Abstract
The metropolitan areas act as incubators of new knowledge, and play a central role in the process of scientific knowledge production. On the basis of highly cited papers data, this paper adopts spatial scientometrics and social network analysis to investigate the geography, position and link of science cities between 2007 and 2017. The results are demonstrated below: (1) The two seemingly paradoxical trends, the regional concentration and global spread, coexist in the process of knowledge production, which are rapidly reshaping the global pattern of science. (2) The whole knowledge collaboration network has been dominated by the Global North cities, while the rise of the Global South cities has an increasing influence in the network, both driving the evolution of the world order. (3) The number of scientific collaborations between cities has increased dramatically, while domestic collaborations have higher strength than international collaborations. Finally, we discuss the limitations of this study and set out three directions in the future research agenda of knowledge production.
Similar content being viewed by others
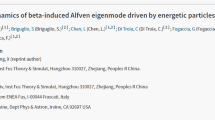
Avoid common mistakes on your manuscript.
Introduction
Over the past decades, scientific knowledge production has been paid large attention in economic geography (Heimeriks & Boschma, 2014), urban studies (Grossetti, et al., 2014; Lambooy, 2002) and information science (Csomos, 2018; Jack, et al., 2021). Due to the availability of scientific publication data, a number of literature have focused on examining country and organizational level (Csomos, 2019). However, the globalization of economy associates with the rise to prominence of cities and the relative decline in the significance of nation-states (Bunnell, 2002). Castells (1989) emphasizes that cities should be regarded as the key actors in the age of globalizing knowledge economy. In recent years, a growing number of scholars focus on the increasingly important role of cities or metropolitan areas as a driver for scientific knowledge production (Balland, et al., 2020; Cohen, et al., 2016; Nomaler, et al., 2014).
Cities and metropolitan areas are not only containers for research activities, but are actively involved in the creation of new knowledge (Florida, et al., 2017). Generally speaking, large cities are the hotbeds for the formation of ecosystems of knowledge production (WIPO, 2019), because cities attract diverse talents, including entrepreneurs, scientists and researchers, and act as incubators for new knowledge (Duranton & Puga, 2001). The city, as a lab, is becoming a platform for the knowledge economy (Cohen, et al., 2016). Most of the world’s science and research is highly concentrated in a few megacities. Therefore, scientific knowledge production is an urban enterprise. According to 2018 Revision of World Urbanization Prospects, 68% of the world’s population will live in urban areas by 2050 (United Nations Population Division, 2018). Cities, especially leading metropolises, are projected to become the domain of knowledge (Mallapaty, 2017). Some scholars claim that cities are also knowledge centers (Csomos & Toth, 2016; Matthiessen and Schwarz, 1999).
Against the above-mentioned background, the aim of this study is to further our understanding of the changing geography of scientific knowledge production on the metropolitan area level. This study will answer the following three questions: (1) Concentration or de-concentration? How do the geography of science cities evolve over time? (2) The Global North or Global South? How do the position of science cities change over time? (3) Domestic or international collaborations? How do the link of science cities evolve over time? With the globalization of science as the background of the research and the metropolitan area as the unit of analysis, this study uses publication data from the Web of Science database to measure scientific knowledge production. By applying spatial scientometrics and social network analysis, we investigate the dynamics in the geography of scientific knowledge production, the position of cities in the collaboration network, and the bilateral links between cities.
This article aims to make three contributions. Firstly, this paper uses metropolitan area as the unit of analysis, because metropolitan areas can provide rich and internationally comparable information on scientific knowledge production (Csomos & Toth, 2016; Matthiessen, et al., 2010). Although some scholars have paid attention to spatial scientometrics on the city level (Bornmann & de Moya, 2019; Csomos, 2018), there is a lack of research on the metropolitan area level. Csomos (2019) highlights that metropolitan area is an ideal solution, which can lead to consensus among scholars and make research results comparable as well as reliable. Secondly, scientific knowledge production is entering the collaborative era, and an increasing majority of scientific papers are the result of collaboration (Wagner, 2018). While much has been written about the spatial distribution of knowledge production, there remains a gap in our understanding of the scientific collaborations between cities (Csomos, et al., 2020; Maisonobe, et al., 2016). Thirdly, this paper tries to unite economics, and economic geography to tentatively explain the changing geography of knowledge production. The nature of scientific knowledge production is complex because it is not only subject to a linear input-output process, but also a path-dependent and interactive process (Bathelt, et al., 2004; Heimeriks & Boschma, 2014), which means that geography has always played a critical role in the process of scientific knowledge production.
The rest of the article is organized as follows: Sect. 2 provides a literature review. Data and methodology are introduced in Sect. 3. Section 4 presents the empirical results and findings. Conclusions are drawn in the final section.
