Abstract
An attempt was made to explore the effect of copper sulphate treatment on growth, photosynthesis, osmolytes and antioxidants in 15 days old seedlings of C. cajan (Pigeonpea). C. cajan seedlings were grown in 0, 1, 5 and 10 mM concentrations of copper sulphate in petriplates lined with Whatman filter paper for 15 days. Root length and shoot length was decreased in a dose dependent manner with highest decrease of 82.80 and 45.92% in 10 mM Cu stress. Photosynthetic efficiency (qP, qN and Y) was decreased in a dose dependent manner whereas NPQ was increased in 1 and 5 mM and decreased in 10 mM Cu. Photosynthetic pigments viz total chlorophyll and carotenoids were increased in low concentrations and decreased in high concentrations of Cu. Osmolytes such as proline, glycine betaine and sugars were found to be increased in a dose dependent manner. Similarly antioxidants such as superoxide dismutase and catalase increased to 129.17 and 169.7%, respectively under Cu stress. Vitamin C and vitamin E was also increased in different concentrations of Cu to a significant level. It can be concluded from the present study that C. cajan can tolerate Cu stress up to 5 mM by adjusting the proportion of proline, glycine betaine, sugars and vitamins along with increasing the activity of some of the antioxidant enzymes.
Similar content being viewed by others
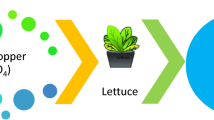
Explore related subjects
Discover the latest articles, news and stories from top researchers in related subjects.Avoid common mistakes on your manuscript.
Introduction
Copper (Cu) is an indispensable micronutrient for plant growth and development (Sharma and Agrawal 2005). It is a core ion in plant pigments and enzymes such as plastocyanin, polyphenol oxidase, superoxide dismutase, tyrosinase, cytochrome c oxidase (Yruela 2005). It plays an important role in various physiological processes such as cell wall metabolism, hormone signaling, photosynthesis and respiration. (Marschner 1995; Raven et al. 1999). However higher concentration of Cu causes oxidative damage in the plant cell, which affects plant growth and development. Thounaojam et al. (2012) also detailed about induction of oxidative stress in increased concentration of Cu in Oryza sativa. Inhibition of seed germination, root length and shoot length was reported in tomato, wheat and barley seedlings subjected to Cu stress conditions (Ashagre et al. 2013; Singh et al. 2007; Kalai et al. 2014). Peng et al. (2013) revealed that Cu deficiency leads to growth reduction, and decrease in photosynthetic parameters while excess of Cu cause reduced iron accumulation and decreased chlorophyll as well as carotenoid content in Elsholtzia splendens. Cu ions also decline photosynthetic gas exchange, net photosynthesis, florescence parameters and stomatal conductance in Aaviceania germinans (González-Mendoza et al. 2013). Similar decrease in PSII efficiency and increase in non-photochemical quenching was reported in cabbage (Wang et al. 2013) and decrease in chlorophyll pigment in presence of Cu was documented in Atriplex helimus as well as Camellia sinensis (Brahim and Mohamed 2011; Dey et al. 2014). Malfunctioning of photosynthetic machinery leads to the production of reactive oxygen species in the plants. Increased production of H2O2 and lipid peroxidation was reported in rice plants, alfa alfa, Solanum nigrum and Allium sativum (Meng et al. 2007; Thounaojam et al. 2012; Fidalgo et al. 2013; Hattab et al. 2013). Plants produce various enzymatic as well as non-enzymatic antioxidants to combat highly toxic reactive oxygen species produced during Cu stress. Increased activities of superoxide dismutase (SOD), peroxidases (POD), ascorbate peroxidase and glutathione reductase in response to excess Cu was documented in maize leaves (Tie et al. 2012). SOD and POD were also increased in Festuca arundinacea (Zhao et al. 2010) and catalase (CAT) activity was higher in the leaves of Allium sativum in presence of cu stress (Meng et al. 2007). To maintain the cellular homeostasis, plants produce osmoprotectants such as glycine betaine, sarcosine, proline etc. Increase in proline contents in Cu toxicity was seen in Triticum aestivum (Azooz et al. 2012), sunflower (Zengin and Kirbag 2007), and pigeonpea (Poonam et al. 2013). Cu stress in plants initiates auxin (Nomura et al. 2015), jasmonate (Ritter et al. 2008) abscisic acid as well as salicylic acid signaling (Wu et al. 2012; Yan and Dong 2014).
