Abstract
About 11% of the world’s primary energy consumption comes from biomass. However, a continuing material deficit indicates the need to find suitable timber for use as bioenergy. In this context, this study aims to determine some chemical and energetic properties, wood density, and fiber features of 10 Hevea brasiliensis clones, including Eucalyptus tereticornis and E. pellita species, to understand how the characteristics of studied woods might interfere with higher heating value (HHV) and determine if these woods and their residues would present potential for bioenergy. In general, HHV results corroborate those in the literature, e.g., E. pellita (16,502 kJ kg−1) lower value, and MT45 H. brasiliensis clone (19,757 kJ kg−1) higher value, and woods with higher extractive contents and lignin content, but lower holocellulose content, of wood pulp, in addition to denser woods with longer fibers and thicker walls, are woods considered to have higher heating values and, hence, indicated as potential woods for use in bioenergy. However, it is suggested that wood characteristics should be analyzed together to determine the most suitable material for use in bioenergy since a high value of one factor influencing HHV would not, in and of itself, establish suitability of the wood for bioenergy. Nonetheless, all wood samples could have their waste exploited for bioenergy since they range from 16,502 kJ kg−1 in E. pellita to 19,757 kJ kg−1 in the MT45 clone of H. brasiliensis.
Similar content being viewed by others
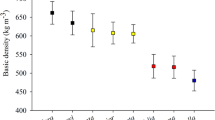
Avoid common mistakes on your manuscript.
Introduction
Based on data from the International Energy Agency, Vale and Gentil [1] report that about 11% of the world’s primary energy consumption comes from biomass and that such percentage may be higher in developing countries, particularly in the case of inaccurate official information. Most of this energy is generated directly by wood, or its residues, derived from industrial processes that use wood as a raw material. In general, the contribution of waste of different types to Brazilian energy balance is still relatively small, and available potential of agroforestry waste is not yet fully known [1].
Among the largest producers of forest derivatives in 2005, Brazil contributed 8% of the world’s production of wood for energy, behind only India (17%) and China (11%). In 2006, Brazil had the second largest area reforested by Eucalyptus in the world, behind only India. In summary, Brazil has, so far, met the exceptional demands of forest-based industries, and the country has developed technologies for genetic improvement and forest management that place Brazil among producers of forest products at the lowest cost and highest productivity. In addition, the country’s expansive lands and good soil and climatic conditions contribute to tree plantation and rapid forest growth. Additionally, it has a workforce with technological knowledge of forestry activities [2].
According to Nahuz [3], the industrial consumption of Pinus and Eucalyptus wood is mainly intended to supply the pulp and charcoal industry with raw material, especially for the steel industry. The Brazilian consumption of charcoal was 38 million m3 in 2005; however, a wood deficit of 200 thousand ha/year persists. In the case of Hevea brasiliensis, production in the state of São Paulo in 2015 reached 134,088 tons, which was harvested in an area of 60,358 ha [4]. Most of this production was located in the northwestern part of the state, and according to projections between 2039 and 2043, the goal is that more than 90,000 m3 of wood will be produced. Northwestern São Paulo state is one of the main furniture manufacturing hubs. The infrastructure of H. brasiliensis wood here not only impacts furniture manufacture, but also latex exploration and the use of wood residues for bioenergy [5]. The rubber crop has a productive cycle of about 30 years [6], and the demand for increased production for latex extraction is ongoing; however, this increase will also lead to the generation of waste that up to now has not found any practical uses. Once such purposes have been established, these residues could be disseminated to small and medium producers. Since (1) latex extraction is a priority and (2) silvicultural practices have not been adopted for the production of wood, all woody material can be used as biomass in the form of firewood or pellets. Thus, the large volume of H. brasiliensis wood produced suggests its potential use in bioenergy.
In the chain of planted forests, 75 to 90% of waste is generated throughout the production process [7]. Depending on waste volume, these residues can be processed into pellets (6–16 mm in diameter and 25–30 mm in length) or briquettes (50–100 mm in diameter and 250–400 mm in length). In addition to presenting higher heating value than firewood, pellets and briquettes have greater size and shape uniformity, greater ease of storage, and greater fire safety. These advantages over raw wood have increased growth in the supply of pellets and briquettes in the domestic market, as well as their export to European and Asian countries that have increased consumption, mainly for domestic heating and use in small and medium companies [8].