Literature Review
The Nature of Scientific Knowledge Production
The nature of scientific knowledge production provides opportunities but also sets constraints for knowledge diffusion, and determines in part the geography of scientific activity. However, there is no consensus about the nature of knowledge production among scholars. In economic thinking, knowledge production is often described as a linear input-output process (Jaffe, 1989). Inspired by Cobb-Douglas production function, knowledge production function theory is first proposed by Griliches (1979). The theory implicitly argues that the functional relationship between knowledge output and knowledge input is linear, that is, knowledge output is heavily dependent on knowledge input, such as research and development (R&D) expenditure and R&D human capital. Therefore, the knowledge production function is also called ‘the linear model of knowledge’. A plenty of literature use the knowledge production function model to confirm the positive effect of R&D inputs (Huallachain & Leslie, 2007; Ponds et al., 2010), which is widely used to explain the inequalities of scientific knowledge. Scientific knowledge production is geographically uneven and concentrated in a relatively small number of metropolitan areas, because those large cities can offer research expenditure, highly skilled talents, and the best environment to cultivate new knowledge. In recent years, a growing number of cities have heavily increased its investment in R&D, leading to the global spread of knowledge.
In evolutionary economic geography, knowledge production is often seen as a cumulative and path-dependent process (Dosi, 1982; Nelson & Winter, 1982), emphasizing the significance of internal knowledge bases. The core hypothesis is that new knowledge stems from combining and recombining the existing knowledge elements (Schumpeter, 1934; Weitzman, 1998). From an evolutionary perspective, the previous scientific knowledge provides keystones for further knowledge production. Consequently, the new knowledge is not random, which is constrained by the existing knowledge (Boschma, et al., 2014). In addition, the cumulative and path-dependent nature of knowledge is more likely to result in the place-dependent (Heimeriks & Boschma, 2014). This explains why large metropolitan areas produce a growing number of scientific knowledge and the spatial concentration of scientific activities has increased.
Different from economics and evolutionary economic geography, relational economic geography sees knowledge production as an interactive process, which highlights the importance of the external interaction. Specifically, knowledge production is all best understood as the outcome of interactive learning processes where actors come together to solve particular problems (Bathelt, et al., 2004), that is, new knowledge is the result of interaction between actors (Kline & Rosenberg, 1986). Scientific knowledge as a public good, has the non-rivalrous and non-excludable properties (Arrow, 1962), which mean that the collaborators can benefit from openness. In the process of sharing, the public good nature of knowledge, which anyone can use without loss of value. Consequently, collaboration is increasingly the norm for knowledge production (WIPO, 2019). The current science has benefited from the shift from individual work to collective effort, with over 90% of the high-quality research produced by collaborations in the 21st century (Dong, et al., 2017). Scientific collaboration is increasingly common and frequent, which happen not only on the local level but also on the global scale. However, the tacit nature of knowledge require face-to-face interaction, so it does not easily flow over long distance (Gertler, 2003).
The Geography of Knowledge Production
When it comes to the geography of knowledge production, there are two different views. One stream of the literature point out that knowledge production is regional concentration. A growing body of studies show that knowledge production is highly concentrated in a tiny fraction of cities (Balland, et al., 2020; Florida, 2004). In terms of scientific publication, global science is geographically uneven. The traditional scientific monopolies (the USA and Europe) have dominated knowledge output (Royal Society, 2011), and the science hotspots are spatially clustered in three major geographic regions: North America, East Asia and Western Europe (Csomos, 2018). The high-quality research output also concentrates disproportionately in a few large cities. According to Nature Index 2017 Science Cities, the top 100 metropolises gather 60% of the share of authorship, and the top 10 cities are responsible for 17% of the research output (Mallapaty, 2017). Based on the 74 largest metropolitan units, Matthiessen et al. (2010) show that knowledge production presents a concentration process, London and Tokyo–Yokohama are giants.
Another stream emphasizes that knowledge production is increasingly global spread, because science is happening in an increasing number of countries and cities (WIPO, 2019). Scientific knowledge is undergoing a gradual process of de-concentration, and the gap between cities is narrowing, which leads to a more balanced distribution (Grossetti, et al., 2014; Maisonobe, et al., 2018). Furthermore, the mean center of gravity of global knowledge production is moving from the West to the East (Gui, et al., 2019a). The rise of emerging scientific nations are reshaping the global scientific landscape, and try to create a multipolar world order (Royal Society, 2011). Evidence from 2.8 million highly-cited scientific papers, Maisonobe et al. (2018) reveal a more even spread case: the number of urban areas with at least one paper has increased dramatically from 3,180 cities in 171 countries in 2000 to 4,841 ones in 190 nations in 2013. WIPO (2019) also confirms knowledge production has greater international spread in the last two decades. On the basis of publication data over the period 1987–2007, Grossetti et al. (2014) find that scientific activity is deconcentrating both global level and within countries. Taking knowledge production in biotech 1986–2008 for example, Heimeriks and Boschma (2014) find that the slow growing cities are mainly located in USA, while a number of those fast growing cities are found in Asian, particularly in South Korea and China.