Pigeonpea [Cajanus cajan (L.) Millsp.] is a member of family Fabaceae and a major legume crop grown and consumed all over the world. It is extremely nutritious and have medicinal, toxicological, hepatoprotective, antisicklig hypolipidemic and antioxidant activities. (Osuagwu 2010; Ezike et al. 2010; Oyewole et al. 2010; Singh et al. 2011). Due to its wide-ranging cultivation pigeonpea may face different type of biotic and abiotic stresses. Therefore this study was carried out to investigate the effect of different concentrations of Cu on growth, photosynthesis, osmoprotectants and antioxidants properties of pigeonpea seedlings. This study could be helpful to understand the mechanism of Cu tolerance as well as toxicity in pigeonpea seedlings.
Materials and methods
Plant material and stress treatments
Pigeonpea (Cajanus cajan AL-201) seeds were purchased from Department of Plant Breeding and Genetics, Punjab Agriculture University, Ludhiana, India. Carefully chosen healthy seeds were treated with 5% hypochloride (v/v), a commercial fungicide for 5 min. These sterilized seeds were soaked DW as control, for 6 h and then grown in 0, 1, 5 and 10 mM concentrations of Cu in petriplates line with filter paper. Seeds of each treatment were sowed in triplicate and in 10 mL of DW or 10 mL of Copper sulphate pentahydrate at 24 °C under controlled condition (200 PAR light, 80% humidity and 16 ± 8 photoperiod). Fifteen days after sowing (DAS), seedlings were harvested, frozen in liquid nitrogen and stored at −80 °C until used.
Growth parameters
Different growth parameters include percentage germination, root length, shoot length, percentage biomass and moisture content were measured in seedling grown in Cu stress for 15 days.
Measurement of chlorophyll fluorescence
Junior PAM chlorophyll flurometer having Wincontrol software. (Walz, Effeltrich, Germany) was used for the analysis of chlorophyll florescence. Seedlings were kept in dark for 30 min to relax the reaction centres. Leaf of dark adapted seedlings was clamped on the stage and measurements were initiated by default parameters. The following parameters were calculated in the light as well as dark adapted states: coefficient of photochemical quenching (qP, qN), coefficient of non-photochemical quenching of variable florescence (qN), non-photochemical quenching (NPQ), maximum quantum efficiency of PSII photochemistry in the dark adapted state (Fv/Fm) and quantum yield (Y).
Measurement of photosynthetic pigments
Total chlorophyll, Chlorophyll a and chlorophyll b and total carotenoids were determined by Lichtenthaler (1987) method. These pigments were extracted using 80% acetone and their concentrations were determined spectrophotometrically (UV-160A Shimadzu, Japan).
Measurements of osmoprotectants
Total carbohydrates
Total carbohydrates were estimated according to method of Dubois (1956). Total carbohydrate was estimated at 485 nm using UV–visible spectrophotometer.
Total soluble sugars
Total sugars content was estimated by following Loewus (1952) method. Known weight of dried plant material was homogenised in 80% of ethanol and centrifuged at 3000×g for 15 min and the extract was collected for sugars estimation. For total sugars 0.05 ml of extract was diluted to 2 ml by distilled water and 3 ml cold anthrone reagent was added. Mixture was heated for 10 min and cooled. O.D. was recorded at 630 nm.
Reducing sugars and non-reducing
Reducing sugars and non-reducing sugars were estimated according to method of Nelson (1944) method by taking absorbance at 620 nm.
