Abstract
Concentrations of heavy metals were analyzed in the soils to know the metal contamination due to anthropogenic activities in Peenya Industrial Area, Bengarulu, India. Samples were collected from the soil horizon from an industrial area at twelve locations at different depths viz., 0–30 cm, 30–90 cm, and 90–150 cm to understand the soil pollution level due to industrial activities. Heavy metals in the soil were analyzed using ICP-MS X Series-II from M/s. Thermo Fisher Scientific, C-MET, Hyderabad. Analyzed data show that the soil in the industrial area is significantly contaminated with heavy metals than its normal values. Concentration values at 0–30-cm depth for Al ranged from (21647–41,682 mg/kg), As (0–3.90 mg/kg), B (43.88–144.97 mg/kg), Ba (27.06–459.53 mg/kg), Cd (0.22–1.01 mg/kg), Co (7.36–185.71 mg/kg), Cr (14.97–718.13 mg/kg), Cu (1.32–397.60 mg/kg), Fe (11919–72,064 mg/kg), Mn (162.10–1665.82 mg/kg), Mo (0–9.21 mg/kg), Pb (12.62–139.94 mg/kg), Sb (0.48–25.48 mg/kg), Se (0–206.88 mg/kg), Sr (6.19–113.98 mg/kg), Ti (0–0.29 mg/kg), V (16.63–182.62 mg/kg), and Zn (38.81–327.13 mg/kg). Similarly, higher concentration was observed in soil samples collected at 30–90 cm and 90–150 cm depth. Soil contamination was assessed based on index of geoaccumulation (Igeo), enrichment factor (EF), contamination factor (CF), degree of contamination (Cdeg), modified degree of contamination (mCdeg), pollution load index (PLI), ecological risk factor (ER), potential ecological risk index (RI), toxic units (TU), and principal component analysis (PCA). The high values of these indices/factors obtained for soil samples revealed considerable heavy metal pollution due to improper waste management practices within the industrial area. A contaminated site possesses a significant impact on human, terrestrial, and aquatic ecosystems. The comparison was made with the other soil areas from India, Asia, and worldwide. Mobility of heavy metals in groundwater and soil has been discussed in the article.
Similar content being viewed by others
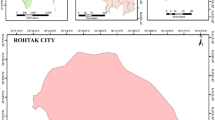
Explore related subjects
Discover the latest articles, news and stories from top researchers in related subjects.Avoid common mistakes on your manuscript.
Introduction
The fast development of the industrial sector during the last few decades has a severe impact on water, soil, and aquatic system. During the development of an industrial sector, the improper management of solid or liquid waste products became a serious problem throughout the world. Contamination of soil considered to be the main sources from metallurgical and other allied activities in the industrial area (Alloway 1990; Saiful et al. 2015; Kormoker et al. 2019). If the elements are present more than the prescribed limits or some alterations occurred to its natural environment, the soil is considered to be contaminated (CEQG 2002). Soil is often contaminated by factors like excessive use of fertilizers/pesticides, improper management of hazardous waste, accidental release of chemicals, anthropogenic or geogenic activities, weathering and erosion of crustal material, and human activities will alter the physical, chemical, and biological quality of soil (Machender et al. 2011; Shrivastava and Mishra 2011; Garba et al. 2014; Saiful et al. 2016). Most of the metals are not soluble and degradable, and persist for longer period as a separate entity in water and soil (Kontas 2007).
Metals like Cu, Fe, Ni, and Zn are beneficial to human organisms, if present in small concentrations and become harmful at high concentrations beyond the prescribed limits (CEQG 2002). Heavy metal were categorized into various groups of elements that vary in their physical, chemical, and biological properties, and as an environmental pollutant due to their toxic effects on living organisms including flora and fauna. Metal are non-bio-degradable and tend to accumulate and enters the food chain leading to health and environmental problems. The occurrence of heavy metals in the soils mainly depends on their mobility, climatic conditions, soil mineralogy, soil texture, pH, temperature, redox potential, cation exchange capacity, and content of organic carbon (Corwin et al. 1999; Ashworth and Alloway 2004; Choppala et al. 2010; Moon et al. 2000; Mapanda et al. 2005; Skordas and Kelepertsis 2005).
Regions with high population centers have become a serious concern for soil contamination, where land has been intensely used for different purposes (Van Straalen 2002). Soils are considered as an ultimate sink for the discharge of heavy metals into the environment. The soil contamination is does not only depend on soil properties but its ability to migrate the chemical elements down to the sediments and to the aquifer regime by dissolution and adsorption processes (Chlopecka et al. 1996; Dang et al. 2002; Jonathan et al. 2004). Soil pollution has a considerable impact on surface and groundwater regime in the region of soil-polluted areas (Jayaprakash et al. 2010; Raju et al. 2011; Mohammed Ashaiekh et al. 2017; Shah et al. 2018; Ram et al. 2019).
The present study is aimed to investigate the distribution and migration of heavy metals in the soil at Peenya Industrial Area (PIA), Bengaluru, India. The soil contamination was assessed by using various indices/factors viz., index of geoaccumulation (Igeo), enrichment factor (EF), contamination factor (CF), degree of contamination (Cdeg), modified degree of contamination (mCdeg), pollution load index (PLI), ecological risk index (ER), potential ecological risk index (RI), toxic units (TU), and principal component analysis (PCA). In order to assess the contamination level in the soil horizon of PIA, soil samples at different depths were collected and analyzed for heavy metals. The degree of pollution level due to industrial activities is discussed in the article.
Study area background
Peenya Industrial Area (PIA) lies between 13° 1′ 42′′ N latitude and 77° 30′ 45′′ E longitude on the north-western suburbs of Bengaluru City, Karnataka State, India. PIA is considered one of the oldest and largest industrial sectors in Southeast Asia. Peenya industrial estate was established during the 1970s by the Karnataka Small Industries Development Corporation. The total extent of the industrial area is about 40 Km2. Total number of operating industries in the area is 1690; out of 1690 industries, more than 200 are electroplating, power coating, heat treatment, and spray painting industries. Soil sampling at various depths is carried out in PIA to know the contaminant distribution of heavy metals in soil. The map of the study area along with industrial boundary is shown (Fig. 1). The objectives of the study are to make a systematic assessment and evaluation of soil contamination under the influence of the high influx of metal pollutants from the industrial area and regarding the deterioration of groundwater quality around the industrial area.
Materials and methods
Soil samples were collected at twelve locations in the PIA, Bengaluru at different depths viz., 0–30 cm, 30–90 cm, and 90–150 cm (total 36 samples). The location of soil samples is shown in Fig. 1. The soil horizon was drilled by mechanized auger, and samples were collected at different depths in a clean polythene bags containing about 1 kg. The collected samples were numbered sequentially and brought to the laboratory. At 60 °C, the samples were dried for 48 h to avoid the moisture content. By using swing grinding mill, the sample was finely powered to 250-mm mesh size. Soil sample of 10 g each was taken in the Petri dish and dried at 110 °C for 1 h. From that, 0.1 g of the sample was taken in Teflon tubes and added 4 mL of hydrofluoric acid, 3 mL of nitric acid, and 1 mL of hydrogen peroxide and allowed for 10 min outside. Teflon tubes were placed in the microwave digestion system. The samples were dissolved using microwave digestion system (Anton Paar Multiwave 3000). Microwave system parameters are fixed as pressure 50 bar, wattage 900, temperature 240 °C, and time 90 min. After cooling, the Teflon tubes were taken out from the microwave digestion system, and sample solutions were made to 50 ml and filtered. These solutions were used for elemental analysis using ICP-MS instrument (Thermo Fisher X Series-II).
Instrumentation and analysis
The ICP-MS X Series-II from M/s. Thermo Fisher Scientific was configured with a peltier cooled, quartz impact bead spray chamber, glass concentric nebulizer, and single piece, 1.5-mm injector torch. For sample handling, CETAC ASX-520 Auto sampler was used. Electron multiplier is used as a detector. With its high sensitivity and analytical robustness, the ICP-MS X-Series-II is used for the analysis of heavy metals in soil samples. During the course of analysis to monitor the accuracy and quality data, NIST traceable standard reference solutions of M/s. inorganic ventures, USA NIST, were used. The instrument was optimized once at the beginning of the evaluation using the plasma Lab Autotune facility, and the plasma Lab performance report software tool was used to check the system performance prior to commencing the analysis. The samples were dissolved using Anton Paar Multi-wave 3000 microwave digestion system using a combination of sub boiled hydrofluoric acid, nitric acid, and hydrogen peroxide, then made to 50 ml by using 18.2 MΩ water. The statistical summary of analyzed heavy metals at different depths is given in Table S1 (Supplementary Table).
