Abstract
Neurogenesis plays an important role in adult hippocampal function, and this process can be modulated by intracellular calcium. The activation of transient receptor potential vanilloid 4 (TRPV4) induces an increase in intracellular calcium concentration, but whether neurogenesis can be modulated by TRPV4 activation remains unclear. Here, we report that intracerebroventricular injection of the TRPV4 agonist GSK1016790A for 5 days enhanced the proliferation of stem cells in the hippocampal dentate gyrus (DG) of adult mice without affecting neurite growth, differentiation, or survival of newborn cells. GSK1016790A induced increases in the hippocampal protein levels of cyclin-dependent kinase (CDK) 6, CDK2, cyclin E1, and cyclin A2 but did not affect CDK4 and cyclin D1 expression. The phosphorylation of retinoblastoma protein (Rb) in hippocampi was enhanced in GSK1016790A-injected mice compared with control mice. Moreover, hippocampal protein levels of extracellular signal-regulated kinase 1/2 (ERK1/2) and p38 mitogen-activated protein kinase (p38 MAPK) phosphorylation were enhanced by GSK1016790A. Finally, GSK1016790A-enhanced proliferation was markedly blocked by a MAPK/ERK kinase or p38 MAPK antagonist (U0126 or SB203580, respectively). The increased protein levels of CDK2 and CDK6, as well as those of cyclin E1 and cyclin A2, in GSK1016790A-injected mice were substantially reduced by co-injection of U0126 or SB203580. We conclude that TRPV4 activation results in the proliferation of stem cells in the adult hippocampal DG, which is likely mediated through ERK1/2 and p38 MAPK signaling to increase the expression of CDKs (CDK6 and CDK2) and cyclins (cyclin E1 and A2), phosphorylate Rb consequently, and accelerate the cell cycle ultimately.
Similar content being viewed by others
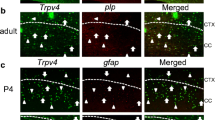
Avoid common mistakes on your manuscript.
Introduction
In the hippocampus, endogenous neurogenesis continues throughout adulthood in the subgranular zone (SGZ) of the hippocampal dentate gyrus (DG) [1]. Adult hippocampal neurogenesis is a complex process that begins with the proliferation of stem cells, followed by commitment to a neuronal phenotype, with newborn neurons finally integrating into the hippocampal circuitry after 3 to 4 weeks of morphological and physiological maturation. Neurogenesis plays an important role in adult hippocampal function, and alterations in neurogenesis have been found in neurological diseases such as neurodegenerative diseases, depression, and temporal lobe epilepsy [2]. During adult neurogenesis, the proliferation of stem cells is a key event that can be modulated by endogenous and exogenous factors [2]. Calcium (Ca2+) is an important second messenger that regulates many cellular processes, including cell proliferation, development, migration, invasion, and survival [3, 4]. Ca2+ influx has been shown to control cell growth and proliferation in several cell types. In the central nervous system, Ca2+ entry through L-type voltage-dependent calcium channels is involved in the hypoxia-induced proliferation of neural progenitor cells [5]. Ca2+-permeable glutamate receptors are important in precursor cell proliferation and neuronal motility [6]. Therefore, targeting Ca2+-permeable channels may modulate cell proliferation and thereby provide an effective strategy for the regulation of neurogenesis.
Transient receptor potential vanilloid 4 (TRPV4), a member of the vanilloid transient receptor potential (TRP) channel family, is selectively permeable to Ca2+ [7]. TRPV4 activation has been reported to increase the intracellular free Ca2+ concentration ([Ca2+]i) in various cell types. Furthermore, TRPV4 activation, accompanied by elevations in [Ca2+]i, participates in the regulation of human brain capillary endothelial cell proliferation and triggers collateral vessel growth during the regeneration of arterial circulation [8, 9]. Activation of TRPV4, together with TRPV1, is involved in the proliferation and migration of pulmonary arterial smooth muscle cells and is dependent on increases in [Ca2+]i [10]. Activation of transient receptor potential canonical 1 (TRPC1), another Ca2+-permeable member of the TRP superfamily, has also been shown to induce the proliferation of neural progenitor cells in the adult hippocampus [11]. However, TRPV4 activation has also been found to selectively inhibit tumor endothelial cell proliferation as well as the transforming growth factor-β1-induced proliferation of hepatic stellate cells [12, 13]. Currently, it is still unclear whether TRPV4 activation can modulate the proliferation of stem cells in the adult hippocampal DG.