Proline
Proline was evaluated using Bates et al. (1973) method. Plant tissue was homogenized in 3% sulphosalicylic acid and centrifuged at 11,500×g. The homogenate was filtered followed by addition of glacial acetic acid and acid-ninhydrin. Sample was incubated at 100 °C for 1 h, toluene was added and absorbance was taken at 520 nm.
Glycine betaine
Plant tissue was mechanically shaken with 20 ml of deionized water. 2 N sulphuric acid was added to thawed extracts in 1:1 ratio and added cold potassium iodide-iodine reagent by following the method of Greive and Grattan (1983). Stored the reaction mixture at 0–4 °C for 16 h. The supernatant was removed carefully after centrifugation and the periodite crystals were dissolved in 9 ml of 1,2-dichloro ethane. Absorbance was measured at 365 nm.
Oxidative stress markers and antioxidant analysis
Malonaldehyde (MDA)
MDA content was measured according to the modified method of Heath and Packer (1968). One g fresh tissue was taken and ground in 10 ml of 0.5% TBA in 20% TCA. The mixture was incubated at 95º C in water bath for 30 min and quickly cooled in an ice bath. The homogenate was centrifuged and absorbance of the supernatant was read at 532 nm.
NADPH oxidase
NADPH oxidase activity was measured by using the method of Juan et al. (2012). The standard assay mixture was prepared by adding 0.5 ml enzyme extract to the 0.5 ml of 730 µM nitroblue tetra zolium (NBT), 1 ml of 100 µM NADPH solution. NBT reaction was determined by observing reduction at 530 nM.
Lipase
Lipase activity was measured by using the method of Winkler and Stuckman (1979). The standard assay mixture was prepared by adding 10 ml of isopropanol containing 4-nitrophenyl palmitate (pNPP) and 0.05 M phosphate buffer (pH 8.0) containing 207 mg of sodium deoxycholate and 100 mg of gum arabic. A total of 2.4 ml of freshly prepared substrate solution was then pre-warmed at 37 °C and mixed with 0.1 ml of enzyme solution. After incubation at 37 °C for 15 min, the absorbance was measured at 410 nm against an enzyme free control.
Lipoxygenase assay
Lipoxygenase activity was measured by using the method of Grossman and Zakut (1979). The standard assay mixture was prepared by adding 10 µl of linoleic acid or linolenic acid and 50 µl of tween 20 in 10 ml of 0.1 M phosphate buffer, pH 9.0. The reaction was started by the addition of 100 µl enzyme extract to the assay mixture. LOX activity was measured by monitoring the change in absorbance at 234 nm over a period of 2 min and activity was calculated.
Superoxide dismutase
Superoxide dismutase activity was measured by using the method of Kono (1978) method. To 0.5 ml of enzyme extract, 1.8 µl of 50 mM of Sodium Carbonate buffer (pH-10), 750 µl of 96 µM NBT and 150 µl Triton X-100 were added. The reaction was initiated by adding 0.4 ml of 1 mM hydroxylamine hydrochloride. Absorbance was taken at 540 nm using spectrophotometer mentioned elsewhere, and activity of SOD was taken as an increase in absorbance for 2 min at 25 °C.
Guaiacol peroxidase
Guaiacol peroxidase was assayed by mixing 50 µl of Guaiacol, 30 µl of H2O2 and 3 ml of potassium phosphate buffer (pH 7.0) and enzyme extract. Blank was prepared by adding all the reagents except enzyme extract by following the method of Putter (1974).
Catalase
Catalase activity was assayed by Aebi (1983) method by mixing H2O2, potassium phosphate buffer (pH 7.0) and enzymes extract.
Vitamin C
Vitamin C was estimated according to the Chinoy et al. (1976) method. 2 ml of plant extract was prepared and added to 8 ml of 2, 6-dichlorophenol indophenols dye. OD was recorded at 530 nm.