Index of geoaccumulation (Igeo)
It is applied to measure the contamination by comparing the current and past concentrations of sediments (Taylor and Mckenzie 1966). It is enumerated by the following equation:\( {I}_{\mathrm{geo}}={\log}_2\frac{C_n}{1.5{B}_n} \).
where Cn is the measured concentration of the metal and Bn is the background of observed in sediment. The constant 1.5 is the natural fluctuations of a given substance in the environment. The six classes of contamination based on Igeo values suggested (Muller 1969) were given in Table 1. The statistical summary of the Igeo at different depths was summarized in Table 2.
Enrichment factor (EF)
EF value was enumerated as suggested by (Loska et al. 2004). EF values were calculated based on analysis elements against the reference element. The reference element is one among the analyzed element with its low occurrence (Reimann and De Caritat 2000; Sutherland 2000). Enrichment Factor (EF) for heavy metals in the soil sample and the concentration of the analyzed metals can be normalized to a reference element. In the present study, we consider vanadium (V) as reference element due to its low concentration. Bref (Background) crustal content of banadium (V) value in the earth’s crust was used as 60 mg/kg (Taylor and Mclennan 1995). The EF can be estimated by using the following equation:
where Cn(Sample) represents the observed element, Cref(Sample) represents the reference element, Bn(Background) represents the observed element, and Bref(Background) represents the reference element. Five different categories of EF are suggested for soil classification (Table 1) (Sutherland 2000). The statistical summary of EF was summarized (Table 3).
Toxic unit (TU)
The calculation of toxic units (TU) is considered as severe toxicity of toxic metals in soil. TU analysis is calculated as the ratio of the measured concentration of toxic elements in the soil to that of probable effect level (PEL) (MacDonald et al. 2000). A moderate to serious toxicity of hazardous metals remain in soil when the sum of TU for all soil samples is more than 4 (Bai et al. 2011). Sum of the toxic unit (ΣTU) represents the possible heavy metal toxicity in soils. TU for each metal was calculated using the following formula:
where Cm is the heavy metal concentration in soil and PEL is the probable effect levels value of heavy metals in soil.
Contamination factor (CF)
Soil contamination can also be assessed by using the contamination factor (\( {C}_f^i \)). The \( {C}_f^i \) is the single element index and can be assessed directly the level of contamination of soil at a specific location. It can be calculated as the observed concentration of each metal in the soil to that of regional background concentration of soil and can be expressed by the following equation suggested by (Hakanson 1980).
where \( {C}_{0-1}^i \) is a mean value of the particular metal at sampling sites and\( {C}_n^i \) is the pre-industrial concentration of individual metal.
The assessment of soil contamination in the study area was carried out by using the above equation and classified into four groups (Table 1). The calculated CF was given in Table 4.
Degree of contamination (Cdeg)
Degree of contamination (Cdeg) is an indicator to assess the overall contamination after taking in account the contamination factor (CF) values of all single metals together for any site (Hakanson 1980). The degree of contamination has been used for assessing soil and sediment contamination levels (Loska et al. 2004). Degree of contamination was evaluated by the extent of multi-metal pollution by summing the particular CF values of metals and was enumerated by using the following equation:
where, n is the number of elements and CF is the contamination factor. Soil classification based on the degree of contamination was given in Table 5. In the present study, we aggregate CF values of 17 elements to calculate Cdeg for all samples at different depths viz., 0–30 cm, 30–90 cm, and 90–150 cm (Table S2) (Supplementary Table). Contamination classes by Cdeg depend upon the number of contaminants and categorized (Table 5) (Zahran et al. 2015).
Modified degree of contamination (mCdeg)
Modified degree of contamination can be calculated by dividing the Cdeg by the number of contaminants taken into an account is as follows (Abrahim 2005).
where, Cdeg is the degree of contamination and ‘n’ is the number of contaminants considered.
To modify Cdeg into mCdeg, minimum 3 samples were required (Rahman et al. 2012). In the present study, we collected 12 samples each at a depth of 0–30 cm, 30–90 cm, and 90–150 cm. The categorization of mCdeg is given in Table S2 (Supplementary Table).
Pollution load index (PLI)
The pollution load index (PLI) was proposed by (Tomlinson et al. 1980). This assesses the magnitude of pollution load by the analysis of metal at soil sample collected site. This approach of calculating PLI becomes a tool to generate an action plan (Angulo 1996). It is evaluated as a geometric mean of contamination factor (CF) and expressed as follows:
For the present study, we considered 17 (n) elements and categorized into two groups, if PLI ≤ 1 represents background pollution levels and if PLI ≥ 1 indicates deterioration of soil quality or pollution load. The calculated PLI for the present study shows most of the values was 1 or > 1 indicating a continuous load of pollutants on soil horizon (Table S2) (Supplementary Table).
Ecological risk factor (ER)
This factor can be calculated for any single contaminant metal by its own toxicity response (Hakanson 1980). This can be expressed as follows:
where, Tr is the toxic response of metal, and CF is the contamination factor of that metal. In the present scenario, we considered 8 metals viz., As, Cd, Co, Cr, Cu, Pb, V, and Zn on the availability of their toxic factors and importance to health and ecology. The toxic factor for these metals (As = 10, Cd = 30, Cr = 2, Co = Cu = Pb = 5, V = 2, and Zn = 1) provided by (Hakanson 1980). The risk factors describing these metals are given in Table 6.
Potential ecological risk index (RI)
The RI is a modified version of ER, and it is an important index for evaluating the rate of environmental sensitivity caused by heavy metals in the soil (Aktaruzzaman et al. 2013; Jiao et al. 2015). It is the sum of all risk factors of metals considered for evaluating the RI and can be expressed as follows (Hakanson 1980).
where ER is the ecological risk factor of individual metal and RI has been calculated for 8 (n) metals. The RI values were categorized into four classes (Jiao et al. 2015) given in Table 5, and calculated RI values were given in Table 6.
Results and discussions
Statistical summary of analyzed heavy metals in the soil at different depths was shown in Table S1 (Supplementary Table). Al concentration ranges from 21,647 to 41,682 mg/kg, 28,568–63,132 mg/kg, and 25,424–49,620 mg/kg at 0–30 cm, 30–90 cm, and 90–150 cm depth in soil samples. Similarly, Co ranges from 7.36 to 185.71 mg/kg, 10.58–46.95 mg/kg, 8.79–413.63 mg/kg; Cr ranges from 14.97 to 718.13 mg/kg, 9.06–140.45 mg/kg, 15.17–116.18 mg/kg; Co ranges from 1.32 to 397.60 mg/kg, 1.09–345.92 mg/kg, 7.14–150.86 mg/kg; Fe ranges from 11,919 to 72,064 mg/kg, 17,717–146,251 mg/kg, 13,094–73,422 mg/kg; Pb ranges from 12.62 to 139.94 mg/kg, 0–102.98 mg/kg, 1.84–83.05 mg/kg, and Zn ranges from 38.81 to 327.13 mg/kg, 23.23–945.52 mg/kg, 27.22–2056.48 mg/kg at a depth of 0–30 cm, 30–90 cm, and 90–150 cm respectively. The analyzed soil data reveals that some metal concentrations were exceeding the normal prescribed values of soil give raise the concern of the unsuitability of soil.
The heavy metal concentrations in the soil of contaminated areas of India, Asia, and the World were compared with the present study (Table S3) (Supplementary Table). As concentration was higher in soils of Indian cites (38.61 mg/kg) (Adimalla et al. 2019), Kazipalli, Telangana (796.3 mg/kg) (Krishna and Mohan 2016), Bangladesh 23.14–90 mg/kg (Saiful et al. 2015, 2016; Kormoker et al. 2019; Ram et al. 2019), China 12.15–279 mg/kg (Jiao et al. 2020; Li et al. 2007; Pan et al. 2018), Iran (18.94 mg/kg) (Modabberi et al. 2018), USA (13.2 mg/kg) (Cannon and Horton 2009), Turkey (16.8 mg/kg) (Gemici and Gultekin 2007), while in other areas it is below the prescribed value (Supp. Table 9). In the present study, As concentration ranges from 3.90 to 12.27 mg/kg.