Knowledge Collaboration and Intercity Linkage
Scientific knowledge production is entering the collaborative era, and we create and share knowledge—in collective effort (Adams, 2013; Wagner, 2018). Collaboration is increasingly the norm in the process of knowledge production, and has a significant impact on the organization, patterns and flows of knowledge. Informal or formal collaborations contribute to knowledge diffusion over long distances, even cross-border flows (Ponds et al., 2010). Knowledge collaboration as a typical type of inter-city linkage, provides a new pathway to understand the relationship between cities and the evolution of city system (Li & Phelps, 2018). Cities are embedded in various links and networks, which are crucial to understand the nature of cities (Jacobs, 1969). As Gottmann (1976) puts it, metropolitan areas not only act as the incubator of knowledge at the regional level, but also play the role of the hub in linking national innovation system to global innovation network. As science globalization develops, scientific collaborations between cities have dramatically increased, and the proportion of single-city publications has declined (Maisonobe, et al., 2016). Cities in developed countries are more actively integrated into the international network than counterparts in emerging economies. Matthiessen et al. (2010) find the rise of south-east Asian cities and the decline of the Anglo-American ones in the world cities of knowledge network. Taking ICT R&D network for example, Nepelski and De Prato (2015) report that the whole network is dominated by the US and European cities. At the same time, Chinese cities gradually occupy key positions in the network. Csomos et al. (2020) explore the changing geographical pattern of international scientific collaborations between cities, and indicate that the intensity of intercity collaboration has increased. Studies of Greater Bay Area (GBA) megalopolis and Yangtze River Delta (YRD) megalopolis show that Hong Kong and Shanghai play the hub role in the national scale, while relatively weak role in the local scale (Li & Phelps, 2018; Ma et al., 2021).
Data and Methods
Data Sources and Collection
The spatial scientometrics has been concerned with analyzing spatial aspects of the science system, which offers researchers a powerful methodological toolkit for investigating the spatial distribution, spatial biases and citation impact of scientific activities (Frenken, et al., 2009). This study uses spatial scientometrics to collect knowledge output data. To track the changing patterns of high-quality knowledge output in the sciences across the globe, highly cited papers are selected for their exceptional influence. Highly cited papers refer to document types that rank top 1% by citations for the research field and publication year. Although the highly cited papers only represent a very small fraction of knowledge production around the world, they have significant and broad influence across several fields. Our data is acquired from Web of Science database, which provides the list of highly cited papers during the last decade. A total of 148,583 papers in the period 2007–2017 are downloaded on March 15, 2018. The annual number of highly cited papers shows a steady increase from 10,524 to 2007 to 16,033 in 2017. It is noted that Web of Science database is dominated by Anglo-American journals and English-speaking publications. Given the known language bias, the analytical results may underestimate some cities in non-English regions, like Japan and French. Therefore, the conclusion may have certain limitations to some extent.
The following steps are taken: first, we use Python and BibExcel to extract the address (city and country) information from the publication data. It is noted that cities in the worldwide cannot be directly compared because they are in different analysis scales. Metropolitan area is a suitable unit and measured by urban populations and built-up urban areas, which can make research outcomes more comparable (Csomos, 2019). Therefore, we select Demographia World Urban Areas (DWUA) 2017 Revision, which annually publishes metropolitan area lists that contain consistently defined entities (DWUA, 2017). Second, following the approach of Matthiessen et al. (2010), Csomos and Toth (2016), we aggregate small cities and towns into metropolitan areas. For example, Cambridge, MA, USA and Medford, MA, USA are incorporated into Boston metro, USA. The paper uses article count (AC) to measure knowledge output, that is, a metropolitan area is given an AC of 1 for each article having at least one author from that metropolitan area. The metropolitan area is counted only once. If a city cannot be incorporated into any list, then it will be kept only when it has more than 10 highly cited papers published in a calendar year. Finally, City-by-city collaboration matrix is constructed with Python, where diagonal cell values denote the number of co-authored papers. The outline of the research methods is shown in Fig. 1.
Social Network Analysis
Social network analysis (SNA) is a promising tool for revealing cities’ power and position in the system, which is widely employed in city network (Alderson & Beckfield, 2004; Sigler & Martinus, 2017). We select degree centrality measure, which is the most straightforward and classic measure of node’s position, and indicate the number of direct partners of a city in the network, which is measured by the following equation:
where Di is the degree centrality of city i in the network, Dij is 1 if city i and j are partnership, otherwise 0.
Results
The Geography of Science Cities
Our study starts with exploring the spatial evolution of scientific knowledge production in the world. Figure 2 shows geographical distribution of science cities in 2007 and 2017, and Table 1 lists the top 20 metropolitan areas. Comparing between two years produces some interesting results.