Previously, we found that TRPV4 activation enhanced the activation of mitogen-activated protein kinase (MAPK) (including extracellular signal-regulated kinase 1 and 2 (ERK1/2) and p38 MAPK) signaling pathways which are involved in the modulation of cell proliferation, differentiation, and survival [14, 15]. Here, we investigated whether TRPV4 activation can modulate adult hippocampal neurogenesis and further explored the mechanism underlying TRPV4 action.
Materials and Methods
Experimental Animals
Male mice (9–10 weeks old at the start of the experiment; ICR, Oriental Bio Service Inc., Nanjing, China) were used in the experiments. Animals were housed under standard conditions (room temperature 23 ± 2 °C, humidity 55 ± 5 %, 12:12-h light/dark cycle) in the animal research center of Nanjing Medical University and were permitted free access to food and water. All animal experiments were approved by the Animal Experimental Committee of Nanjing Medical University. Each experimental group contained nine mice.
Drug Treatment
Drugs were intracerebroventricularly (icv.) injected as previously reported [14, 15]. Drugs were first dissolved in DMSO and then in 0.9 % saline for a final volume of 2 μl with a DMSO concentration of 1 %. Drugs were injected into the right lateral ventricle (0.3 mm posterior, 1.0 mm lateral, and 2.5 mm ventral to the bregma) using a stepper-motorized microsyringe (Stoelting, Wood Dale, IL, USA) at a rate of 0.2 μl/min. GSK1016790A (0.2 to 10 pmol/mouse/day) was injected once daily for 5 days (GSK1016790A-injected mice). SB203580 is a selective antagonist of p38 MAPK signaling, and U0126 is an effective inhibitor of MAPK/ERK signaling [16, 17]. U0126 (0.3 nmol/mouse/day) or SB203580 (2 nmol/mouse/day) was injected 30 min before GSK1016790A injection and then injected once daily for 5 days. The doses of the above antagonists were chosen based on previous reports [14, 15]. Control mice were injected with the same volume of vehicle.
Histological Examination
For 5-bromo-2-deoxyuridine (BrdU) immunostaining, BrdU was dissolved in 0.9 % saline at a final concentration of 10 mg/ml and the BrdU solution was made fresh just before the injection. Mice received three intraperitoneal (ip.) injections of BrdU (50 mg/kg) at 8-h intervals for mitotic labeling and that day as termed day 0 (Fig. 1a). The mice were anesthetized with chloral hydrate (400 mg/kg, ip.) and transcardially perfused with 4 % paraformaldehyde at different time points after the last BrdU injection (Fig. 1a). Brains were post-fixed overnight in 4 % paraformaldehyde at 4 °C and coronally sectioned (40 μM thick) using a vibrating microtome (Microslicer DTK 1500; Dousaka EM Co., Kyoto, Japan). Every fifth free-floating section was treated with 2 M HCl for 30 min at 37 °C. The sections were treated with 3 % normal goat serum and then incubated overnight with mouse monoclonal anti-BrdU (1:1000; Millipore, Billerica, MA, USA) at 4 °C. After several rinses with PBS, the sections were incubated for 2 h with biotin-labeled goat anti-mouse IgG (1:500; Bioworld Technology, Inc., St. Louis Park, MN, USA). Immunostaining was visualized by avidin-biotin horseradish peroxidase