Pb concentration 1830 mg/kg was high only in Kazipalli, Telangana (Krishna and Mohan 2016), while in other areas of India, it is below the prescribed value. Pb concentration in Bangladesh 567 mg/kg (Saiful et al. 2015), Tunisia 5950.5 mg/kg (Intissar et al. 2020), Italy 141 mg/kg (Cicchella et al. 2008), USA 198 mg/kg (Cannon and Horton 2009), Romania 27.73 mg/kg (Apostoae and Iancu 2009), Iran 93.18 mg/kg (Jamshidi-Zanjani and Saeedi 2013; Salehi et al. 2014) and Nigeria 568 mg/kg (Odewande and Abimbola 2008; Nwachukwu et al. 2010). In the present study, Pb ranges from 83.05 to 139.94 mg/kg. Similarly, Cr concentration was high in Uttar Pradesh − 2652.3 mg/kg (Gowd et al. 2010), Chennai − 75.02 mg/kg (Raju et al. 2011), Manali, Chennai − 221.7 mg/kg (Krishna and Govil 2008), Wailpally, Nalgonda − 60.6 mg/kg (Krishna et al. 2011), Patancheru, Hyderabad − 70.13 mg/kg (Dasaram et al. 2011), Bengal − 194.83 mg/kg (Muthuraj and Jayaprakash 2007), Indian cities − 412.28 mg/kg (Adimalla 2019), Nirmal Province, Medak District, Ranga Reddy District and Kazipalli areas of Telangana state − 135.8 mg/kg, 751 mg/kg, 64.50 mg/kg, and 598.6 mg/kg (Adimalla and Wang 2018; Adimalla et al. 2019, 2020; Krishna and Mohan 2016) and West Bengal − 182.55 mg/kg (Krishnendu Kumar et al. 2020). In Bangladesh − 1120 mg/kg (Saiful et al. 2015, 2016), China − 109 mg/kg and 73 mg/kg (Jiao et al. 2020; Pan et al. 2018), Norway − 81 mg/kg (Andersson et al. 2010; Lu et al. 2013), USA − 65 mg/kg (Cannon and Horton 2009), while in the present study area, it ranges from 116.18 to 718.13 mg/kg.
Zn prescribed value in soil was 200 mg/kg. Zn concentration was high in Indian cities 437.44 mg/kg (Adimalla 2019), Kazipally, Telangana − 879 mg/kg (Krishna and Mohan 2016), and Tunisia − 12,488.5 mg/kg (Intissar et al. 2020), while in the present study, Zn concentration was ranged from 327.13 to 2056.48 mg/kg. Similarly, Ni prescribed value in soil was 50 mg/kg. Ni concentration in Indian cities − 120.43 mg/kg (Adimalla 2019) and Kazipally area − 129.6 mg/kg (Krishna and Mohan 2016), while in other places of India it is below the threshold value. Ni concentration in Bangladesh urban soil was 443 mg/kg (Saiful et al. 2015), Jhenaidah and Kushtia district, Bangladesh − 77.32 mg/kg (Kormoker et al. 2019), Sudan − 163.20 mg/kg (Mohammed Ashaiekh et al. 2017), Iran − 105.21 mg.kg (Modabberi et al. 2018), and Italy it was − 89 mg/kg (Cicchella et al. 2008), while in the present study, Ni concentration was below the threshold value. For remaining heavy metals like Co, Cu, V, Sr, Ba, and Zr, comparison study was presented (Table 9) (Supplementary Table).
Aluminum (Al)
The average Al concentration in the soil was 33,838.69 mg/kg, 38,689.80 mg/kg, and 36,257.76 mg/kg, and the concentration ranges from 21,647.84 to 41,682.80 mg/kg, 28,568.85–63,132.90 mg/kg, and 25,424.73–49,620.78 mg/kg at a depth of 0–30 cm, 30–90 cm, and 90–150 cm respectively (Table S1) (Supplementary Table). The average Igeo value for Al was 17.44, 17.62, and 17.54, and Igeo values range from 16.82 to 17.76, 17.22–18.36, and 17.05–18.02 at different depths respectively (Table 2 and Fig. 2). EF value ranges from 1703 to 12,786, 3193–9909, and 1007–8546 with average values of 6174, 5355, and 5199 respectively (Table 3 and Fig. 3). Similarly, CF values ranges from 0.81 to 1.56, 0.61–1.35, and 0.73–1.41 with average values of 1.03, 1.04, and 1.04 respectively (Table 4). The values of Cdeg (12.41, 12.57, and 12.41) and values of PLI (1, 1, and 1) at respective depths are shown (Table S2) (Supplementary Table). The high content of Al in soil was due to the improper management of waste product in the industrial area. The mobility and solubility of Al was strongly related to the organic matter in soil and found a strong and significant relationship between dissolved organic compound (Feike and Ross 2003). The high concentration of Al was observed in the leaves of Buckwheat and its distribution in leaves control by transpiration. Al is not mobile once it is accumulated in leaf, and the mobility of phosphate’s across the soil root interface is strongly influenced by the pH and aluminum content (Renfang and Jian 2001; Carlo et al. 2005).
Arsenic (As)
The average As concentration in the soil was 0.92 mg/kg, 4.40 mg/kg, and 1.45 mg/kg, and its values were ranges from 0 to 3.90 mg/kg, 0–4.40 mg/kg, and 0–12.27 mg/kg at a depth of 0–30 cm, 30–90 cm, and 90–150 cm respectively (Table S1) (Supplementary Table). As contents in the soil are normal at a depth of 0–30 cm and 30–90 cm, but little high contents were observed in the soil sample collected at a depth of 90–150 cm. The average Igeo value for As was − 0.05, − 0.04, and 0.17 and it ranges from − 2.6 to 1.96, − 2.01 to 2.13 and 0 to 1.72 at respective depths. The Igeo values calculated for soil representing that the soil is uncontaminated to moderately contaminated with As (Table 2 and Fig. 2). EF value for As ranges from 0 to 5.1, 0–4.08, and 0–12.85 with average values of 0.98, 0.68, and 1.52 at respective depths (Table 3 and Fig. 3). Similarly, CF values for As range from 0. to 5.6, 0–3.2, and 0–2.47 with average values of 1.15, 0.69, and 0.34 at respective depths (Table 4). The values of Cdeg (13.86, 8.28, and 4.16), values of PLI (1, 0.98, and 0.96), and values of RI (138.62, 82.84, and 41.62) at respective depths are shown (Table S2 (Supplementary Table) and Table 6).
Retention of As in soil primarily depends on the factors like Fe and Al oxides, redox potential, pH, clay content, and cation-anion exchange and adsorption of hydrous oxides of Fe and Al (Corwin et al. 1999; Elkhatib et al. 1984; Fuller et al. 1993; Wilkie and Hering 1996). As mobility in soil mainly depends on the physical-chemical condition, sorption capacity, redox potential, pH, and clay contents. The greater the sorption capacity of As into soil, lesser its mobility through soil pores (Davis et al. 1994; Mariner et al. 1996). Generally, As(V) adsorption is maximum than As(III) at lower pH conditions and its retention depends on the clay type (Elkhatib et al. 1984). The elevated content of As in soil is due to the anthropogenic processes in the study area.
Barium (Ba)
The average Ba content is 174.50 mg/kg, 122.33 mg/kg, and 114.39 mg/kg, and it ranges from 27.06 to 469.53 mg/kg, 20.03–233.32 mg/kg, and 38.29–213.28 mg/kg at a depth of 0–30 cm, 30–90 cm, and 90–150 cm respectively (Table S1) (Supplementary Table). Maximum prescribed limit of Ba in soil is 500 mg/kg (CEQG 2002) indicating the soil was getting enriched with Ba concentration at a depth of 0–30-cm depth. The Igeo values obtained ranges from 13.27 to 17.36, 12.84–16.38, and 13.77–16.25 with average values of 15.58, 15.21, and 15.18 at a various depths indicating the soil was very heavily contaminated (Table 2 and Fig. 2), while the EF values range from 0.02 to 1.21, 0.03–0.57, and 0.01–0.6 at different depths indicating the soil was depletion to minimal enrichment (Table 3 and Fig. 3). CF values for Ba ranges from 0.37 to 6.44, 0.52–6.1, and 0.53–2.98 with average values of 1.85, 1.52, and 1.27 at respective depths (Table 4). The values of Cdeg (22.31, 18.29, and 15.28) and values of PLI (1.02, 1.01, and 1.01) at respective depths are shown (Table S2) (Supplementary Table). Various researchers noticed that high Ba content in soils of Wailpally, Patancheru, and Balanagar industrial area (Machender et al. 2011; Krishna et al. 2011; Dasaram et al. 2011). The impact of barite waste was observed in stream sediment of Kupa and Kupica River which is being used as drinking water source (Franciskovic-Bilinski et al. 2007). High concentration of Ba in soil and water will have a direct impact on human health and ecosystem. Ba is released into the air during the process of manufacturing of barium chemicals in the study area.