Such data highlight the need to find materials that will meet the demand for lumber and the demand for its waste as bioenergy. Quirino et al. [9] compiled information from higher heating value (HHV) and density of 240 woods, giving the scale of diversity of biomass production from wood residues. However, it is essential that the potential for biomass also be determined from planting conditions in which ages and spacing of trees are known so that accurate information is provided to producers. In the present study was chosen a Brazilian native species, Hevea brasiliensis. The species is very important in latex production, but at the end of latex exploration, few studies have assessed its potential for wood applications, and as mentioned above by Gonçalves [5], this species represents a large number of plantations in the state of São Paulo. Were also studied two species of Eucalyptus: E. tereticornis and E. pellita. Both were tested in the Forest Genetic Improvement Program of the Forest Institute to determine seed production and certify wood qualities and forest derivatives [10].
Hevea brasiliensis (Willd. ex A. Juss.) Müll. Arg (Euphorbiaceae) is a native species, not endemic to Brazil, with geographical distribution in the northern and northeastern regions of Brazil [11]. Eucalyptus tereticornis Sm. (Myrtaceae) presents extensive natural distribution over a range of about 100 km in width south of Papua, New Guinea, and in northern Queensland, south of Victoria, along the eastern coast of Australia. It was one of the first Eucalyptus trees exported from Australia, and it is currently grown all over the tropics on a large scale in both India and Brazil. The wood is used for construction, railway sleepers, and particleboard, as well as various wood products [12]. Eucalyptus pellita F. Muell (Myrtaceae) is a perennial tree with a large, heavily branched crown, and it can grow up to 40 m in height. The best specimens have a straight trunk up to half the total height of the tree and up to 100 cm in diameter. It has been planted for wood in many areas of the subtropics and tropics, including Papua, New Guinea, Indonesia, India, Kenya, the Congo, and Brazil. Owing to its wide crown, E. pellita is suitable for shade and windbreak. It is recommended for afforestation of coastal lands, and with its ornamental value, it is often planted in parks [13].
While these species are important for wood purposes, few studies have characterized their properties in homogeneous plantations with known spacing. Thus, this is a unique opportunity to increase knowledge about the chemical properties, wood density, and anatomy of a native species and two reforestation species. In this context, this study aims to determine some chemical and energetic properties, wood density, and fiber features of 10 Hevea brasiliensis clones, as well as Eucalyptus tereticornis and E. pellita, to understand how these characteristics might interfere with higher heating value (HHV) and determine if these woods and their residues represent potential for bioenergy.
Materials and Methods
Location and Sampling
Wood samples of Hevea brasiliensis were collected from 30 trees, three of each clones, in the municipality of Selvíria, Mato Grosso do Sul State (20° 20′ S, 51° 24′ W, elevation 350 m). The Hevea brasiliensis plantation was established in 2006 at a spacing of 3 × 3 m from seeds of free-pollinated clones (from crossing clones of RRIM600, PB235, IRCA111, IAC15, ROI110, IAC307, IAC44, FX3864, MT45, and 1-12-56-77). Soil in the experimental area was classified as Red Latosol, a clayey texture [14].
Wood samples of Eucalyptus tereticornis and Eucalyptus pellita were collected from 10 trees of each species in the municipality of Batatais, São Paulo State (47° 31′ S, 47° 21′ W, elevation 880 m) [15]. The E. tereticornis plantation was established in 1986 at a spacing of 3 × 2 m with seeds from Helenvale, Ravenshoe and Mt. Garnet, Australia. The E. pellita plantation was established in 1986 at a spacing of 4 × 4 m with seeds from Helenvale and Cole, Australia. Soil in the experimental area was classified as dystroferric Red Latosol (Oxisol) and dystrophic Red-Yellow Latosol, a medium texture [14].
In 2017, selected trees were felled and discs 10 cm in thickness from each tree at breast height (1.3 m from the ground) were cut. From each tree, a DBH disc (diameter at breast height—1.30 m) was obtained and from each disc, samples close to the bark were used to determine higher heating value, chemical constituents, wood density, and fiber dimensions (Fig. 1). Mean height and DBH are shown in Table 1.
Higher Heating Value
The samples were fragmented into smaller pieces with a hammer and chisel and milled in a micro mill. Higher heating value was determined after thermal rectification with dry samples. To perform the analysis, the isoperibolic method was used with an IKA C200 calorimeter, according to ASTM D5865-98 [16].