complex (ABC Elite Kit; 1:50; Vector Laboratories, Inc., Burlingame, CA, USA) using 3,3′-diaminobenzidine as a chromogen. For Ki-67 immunostaining, the sections were incubated with rabbit monoclonal anti-Ki-67 (1:1000; Abcam; Cambridge, UK) followed by biotin-labeled goat anti-rabbit IgG (1:500; Santa Cruz Biotechnology; Santa Cruz, CA, USA). For doublecortin (DCX) immunostaining, the sections were incubated with goat polyclonal anti-DCX (1:500; Santa Cruz Biotechnology; Santa Cruz, CA, USA) followed by biotin-labeled rabbit anti-goat IgG (1:200; Bioworld Technology, St. Louis Park, MN, USA). For BrdU and neuron-specific nuclear protein (NeuN) or BrdU and the glial marker glial fibrillary acidic protein (GFAP) double immunostaining, the sections were incubated with rat monoclonal anti-BrdU (1:200; Abcam, Cambridge, UK) and developed using CY3-labeled anti-rat IgG (1:500; Millipore, Billerica, MA, USA); mouse monoclonal anti-NeuN or anti-GFAP (1:200; Millipore, Billerica, MA, USA) was developed using FITC-labeled anti-mouse IgG (1:50; Millipore, Billerica, MA, USA).
Effect of the TRPV4 agonist GSK1016790A on the proliferation of stem cell. a The time course of the experimental procedure. BrdU staining was examined 1, 7, 14, 21, and 28 days after the last BrdU injection; Ki-67 staining was examined 5 days after GSK1016790A injection; DCX staining was examined 14 days after the last BrdU injection; BrdU/NeuN or BrdU/GFAP double staining was examined 28 days after the last BrdU injection. b Injection of GSK1016790A (2 pmol/mouse/day) increased the number of 1-day-old BrdU+ cells. Representative images of 1-day-old BrdU+ cells (black arrows) in control and GSK1016790A-injected mice; ×20 objective, scale bar = 100 μM. **p < 0.01 vs. control. c Numbers of 1-day-old BrdU+ cells in the presence of different doses of GSK1016790A. **p < 0.01 vs. control. d Dose-dependent GSK1016790A-mediated increase in 1-day-old BrdU+ cells. e Injection of GSK1016790A increased the number of Ki-67+ cells. Representative images of Ki-67+ cells (red arrows) in control and GSK1016790A-injected mice; ×20 objective, scale bar = 100 μM. **p < 0.01 vs. control. f Injection of GSK1016790A increased the hippocampal PCNA protein level. **p < 0.01 vs. control (color figure online)
BrdU-positive (BrdU+) and Ki-67-positive (Ki-67+) cells were observed every fifth section (200 μm apart) on a conventional light microscope (Olympus DP70, Tokyo, Japan) with a ×40 objective. Total number of BrdU+ or Ki-67+ cells per section were counted and multiplied by 5 to obtain the total number of cells in DG [18]. BrdU+ cells expressing NeuN (BrdU+/NeuN+) and BrdU+ cells expressing GFAP (BrdU+/GFAP+) cells were observed via confocal laser scanning microscopy (Leica, Heidelberg, Germany) [18]. DCX+ fibers in molecular layer were counted on a conventional light microscope with an oil immersion lens (×100) and analyzed with ImageJ software (NIH Image, Bethesda, MD, USA) [18]. The density and the length of DCX+ fibers were expressed as the number of DCX+ dendrites per millimeter in the middle third and the outer third of the molecular layer divided by the number of DCX+ cells [18, 19].