Vitamin E
Vitamin E was estimated by using Rosenberg (1992) method. Sample was mixed slowly with 0.1 N sulphuric acid and incubated at room temperature for overnight and then filtered. To the 1.5 ml of tissue extract, 1.5 ml of xylene added and centrifuged. Then 1.0 ml of xylene was separated and mixed with 1.0 ml of 2, 2-pyridyl and noted the absorbance at 460 nm. At the beginning, 0.33 ml FeCl3 was added in blank and mixed well. After 15 min, read the test and standard against the blank at 520 nm.
Antioxidant potential
The DPPH radical scavenging assay was performed as described by Miliauskas et al. (2004) with slight modifications. The kinetics of 400 µl aqueous extract in 2.8 ml of DPPH (80 µM in ethanol) were registered in 5 min by monitoring DPPH disappearance at 515 nm.
Statistical analysis
All analysis was done on a completely randomized design. All data obtained was subjected to one way analysis of variance (ANOVA) using Graphpad Prism 5.0 software. Each data was the mean of three replicates (n = 3) except for shoot and root length (n = 5) and comparisons of p-values < 0.05 were considered significant and different from control.
Results
Effect of Cu on growth
Percentage germination, root length and shoot length was decreased in different concentrations of Cu in a dose dependent manner and highest decrease of 34% in seed germination, 82.80% in root length and 45.92% in shoot length was observed in 10 mM Cu (Table 1). Biomass percentage was found to be increased in Cu stress treatment as compared to the control and highest increase was seen in 5 mM Cu stress. However, moisture content was decreased in various concentrations of Cu and highest decrease was 11% in 5 mM Cu stress.
Effect of Cu on photosynthetic pigments
Increase in total chlorophyll pigment was seen in different concentrations of Cu and highest increase was reported in 10 mM Cu up to 36.71% (Table 2). However, chlorophyll a was increased to 8.65 and 40.63% in 1 and 5 mM Cu and decreased to 7.45% in 10 mM Cu stress. Chlorophyll b content was increased to 29.77% in 5 mM Cu and decreased in 1 and 10 mM Cu. Total carotenoid content was also increased in 1 and 5 mM Cu up to 4.77 and 47.53%, respectively and decreased in 10 mM Cu to 3.51%.
Effect of Cu on Photosynthetic parameters
Decrease in qP and qN and Y was seen in pigeonpea seedlings in a dose dependent manner and highest decrease was seen in 10 mM Cu stress (Table 3). Fv/Fm was also decreased in these seedlings however highest decrease was seen in 5 mM Cu stress. Non photochemical quenching was increased in 1 mM Cu stress and decreased in 5 and 10 mM Cu in a dose dependent manner.
Effect of Cu on osmolytes
Increase in total carbohydrate content was seen in different concentration of Cu and highest increase of 63.0% was observed in 5 mM Cu stress (Table 4). In our study, total soluble sugars were also increased in Cu stress and highest increase was estimated in 5 mM Cu up to 54.6%. Reducing sugars were decreased in a dose dependent manner and highest decrease of 208.8% was seen in 10 mM Cu. However non-reducing sugars were highest in 51.71% in 5 mM Cu. Proline content was increased in different concentrations of Cu and highest increase of 428.87% was seen in 5 mM Cu stress. Glycine betaine content was reduced in 1 mM Cu stress to 21.2% and increased in 5 and 10 mM Cu to 90.18 and 94.9%, respectively.
Effect of Cu on oxidative stress markers
Regular increment in MDA was recorded in Cu treated seedlings from 1 mM Cu (14.84%) to 5 mM Cu (44.54%), which was slightly declined in 10 mM Cu (39.84%) treated seedlings (Table 5). Cu concentrations also affected the NADPH oxidase activity towards increasing side and highest of all time was recorded in 10 mM Cu where 80.2% additional NADPH oxidase activity was recorded than CN DDW seedlings. Different concentrations of Cu also enhanced LOX activity to many folds than CN DDW seedlings and LOX activity was 458.9, 479.4 and 441% more than CN DDW seedlings. Enhanced lipase activity was eventual from 1 mM Cu to 5 mM Cu and highest of all time was in 10 mM Cu treated seedlings.