Chemical Assays
To determine extractives (EX) and lignin (LI) contents, TAPPI standards T204 (2004a) and T222 (2004b) were used, respectively. The samples were fragmented into smaller pieces with a hammer and chisel and milled in a micro mill. The resulting powder was sieved through 40 and 60 mesh screens, and the material retained on the last sieve was used for analysis. The analyses were sequential such that the extractives were first removed, then lignin by acid treatment and holocellulose content was calculated. For extractive contents, solutions of toluene: alcohol (2:1 v:v) and alcohol extractions were employed, at times exceeding 12 h in a Soxhlet extractor. For lignin, extractive-free powder was prepared in several stages with 72% sulfuric acid to obtain insoluble and soluble lignin (Cary 100 UV–visible spectrophotometer). Finally, the two values of lignin were added. Insoluble lignin (IL) content was determined as IL = [(DWlig) / (DW)] × 100, where DW = dry sawdust weight, and DWlig = dry weight of insoluble lignin. Soluble lignin (SL) filtrates were analyzed and the blank were read at two wavelengths (215 nm and 280 nm) using quartz cuvettes, soluble lignin content was determined as the SL = [4.53 × (L.215 – blank) – (L.280 – blank) / (300 × DW) × 100], where DW = dry sawdust weight. Ex and Li were expressed as a percentage (%) of oven-dry weight of unextracted wood. Then, the holocellulose (HO) content was determined as Ho = [100 − (Ex + Li)].
Density (ρ12)
Wood density was determined according to Glass and Zelinka [17], which consisted of evaluating the mass and volume at 12% moisture content (MC). Specimens of 2 cm × 2 cm × 3 cm were conditioned at constant temperature (21 °C) and 65% MC, respectively, and in these conditions, the mass was determined using an analytical balance, and the volume was estimated by means of measurements of their dimensions with a caliper.
Fiber Analysis
Fragments to prepare macerations were taken from same samples used for wood density measurements. These fragments were prepared according to the modified Franklin method [18], stained with aqueous safranin, and mounted in a solution of water and glycerin (1:1). Fiber measurements were performed on an Olympus CX 31 microscope equipped with a camera (Olympus Evolt E330) and a computer with image analyzer software (Image-Pro 6.3). Terminology followed the IAWA list [19]. Fiber length (FL), fiber diameter (FD), and fiber wall thickness (FWT) were evaluated.
Statistical Analysis
Descriptive statistical analysis was initially performed. This was followed by performing a normality test to observe the data distribution. For the comparison among Hevea brasiliensis clones and Eucalyptus species, a parametric analysis of variance (one-way analysis of variance) was applied. In the case of significant difference, Tukey’s test was applied to identify pairwise determinants of differences. Results with p < 0.001 were considered as significant. All statistical analyses were performed using the SigmaPlot software - Exact Graphs and Data Analysis - version 12.3 (Systat Software Inc., San Jose, CA, USA).
Results
Higher heating value oscillated among Hevea brasiliensis clones; therefore, it was not possible to identify any one clone standing out from the others. Eucalyptus tereticornis had higher HHV than E. pellita, which was the lowest value observed among all samples (Table 2). The RRIM 600 clone had higher content of extractives compared to the other clones, but did not differ from that of E. tereticornis and E. pellita, which, in turn, did not differ from each other (Table 2). In H. brasiliensis, higher lignin contents were observed in ROI110, PB235, IRCA111, IAC15, and FX3864. E. pellita had higher lignin content than E. tereticornis (Table 2). In H. brasiliensis, IAC44 and IAC307 had higher holocellulose content than RRIM600. E. tereticornis had higher holocellulose content than E. pellita (Table 2).
In H. brasiliensis, wood density was higher in RRIM600, IAC115, ROI110, IAC307, and FX3864. Also, among these clones and E. pellita, the highest density was observed in E. tereticornis (Table 2). Fiber length among H. brasiliensis clones did not show evident variations, but IAC44 had longer fibers than IAC15, FX3864, MT45, and 1-12-56-77. E. tereticornis had longer fibers than E. pellita (Table 2). Fiber diameter and fiber wall thickness showed a similar result with oscillation of values among H. brasiliensis clones, with IAC307 having wider and thicker fibers than MT45. E. tereticornis had larger fibers than E. pellita (Table 2).
Discussion
Apart from latex, H. brasiliensis produces a large quantity of biomass. For example, it is estimated that a standing tree (trunk, branches, and leaves) can produce up to 2.1 m3 of biomass [20]. E. tereticornis and E. pellita wood have low impact in the Brazilian timber trade, which, in part, results from the scant knowledge about its wood under planting conditions and trees older than 30 years, which is the case of present study. Thus, considering the need preserve forests and reduce dependence on fossil fuels for energy [20], studies to identify potential wood for energy from biomass are needed.