Western Blot Analysis
Western blot analysis was performed 1 day after the last GSK1016790A injection. Hippocampal protein concentrations were determined using a BCA Protein Assay Kit (Pierce, Rochford, IL, USA). Equal amounts of protein were separated by SDS-polyacrylamide gel electrophoresis and transferred to PVDF membranes. The membranes were blocked using 5 % nonfat milk in Tris-buffered saline (TBS)/Tween-20 and then incubated with antibodies against phospho-ERK1/2 (p-ERK1/2, 1:1000, Cell Signaling Technology, Beverly, MA, USA), phospho-p38 MAPK (p-p38 MAPK, 1:1000, Cell Signaling Technology, Beverly, MA, USA), cyclin-dependent kinase (CDK) 2 (1:1000, Abcam, Cambridge, UK), CDK4 (1:1000, Abcam, Cambridge, UK), CDK6 (1:1000, Cell Signaling Technology, Beverly, MA, USA), cyclin E1 (1:1000, Cell Signaling Technology, Beverly, MA, USA), cyclin A2 (1:1000, Abcam, Cambridge, UK), cyclin D1 (1:1000, Abcam, Cambridge, UK), PCNA (1:1000, Abcam, Cambridge, UK), phospho-retinoblastoma protein (p-Rb, 1:1000, Cell Signaling Technology, Beverly, MA, USA), proliferating cell nuclear antigen (PCNA) (Cell Signaling Technology, Beverly, MA, USA) and glyceraldehyde-3-phosphate dehydrogenase (GAPDH) (1:5000, Abcam, Cambridge, UK) at 4 °C overnight. After being washed with TBST, the membranes were incubated with HRP-labeled secondary antibody, developed using an ECL Detection Kit (Amersham Biosciences, Piscataway, NJ) and analyzed using ImageJ software (NIH). Following visualization of p-ERK, p-p38 MAPK, and p-Rb, the blots were stripped by incubation in stripping buffer (Restore, Pierce Chemical Co., Rockford, IL) for 5 min, reblocked for 60 min with skim milk at room temperature, and then incubated with ERK1/2 (1:1000, Cell Signaling Technology, Beverly, MA, USA), p38 MAPK (1:1000, Cell Signaling Technology, Beverly, MA, USA), or Rb (Cell Signaling Technology, Beverly, MA, USA) antibody, respectively. Hippocampal samples collected from the hemispheres of three mice were considered a set for western blot analysis. The data represent the average of three experimental sets.
Chemicals
All chemicals, unless otherwise stated, were obtained from Sigma-Aldrich Co.
Data Analysis
Data are expressed as the mean ± SEM, and statistical analyses were performed using SPSS software, version 16.0 (SPSS Inc., USA). ANOVA followed by Bonferroni’s post hoc test was used to evaluate statistical significance, and the significance level was set at p < 0.05 and p < 0.01. The protein levels in mice injected with GSK1016790A and/or the kinase antagonist were expressed as the percentage of that in vehicle-injected mice (control mice). The increases in the number of 1-day-old BrdU+ cells resulting from different doses of GSK1016790A were first normalized to the increase caused by 10 pmol GSK1016790A. The dose-response curve was fitted by the Hill equation, a = amax/[1 + (EC50/C)n ], where n is the Hill coefficient and EC50 value is the dose of GSK1016790A that produced a 50 % effect.
Results
Effect of the TRPV4 Agonist GSK1016790A on the Proliferation of Stem Cell
GSK1016790A was icv. injected for five consecutive days before the last BrdU injection (day −4 to day 0, Fig. 1a). BrdU+ cells in the hippocampal DG region were examined at different time points (1, 7, 14, 21 and 28 days) after the last BrdU injection (1-, 7-, 14-, 21- and 28-day-old BrdU+ cells; Fig. 1a). The proliferating cells complete one mitotic division within 24 h; therefore, 1-day-old BrdU+ cells indicate the proliferation of stem cells. Compared with the control group, more 1-day-old BrdU+ cells were found in mice injected with GSK1016790A (GSK1016790A-injected mice) (Fig. 1b, c). The number of 1-day-old BrdU+ cells showed a dose-dependent increase in response to GSK1016790A at doses ranging from 0.2 to 10 pmol/mouse/day, with a Hill coefficient and EC50 of 1.36 and 1.10 ± 0.16 pmol/mouse/day, respectively (Fig. 1c). The number of 1-day-old BrdU+ cells increased by 110.00 ± 3.70 % (p < 0.01) after the administration of 2 pmol/mouse/day GSK1016790A, and this dose was used in the following experiments.