Cobalt (Co)
The average concentration of Co is 32.42 mg/kg, 23.78 mg/kg, and 54.45 mg/kg, and it ranges from 7.36 to 185.71 mg/kg, 10.58–46.95 mg/kg, and 8.79–413.63 mg/kg at respective depths (Table S1) (Supplementary Table). Calculated Igeo ranges from 5.61 to 10.27, 6.14–8.29, and 5.87–11.42 with average values of 7.07, 7.17, and 7.3 at respective depths respectively (Table 2 and Fig. 2) indicating that soil is very heavily contaminated. The EF values range from 1.36 to 13.4, 1.84–4.09, and 0.47–4.06 with average values of 3.21, 2.38, and 1.83 addressing a significant enrichment of Co in soil (Table 3 and Fig. 3). CF values for Co range from 0.17 to 4.4, 0.5–2.24, and 0.13–6.19 with average values of 2.01, 1.2, and 3.15 at respective depths (Table 4). The values of Cdeg (24.20, 14.40, and 37.89), values of PLI (1.04, 1.0, and 1.07), and values of RI (121.01, 71.96, and 189.44) at respective depths are shown (Table S2 (Supplementary Table) and Table 6). Co-mobility and sorption behavior in groundwater have been studied (Bangash et al. 1992; Scott et al. 1998). Co-transportation in groundwater is mainly influenced by pH conditions and microbiological factors. Several researchers proposed that the hydrous metal oxides of Fe and Mn are the controlling factors for the distribution of Co (Bangash et al. 1992). The results of sorption studies have shown a high capacity of oxide minerals for Co sorption (Rahner et al. 1993). The variation of Co concentration at different depths is shown (Fig. 4).
Chromium (Cr)
Concentration of Cr in soil ranges from 14.97 to 718.13 mg/kg, 9.06–140.45 mg/kg, and 15.17–116.18 mg/kg with average values of 121.61 mg/kg, 62.71 mg/kg, and 57.97 mg/kg at a depth of 0–30 cm, 30–90 cm, and 90–150 cm respectively (Table S1) (Supplementary Table). Igeo values range from 8.44 to 14.03, 7.72–11.67, and 8.46–11.4 (Table 2 and Fig. 2). Most of the Igeo values fall into the class very heavily contaminated with Cr. EF obtained for Cr ranges from 0.58 to 14.8, 0.46–4.97, and 0.47–4.06 (Table 3 and Fig. 3), with average values of 3.85, 1.84, and 1.83 at various depths, indicating the class of significant enrichment. CF values for Cr range from 0.16 to 8.12, 0.44–6.91, and 0.49–3.82 with average values of 2.35, 1.58, and 1.41 at respective depths (Table 4). The values of Cdeg (228.25, 19.08, and 16.93), values of PLI (1.04, 1.01, and 1.01), and values of RI (56.50, 38.15, and 33.86) at respective depths are shown (Table S2 (Supplementary Table) and Table 6). Sources of Cr in the industrial area appear to be due to Cr electroplating industries and improper disposal of waste. The variation of Cr is shown in Fig. 5.
Cr occurs in two valence states as trivalent (Cr+3) and hexavalent (Cr+6). Cr+6 are considered to be toxic to plants and animals. Its mobility is low, under moderate oxidizing and reducing conditions, and at neutral pH. Adsorption of Cr in soil depends on pH condition, with an increase in pH, adsorption of Cr+6 decreases, and adsorption of Cr+3 increases with increase in pH, while Cr+6 are weakly absorbed to soils having alkaline or slight acidic nature, but adsorption is high in soil containing iron. Cr+6 is more stable with atmospheric oxygen, but it reduces to Cr+3 in the presence of organic matter (Choppala et al. 2010). The mobility of Cr also depends on the retardation factor of soil. Cr+6 have higher mobility than Cr+3, and readily transported (Choppala et al. 2010; Scott 1995). The migration and contamination of Cr from the tailing dumps, rejected ore, storage yards, and mining pits area into the groundwater regime were evaluated through simulation modeling study (Zayed and Terry 2003; Dhakate and Singh 2008; Dhakate et al. 2008).
Copper (Cu)
Concentration of Cu in the soil ranges from 1.32 to 397.60 mg/kg, 1.09–345.92 mg/kg, and 7.14–150.86 mg/kg with average values of 121.61 mg/kg, 62.71 mg/kg, and 57.97 mg/kg at respective depths (Table S1) (Supplementary Table). The Igeo values for Cu ranges from 4.46 to 12.69, 4.19–12.49, and 6.89–11.29 with average values of 9.39, 8.71, and 9.34 at respective depths pointing towards heavily contaminated soil (Table 2 and Fig. 2). Similarly, EF values ranges from 0.19 to 26.09, 0.05–9.53, and 0.47–7.73 with average values of 4.53, 2.07, and 2.17 at respective depths indicating moderate to significant enrichment pollution and indicating alarming signals (Table 3 and Fig. 3). CF values for Cu ranges from 0.19 to 59.85, 0.17–54.81, and 0.34–7.23 with average values of 6.82, 8.2, and 2.0 at respective depths (Table 4). The values of Cdeg (81.89, 98.50, and 24.08), values of PLI (1.06, 1.07, and 1.02), and values of RI (409.46, 492.48, and 120.41) at respective depths are shown (Table S2 (Supplementary Table) and Table 6). Higher concentration of Cu, Igeo, and EF may be due to the industrial activities and improper waste management. Several researchers reported a high concentration of Cu in soils of many industrial areas in India (Krishna and Govil 2008; Machender et al. 2011; Raju et al. 2011). Mobility of Cu mainly depends on pH condition, presence of organic matter, high content of Fe and Al hydroxide, and soluble complexes (Ashworth and Alloway 2004). Cu normally retains in the soil horizon by the process of bioaccumulation of metals and recent anthropogenic activities (Kabata-Pendias 2004). The variation of Cu is shown in Fig. 6.
Nickel (Ni)
Ni content in soils was nil at a depth of 0–30 cm depth, and it ranges from 0 to 44.17 mg/kg and 0–18.61 mg/kg at a depth of 30–90 cm and 90–150 cm with averages of 3.68 mg/kg and 1.55 mg/kg (Table S1) (Supplementary Table). Usually, Ni present in a bounded form in the soil. In acidic and neutral pH conditions, the mobility and bioavailability of Ni increases (Kabata-Pendias and Pendias 1999). The mobility of Ni in soil and groundwater mainly depends on pH values, organic matter content, and high content of Fe and Al hydroxides. These conditions make the Ni more soluble with other heavy metals in soil and groundwater regimes (Ashworth and Alloway 2004).
Lead (Pb)
The average concentration of Pb in the soil was 44.09 mg/kg, 22.73 mg/kg, and 27.82 mg/kg and ranges from 12.62 to 139.94 mg/kg, 0–102.98 mg/kg, and 1.84–83.05 mg/kg at respective depths (Table S1) (Supplementary Table). Igeo values for Pb range from 7.39 to 10.86, 0–9.2, and 0–7.95 at respective depths indicating most of the soil fall under very heavily contaminated (Table 2 and Fig. 2). EF for Pb ranges from 0.2 to 12.57, 0–5.87, and 0.06–5.07 indicating the soil in the study area was moderate to significant enrichment (Table 3 and Fig. 3). CF values for Pb range from 0.31 to 3.49, 0–3.06, and 0.33–15.12 with average values of 1.69, 1.34, and 2.86 (Table 4). Values of Cdeg (20.37, 16.13, and 34.39), values of PLI (1.02, 1.01, and 1.04), and values of RI (101.83, 80.64, and 171.96) at respective depths are shown (Table S2 (Supplementary Table) and Table 6). Pb species vary with soil type, and its mobility in soil and groundwater mainly depends upon the pH condition, soil containing more clay, high organic matter, and Fe and Al hydroxides (Ashworth and Alloway 2004).