For decades, the mean value of HHV in wood ≈ 18,830 kJ kg−1 has been known [21]. Other studies report different values. For example, Phyllis [22] compiled data from many woods and HHV for hardwood species ranging from 17,384–23,052 kJ kg−1. Alakangas [23] reported values between 21,200 and 21,400 kJ kg−1 in wood from Finland. In another work compiled by Huhtinen [24], wood does not vary much from one tree species to another (18700–21,900 kJ kg−1), emphasizing that slightly higher values occur in coniferous compared to hardwood species. According to Dietenberger and Hasburgh [25], HHV for wood is about 20,000 kJ kg−1. Telmo and Lousada [26] studied 12 of the main wood species in Portugal, and among five industrial wood tropical residues, Eucalyptus globulus obtained the lowest HHV (17,631 kJ kg−1), while the highest HHV was obtained by Bowdichia nitida (20,809 kJ kg−1), a native species, but not endemic to Brazil [27]. As noted above, HHV values may vary between countries, and a consensus of the HHV ranges considered ideal may be adjusted according to demand and the species available. According to Brazilian market, values between 16,500 and 18,000 kJ kg−1 are suitable values for use in bioenergy.
Thus, while only small differences were observed among the ten H. brasiliensis clones, as well as differences between these clones and the two Eucalyptus species, the results indicate that all the studied woods have potential for bioenergy use since the results varied between E. pellita (16,502 kJ kg−1) at the lower limit and MT45 H. brasiliensis (19,757 kJ kg−1) at the higher limit. Quirino et al. [9] compiled HHV information and density of 240 woods, but without age or plant spacing data. The authors found an average value of 19,798 kJ kg−1 with the lowest HHV occurring in Eriotheca globosa (16,267 kJ kg−1) and the highest occurring in Mezilaurus itauba (22,007 kJ kg−1). Among the species studied were Hevea guianensis (18,765 kJ kg−1) from same genera of H. brasiliensis. Tan et al. [28] reported HHV of 19,700 kJ kg−1 in an experiment with H. brasiliensis, a value close to that of Werther and Saenger [29] who reported 18,410 kJ kg−1 for H. brasiliensis waste. Müzel et al. [30] reported HHV of 17,880 kJ kg−1 for Hevea brasiliensis RRIM600 and GT1 clones.
Between Eucalyptus species, Quirino et al. [9] reported HHV in E. tereticornis of 19,501 kJ kg−1 and E. pellita of 21,016 kJ kg−1. Pereira et al. [31] studied 10.5-year-old E. tereticornis and E. pellita planted at a spacing of 3 × 2 m in Uberaba, Minas Gerais. The authors reported HHV of 18,317 kJ kg−1 in E. tereticornis and 18,982 kJ kg−1 in E. pellita. In the present study HHV for E. tereticornis was 19,266 kJ kg−1, whereas the lowest HHV was for E. pellita at 16502 kJ kg−1, as mentioned above. Kumar et al. [32] studied the effect of tree age on calorific value and other fuel properties of Eucalyptus hybrid and reported that HHV increases with tree age. This could explain the values of E. tereticornis, but in E. pellita, other factors may be at play, such as spacing, as discussed below with reference to wood density. Lachowicz et al. [33] studied the influence of location, forest habitat type and tree age on basic fuel properties of Betula pendula, and found that all variables, except tree age, have significant influence on HHV, including location. Perhaps the difference between planting sites and possibly between progenies and seed origin may explain the differences in HHV in Eucalyptus species or among H. brasiliensis clones. Thus, it is obviously essential that industries using these materials for energy understand the potential of nearby plantations in order to decrease the value with transportation.
As higher HHV values were very close, it was tried to understand what wood characteristics most strongly influence HHV. In terms of the chemical constituents, Telmo and Lousada [26] and Günther et al. [34] reported that extractives and lignin content are positively related to HHV. Additionally, Bufalino et al. [35], in a study with Toona ciliata and Castro et al. [36], in a study with Eucalyptus clones, explain that extractives contribute to higher HHV values owing to their high calorific value. Similarly, higher lignin content correlates with higher HHV [37]. According to Pereira [31], high lignin values contribute to higher gravimetric yield of charcoal from higher resistance to thermal lignin degradation, which, according to Castro et al. [36], occurs from the presence of more condensed structures. In the case of holocellulose for charcoal production, the most suitable woods are those with lower lignin content, since the thermal degradation of holocellulose is faster than lignin, which is more resistant [38, 39]. Dietenberger and Hasburgh [25] reported different HHV for cellulose and hemicellulose (18.6 kJ kg−1), lignin (23.2–25.6 kJ kg−1), and extractives (32–37 kJ kg−1). These values highlight why higher extractives and lignin contents contribute more than holocellulose to increase of HHV.