First, knowledge production is regional concentration, and intensely concentrated in a few large metropolitan areas. This is supported with the following two reasons. One is that the spatial concentration of scientific knowledge has increased over time. In 2007, Boston was the world’s largest city with 985 highly-cited papers, whose share of the global was 9.35%. The New York metropolitan area, was the top science city globally, with 1, 508 highly-cited papers in 2017, accounting for a 9.41% share of the world. There is clear evidence that the dominance of the primary city has been consolidated, accounting for a much higher percentage. Within many countries, the production share of the top science city has grown. For example, London’s national share of highly-cited publications increased from 36.2 to 42.1% for the period 2007–2017.Another one is that the fastest-growing cities still tend to be large metropolitan areas, such as Beijing, New York and London, which indicates that the growth of scientific knowledge disproportionately concentrates in a few large cities. Between 2007 and 2017, Beijing, New York, London, Boston, Shanghai and San Francisco-San Jose each increased their production of highly-cited publications by more than 400 papers. Why is knowledge production regional concentration? From an evolutionary economic geography perspective, the cumulative nature of knowledge is likely to lead to the place-dependent and spatial concentration (Heimeriks & Boschma, 2014). Scientific knowledge accumulates in metropolitan areas and is spatially sticky (Markusen, 1996), because the metropolitan areas provide opportunities for the transmission of tacit knowledge and face-to-face contact. In addition, the complexity of knowledge production has increased. Balland et al. (2020) suggest that the spatial concentration of scientific activities increases with their complexity.
Second, knowledge production is increasingly globally dispersed. Two main facts can illustrate this phenomenon. One is that the geography of knowledge production has significantly expanded, which indicates a de-concentration process (Grossetti, et al., 2014; Maisonobe, et al., 2018). There were 590 science cities that came from 111 countries in 2007. By 2017, the number of cities publishing at least one highly-cited paper had increased to 930, in 147 countries, which confirms that knowledge production is taking place in more metropolitan areas. In addition, there is a narrowing gap between the primate city and other cities in research production (Table 1). These changes suggest a more balanced distribution of scientific knowledge. Another one is that the spatial dispersal of knowledge production is accelerating, which is rapidly reshaping the landscape for science, particularly the rise of the Asia-Pacific cities. In 2007, the top 200 cities were mainly located in two geographic regions: 39.5% of cities in North America and 44.5% in Europe. The top 20 metropolitan areas included 15 North American cities and three European cities (Table 1). While a bipolar world was substituted by a tri-polar pattern in which knowledge production was dominated by North America, Europe and Asia Pacific in 2017. Cities from these three regions accounted for 31%, 42.5%, and 22% of the top 200 cities, respectively. The top 20 consist of 11 North American cities, 4 European cities and 5 Asia Pacific cities. In terms of the number of cities in top 200, China is the second only behind USA. Beijing becomes the fifth largest city, and Shanghai ranks 11th. As an emerging scientific country, China has heavily increased its investment in science since the 1990s, with spending growing by 20% per year (Royal Society, 2011). The long-term government investments is one of the key drivers of China’s research rise (Xie, et al., 2014). From an evolutionary approach, knowledge production is a path-dependent process of branching, and the new science hotspots also appear (Heimeriks & Boschma, 2014).
The Position of Science Cities
This section focuses on the position of individual city in the collaboration network. Figure 3 describes the topological structure of the collaboration network, in which nodes denote cities whose sizes are directly proportional to degree centrality. The color of a node is determined by the continent to which the city belongs to. Circle pack layout algorithm in Gephi software is used to visualize the graph, which brings most connected nodes to the center and less connected ones to the margin of the graph. Two conclusions can be drawn.
First, the knowledge collaboration network is dominated by the Global North cities, and the traditional science hotspots have occupied central positions. In 2007, New York had the highest centrality and was the core of the network. Boston and London were ranked the second and the third, respectively. Beijing, was the only emerging city in the top 20 (Table 2), while others were almost located in established economies. In 2017, there were 18 cities from developed countries, suggesting that the dominance of the Global North cities remained strong in the network. London had the largest number of partner, and became the most connected city, followed by New York, Boston and San Francisco-San Jose. In sum, the Global North cities, such as New York, London, Paris and Boston, ranked at the top, which confirms them as the center of knowledge collaboration. Our finding corroborates the ‘North-South’ division in the world city network through the lens of advanced producer service firms (Derudder & Taylor, 2018). From a relational perspective, knowledge production is a result of the interactive learning processes, and cities are embedded in the scientific collaboration networks (Bathelt, et al., 2004). According to social network theory and social capital theory, the core network position is more likely to result in the better performance (Borgatti, et al., 2009). Therefore, the Global North cities gain more the benefits of collaboration due to preferential attachment in network science (Barabasi & Albert, 1999). The Global North cities are more likely to become the hub for communication and collaboration.
Second, the Global South cities have strengthened the centrality and influence in the network, and tried to move from the periphery to the core. The most forceful evidence is that Beijing and Moscow ranked amongst the top 20 cities globally. Beijing collaborated with 705 cities, making it one of the most connected nodes (from thirteen to three) in the network. Moscow rose from 39th to 19th. In addition, fast growth cities in degree centrality are chiefly located in developing countries, such as China, South Africa, and India, implying that there is a strong diffusion of knowledge from developed countries to developing countries. Between 2007 and 2017, a growing number of the Global South cities actively participate in global collaboration network. A stark example is that three cities exhibit very fast growth, especially Kuala Lumpur, Johannesburg and Jeddah. The rise of the Global South cities may be attributed to the public good nature of knowledge, and benefits from the openness in science (WIPO, 2019). As Keller (2004) points out, accessing international knowledge is crucial for the Global South to achieve catch-up strategy target. The Global South cities are making a significant impact and tries to challenge the dominance of the Global North ones.