Ki-67 and PCNA are intrinsic markers of cell proliferation [20]. As shown in Fig. 1e, more Ki-67+ cells were found in GSK1016790A-injected mice than in control mice (p < 0.01). PCNA protein level was markedly higher in GSK1016790A-injected mice compared with control mice (Fig. 1f) (p < 0.01). These results indicate that TRPV4 activation promotes the proliferation of stem cells in the hippocampal DG.
Effect of GSK1016790A on the Expression of Cell Cycle-Related Proteins
In mammals, the cell cycle is driven by the concerted action of CDKs and their activating partners, the cyclins [21]. In the present study, CDK6 and CDK2 protein levels were markedly enhanced following GSK1016790A administration (p < 0.01 in each case), whereas CDK4 level was nearly unchanged (Fig. 2a). Additionally, cyclin E1 and cyclin A2 protein levels were higher in GSK1016790A-injected mice (p < 0.01 in each case), with cyclin D1 protein level nearly unchanged (Fig. 2b). As expected, phosphorylated Rb (p-Rb) protein level was significantly increased upon GSK1016790A administration (p < 0.01) (Fig. 2c). The above results indicate that TRPV4 activation may accelerate the cell cycle by increasing the expression of CDK2/6 and cyclin E1/A2 to facilitate Rb phosphorylation, resulting in enhanced stem cell proliferation.
Involvement of MAPK Signaling Pathways in the GSK1016790A-Induced Promotion of Stem Cell Proliferation and Increase of Cell Cycle-Related Protein Expression
MAPK signaling pathways, including ERK, p38 MAPK, and c-Jun N-terminal kinase (JNK), play an important role in regulating cell proliferation, differentiation, and survival [22]. In the present study, p-ERK1/2 and p-p38 MAPK protein levels were enhanced in mice injected with GSK1016790A for 5 days (p < 0.01) (Fig. 3a, b). Next, we examined the involvement of ERK1/2 and/or p38 MAPK signaling pathways in the GSK1016790A-enhanced proliferation of stem cell. We found that 1-day-old BrdU+ cell count in GSK1016790A-injected mice was significantly reduced by administration of a MEK antagonist, U0126, or a p38 MAPK antagonist, SB203580 (Fig. 3c). GSK1016790A-induced increase in 1-day-old BrdU+ cells was attenuated from 110.00 ± 3.70 to 38.32 ± 5.56 % by U0126 and to 4.70 ± 1.67 % by SB203580, respectively (p < 0.01 in each case). Fewer Ki-67+ cells were found in mice that were co-injected with GSK1016790A and U0126 or SB203580 (Fig. 3d). The increase in Ki-67+ cells caused by GSK1016790A was reduced from 100.6 ± 1.89 to 31.52 ± 5.62 % by U0126 and to 3.45 ± 0.98 % by SB203580, respectively (p < 0.01 in each case). Additionally, the increased PCNA protein level in GSK1016790A-injected mice was blocked by U0126 or SB203580 (p < 0.01 in each case) (Fig. 3e). These results indicate that ERK1/2 and p38 MAPK signaling are involved in the enhanced stem cell proliferation caused by TRPV4 activation.