In this context, woods with the greatest potential for bioenergy use would be those with comparatively higher extractives and lignin content, but lower holocellulose contents. In H. brasiliensis, RRIM600 (16.3%) and IAC307 (14.5%) showed higher extractives content, although IAC307 did not differ from MT45 (14.5%). Eucalyptus tereticornis (15.7%) and E. pellita (15.9%) also did not present differences compared with these values in H. brasiliensis clones. For lignin contents in H. brasiliensis, it was not detected a prominence among the highest values, but the IAC44 clone showed lowest lignin content (22.4%) and, consequently, highest holocellulose (64%). Thus, to select materials only for their chemical constituents, among H. brasiliensis clones, RRIM600 would be interesting for higher extractives content and one of the lowest contents of holocellulose, despite low lignin content. Between the two Eucalyptus species, E. pellita, despite lower HHV, showed highest lignin (38.8%) and lowest holocellulose content (45%) compared to E. tereticornis. According to lignin and holocellulose contents, Eucalyptus pellita would be more indicated for energy generation than E. tereticornis; however, the latter seems to be more indicated for energy generation, owing to higher density.
Another property that has a direct relationship with HHV is wood density. Studying coal production from lumber industry residues in northern Brazil, Silva et al. [2] mention that wood density can be used as one of parameters to select species with energy potential. Thus, when analyzing wood density as an indicator of HHV in H. brasiliensis, the selected clones would be RRIM600, IAC115, ROI110, IAC307, and FX3864. E. tereticornis had higher potential than E. pellita. It is known that silvicultural management can interfere with wood density. In H. brasiliensis, no difference in spacing occurred; therefore, spacing had no effect on wood properties among clones. The spacing in E. tereticornis was 3 × 2 m, whereas in E. pellita, it was 4 × 4 m. In a study with E. camaldulensis, Neto et al. [40] report spacing to be one of the factors that interferes with growth in diameter and, consequently, in wood properties. While larger spacing between trees produces high dry matter of shoot, as a result of greater growth in diameter, at reduced spacing, a greater production of biomass per area is realized since the number of plants per area is greater. Considering that density is positively related to HHV [7] is suggested that the potential of E. pellita wood for energy can be increased by reducing the planting spacing. Additionally, Quirino et al. [8] suggest that woods with lower densities can also be used in energy generation; however, the waste must be transformed. According to Telmo and Lousada [26] and Dias et al. [7], transformation of residues into pellets and briquettes increases HHV compared to raw wood. Moreover, high-density products are desirable in terms of transport, storage, and handling. Artemio et al. [41] studied pellets obtained from tropical species and concluded that high-density pellet values can positively affect energy.
Anatomical composition directly influences physical, mechanical, and chemical wood properties [42]. Thus, fiber dimensions must influence wood density, which, in turn, influences HHV. In general, since fibers are the most abundant cells in woods, especially in the aerial part, compared with roots, it is expected that fiber variations will affect HHV values, especially variations in fiber wall thickness, which directly reflects the quantity of material (wood). In the present study, fiber dimensions did not show significant differences among H. brasiliensis clones, and since HHV is determined to be promising for energy use in raw wood if the materials are transformed into briquettes or pellets, they will reach higher HHV. Fiber dimensions were determined in 10-year-old H. brasiliensis trees and ranged between 1097 and 1189 μm for FL, 26.9 and 28.5 μm for FD, and 3.1 and 5.1 μm for FWT. The values were close to those found in the literature. Naji et al. [43] studied radial variation of wood cell features under different stocking densities management of on 9-year-old H. brasiliensis clones, RRIM2020 and RRIM2025, and found values between 1187 and 1340 μm for FL, 25.6 and 31.8 μm for FD, and 3.5 and 4.7 μm for FWT, further reporting that these anatomical features are affected by planting density. Interestingly, fiber dimensions in 31-year-old Eucalyptus species were smaller when compared to H. brasiliensis. In Eucalyptus species, fiber dimensions in E. tereticornis ranged from 800 to 1183 μm for FL to 7.1–20.8 μm for FD and 1.4–7.3 μm for FWT. In E. pellita, fiber dimensions ranged from 555 to 1596 μm for FL to 11.7–17.5 μm for FD and 1.7–4.7 μm for FWT. Sreevani and Rao [44] reported 880–1130 μm for FL, 12.5–13.1 μm for FD, and 4.6–5.7 μm for 4.5-year-old E. tereticornis trees. Lukmandaru et al. [45] reported 860–1170 μm for FL, 10.8–17.0 μm for FD, and 2.1–4.0 μm for 9-year-old E. pellita trees. It is well known that variations between anatomical features may result from plant spacing, different progenies, or temperature and rainfall conditions. Thus, if wood selection were to be made by fiber dimensions, it would be safe to indicate H. brasiliensis clones as more promising than Eucalyptus species.