The Link of Science Cities
Moving from city to tie city-pair, Fig. 4 illustrates spatial evolution of inter-city collaboration network. Cities are sized by the number of highly cited papers and connected to each of their collaborators. The width of each line represents the size of knowledge collaboration. Given space constraints, only the top 5% of links are presented. Two interesting findings stand out.
First, domestic ties have dominated the high strength of bilateral collaboration, because the top inter-city collaborations take place in the same country. In the top 10 bilateral partnerships, 9 city pairs took place in the USA in 2007, and this value was 8 in 2017. Interestingly, the Nature Index science cities (2018) shows that the top city links chiefly takes place in their national boundaries. Boston and New York are the top collaborators in the network, followed by New York and San Francisco-San Jose, Boston and San Francisco-San Jose. In addition, 70% of city links belonged to domestic collaboration in the top 100 city pairs, and 62% in 2017. Most of these top city pairs are found in the same country, and two cities within national boundaries are more likely to frequently collaborate, which reflects that knowledge collaboration production is ‘nationalized’ than ‘internationalized’ (Derudder & Liu, 2016). Some factors can partially explain the limited number of international interactions in the top city pairs: distance decay and administrative barriers. According to the first law of geography and the gravity law, the global diffusion of scientific knowledge faces distance-decay effects (Lengyel, et al., 2020; Tobler, 1970). It is widely accepted that the frequency of collaboration increases with a decrease in geographical distance (Hoekman, et al., 2009; Pan, et al., 2012), especially tacit knowledge. In addition, national boundaries also play an important role in the process of scientific collaboration, because research institutions in the same country follow the similar institutional and organizational framework, and easily acquire national research funding (Cappelli & Montobbio, 2016; Defazio, et al., 2009).
Second, inter-national ties have absolute predominance in low strength of bilateral collaboration. The number of internationally urban connections have increased dramatically from 20,058 to 2007 to 101,311 in 2017. At the same time, the percentage of international city links has grown from 82.49 to 92.15%. The increasing trends suggest that there is a process of an ongoing internationalization of scientific knowledge collaboration. However, the number of links with value equal to 1 account for 35% of the total, suggesting that the majority of international collaborations have low strength and are casual interactions. The link between London and New York has the largest magnitude of international alliance, confirming the presence of ‘NY-LON’ in the worldwide knowledge collaboration. In 2007, New York and London, collaborating 83 co-publications, and were the only international city pair in the top 10. New York and London were also the biggest international collaborations between two cities in 2017. The prominence of the New York–London axis has been referenced in advanced produce services firms (Taylor, et al., 2014). The number of international links has greatly increased over time, which can be attributed to the globalization of science (Gui, et al., 2019b). Scientific knowledge production is driven by international collaborations (Adams, 2013). From all corners of the globe, researchers come together to address some of the most pressing global challenges of our time and tackle the big questions in science (Royal Society, 2011).
Conclusions and Discussion
Based on highly cited papers data on the metropolitan area level, this paper uses a spatial bibliometric approach and social network analysis to explore the worldwide spatial evolution of high-quality knowledge output in the period 2007–2017. Drawing on the literature on economics and economic geography, this study tentatively furthers our understanding of the nature of scientific knowledge production.
Firstly, the geography of scientific knowledge production presents the two seemingly paradoxical trends: regional concentration and global spread. On the one hand, knowledge production is geographically uneven and intensely concentrated in a limited number of metropolitan areas, such as New York, London, Beijing and Paris. On the other hand, knowledge production is increasingly global, occurring in more and more cities, especially in Asia-Pacific cities. The two different but complementary trends, geographical concentration and dispersal, are found in scientific knowledge production (Sassen, 2011). The ‘concentration’ terminology does not fully capture the dynamics of the knowledge production. Concentration and dispersion phenomena coexist, which are rapidly reshaping the global landscape of knowledge production. In other words, the global spread of knowledge has been accompanied at city level by increased concentration within a few large metropolitan areas (WIPO, 2019).
Secondly, the Global North cities have occupied central position, while the rise of the Global South cities have gradually increasing influence in the network. On the one hand, the degree centrality ranking confirms dominance of the Global North cities in scientific collaboration. About 90% cities among the top 20 come from the established economies, such as London, New York, Boston, San Francisco-San Jose and Paris, which have a number of partners, and became the most connected nodes in the network. Cities located in developed countries are more actively integrated into the international collaboration than counterparts in developing countries. On the other hand, the Global South cities are rising, including Beijing, Moscow, Seoul, Sao Paulo, Shanghai and Cape Town. Those cities can be classified as ‘fast growing’ in degree centrality, and the number of partners has increased dramatically.
