, Carlton JM, Joshi H (2012) The complexities of malaria disease manifestations with a focus on asymptomatic malaria. Malar J 11:29. https://doi.org/10.1186/1475-2875-11-29
Martins ML, da Silva AR, Santos HC, Alves MT, Schmidt LC, Vertchenko SB, Dusse LMS, Silva Malta MCFD (2017) Duffy blood group system: new genotyping method and distribution in a Brazilian Extra-Amazonian population. Mol Cell Probes 35:20–26. https://doi.org/10.1016/j.mcp.2017.06.001
Mattos LC, Moreira HW (2004) Genetic of the ABO blood system and its link with the immune system. Rev Bras Hematol Hemoter 26:60–63. https://doi.org/10.1590/S1516-84842004000100012
Migot-Nabias F, Mombo LE, Luty AJ, Dubois B, Nabias R, Bisseye C, Millet P, Lu CY, Deloron P (2000) Human genetic factors related to susceptibility to mild malaria in Gabon. Genes Immun 1(7):435–441. https://doi.org/10.1038/sj.gene.6363703
Miller LH, Mason SJ, Clyde DF, McGinniss MH (1976) The resistance factor to Plasmodium vivax in Blacks: the Duffy-blood-group genotype, FyFy. N Engl J Med 295(6):302–304. https://doi.org/10.1056/NEJM197608052950602
Miller LH, McAuliffe FM, Mason SJ (1977) Erythrocyte receptors for malaria merozoites. Am J Trop Med Hyg 26:204–208. https://doi.org/10.4269/ajtmh.1977.26.204
Ministry of Public Health (2020) Thailand malaria elimination program. http://malaria.ddc.moph.go.th/malariaR10/index_newversion.php. Accessed 8 July 2021
Mitra R, Mishra N, Rath GP (2014) Blood groups systems. Indian J Anaesth 58(5):524–528. https://doi.org/10.4103/0019-5049.144645
Muro T, Fujihara J, Imamura S, Nakamura H, Kimura-Kataoka K, Toga T, Iida R, Yasuda T, Takeshita H (2012) Determination of ABO genotypes by real-time PCR using allele-specific primers. Leg Med (tokyo) 14(1):47–50. https://doi.org/10.1016/j.legalmed.2011.10.002
Nathalang O, Intharanut K, Siriphanthong K, Nathalang S, Kupatawintu P (2015) Duffy blood group genotyping in Thai blood donors. Ann Lab Med 35(6):618–623. https://doi.org/10.3343/alm.2015.35.6.618
Olsson ML, Hansson C, Avent ND, Akesson IE, Green CA, Daniels GL (1998) A clinically applicable method for determining the three major alleles at the Duffy (FY) blood group locus using polymerase chain reaction with allele-specific primers. Transfusion 38(2):168–173. https://doi.org/10.1046/j.1537-2995.1998.38298193099.x
Palacajornsuk P, Jamrassri L, Kongtes P (2015) Detection of FY*A, FY*B and FY*X genotypes in Duffyblo group system by polymerase chain reaction-sequence specific primer (PCR-SSP). Bull Chiang Mai Assoc Med Sci 48(2):136–143. https://doi.org/10.14456/jams.2015.11
Panda AK, Panda SK, Sahu AN, Tripathy R, Ravindran B, Das BK (2011) Association of ABO blood group with severe Falciparum malaria in adults: case control study and meta-analysis. Malar J 10:309. https://doi.org/10.1186/1475-2875-10-309
Pathirana SL, Alles HK, Bandara S, Phone-Kyaw M, Perera MK, Wickremasinghe AR, Mendis KN, Handunnetti SM (2005) ABO-blood-group types and protection against severe, Plasmodium falciparum malaria. Ann Trop Med Parasitol 99(2):119–124. https://doi.org/10.1179/136485905X19946
Pimpat Y, Leelawat K, Lek-Uthai U (2016) Red blood cell Duffy antigen receptor for chemokines and susceptibility to Plasmodium vivax infection in Thais. Southeast Asian J Trop Med Public Health 47(5):885–889
Resende SS, Milagres VG, Chaves DG, Fontes CJF, Carvalho LH, Sousa TN, Brito CFA (2017) Increased susceptibility of blood type O individuals to develop anemia in Plasmodium vivax infection. Infect Genet Evol 50:87–92. https://doi.org/10.1016/j.meegid.2017.03.001