Effect of Cu on antioxidants
SOD activity increased in a dose dependent manner in 1 and 5 mM Cu to 120.83 and 129.17%, respectively (Fig. 1a). However less increase of 41.67% was seen in 10 mM Cu stress while POD was not activated in different concentrations of Cu and decreased to 66.6% in 5 mM Cu (Fig. 1b). Activity of catalase was increased in different concentrations of Cu and highest increase was reported in 169.77% in 5 mM Cu stress (Fig. 1c). Vitamin C content was increased in 1 mM Cu up to 9.38% and decreased to 22.77% and 21.65 in 5 M and 10 mM Cu stress (Fig. 1d). Vitamin E content was increased to 145.83% in 5 mM Cu stress (Fig. 1e). Overall increase in antioxidant potential was observed in a dose dependent manner with highest increase in 10 mM Cu (Fig. 1f).
Discussion
Copper is a micronutrient and plays an important role in plant growth and development. In the present study, the inhibitory effect of toxic concentrations of copper has been observed in pigeonpea seedlings in a dose dependent manner. Similar decrease in seed germination, root length as well as shoot length was seen in Lycopersicon esculetum under Cu stress (Ashagre et al. 2013). Kalai et al. (2014) also reported decline in radicle and shoot length in barley seedlings due to decline in α amylase, acid phosphatase and alkaline phosphatase. Decrease in mitotic index was also reported in Allium sativum under Cu stress (Jiang et al. 2001). Biomass percentage was found to be increased in Cu stress treatment as compared to the control and highest increase was seen in 5 mM Cu stress. However moisture content was decreased in various concentrations of Cu and highest decrease was 11% in 5 mM Cu stress. Similar increase in growth and dry matter was reported in Vigna radiata (L.) under low concentration of Cu (Manivasagaperumal et al. 2011) while high concentration decreased growth and dry matter. In our study decrease in chlorophyll a, chlorophyll b and carotenoid content was reported in 10 mM Cu. Our results are in agreement with the results of Ali et al. (2015) showing decrease in chlorophyll a, b and carotenoid content in stone-head cabbage (Brassica oleracea var. capitata). In our study chlorophyll b was reduced in low as well as high concentration of Cu and in accordance with Peng et al. (2013) who documented the effect of Cu deficiency and excess on chlorophyll as well as carotenoids in Elsholtzia splendens plants. Decrease in qP and qN, Y and Fv/Fm was seen in pigeonpea seedlings in a dose dependent manner. However increase in non-photochemical quenching was observed in a dose dependent manner. These results are in agreement with Wang et al. (2013) who disclosed the reduction of photosynthetic efficiency by damaging photosystem and increasing non-photochemical quenching in non-heading chinese cabbage. Increase in total carbohydrate content was seen in different concentration of Cu. Rodríguez-Calcerrada et al. (2011) reported the role of soluble sugar as organic osmolyte, which increase tolerance to osmotic stress and protects against photo-damage. In our study total soluble sugars were also increased in Cu stress. Chai et al. (2014) reported similar increase in soluble sugars in Spartina alterniflora. Reducing sugars were decreased in a dose dependent manner. However the concentration of non-reducing sugars were increased. The concentrations of proline and glycine betaine, which play important role in maintaining osmotic concentrations of the cell, also were increased in the present study.