Extractives, lignin content and holocellulose content, wood density and cell size, and percentage all impact HHV. However, as seen from the results, an isolated characteristic is not sufficient to determine that a given material possesses the quality required for use in bioenergy. For example, E. pellita presented the highest lignin and lowest holocellulose content, which positively and negatively influence HHV, respectively. However, the wood density of E. pellita did not differ from, or was even higher than, many H. brasiliensis clones with higher HHV. Furthermore, E. pellita has shorter, narrower, and thinner wall fibers than other woods, which indirectly means lower mass and may negatively influence HHV. Thus, when considering all these factors together, E. pellita was considered to present the lowest HHV overall, indicating, in turn, that wood characteristics should be analyzed together to draw a conclusion about the most suitable material for use in bioenergy.
Conclusions
The results corroborate findings in the literature. In general, wood with elevated HHV and, therefore indicated as potentially useful in bioenergy, presents higher extractives and lignin content, lower holocellulose contents, higher wood density, longer fibers, and thicker walls. However, it is suggested that wood characteristics should be analyzed together to indicate a suitable material for use in bioenergy. In other words, a high value of only one of the factors influencing HHV does not guarantee that such wood is the most suitable material for bioenergy. All studied wood may have their waste exploited for bioenergy since they range from 16,502 kJ kg−1 in E. pellita to 19,757 kJ kg−1 in the MT45 clone of H. brasiliensis. Additionally, based on literature and experience is suggest that the qualities of wood herein examined for HHV can be further improved by silvicultural treatments, e.g., plantations with less spacing in E. pellita, and also by transforming their residues into pellets or briquettes.
References
Vale AT, Gentil LV (2008) Produção e uso energético de biomassa e resíduos agroflorestais. Tecnologias aplicadas ao setor madeireiro III Jerônimo Monteiro-ES 12:196–246. https://doi.org/10.5902/198050981702
Silva MG, Numazawa S, Araujo MM, Nagaishi TYR, Galvão GR (2007) Carvão de resíduos de indústria madeireira de três espécies florestais exploradas no município de Paragominas, PA. Acta Amazonica 2007 37(1):61–70. https://doi.org/10.1590/S0044-59672007000100007
Nahuz M (2007) Atividades industriais, usos e aplicações de madeiras no Brasil, com ênfase em Pinus e Eucalyptus. Tecnologias aplicadas ao setor madeireiro Jerônimo Monteiro–ES:159–208
IEA. Instituto de Economia Agrícola (2014) Metodologia de custos operacionais de rentabilidade e procedimentos do estudo, produção planta / ano. Available at: <http://www.iea.sp.gov.br/out/LerTexto.php?codTexto=13598>>. Access on 27/ago/2017
Gonçalves ECP, da Seringueira SCT (2010) A cultura da seringueira para o Estado de São Paulo. Campinas, CATI (Manual, 72), 90p
Martins A L (2010) Produção de mudas de qualidade: fundamental para formação do seringal. Casa da Agricultura, São Paulo, ano 13, n. 4: 14-15
Schneider VE, Peresin D, Trentin A C, Bortolin T A, Sambuichi R H R (2012) Diagnóstico dos Resíduos Orgânicos do Setor Agrossilvopastoril e Agroindústrias Associadas, Relatório de pesquisa. Brasília, DF: IPEA. Available at: <http://hbjunior19.files. wordpress.com/2012/11/120917_relatorio_residuos_organicos.pdf>. Access on: dez. 2012
Dias AC, Arroja L (2012) Environmental impacts of eucalypt and maritime pine wood production in Portugal. J Clean Prod 37:368–376. https://doi.org/10.1016/j.jclepro.2012.07.056
Quirino WF, Vale AT, Andrade AD, Abreu VLS, Azevedo ADS (2005) Poder calorífico da madeira e de materiais ligno-celulósicos. Revista da madeira 89(100):e106