Strontium (Sr)
Sr concentration in the soil ranges from 6.19 to 113.98 mg/kg, 3.84–86.11 mg/kg, and 5.25–92.19 mg/kg with average values of 61.53 mg/kg, 31.98 mg/kg, and 35.17 mg/kg at respective depths (Table S1) (Supplementary Table). Igeo values obtained for Sr were ranges from 10.49 to 14.69, 0–12.99, and 0–13.18 with average values of 13.36, 6.64, and 10.19 at respective depths (Table 2 and Fig. 2) indicating the soil was very heavily contaminated with Sr. Similarly, EF obtained for Sr ranged from 0.01 to 1.14, 0.01–0.28, and 0.007–0.36 with average values of 0.32, 0.1, and 0.11 at respective depths indicating the soil was depletion to minimal enrichment (Table 3 and Fig. 3). CF values for Sr range from 0.53 to 9.93, 0.37–8.32, and 0.38–6.69 with average values of 2.27, 2.4, and 2.35 (Table 4). Values of Cdeg (27.27, 28.87, and 28.31) and values of PLI (1.03, 1.03, and 1.04) are shown (Table S2) (Supplementary Table). Sr was more mobile in the weathered zone, soil with high clay content, organic matter, and acidic environments (Gowd et al. 2010).
Zinc (Zn)
Concentration of Zn in soil ranges from 38.81 to 327.13 mg/kg, 23.23–945.52 mg/kg, and 27.22–2056.48 mg/kg with average values of 107.76 mg/kg, 140.54 mg/kg, and 226.91 mg/kg at respective depths (Table S1) (Supplementary Table). Igeo values for Zn range from 10.84 to 13.91, 10.1–15.44, and 10.33–16.57 with average values of 12, 11.7, and 11.77 at respective depths (Table 2 and Fig. 2). Igeo values indicate that the soil in the study area was very heavily contaminated. Similarly, EF values obtained for Zn range from 0.33 to 7.55, 0.42–10.1, and 0.35–20.85 indicating the soil in the study area is significant to very high enrichment (Table 3 and Fig. 3). CF values for Zn range from 0.32 to 2.77, 0.14–6.04, and 0.11–8.33 with average values of 1.45, 2.71, and 4.22 at respective depths (Table 4). Values of Cdeg (17.48, 32.56, and 50.71); values of PLI (1.02, 1.05, and 1.10); values of RI (17.47, 32.56, and 50.71) at respective depths are shown (Table 6 and Table S2 (Supplementary Table). The main sources of Zn pollution are due to the industrial waste disposal and manufacture of agrochemicals and pesticides.
Vanadium (V)
Concentration of V in the soil ranges from 16.63 to 182.62 mg/kg, 26.39–116.38 mg/kg, and 32.04–241.57 mg/kg with average values of 10.7.76 mg/kg, 140.54 mg/kg, and 68.36 mg/kg at respective depths (Table S1) (Supplementary Table). Igeo values for V range from 9.37 to 12.83, 10.04–12.18, and 10.32–13.23 with average values of 10.85, 11.12, and 11.16 at respective depths (Table 2 and Fig. 2) indicating the soil in the area was very heavily contaminated with V. The minimum and maximum values of EF for V are 1 for all respective depths; soil indicates the depletion to minimal enrichment (Table 3 and Fig. 3). CF values for V range from 0.31 to 3.48, 0.51–2.28, and 0.28–3.12 with average values of 1.49, 1.16, and 1.32 (Table 4). Values of Cdeg (17.92, 13.94, and 15.91) and values of PLI (1.02, 1.0, and 1.01) at respective depths are shown (Table S2 (Supplementary Table) and Table 6).
Molybdenum (Mo)
Concentrations of Mo in the study area range from 0 to 9.21 mg/kg, 0–22.73 mg/kg, and 0–3.27 mg/kg with average values of 2.66 mg/kg, 3.22 mg/kg, and 3.27 mg/kg at respective depths (Table S1) (Supplementary Table). Igeo values for Mo range from − 1 to 3.2, − 2.89 to 4.5, and − 4.37 to 0.24 at respective depths (Table 2 and Fig. 2) indicating the soil is practically uncontaminated to heavily contaminated. Similarly, EF value ranges from 0 to 11.04, 0–11.5, and 0–2.1 at various depths indicating significant enrichment (Table 3 and Fig. 3). CF values for Mo range from 0 to 5.34, 0–23.91, and 0–9.51 with average values of 0.92, 2.02, and 0.87 (Table 4). Values of Cdeg (11.12, 24.26, and 10.48) and values of PLI (0.99, 0.98, and 0.97) at respective depths are shown (Table S2) (Supplementary Table).
Toxic unit analyses
The sum of toxic units (ΣTU) determines the possibility of heavy metal toxicity in the soils. Toxic units can be calculated as the ratio of heavy metal concentration in soil against the measured probable effect levels (PELs). Total toxic units with toxic units due to heavy metals toxicity in soil sample location in the industrial area were presented (Fig. 7). The sum of toxic units for the studied metals for the sites S5 and S8 for a depth of 0–30 cm, S9 and S11 for a depth of 30–90 cm, and S11 for a depth of 90–150 cm exceeding the value of 4 and caused a serious threat to the environment.
Correlation of heavy metals
Pearson’s correlation method has been adopted for understanding the relationship among the elements for the soil samples collected at respective depths (Table S4, S5 and S6) (Supplementary Table). The following correlations are considered as strong positive correlation between Al with Fe (0.64), B-Cd (0.75), B-Fe (0.97), B-V (0.93), Cd-Fe (0.80), Cd-V (0.73), Co-Cr (0.97), Co-Sb (0.93), Co-Se (0.97), Cr-Sb (0.95), Cr-Se (0.99), Fe-V (0.97), Mn-Mo (0.63), Mn-Ti (0.85), Pb-Ti (0.78), and Sb-Se (0.96) for a depth of 0–30-cm soil sample (Table S4) (Supplementary Table). Similar, strong correlation was observed between Al and Pb (0.79), Al-V (0.62), As-B (0.65), As-Cd (0.69), As-Cu (0.77), As-Fe (0.68), As-Se (0.64), B-Cd (0.97), B-Co (0.61), B-Cu (0.87), B-Fe (0.99), B-Mn (0.83), B-Se (0.87), B-V (0.62), Cd-Cu (0.94), Cd-Fe (0.98), Cd-Mn (0.81), Cd-Se (0.87), Cd-Sr (0.62), Co-Mn (0.86), Co-Pb (0.68), Co-V (0.87), Cr-Se (0.68), Cr-Sr (0.95), Cu-Fe (0.91), Cu-Mn (0.65), Cu-Se (0.87), Cu-Sr (0.64), Fe-Mn (0.79), Fe-Se (0.89), Fe-Sr (0.63), Mn-Se (0.64), Mn-V (0.88), Mo-Zn (0.87), Pb-V (0.71), and Se-Sr (0.76) at a depth of 30–90 cm (Table S5) (Supplementary Table). Similarly, strong correlation (> 0.60) was observed between As and Pb. B-Cd, Fe-V, Cd-Fe, Se-V, Co-Cr, Mn, Mo-Sr, Cr-Mn, Mo, Sr, Cu-Sb, Se, Fe-V, Mn-Mo, Sr, Mo-Sr, Sb-Se, and Ti-Zn at a depth of 90–150 cm (Table S6) (Supplementary Table). The significant strong positive correlation within these elements reveals that there is a common anthropogenic source of pollution in the study area. Similarly, strong negative correlations were also observed between these elements. Presence of Sr in the soil has a bearing on the accumulation of heavy elements and is highly influenced by the adsorption characteristics of other heavy elements. Association of geochemical of metals like Cd-Cr-Cu-Zn deposited in the soil indicates an anthropogenic sources contributing to the soil horizon.
Source analysis of heavy metals in soil
Principal component analysis (PCA) was carried out to obtain initial Eigen values and variance percent of the data to reduce the dimensionality from the 17 parameters to five principal components (PCs) for soil data at respective depths by using Statistical Package for Social Sciences (SPSS) software (Table S7) (Supplementary Table). PCA was applied to the varimax normalized data to compare the compositional patterns between the analyzed soil samples and to identify the factors that influence. The factor loading are classified as strong, moderate, and weak corresponding to absolute loading values of ˃ 0.75, 0.75–0.50, and 0.50–0.30, respectively (Mor et al. 2006).
The output of the final rotated loading matrix obtained for soil data at 0–30 cm depth results five principal component analyses which explains 91.83% of the total variance. PC1 represent 36.90% of variance with the loading of Cr, Se, Sb, and Co are possibly due to the runoff and discharge of wastewater from industrial areas. PC2 represented 21.10% of the total variance and had high loadings of V, Fe, B, and Cd suggesting that these elements have a similar chemical behavior, which was presented partly in oxide form and partly in hydroxide form (Chapman et al. 1998). PC3 represented 18.81% of the total variance and high loadings on Cu and Zn which indicate that the fertilizers in agricultural areas are a major external source for these metals. PC4 represents 9.01% of the total variance with high loadings of Mn, Pb, Mo, and Ba which indicates that the natural as well as anthropogenic sources (coal based, metals and alloys, leather processing, electroplating, lead batteries, welding units, etc.) are attributed. The PC5 with total variance of 6.0% represents the high loadings on As and Sr and negative loading of Al which indicate anthropogenic sources.