MDA content was increased which was found to be in accordance with Zhao et al. (2010) where increase in MDA content was reported in roots of turfgrass under copper stress. In the present study, increased NADPH oxidase and lipase activity demonstrated the unstable plasma membrane integrity. This study shows enhancement of lipoxygenase activity, which shows the initiation of jasmonates signalling under Cu stress. Similarly, Ritter et al. (2008) reported octadecanoid and eicosanoid biosynthesis in the brown algal kelp Laminaria digitata. Further increase in SOD activity was also reported in this study while POD was not activated in different concentrations of Cu. Similar decrease in POD activity was reported in Lolium perenne however increase in activities of SOD and POD was documented in Festuca arundinacea (Zhao et al. 2010). Activity of catalase was increased in different concentrations of Cu and highest increase was reported 5 mM Cu stress. On the contrary Hattab et al. (2013) reported decrease in the activities of CAT, GR and SOD in alfa alfa. We also observed increased content of vitamin C and vitamin E, which helps in decreasing oxidative stress in plants. Overall increase in antioxidant potential was observed in a dose dependent manner with highest increase in 10 mM Cu.
Conclusion
This study highlighted the relationship between osmoprotectants and oxidative stress tolerance in pigeonpea seedlings subjected to Cu stress conditions. Pigeonpea seedlings grown in 5 mM Cu stress contain highest concentration of proline, glycine betaine as well as sugars, which help in maintaining osmoregulation in the plant cell membrane and organelles from physical damage. Antioxidants activities such as superoxide dismutase, catalase, vitamin E also help to improve oxidative stress tolerance.
References
Aebi H (1983) Catalase. In: Bergmeyer HU (ed) Methods of enzymatic analysis. Verlag-Chemie, Weinham, pp 673–684
Ali S, Shahbaz M, Shahzad AN, Khan HAA, Anees M, Haider, Fatima A (2015) Impact of copper toxicity on stone-head cabbage (Brassica oleracea var. capitata) in hydroponics. Peer J 3:e1119
Ashagre H, Almaw D, Feyisa T (2013) Effect of copper and zinc on seed germination, phytotoxicity, tolerance and seedling vigor of tomato (Lycopersicon esculentum L. cultivar Roma VF). Int J Agric Sci Res 2:312–317
Azooz MM, Abou-Elhamd MF, Al-Fredan MA (2012) Biphasic effect of copper on growth, proline, lipid peroxidation and antioxidant enzyme activities of wheat (Triticum aestivum cv. Hasaawi) at early growing stage. Aust J Crop Sci 6:688–694
Bates LS, Waldren RP, Teare ID (1973) Rapid determination of free proline for water stress studies. Plant Soil 39:205–208
Brahim L, Mohamed M (2011) Effects of copper stress on antioxidative enzymes, chlorophyll and protein content in Atriplex halimus. Afr J Biotechnol 10:10143–10148
Chai M, Shi F, Li R, Qiu G, Liu F, Liu L (2014) Growth and physiological responses to copper stress in a halophyte Spartina alterniflora (Poaceae). Acta Physiol Plant 36:745–754
Chinoy JJ, Singh YD, Gurumurthi K (1976) The role of ascorbic acid in growth, differentiation and metabolism of plants. J Plant Physiol 22:122
Dey S, Mazumder PB, Paul SB (2014) Effect of copper on growth and chlorophyll content in tea plants (Camellia sinensis (L.) O. Kuntze). Int J Res Appl Natl Soc Sci 2:223–230
Dubois M, Gilles KA, Hamilton JK, Rebers PA, Smith F (1956) Colorimetric method for determination of sugars and related substances. Anal Chem 28(3):350–356