Gurgel-Garrido LMA, Siqueira ACMF, Cruz SF, Romanelli RC, Ettori LG, Crestana CSM, Sato AS (1997) Programa de melhoramento genético florestal do Instituto Florestal. IF Série Registros 18:1–53
Cordeiro I, Secco R (2015) Hevea in Lista de Espécies da Flora do Brasil. Jardim Botânico do Rio de Janeiro. Available at: <http://floradobrasil.jbrj.gov.br/jabot/floradobrasil/FB22704>. Access on: 10/02/2019
Brink M (2008) Eucalyptus tereticornis Sm. In: Louppe D, Oteng-Amoako AA, Brink M (eds) Plant Resources of Tropical Africa, 7:262–274
Fern K, Fern A, Morris R, (2014) Useful tropical plants database. Available at: http://tropical.theferns.info/ access on: 10/02/2019
Santos HD, Jacomine PKT, Anjos LD, Oliveira VD, Oliveira JD, Coelho MR, Cunha TD (2006) Sistema brasileiro de classificação de solos. Rio de Janeiro 412
Zanata M (2009) Efeito do manejo na qualidade do solo e da água em microbacias hidrográficas, Batatais, SP. 2009. xiii, 79 f. Dissertação (mestrado) - Universidade Estadual Paulista, Faculdade de Ciências Agrárias e Veterinárias. Available at: <http://hdl.handle.net/11449/96987> Access on: 10/02/2019
American Society for Testing and Materials. D5865–98: standard test method for gross calorific value of coal and coke. Philadelphia, 1998. 10p
Glass SV, Zelinka SL (2010) Moisture relations and physical properties of wood. In: Ross R. (ed.). Wood handbook – wood as an engineering material. 100th ed. Madison: U.S. Department of Agriculture, Forest Service, Forest Products Laboratory, 2010. p. 4-1-4-19
Ruzin SE (1999) Plant microtechnique and microscopy, vol 198. Oxford University Press, New York
Wheeler EA, Baas P, Gasson PE (Eds.) (1989) IAWA list of microscopic features for hardwood identification, 219–332
Ratnasingam J, Scholz F (2009) Rubberwood: an industrial perspective. World Resources Institute, p 74
Franz FPK, Kuenzi EW, Stamm AJ (1975) Principles of wood science and technology. Springer-Verlag
Phyllis ECN (2012) Database for biomass and waste. Energy Research Centre of the Netherlands. Avaible in: https://phyllis.nl/
Alakangas E (2005) Properties of wood fuels used in Finland. VTT Processes, Project report PRO2/P2030/05 (Project C5SU00800), Technical Research Centre of Finland, Jyväskylä
Huhtinen, M. (2006). Wood biomass as a fuel. Finland. Available at: https://www.hnee.de/_obj/CD8F42A0-B396-40E7-9F36 FF60A55DEFF7/outline/5Eures_- WoodPropertiesPDF.pdf
Dietenberger M, Hasburgh L (2016) Wood products thermal degradation and fire. Reference Module in Materials Science and Materials Engineering:1–8
Telmo C, Lousada J (2011) Heating values of wood pellets from different species. Biomass Bioenergy 35:2634–2639. https://doi.org/10.1016/j.biombioe.2011.02.043
Cardoso, DBOS (2019) Bowdichia in Flora do Brasil 2020 em construção. Jardim Botânico do Rio de Janeiro. Available at: <http://floradobrasil.jbrj.gov.br/reflora/floradobrasil/FB22835>. Access on: 05 Fev. 2019
Tan AG (1989) Pyrolysis of rubberwood-a laboratory study. J Trop For Sci 1(3):244–254
Werther J, Saenger M, Hartge EU, Ogada T, Siagi Z (2000) Combustion of agricultural residues. Prog Energy Combust Sci 26(1):1–27. https://doi.org/10.1016/S0360-1285(99)00005-2
Müzel SD, De Oliveira KA, Hansted FAS, Prates GA, Goveia D (2014) Wood calorific power from Eucalyptus grandis and Hevea brasiliensis species. Revista Brasileira de Engenharia de Biossistemas 8(2):166–172
Pereira JCD, Sturion JA, Higa AR, Higa RCV, Shimizu JY (2000) Características da madeira de algumas espécies de eucalipto plantadas no Brasil. Embrapa Florestas, Colombo, 113p
Kumar R, Pandey KK, Chandrashekar N, Mohan S (2010) Effect of tree-age on calorific value and other fuel properties of Eucalyptus hybrid. J For Res 21(4):514–516. https://doi.org/10.1007/s11676-010-0108-x
Lachowicz H, Sajdak M, Paschalis-Jakubowicz P, Cichy W, Wojtan R, Witczak M (2018) The influence of location, tree age and forest habitat type on basic fuel properties of the wood of the silver birch (Betula pendula Roth.) in Poland. BioEnergy Research, 1–14. https://doi.org/10.1007/s12155-018-9926-z