The soil at depth 30–90 cm obtained the results of the five-factor analysis explains 90.25% of the total variance, the PC1, PC2, PC3, PC4, and PC5 were found to be responsible for the variations in soil quality explains 46.90%, 14.76%, 12.20%, 9.10%, and 7.32% of total variance respectively. PC1 shows high loadings on As, Cu, Fe, Cd, B, and Sb, thus, covering the metals having origin both as natural and anthropogenic sources. PC2 is influenced by Co, Mn, V, and Sr; and PC3 includes variable like Cr, Se, Sb, and Al which indicates that the chromium salts in leather processing and discharges wastewater containing high levels of chromium (Sinha et al. 2002). The PC4 shows high positive loadings of Zn and Mo. PC5 shows high positive loadings of Ba and negative loadings of Pb and Sr.
The soil at depth 90–150 cm also obtained the results of the four-factor analysis explains 84.11% of the total variance, the four PC1, 2, 3, and 4 were found to be responsible for the variations in soil quality explains 36.70%, 23.10%, 16.49%, and 8.02% of total variance respectively. PC1 shows high loadings of Co, Mn, Se, Mo, and Cr which are possible long term, and extensive use of pesticides in farmland may cause heavy metals such as Co, Mn, Se, Mo, and Cr to accumulate in the topsoil (Huang et al. 2010; Shao 2012). The PC2 is influenced by Fe, B, Cd, V, and negative loading of Ba indicating the source is anthropogenic activity. PC3 includes metals like Sr, Zn, Pb, and Cu indicating the source was due to industrial activities. The PC4 shows high positive loadings of Sb, and Cu and negative loadings of As, Ba, and Al indicating that due to improper management of industrial waste products. All these factor score and variance pointing towards soil contamination due to industrial activities.
The relationships among all soil samples were also examined in terms of the total contents of heavy metals by hierarchical cluster analysis. The obtained dendogram is depicted (Fig. 8). For 0-30-cm depth soil, the observed dendogram for the heavy metals with significant linkage distance, indicating relatively high independency for each cluster. The first cluster of metals are Cr, Se. Co, Sb, and As are well correlated with each other and the second cluster was associated with Cu, Zn, Mn, Pb, Ba, and Mo, and the third cluster was associated with B, Fe, V, Cd, Al, and Sr (Fig. 8). Cluster one is joined to the cluster two and cluster three by a significantly large linkage distance which indicates relatively high independency for each group. Cluster one may be attributed to the anthropogenic sources and clusters two and three to both the natural and anthropogenic sources. For 30–90 cm depth soil, the observed dendogram shows significant linkage distance, indicating relatively high independency for each cluster. In the first cluster, elements like B, Cd, Fe, Sb, As, and Cu are well correlated with each other and form the second cluster which associated with Mo, Zn, Pb, Sr, and form the third cluster which associated with Cr, Se, Co, V, Mn, Al, and Ba which is attributed to local industrial effluents. The producing progress of industrials, accompanied by the drainage of untreated effluents like acid wash and plating liquid results in polluting soil (Fig. 8). The hierarchical cluster analysis was used to systematically cluster for fourteen elements for at depth of 90–150 cm soil is observed. Before the analysis, the Z score was used to standardize the variables to reduce the influence of various element content differences on clustering. Hierarchical cluster analysis (HCA) produces similar results as PCA. The fourteen elements can be roughly classified into three categories: the first type includes Co, Mn, and Mo; the second type is Cu, Pb, Sr, As, Zn; and the third type is Al, B, Fe, Cd, V, Cr, Se, and Sb (Fig. 8). The first group may derive from nonferrous metal smelting. The second category may come from anthropogenic sources. The third category is mainly from natural sources and industrial activities.
Conclusion
The soil of the Peenya Industrial Area is contaminated due to improper management of solid/liquid waste disposal and industrial activities and persisting for a long duration. Elevated contents of metal like As, Ba, Cr, Cu, Fe, Pb, Sr, and Zn in the soil of the industrial area indicates that the soil is polluted with these metals, and these metals are originated from the industrial activities and have a direct impact on human health, groundwater, terrestrial, and the ecological system. The mobility of these metals in soil and groundwater has also been studied and compared with other studies carried out in India, Asia, and worldwide. The study alerts to take immediate precautional measures to prevent the soil pollution of the area and requires various remediations like bio-remediation and phytoremediation. The analysis results of a heavy metal show that the average concentrations of few metals are more than the permissible values. Indices like Igeo, EF, CF, Cdeg, mCdeg, PLI, ER, and RI revealed that the soil of the study area has significant to very high enrichments of few metals. Igeo values obtained for analyzed metals indicate that the soil is heavy to very heavily contaminated with metals like As, Mo, Sb, Se, and Ti. EF and CF values obtained for different metals also indicate the soil is moderate to significant enrichment with most of the metals. Other indices like the degree of contamination (mCdeg), toxic unit, pollution load index (PLI), ecological risk factor (ER), and potential ecological risk index (RI) show the significant variations in their spatial distribution of contamination level. Modified degree of contamination (mCdeg) values for most of the metals at various depth soil samples show significant contamination. Similarly, the analysis of the toxic unit indicates the few locations are more toxic as the value of the toxic unit is more than 4. Pollution load index (PLI) values for most elements are > 1 indicating deterioration of soil quality in the area. ER values obtained for metals like As, Cd, Co, Cr, Cu, Pb, V, and Zn indicate that low ecological risk with these metals except for Cd for few samples indicating moderate risk. Similarly, RI values obtained for As, Cd, Co, Cr, Cu, Pb, V, and Zn indicate that the soil is at moderate risk with As, Co, Cu, Pb, and Zn and considerable risk with Cd and Cu, PCA, prevalent five principal components, and the indentification of the sources. The soil sample data at 0–30-cm depth, 30–90-cm depth, and 90–150-cm depth explain the 91.83%, 90.25%, and 84.11% variance with a maximum loading of elements like As, Cd, Co, Cr, and Fe. Despite low ecological risk potential, there is a need for further study to monitor the contamination level.