Thirdly, domestic links are dominant in high strength of collaboration, while many of international collaborations are weak. More than two out of three inter-city collaborations belong to intra-national links in the top 100 city pairs. The collaboration between New York and Boston is the largest inter-city links. There is limited international interaction in knowledge production, in spite of the rise of collaboration networks. Inter-city collaborations are heavily skewed towards domestic interaction, because the top collaborating links are located in the same country. It is noted that this tendency towards being inward looking shows a gradually decline in the period 2007–2017. As the rise of network, international collaborations are rapidly expanding. However, the majority of international collaborations have low strength and are casual interactions.
Despite that this paper is interesting, we have to acknowledge the limitations of this research. First, knowledge production is an abstract concept, so that it is difficult for scientific papers alone to fully capture the dynamics of the knowledge production. At the same time, not all scientific activities necessarily result in the publication of research articles (Royal Society, 2011). In addition, the highly cited papers data is updated regularly and may change in the following periods, and only represent the part of knowledge production. Second, Web of Science database are highly skewed towards English-speaking journals and Anglo-American journals, conforming to the fact that contemporary academic paradigm is dominated by Anglo-American hegemony (Aalbers, 2004; Derudder & Liu, 2016). Therefore, our finding would be very careful. Third, this study uses article count, to calculate the number of highly cited papers on the metropolitan area level, which means that the same article can contribute to the AC of multiple metropolitan areas. The fractional count takes into the share of authorship on each article account, and provides more real information (Csomos, 2019; Maisonobe, et al., 2016).
With regard to future research agenda, there is three directions to be done. First, knowledge production is dynamic: the emergence of new research topics and the disappearance of existing topics in cities. For a better interpretation of knowledge production, our future work will investigate the evolution of knowledge trajectories in cities. Recent examples include studies by Boschma et al. (2014) and Nomaler et al. (2016) on scientific knowledge dynamics in biotech cities as well as technological trends of inventive clusters. Second, knowledge production take place not just at city level but at regional, national, and global scale. Due to spatial reflexivity, a multi-scale analysis may provide novel insights. Third, the new and advanced approaches are used to address the challenges of spatial scientometrics on the city level, such as machine learning and artificial intelligence (Csomos, 2019; Fortunato, et al., 2018). These new tools may significantly improve the reliability of research results.
References
Aalbers, M. B. (2004). Creative destruction through the anglo-american hegemony: A non-anglo-american view on publications, referees and language. Area, 36(3), 319–322.
Adams, J. (2013). The fourth age of research. Nature, 497(7451), 557–560.
Alderson, A. S., & Beckfield, J. (2004). Power and position in the world city system. American Journal of Sociology, 109(4), 811–851.
Arrow, K. (1962). Economic welfare and the allocation of resources for invention. In R. R. Nelson (Ed.), The rate and direction of inventive activity: Economic and social factors (pp. 609–626). Princeton, NJ: Princeton University Press.
Balland, P. A., Jara-Figueroa, C., Petralia, S. G., Steijn, M. P. A., Rigby, D. L., & Hidalgo, C. A. (2020). Complex economic activities concentrate in large cities. Nature Human Behaviour, 4(3), 248–254.
Barabasi, A. L., & Albert, R. (1999). Emergence of scaling in random networks. Science, 286(5439), 509–512.
Bathelt, H., Malmberg, A., & Maskell, P. (2004). Clusters and knowledge: Local buzz, global pipelines and the process of knowledge creation. Progress in Human Geography, 28(1), 31–56.
Borgatti, S. P., Mehra, A., Brass, D. J., & Labianca, G. (2009). Network analysis in the social sciences. Science, 323(5916), 892–895.
Bornmann, L., & de Moya-Anegon, F. (2019). Spatial bibliometrics on the city level. Journal of Information Science, 45(3), 416–425.
Boschma, R., Heimeriks, G., & Balland, P. A. (2014). Scientific knowledge dynamics and relatedness in biotech cities. Research Policy, 43(1), 107–114.
Bunnell, T. (2002). Cities for nations? Examining the city-nation-state relation in information age Malaysia. International Journal of Urban and Regional Research, 26(2), 284–298.
Cappelli, R., & Montobbio, F. (2016). European integration and knowledge flows across european regions. Regional Studies, 50(4), 709–727.
Castells, M. (1989). The informational city: Information technology, economic restructuring and the urban-regional process. Oxford: Blackwell.
Cohen, B., Almirall, E., & Chesbrough, H. (2016). The city as a lab: Open innovation meets the collaborative economy. California Management Review, 59(1), 5–13.
Csomos, G. (2018). A spatial scientometric analysis of the publication output of cities worldwide. Journal of Informetrics, 12(2), 547–566.
Csomos, G. (2019). On the challenges ahead of spatial scientometrics focusing on the city level. Aslib Journal of Information Management, 72(1), 67–87.
Csomos, G., & Toth, G. (2016). Exploring the position of cities in global corporate research and development: A bibliometric analysis by two different geographical approaches. Journal of Informetrics, 10(2), 516–532.
Csomos, G., Vida, Z. V., & Lengyel, B. (2020). Exploring the changing geographical pattern of international scientific collaborations through the prism of cities. Plos One, 15(11), e0242468.