Ezike AC, Akah PA, Okoli CC, Okpala CB (2010) Experimental evidence for the antidiabetic activity of Cajanus cajan leaves in rats. J Basic Clin Pharmacol 1:25–30
Fidalgo F, Azenha M, Silva AF, Sousa A, Santiago A, Ferraz P, Teixeira J (2013) Copper-induced stress in Solanum nigrum L. and antioxidant defense system responses. Food Energy Secur 2:70–80
González-Mendoza D, Gil FEY, Escoboza-Garcia F, Santamaría JM, Zapata-Perez, OM (2013) Copper stress on photosynthesis of black mangle (Avicennia germinans). Acad Bras Cienc 85:665–670
Greive CM, Grattan SR (1983) Rapid assay for determination of water-soluble quaternary amino compounds. Plant Soil 70:303–307
Grossman K, Zakut R (1979) Determination of the activity of lipoxygenase. Methods Biochem Anal 25:303–329
Hattab S, Hattab S, Banni M, Hernández LE, Boussetta H (2013) Modulation of antioxidant responses of Medicago sativa under cadmium and copper stress. Afr J Agric Res 8:2297–2306
Heath RL, Packer L (1968) Photoperoxidation in isolated chloroplast: 1. Kinetics and stoichiometry of fatty acid peroxidation. Arch Biochem Biophy 125:189–198
Jiang W, Liu D, Liu X (2001) Effects of copper on root growth, cell division, and nucleolus of Zea mays. Biol Plant 44:105–109
Juan JH, Lu Z, Qing LH, Jia SK, Hao W, Sheng WY, Yuan HG, Rong TX (2012) Exogenous phytic acid inhibits early growth of wheat seedlings (Triticum aestivum L.) by decreasing superoxide anion derived from NADPH oxidase. Aust J Crop Sci 8:1221–1227
Kalai T, Khamassi K, da Silva JAT (2014) Cadmium and copper stress affect seedling growth and enzymatic activities in germinating barley seeds. Arch Agron Soil Sci 60:6
Kono Y (1978) Generation of superoxide radical during autooxidation of hydroxylamine and an assay for superoxide dismutase. Arch Biochem Biophys 186:189–195
Lichtenthaler HK (1987) Chlorophylls and carotenoids: pigments of photosynthetic biomembranes. In: Packer L, Douce R (eds) Methods in enzymology, vol 148. Academic Press, New York, pp 350–382
Loewus FA (1952) Improvement in the anthrone method for determination of carbohydrates. Ann Chem 2:219
Manivasagaperumal R, Balamurugan S, Thiyagarajan G, Sekar J (2011) Effect of zinc on germination, seedling growth and biochemical content of cluster bean (Cyamopsis tetragonoloba (L.) Taub). Curr Bot 2:11–15
Marschner H (1995) Mineral nutrition of higher plants, 2nd edn. Academic Press, London, p 889
Meng QM, Zou J, Zou JH, Jiang WS, Liu DH (2007) Effect of Cu2+ concentration on growth, antioxidant enzyme activity and malondialdehyde content in Garlic (Allium sativum L.). Acta Biol Crac Bot 49:95–101
Miliauskas G, Venskutonis PR, Van Beek TA (2004) Screening of radical scavenging activity of some medicinal and aromatic plant extracts. Food Chem 85:231–237
Nelson N (1944) A photometric adaptation of Somogyi method for the determination of glucose. J Biol Chem 153:315–380
Nomura T, Itouga M, Kojima M, Kato Y, Sakakibara H, Hasezawa S (2015) Copper mediates auxin signalling to control cell differentiation in the copper moss Scopelophila cataractae. J Exp Bot 66:1205–1213
Osuagwu CG (2010) Mechanism of the antisickling effects of Cajanus cajan and phenylalanine. Niger J Biochem Mol Biol 25:68–71
Oyewole O, Owoseni AA, Faboro EO (2010) Studies on medicinal and toxicological properties of Cajanus cajan, Ricinus communis and Thymus vulgaris leaf extracts. J Med Plant Res 4:2004–2008
Peng H, Kroneck PM, Küpper H (2013) Toxicity and deficiency of copper in Elsholtzia splendens affect photosynthesis biophysics, pigments and metal accumulation. Environ Sci Technol 47:6120–6128