References
Abrahim GMS (2005) Holocene sediments of Tamaki Estuary: characterisation and impact of recent human activity on an urban estuary in Auckland, New Zealand. Ph.D. thesis. University of Auckland, Auckland, New Zealand p. 361
Adimalla N (2019) Heavy metals contamination in urban surface soils of Medak province, India, and its risk assessment and spatial distribution. Environ Geochem Health. https://doi.org/10.1007/s10653-019-00270-1
Adimalla N, Wang H (2018) Distribution, contamination, and health risk assessment of heavy metals in surface soils from northern Telangana, India. Arab J Geosci 11(21):684
Adimalla N, Qian H, Wang H (2019) Assessment of heavy metal (HM) contamination in agricultural soil lands in northern Telangana, India: an approach of spatial distribution and multivariate statistical analysis. Environ Monit Assess 191(4):246
Adimalla N, Chen J, Qian H (2020) Spatial characteristics of heavy metal contamination and potential human health risk assessment of urban soils: a case study from an urban region of South India. Ecotoxico Environ Saf. https://doi.org/10.1016/j.ecoenv.2020.110406
Aktaruzzaman M, Fakhruddin ANM, Chowdhury MAZ, Fardous Z, Alam MK (2013) Accumulation of heavy metals in soil and their transfer to leafy vegetables in the region of Dhaka Aricha Highway, Savar, Bangladesh. Pak J Biol Sci 16:332–338
Alloway BJ (1990) Heavy metals in soils, vol 339. Blackie, London
Andersson M, Ottesen RT, Langedal M (2010) Geochemistry of urban surface soils-monitoring in Trondheim, Norway. Geoderma 156(3):112–118
Angulo E (1996) The Tomlinson Pollution Load Index applied to heavy metal, ‘Mussel-Watch’data: a useful index to assess coastal pollution. Sci Total Environ 187:19–56
Apostoae L, Iancu OG (2009) Heavy metal pollution in the soils of Ias¸i city and the suburban areas (Romania). Studia Universitatis Babes-Bolyai. Geologia. (Special Issue, MAEGS–16)
Ashworth DJ, Alloway BJ (2004) Soil mobility of sewage sludge derived dissolved organic matter, copper, nickel and zinc. Environ Pollut 127(1):137–144
Bai J, Xiao R, Cui B, Zhang K, Wang Q, Lui X, Gao H, Huang L (2011) Assessment of heavy pollution in wetland soils from the young and old reclaimed regions in the Pearl River Estuary, South China. Envrion Poll 159:817–824
Bangash MA, Hanif J, Khan MA (1992) Sorption behavior of cobalt on illitic soil. Waste Manag 12(1):29–38
Cannon W, Horton JD (2009) Soil geochemical signature of urbanization and industrialization-Chicago, Illinois, USA. Appl Geochem 24:1590–1601
Carlo E, Gessa TM, Salvatore D, Claudio M (2005) Effect of aluminium and pH on the mobility of phosphate through a soil root interface model. Plant Soil 272(1–2):301–311
CEQG (Canadian Environmental Quality Guidelines, 2002) Summary table for soil quality guidelines. Canadian Council of Ministers of the environment
Chapman PM, Wang F, Janssen C, Persoone G, Allen HE (1998) Ecotoxicology of metals in aquatic sediments: binding and release, bioavailability, risk assessment, and remediation. Can J Fish Aquat Sci 55:2221–2243
Chlopecka A, Bacon JR, Wilson HJ, Kay J (1996) Forms of cadmium, lead and zinc in contaminated soils from southwest Poland. J Environ Qual 25:69–79
Choppala G, Nanthi B, Megharaj M, Zuliang C (2010) Sorption and mobility of chromium species in a range of soil types. In: 9th World congress of soil science, soil solutions for a changing world, 1–6, Brisbane, Australia 239–241
Cicchella D, De Vivo B, Lima A, Albanese S, McGill R, Parrish RR (2008) Heavy metal pollution and Pb isotopes in urban soils of Napoli, Italy. Geochemistry: Exploration, Environ, Analysis 8:103–112
Corwin DL, David A, Goldberg S (1999) Mobility of arsenic in soil from the Rocky Mountain Arsenal area. J Contam Hydrol 39(1–2):35–58
Dang Z, Liu C, Haigh MJ (2002) Mobility of heavy metals associated with the natural weathering of coal mine spoils. Environ Pollut 118:419–426
Dasaram B, Satyanarayanan M, Sudarshan V, Krishna AK (2011) Assessment of soil contamination in Patancheru industrial area, Hyderabad, Andhra Pradesh, India. Res J Environ Earth Sci 3(3):214–220
Davis A, Kempton JH, Nicholson A (1994) Groundwater transport of arsenic and chromium at a historical tannery, Woburn, Massachusetts, USA. Appl Geochem 9:569–582
Dhakate R, Singh VS (2008) Heavy metal contamination in groundwater due to mining activities in Sukinda valley, Orissa-a case study. J Geogr Reg Plan 1(4):58–67
Dhakate R, Singh VS, Hodlur GK (2008) Impact assessment of chromite mining on groundwater through simulation modelling study in Sukinda chromite mining area, Orissa, India. J Hazard Mater 160(2–3):535–547
Elkhatib EA, Bennet OL, Wright RJ (1984) Arsenite sorption and desorption in soils. Soil Sci Soc Am J 48:1025–1029
Feike AD, Ross DF (2003) Aluminium solubility and mobility in relation to organic carbon in surface soil affected by six tree species of the northeastern United States. Geoderma 114(1):33–47
Franciskovic-Bilinski S, Bilinski H, Grbac R, Zunic J, Necemer M, Hanzel D (2007) Multidisciplinary work on barium contamination of the karstic upper Kupa River drainage basin (Croatia and Slovenia); calling for watershed management. Environ Geochem Health 29:69–79
Fuller CC, Davis JA, Waychumas GA (1993) Surface chemistry of ferrihydrite: part 2. Kinetics of arsenate adsorption and coprecipitation. Geochim Cosmochim Acta 57:2271–2282
Garba ST, Ahiahmed AJC, Dauda BA (2014) Distribution pattern of heavy metals: Pb, Zn, Cd and Cu in roadside soils of Maiduguri, Metropolis, Borno State Nigeria. Int Res J Pure Appl Chem 4(5):486–493
Gemici U, Gultekin T (2007) Assessment of the pollutants in farming soils and waters around untreated abandoned Turkonu mercury mine (Turkey). Environ Contam Tox 79:20–24
Gowd SS, Reddy RM, Govil PK (2010) Assessment of heavily metal contamination in soils at Jajmau (Kanpur) and Unnao industrial areas of the Ganga Plain, Uttar Pradesh, India. J Hazard Mater 174:113–121
Hakanson L (1980) An ecological risk index for aquatic pollution controls. A sedimentologi-cal approach. Water Res 14:975–1001
Huang YB, Lan YB, Thomson SJ, Fang A, Hoffmann WC, Lacey RE (2010) Development of soft computing and applications in agricultural and biological engineering. Comput Electron Agric 71(2):107–127
Intissar E, Jose ME, Garcıa-Ordiales E, Zouheir E, Garcia-Noguero EM, Jalel B, Juan AC, Pablo LH (2020) Biogeochemical assessment of the impact of Zn mining activity in the area of the Jebal Trozza mine. Central Tunisia Environ Geochem Health. https://doi.org/10.1007/s10653-020-00595-2
Jamshidi-Zanjani A, Saeedi M (2013) Metal pollution assessment and multivariate analysis in sediment of Anzali international wetland. Environ Earth Sci 70(4):1791–1808
Jayaprakash M, Urban B, Velmurugan PM, Srinivasalu S (2010) Accumulation of total trace metals due to rapid urbanization in microtidal zone of Pallikaranai marsh, South of Chennai, India. Environ Monit Assess 170:609–629
Jiao X, Teng Y, Zhan Y, Wu J, Lin X (2015) Soil heavy metal pollution and risk assessment in Shenyang Industrial District, Northeast China. PLoS One 10:01–09
Jiao L, Song L, Chen H, Wu J, Teng Y (2020) Source apportionment of potential ecological risk posed by trace metals in the sediment of the Le’an River, China. J Soils Sed 20:2460–2470
Jonathan MP, Ram Mohan V, Srinivasalu S (2004) Geochemical variations of major and trace elements in recent sediments of the Gulf of Mannar, the south-east coast of India. Environ Geol 45:466–480
Kabata-Pendias A (2004) Soil-plant transfer of heavy metals-an environmental issue. Geoderma 122:143–149
Kabata-Pendias A, Pendias H (1999) Biogeochemistry of trace elements, 2nd edn. PWN, Warsaw (In Polish)
Kontas A (2007) Trace metals (Cu, Mn, Ni, Zn, Fe) contamination in marine sediment and zooplankton samples from Izmir Bay (Aegean Sea, Turkey). Water Air Soil Pollut 188:323–333
Kormoker T, Ram P, Saiful I, Ahmed S, Chandra K, Uddin M, Rahman M (2019) Toxic metals in agricultural soils near the industrial areas of Bangladesh: ecological and human health risk assessment. Toxin Rev. https://doi.org/10.1080/15569543.2019.1650777
Krishna AK, Govil PK (2008) Assessment of heavy metal contamination in soils around Manali industrial area, Chennai, Southern India. Environ Geol 54(7):1465–1472
Krishna AK, Mohan KR (2016) Distribution, correlation, ecological and health risk assessment of heavy metal contamination in surface soils around an industrial area, Hyderabad, India. Environ Earth Sci 75:411–427