Günther B, Gebauer K, Barkowski R, Rosenthal M, Bues CT (2012) Calorific value of selected wood species and wood products. Eur J Wood Wood Prod 70(5):755–757. https://doi.org/10.1007/s00107-012-0613-z
Bufalino L, de Paula PT, Couto AM, Nassur OAC, de Sá VA, Trugilho PF, Mendes LM (2012) Caracterização química e energética para aproveitamento da madeira de costaneira e desbaste de cedro australiano. Pesquisa Florestal Brasileira 32(70):129–121. https://doi.org/10.4336/2012.pfb.32.70.13
Castro JF, Parra C, Yáñez-S M, Rojas J, Teixeira Mendonça R, Baeza J, Freer J (2013) Optimal pretreatment of Eucalyptus globulus by hydrothermolysis and alkaline extraction for microbial production of ethanol and xylitol. Ind Eng Chem Res 52(16):5713–5720. https://doi.org/10.1021/ie301859x
Demirbaş A (2001) Biomass resource facilities and biomass conversion processing for fuels and chemicals. Energy Convers Manag 42(11):1357–1378. https://doi.org/10.1016/S0196-8904(00)00137-0
Gomes Costa T, Bianchi ML, de Paula Protásio T, Trugilho PF, Júnior Pereira A (2014) Qualidade da madeira de cinco espécies de ocorrência no cerrado para produção de carvão vegetal. Cerne, 20(1). https://doi.org/10.1590/S0104-77602014000100005
Gani A, Naruse I (2007) Efeito do conteúdo de celulose e lignina sobre as características de pirólise e de combustão para diversos tipos de biomassa. Renew Energy 32(4):649–661. https://doi.org/10.1016/j.renene.2006.02.017
Neto Oliveira SND, Reis Ferreira MDG, Neves Lima JC (2003) Produção e distribuição de biomassa em Eucalyptus camaldulensis Dehn em resposta à adubação e ao espaçamento Revista Árvore, 27(1)
Artemio CP, Maginot NH, Serafín CU, Rahim FP, Guadalupe RQJ, Fermín CM (2018) Physical, mechanical and energy characterization of wood pellets obtained from three common tropical species. PeerJ 6:e5504. https://doi.org/10.7717/peerj.5504
Hoadley RB (2000) Understanding wood: a craftsman’s guide to wood technology. Taunton press
Naji HR, Sahri MH, Nobuchi T, Bakar ES (2013) Radial variation of wood cell features under different stocking densities management of two new clones of rubberwood (Hevea brasiliensis). J Wood Sci 59(6):460–468. https://doi.org/10.1007/s10086-013-1357-z
Sreevani P, Rao RV (2013) Variation in basic density, fibre and vessel morphology of Eucalyptus tereticornis Sm. clones. Int J Sci Technol Res 2(7):99–102
Lukmandaru G, Zumaini UF, Soeprijadi D, Nugroho WD, Susanto M (2016) Chemical properties and fiber dimension of Eucalyptus pellita from the 2nd generation of progeny tests in Pelaihari, South Borneo, Indonesia. J Korean Wood Sci Technol 44(4):571–588. https://doi.org/10.5658/WOOD.2016.44.4.571
Acknowledgments
The authors thank Sonia G. Campião and Juraci A. Barbosa from the Instituto Florestal, São Paulo, Brasil; Erick P. Amorim from the Programa de Pós-Graduação em Planejamento e Uso de Recursos Renováveis - UFSCAR/Sorocaba, Brasil; and Regina M. Buch from the Laboratório de Química, Celulose e Energia, Escola Superior de Agricultura Luiz de Queiroz (ESALQ) and Universidade de São Paulo (USP), Piracicaba, Brasil, for laboratory assistance.
Author information
Authors and Affiliations
Corresponding author
Additional information
Publisher’s Note
Springer Nature remains neutral with regard to jurisdictional claims in published maps and institutional affiliations.
Rights and permissions
About this article
Cite this article
Menucelli, J.R., Amorim, E.P., Freitas, M.L.M. et al. Potential of Hevea brasiliensis Clones, Eucalyptus pellita and Eucalyptus tereticornis Wood as Raw Materials for Bioenergy Based on Higher Heating Value. Bioenerg. Res. 12, 992–999 (2019). https://doi.org/10.1007/s12155-019-10041-6
Published:
Issue Date:
DOI: https://doi.org/10.1007/s12155-019-10041-6