Poonam S, Kaur H, Geetika S (2013) Effect of jasmonic acid on photosynthetic pigments and stress markers in Cajanus cajan (L.) Millsp. seedlings under copper stress. Am J Plant Sci 04:817–823
Putter J (1974) Peroxidase. In: Bergmeyer HU (ed) Methods of enzymatic analysis. Verlag Chemie, Weinham, pp 685–690
Raven JA, Evans MCW, Korb RE (1999) The role of trace metals in photosynthetic electron transport in 02- evolving organisms. Photosynth Res 60:111–150
Ritter A, Goulitquer S, Salaün JP, Tonon T, Correa JA, Potin P (2008) Copper stress induces biosynthesis of octadecanoid and eicosanoid oxygenated derivatives in the brown algal kelp Laminaria digitata. New Phytol 180:809–821
Rodríguez-Calcerrada J, Shahin O, Carmen del Rey C, Rambal S (2011) Opposite changes in leaf dark respiration and soluble sugars with drought in two Mediterranean oaks. Funct Plant Biol 38:1004–1015
Rosenberg HR (1992) Chemistry and physiology of vitamins. Inter Science Publishers Inc., New York, pp 452–453
Sharma RK, Agarwal M (2005) Biological effects of heavy metals: an overview. J Environ Biol 26:301–313
Singh A, Lawrencea K, Pandita S, Lawrenceb RS (2007) Response of leaves, stems and roots of Withania somnifera to copper stress. Int J Plant Anim Environ Sci 4:1–60
Singh S, Mehta A, Mehta P (2011) Hepatoprotective activity of Cajanus cajan against carbon tetrachloride induced liver damage. Int J Pharm Pharm Sci 3:3–4
Thounaojam TC, Panda P, Mazumdar P, Kumar D, Sharma GD, Sahoo L, Panda SK (2012) Excess copper induced oxidative stress and response of antioxidants in rice. Plant Physiol Biotech 53:33–39
Tie SG, Tang ZJ, Zhao YM, Li W (2012) Oxidative damage and antioxidant response caused by excess copper in leaves of maize. Afr J Biotech 11:4378–4384
Wang XH, Song YP, Ren J, Liu TK, Hou XL, Li Y (2013) Response of biomass and photosynthesis in non-heading chinese cabbage to excess copper. J Anim Plant Sci 23:1659–1665
Winkler UK, Stuckmann M (1979) Glycogen hyaluronate and some other polysaccharide greatly entrance the formation of exolipase by Serratia marcescens. J Bacteriol 138:663–670
Wu Y, Zhang D, Chu JY, Boyle P, Wang Y, Brindle ID (2012) The Arabidopsis NPR1 protein is a receptor for the plant defense hormone salicylic acid. Cell Rep 1:639–647
Yan S, Dong X (2014) Perception of the plant immune signal salicylic acid. Curr Opin Plant Biol 20:64–68
Yruela I (2005) Copper in plants. Braz J Plant Physiol 17:145–156
Zengin FK, Kirbag S (2007) Effects of Cu on chlorophyll, proline, protein and abscisic acid acid level of sunflower (Helianthus annuus L.) seedlings. J Environ Biol 28:561–566
Zhao S, Liu Q, Qi Y, Duo L (2010) Responses of root growth and protective enzymes to copper stress in turfgrass. Acta Biol Crac Bot 52:7–11
Acknowledgment
Authors are highly acknowledged to Department of Science and Technology, New Delhi (DST, New Delhi) for financial support in the form of Women Scientist Project (WOS-A); Head, Department of Botany, Punjabi University Patiala for providing experimental facility and Head, Plant Breeding and Genetics, Punjab Agriculture University, Ludhiana for providing certified seeds of pigeonpea.
Author information
Authors and Affiliations
Corresponding author
Rights and permissions
About this article
Cite this article
Sharma, P., Sirhindi, G., Singh, A.K. et al. Consequences of copper treatment on pigeon pea photosynthesis, osmolytes and antioxidants defense. Physiol Mol Biol Plants 23, 809–816 (2017). https://doi.org/10.1007/s12298-017-0461-8
Received:
Accepted:
Published:
Issue Date:
DOI: https://doi.org/10.1007/s12298-017-0461-8