Krishna AK, Mohan KR, Murthy NN (2011) Multivariate statistical approach for monitoring of heavy metals in sediments: a case study from Wailpalli Watershed, Nalgonda District, Andhra Pradesh, India. Res J Environ Earth Sci 3(2):103–113
Krishnendu Kumar P, Sumanta N, Manash G, Rai AK, Saha R (2020) Sources evaluation, ecological and health risk assessment of potential toxic metals (PTMs) in surface soils of an industrial area, India. Environ Geochem Health. https://doi.org/10.1007/s10653-020-00517-2
Li G, Cao Z, Lan D, Xu J, Wang S, Yin W (2007) Spatial variations in grain size distribution and selected metal contents in the Xiamen Bay. China Environ Geol 52:1559–1567
Loska K, Wiechula D, Korus I (2004) Metal contamination of farming soils affected by industry. Environ Int 30:159–165
Lu Z, Cai M, Wang J, Yin Z, Yang H (2013) Levels and distribution of trace metals in surface sediments from Kongsfjorden, Svalbard, Norwegian Arctic. Environ Geochemistry and Health 35:257–269
MacDonald DD, Ingersoll CG, Berger TA (2000) Development and evaluation of consensus-based sediment quality guidelines for freshwater ecosystems. Arch Environ Contam Toxicol 39:20–31
Machender G, Dhakate R, Prasanna L, Govil PK (2011) Assessment of heavy metals contamination in soil around Balanagar industrial area, Hyderabad, India. Environ Earth Sci 63:945–953
Mapanda F, Mangwayana EN, Nyamangara J, Giller KE (2005) The effect of long-term irrigation using wastewater on heavy metal contents of soils under vegetables in Harare, Zimbabwe. Agric Ecosyst Environ 107:151–165
Mariner PE, Holzmer FJ, Jackson RE, Meinardus HW, Wolf FG (1996) Effects of high pH on arsenic mobility in a shallow sandy aquifer and on aquifer permeability along the adjacent shoreline, Commencement Bay Superfund site, Tacoma, Washington. Environ Sci Technol 30:1645–1651
Modabberi S, Tashakor M, Sharifi Soltani N, Hursthouse AS (2018) Potentially toxic elements in urban soils: source apportionment and contamination assessment. Environ Monit Assess 190(12):715
Mohammed Ashaiekh A, Mohamed Eltayeb AH, Ali H, Ebrahim AM, Salih I, Idris AM (2017) Spatial distribution of total and bioavailable heavy metal contents in soil from agricultural, residential, and industrial areas in Sudan. Toxin Rev 38:93–105. https://doi.org/10.1080/15569543.2017.1419491
Moon JW, Moon HS, Woon NC, Hahn JS, Won JS, Song Y, Lin X, Zhao Y (2000) Evaluation of heavy metal contamination and implication of multiple sources from Hunchun basin, northeastern China. Environ Geol 39:1039–1052
Mor S, Khaiwal R, Dahia RP, Chandra A (2006) Leachate characterization and assessment of groundwater pollution near municipal solid waste landfill site. Environ Monit Assess 118:435–456
Muller G (1969) Index of geoaccumulation in sediments of the Rhine River. Geol J 2:108–118
Muthuraj S, Jayaprakash M (2007) Distribution and enrichment of trace metals in marine sediments of Bay of Bengal, off Ennore, South-east coast of India. Environ Geol 56:207–217
Nwachukwu MA, Feng H, Alinnor J (2010) Assessment of heavy metal pollution in soil and their implications within and around mechanic villages. Int J Environ Sci Technol 7(2):347–358
Odewande AA, Abimbola AF (2008) Contamination indices and heavy metal concentrations in urban soil of Ibadan metropolis, southwestern Nigeria. Environ Geochem Health 30:243–254
Pan L, Wang Y, Ma J, Hu Y, Su B, Fang G, Wang L, Xiang B (2018) A review of heavy metal pollution levels and health risk assessment of urban soils in Chinese cities. Environ Sci Pollut Res 25(2):1055–1069
Rahman SH, Khanam D, Adyel TM (2012) Assessment of heavy metal contamination of agricultural soil around Dhaka Export Processing Zone (DEPZ), Bangladesh: implication of seasonal variation and indices. Appl Sci 2:584–601
Rahner S, Magaritz M, Amiel AJ (1993) Scavenging effects of Fehydroxide coated sand in phreatic aquifers. Appl Geochem 2(Suppl. Iss):45–148
Raju K, Vijayaraghavan K, Srinivasalu S, Jayaprakash M (2011) Impact of anthropogenic input on physicochemical parameters and trace metals in marine surface sediments of Bay of Bengal off Chennai, India. Environ Monit Assess 177:95–114
Ram P, Kormoker T, Saiful I (2019) Distribution, source identification, ecological and health risks of heavy metals in surface sediments of the Rupsa River, Bangladesh. Toxin Rev. https://doi.org/10.1080/15569543.2018.1564143
Reimann C, De Caritat P (2000) Intrinsic flaws of element enrichment factors (EF’s) in environmental geochemistry. Environ Sci Technol 34:5084–5091
Renfang S, Jian FM (2001) Distribution and mobility of aluminium in a Al-accumulating plant, Fagopyrum esculentum Moench. J Exp Bot 52(361):1683–1687
Saiful I, Ahmed K, Habibullah-Al-Mamun, Masunaga S (2015) Potential ecological risk of hazardous elements in different land-use urban soils of Bangladesh. Sci Total Environ 512-513:94–102
Saiful I, Ahmed K, Habibullah-Al-Mamun (2016) Apportionment of heavy metals in soil and vegetables and associated health risks assessment. Stoch Env Res Risk A 30:365–377
Salehi F, Abdoli MA, Baghdadi M (2014) Sources of Cu, V, Cd, Cr, Mn, Zn Co, Ni, Pb, Ca and Fe in soil of Aradkooh landfill. Int J of Environ Research 8(3):543–550
Scott EF (1995) Surface reactions of chromium in soils and waters. Geoderma 67:55–71
Scott CB, Herman JS, Hornberger GM, Mills AL (1998) Biodegradation of cobalt–citrate complexes: implications for cobalt mobility in groundwater. J Contam Hydrol 32:99–115
Shah J, Khattak SA, Muhammad S, Ahmad R, Farooq M, Khan S, Khan A, Ali L (2018) Ecological and health risk assessment of heavy metals in the Hattar industrial estate, Pakistan. Toxin Rev 39:68–77. https://doi.org/10.1080/15569543.2018.1478858
Shao HB (2012) Metal contamination: sources, Detection and Environmental Impact. Nova Science, New York
Shrivastava KBL, Mishra SP (2011) Studies of various heavy metal in surface and groundwater of Birsinghpur Town and its surrounding rural area District Satna (MP). Curr World Environ 6(2):271–274
Sinha S, Saxena R, Singh S (2002) Comparative studies on accumulation of Cr from metal solution and tannery effluent under repeated metal exposure by aquatic plants: its toxic effects. Environ Monit Assess 80:17–31
Skordas K, Kelepertsis A (2005) Soil contamination by toxic metals in the cultivated region of Agia, Thessaly, Greece. Identification of sources of contamination. Environ Geol 48:615–624
Sutherland RA (2000) Bed sediment associated trace metals in an urban stream, Oahu, Hawaii. Environ Geol 39:611–627
Taylor RM, McKenzie RM (1966) The association of trace elements with manganese minerals in Australian soils. Aust J Soil Res 4:29–39
Taylor SR, Mclennan SM (1995) The geochemical evolution of the continental crust. Rev Geophys 33:241–265
Tomlinson DL, Wilson J, Harris CR, Jeffrey DW (1980) Problems in the assessments of heavy metal levels in estuaries and formation of a pollution index. Helgol Meeresunters 33:566–575
Van Straalen NM (2002) Assessment of soil contamination - a functional perspective. Biodegradation 13:41–52
Wilkie JA, Hering JG (1996) Adsorption of arsenic onto hydrous ferric oxide: effects of adsorbate/adsorbent ratios and co-occurring solutes. Colloids Surf A Physicochem Eng Aspects 107:97–110
Zahran MAE, El-Amier YA, Elnaggar AA, Mohamed HAE, El-Alfy MAE (2015) Assessment and distribution of heavy metals pollutants in Manzala Lake, Egypt. J Geosci Environ Protect 3:107–122
Zayed AM, Terry N (2003) Chromium in the environment: factors affecting biological remediation. Plant Soil 249:139–156
Acknowledgments
The authors express their sincere gratitute to the Director, CSIR-NGRI, Hyderabad for his continuous support for research activities. The manuscript Reference No. NGRI/Lib/2018/Pub-70. Authors are thankful to Karnataka State Pollution Control Board, Bangalore for sponsoring this work. Authors are thankful to the Chief Editor for his encouragement and support. Authors also thank the anonymous reviewers for their constructive and scientific suggestion for improvement of the manuscript. Authors thanks to AcSIR-Academy of Scientific and Innovative Research, Ghaziabad for their support.
Author information
Authors and Affiliations
Corresponding author
Additional information
Responsible Editor: Amjad Kallel
Electronic supplementary material
ESM 1
(DOC 323 kb)
Rights and permissions
About this article
Cite this article
Dhakate, R., Ratnalu, G.V. & Laxmankumar, D. Evaluation of heavy metals contamination in soils at Peenya Industrial Area, Bengarulu, India. Arab J Geosci 13, 880 (2020). https://doi.org/10.1007/s12517-020-05900-y
Received:
Accepted:
Published:
DOI: https://doi.org/10.1007/s12517-020-05900-y