Defazio, D., Lockett, A., & Wright, M. (2009). Funding incentives, collaborative dynamics and scientific productivity: Evidence from the EU framework program. Research Policy, 38(2), 293–305.
Derudder, B., & Liu, X. J. (2016). How international is the Annual Meeting of the Association of American Geographers? A social network analysis perspective. Environment and Planning A, 48(2), 309–329.
Derudder, B., & Taylor, P. J. (2018). Central flow theory: Comparative connectivities in the world-city network. Regional Studies, 52(8), 1029–1040.
Dong, Y., Ma, H., Shen, Z. (2017). A century of science: Globalization of scientific collaborations, citations, and innovations. Proceedings of the 23rd ACM SIGKDD international conference on knowledge discovery and data mining, 1437–1446.
Dosi, G. (1982). Technological paradigms and technological trajectories - A suggested interpretation of the determinants and directions of technical change. Research Policy, 11(3), 147–162.
Duranton, G., & Puga, D. (2001). Nursery cities: Urban diversity, process innovation, and the life cycle of products. American Economic Review, 91(5), 1454–1477.
DWUA (2017). Demographia World Urban Areas (Built-Up Urban Areas or World Agglomerations), 13th Annual Edition. Available at: http://www.demographia.com/db-worldua.pdf (accessed 28 August 2017).
Florida, R. L. (2004). The rise of the creative class, revised Paperback Edition. New York, NY: Basic Books.
Florida, R., Adler, P., & Mellander, C. (2017). The city as innovation machine. Regional Studies, 51(1), 86–96.
Fortunato, S., Bergstrom, C. T., Borner, K., Evans, J. A., Helbing, D., Milojevic, S. (2018). Science of science. Science, 359(6379).
Frenken, K., Hardeman, S., & Hoekman, J. (2009). Spatial scientometrics: Towards a cumulative research program. Journal of Informetrics, 3(3), 222–232.
Gertler, M. S. (2003). Tacit knowledge and the economic geography of context, or the undefinable tacitness of being (there). Journal of Economic Geography, 3(1), 75–99.
Gottmann, J. (1976). Megalopolitan systems around the world. Ekistics, 109–113.
Griliches, Z. (1979). Issues in assessing the contribution of research and development to productivity growth. The Bell Journal of Economics, 92–116.
Grossetti, M., Eckert, D., Gingras, Y., Jegou, L., Lariviere, V., & Milard, B. (2014). Cities and the geographical deconcentration of scientific activity: A multilevel analysis of publications (1987–2007). Urban Studies, 51(10), 2219–2234.
Gui, Q. C., Liu, C. L., Du, D. B., & Duan, D. Z. (2019a). The changing geography of global science. Environment and Planning A-Economy and Space, 51(8), 1615–1617.
Gui, Q., Liu, C., & Du, D. (2019b). Globalization of science and international scientific collaboration: A network perspective. Geoforum, 105, 1–12.
Heimeriks, G., & Boschma, R. (2014). The path- and place-dependent nature of scientific knowledge production in biotech 1986–2008. Journal of Economic Geography, 14(2), 339–364.
Hoekman, J., Frenken, K., & van Oort, F. (2009). The geography of collaborative knowledge production in Europe. Annals of Regional Science, 43(3), 721–738.
Huallachain, B. O., & Leslie, T. F. (2007). Rethinking the regional knowledge production function. Journal of Economic Geography, 7(6), 737–752.
Jack, P., Lachman, J., & Lopez, A. (2021). Scientific knowledge production and economic catching-up: An empirical analysis. Scientometrics, 126(6), 4565–4587.
Jacobs, J. (1969). The economy of cities. New York, NY: Vintage.
Jaffe, A. B. (1989). Real effects of academic research. American Economic Review, 79(5), 957–970.
Keller, W. (2004). International technology diffusion. Journal of Economic Literature, 42(3), 752–782.
Kline, S. J., & Rosenberg, N. (1986). An overview of Innovation. In R. Landau, & N. Rosenberg (Eds.), The positive Sum Strategy: Harnessing Technology for Economic Growth. Washington, DC: National Academy Press.
Lambooy, J. G. (2002). Knowledge and urban economic development: An evolutionary perspective. Urban Studies, 39(5–6), 1019–1035.
Lengyel, B., Bokanyi, E., Di Clemente, R., Kertesz, J., & Gonzalez, M. C. (2020). The role of geography in the complex diffusion of innovations. Scientific Reports, 10(1), 15065.
Li, Y. C., & Phelps, N. (2018). Megalopolis unbound: Knowledge collaboration and functional polycentricity within and beyond the Yangtze River Delta Region in China, 2014. Urban Studies, 55(2), 443–460.
Ma, H. T., Li, Y. C., & Huang, X. D. (2021). Proximity and the evolving knowledge polycentricity of megalopolitan science: Evidence from China’s Guangdong-Hong Kong-Macao Greater Bay Area, 1990–2016. Urban Studies, 58(12), 2405–2423.