Rowe JA, Handel IG, Thera MA, Deans AM, Lyke KE, Koné A, Diallo DA, Raza A, Kai O, Marsh K, Plowe CV, Doumbo OK, Moulds JM (2007) Blood group O protects against severe Plasmodium falciparum malaria through the mechanism of reduced resetting. Proc Natl Acad Sci USA 104(44):17471–17476. https://doi.org/10.1073/pnas.0705390104
Rungsihirunrat K, Sibley CH, Mungthin M, Na-Bangchang K (2008) Geographical distribution of amino acid mutations in Plasmodium vivax DHFR and DHPS from malaria endemic areas of Thailand. Am J Trop Med Hyg 78(3):462–467
Singh B, Bobogare A, Cox-Singh J, Snounou G, Abdullah MS, Rahman HA (1999) A genus-and species-specific nested polymerase chain reaction malaria detection assay for epidemiologic studies. Am J Trop Med Hyg 60(4):687–692. https://doi.org/10.4269/ajtmh.1999.60.687
Tangpukdee N, Duangdee C, Wilairatana P, Krudsood S (2009) Malaria diagnosis: a brief review. Korean J Parasitol 47(2):93–102. https://doi.org/10.3347/kjp.2009.47.2.93
Tekeste Z, Petros B (2010) The ABO blood group and Plasmodium falciparum malaria in Awash, Metehara and Ziway areas. Ethiopia Malar J 9:280. https://doi.org/10.1186/1475-2875-9-280
Thway AM, Rotejanaprasert C, Sattabongkot J, Lawawirojwong S, Thi A, Hlaing TM, Soe TM, Kaewkungwal J (2018) Bayesian Spatiotemporal analysis of malaria infection along an international border: Hlaingbwe Township in Myanmar and Tha-Song-Yang district in Thailand. Malar J 17:428. https://doi.org/10.1186/s12936-018-2574-0
WHO (2020) World malaria report 2020: 20 years of global progress and challenges. https://www.who.int/publications/i/item/9789240015791. Accessed 8 July 2021
Zerihun T, Degarege A, Erko B (2011) Association of ABO blood group and Plasmodium falciparum malaria in Dore Bafeno Area, Southern Ethiopia. Asian Pac J Trop Biomed 1(4):289–294. https://doi.org/10.1016/S2221-1691(11)60045-2
Zhang X, Yang M, Zhao H, Hu J, Li L (2017) Relationship between malaria and ABO blood types in East China. Biomed Res Int 2017:8163762. https://doi.org/10.1155/2017/8163762
Acknowledgements
This study was supported by Thammasat University Research Fund [Contract No. TUGG 142/2562] and National Research Council of Thailand in the new researcher development programme according to research and innovation strategic plan for graduated student [Contract No. 19/2562].
Author information
Authors and Affiliations
Contributions
The authors confirm contribution to the paper. Study conception and design was performed by JK. Material preparation, data collection and analysis were performed by JK and PH. The first draft of the manuscript was written by PH. The final version of the manuscript was revised by JK. All authors read and approved the final manuscript.
Corresponding author
Ethics declarations
Conflict of interest
The authors declare that there is no conflict of interest regarding the publication of this paper.
Additional information
Publisher's Note
Springer Nature remains neutral with regard to jurisdictional claims in published maps and institutional affiliations.
Rights and permissions
About this article
Cite this article
Hongfongfa, P., Kuesap, J. Genotyping of ABO and Duffy blood groups among malaria patients in Thailand. J Parasit Dis 46, 178–185 (2022). https://doi.org/10.1007/s12639-021-01432-8
Received:
Accepted:
Published:
Issue Date:
DOI: https://doi.org/10.1007/s12639-021-01432-8