Maisonobe, M., Eckert, D., Grossetti, M., Jegou, L., & Milard, B. (2016). The world network of scientific collaborations between cities: Domestic or international dynamics? Journal of Informetrics, 10(4), 1025–1036.
Maisonobe, M., Jegou, L., & Cabanac, G. (2018). Peripheral forces. Nature, 563(7729), S18–S19.
Mallapaty, S. (2017). Metropolis of minds. Nature, 550(7676), S157–S157.
Markusen, A. (1996). Sticky places in slippery space: A typology of industrial districts. Economic Geography, 72(3), 293–313.
Matthiessen, C. W., & Schwarz, A. W. (1999). Scientific centres in Europe: An analysis of research strength and patterns of specialisation based on bibliometric indicators. Urban Studies, 36(3), 453–477.
Matthiessen, C. W., Schwarz, A. W., & Find, S. (2010). World Cities of Scientific Knowledge: Systems, Networks and potential Dynamics. An analysis based on Bibliometric indicators. Urban Studies, 47(9), 1879–1897.
Nature Index (2018). Nature Index 2018 Science Cities. Available at: https://www.nature.com/nature-index/supplements/nature-index-2018-science-cities (accessed 25 August 2020).
Nelson, R. R., & Winter, S. G. (1982). An evolutionary theory of economic change. Cambridge, MA: The Belknap Press.
Nepelski, D., & De Prato, G. (2015). Corporate control, location and complexity of ICT R&D: A network analysis at the city level. Urban Studies, 52(4), 721–737.
Nomaler, O., & Verspagen, B. (2016). River deep, mountain high: Of long run knowledge trajectories within and between innovation clusters. Journal of Economic Geography, 16(6), 1259–1278.
Nomaler, O., Frenken, K., & Heimeriks, G. (2014). On scaling of scientific knowledge production in US Metropolitan Areas. Plos One, 9(10), e110805.
Pan, R. K., Kaski, K., & Fortunato, S. (2012). World citation and collaboration networks: uncovering the role of geography in science. Scientific Reports, 2.
Ponds, R., van Oort, F., & Frenken, K. (2010). Innovation, spillovers and university-industry collaboration: An extended knowledge production function approach. Journal of Economic Geography, 10(2), 231–255.
Royal Society. (2011). Knowledge networks and nations: Global scientific collaboration in the 21st century. London: The Royal Society.
Sassen, S. (2011). Cities in a World Economy (4th Ed).). Thousand Oaks, CA: Sage Publications.
Schumpeter, J. A. (1934). The theory of economic development: An inquiry into profits, capital, credit, interest, and the business cycle. Cambridge, MA: Harvard University Press.
Sigler, T. J., & Martinus, K. (2017). Extending beyond “world cities’’ in World City Network (WCN) research: Urban positionality and economic linkages through the Australia-based corporate network. Environment and Planning A, 49(12), 2916–2937.
Taylor, P. J., Derudder, B., Faulconbridge, J., Hoyler, M., & Ni, P. F. (2014). Advanced producer service firms as Strategic Networks, Global Cities as Strategic Places. Economic Geography, 90(3), 267–291.
Tobler, W. R. (1970). A computer movie simulating urban growth in the Detroit region. Economic geography, 46(sup1), 234–240.
United Nations Population Division. (2018). The world population prospects: 2018 revision. New York, NY: United Nations.
Wagner, C. S. (2018). Collaborative era in Science. London: Palgrave Macmillan.
Weitzman, M. L. (1998). Recombinant growth. Quarterly Journal of Economics, 113(2), 331–360.
WIPO. (2019). The geography of innovation: Local hotspots, global networks world intellectual property report. Geneva: World Intellectual Property Organization.
Xie, Y., Zhang, C., & Lai, Q. (2014). China’s rise as a major contributor to science and technology. Proceedings of the National Academy of Sciences, 111(26), 9437–9442.
Acknowledgements
This work is supported by the Chenguang Program of Shanghai Education Development Foundation and Shanghai Municipal Education Commission (21CGA29), China Postdoctoral Science Foundation (2022M711152), Ministry of Education of Humanities and Social Science Project (22YJC790032), and Major Program of National Social Science Foundation of China (21ZDA011).
Author information
Authors and Affiliations
Additional information
Publisher’s Note
Springer Nature remains neutral with regard to jurisdictional claims in published maps and institutional affiliations.
Rights and permissions
Springer Nature or its licensor (e.g. a society or other partner) holds exclusive rights to this article under a publishing agreement with the author(s) or other rightsholder(s); author self-archiving of the accepted manuscript version of this article is solely governed by the terms of such publishing agreement and applicable law.
About this article
Cite this article
Gui, Q., Du, D. & Liu, C. The Changing Geography of Scientific Knowledge Production: Evidence from the Metropolitan area Level. Appl. Spatial Analysis 17, 157–174 (2024). https://doi.org/10.1007/s12061-023-09525-y
Received:
Accepted:
Published:
Issue Date:
DOI: https://doi.org/10.1007/s12061-023-09525-y