Involvement of ERK1/2 and p38 MAPK signaling in the GSK1016790A-induced promotion of stem cell proliferation. a, b Western blot analysis of hippocampal p-ERK1/2 (a) and p-p38 MAPK (b) protein levels in control and GSK1016790A-injected mice. **p < 0.01 vs. control. c, d The increased numbers of 1-day-old BrdU+ (black arrows) (c) and Ki-67+ cells (red arrows) (d) were markedly attenuated by the MEK antagonist U0126 or by the p38 MAPK antagonist SB203580. **p < 0.01 vs. control, ^^p < 0.01 vs. GSK1016790A, ##p < 0.01 vs. U0126. e Hippocampal PCNA protein level in GSK1016790A-injected mice was markedly lower in mice co-injected with U0126 or SB203580. **p < 0.01 vs. control, ^^p < 0.01 vs. GSK1016790A, ##p < 0.01 vs. U0126 (color figure online)
We also assessed the effect of U0126 and SB203580 on the GSK1016790A-induced modulation of cell cycle-related protein expression. As shown in Fig. 4a, b, co-injection with U0126 or SB203580 markedly attenuated the increase in CDK6 and CDK2 protein levels in GSK1016790A-injected mice (p < 0.01 in each case). Cyclin E1 and cyclin A2 protein levels were lower in mice co-injected with GSK1016790A and U0126 or SB203580 compared with mice injected with GSK1016790A alone (p < 0.01 in each case) (Fig. 4c, d). Figure 4e shows that the increase in p-Rb protein level in GSK1016790A-injected mice was strongly inhibited by U0126 or SB203580 (p < 0.01 in each case) (Fig. 4f). These results indicate that TRPV4 activation may increase ERK1/2 and p38 MAPK signaling, resulting in the upregulation of CDKs, cyclins, and Rb phosphorylation and consequently accelerating the cell cycle.
Involvement of ERK1/2 and p38 MAPK signaling in the GSK1016790A-induced modulation of cell cycle-related protein expression. Western blot analysis shows that the GSK1016790A-induced increases in hippocampal CDK6 (a), CDK2 (b), cyclin E1 (c), cyclin A2 (d), and p-Rb (e) protein levels were markedly attenuated by U0126 or SB203580. **p < 0.01 vs. control, ^^p < 0.01 vs. GSK1016790A, ##p < 0.01 vs. U0126, $$p < 0.01 vs. SB203580
Effect of GSK1016790A on Neurite Growth, Differentiation, and Survival of Newborn Cells
As shown in Fig. 5a, GSK1016790A injection also caused a significant increase in the numbers of 7- and 14-day-old BrdU+ cells (p < 0.01 in each case) and had no effect on the number of 21- or 28-day-old BrdU+ cells. Here, we noted that the ratio of 7-day-old/1-day-old BrdU+ cells in GSK1016790A-injected mice was smaller than that in control mice (p < 0.01) and that the 14-day-old/7-day-old BrdU+ ratio in GSK1016790A-injected mice was similar to that in control mice (Fig. 5b). DCX, a microtubule-associated protein, is specifically expressed in newly generated healthy neurons, reaching a peak during the second week [23]. As shown in Fig. 5c, the number of DCX+ dendrites per DCX+ cell in the middle and outer third of the molecular layer was unaffected by GSK1016790A. In addition, the numbers of 28-day-old BrdU+/NeuN+ or BrdU+/GFAP+ were similar between GSK1016790A-injected and control mice (Fig. 5d). These results indicate that TRPV4 activation via the present protocol does not affect neurite growth, differentiation, or survival of newborn cells.
Effect of GSK1016790A on neurite growth, differentiation and survival of newborn cells. a Effect of GSK1016790A injection (see procedure in Fig. 1a) on the numbers of 7-, 14-, 21-, and 28-day-old BrdU+ cells. **p < 0.01 vs. control. b The ratios of 7-day-old/1-day-old and 14-day-old/7-day-old BrdU+ cells in control and GSK1016790A-injected mice. **p < 0.01 vs. control. c DCX+ cells dendrites in control and GSK1016790A-injected mice; ×40 objective, scale bar = 100 μM. d BrdU+/NeuN+ and BrdU+/GFAP+ cells in control and GSK1016790A-injected mice. Representative images of BrdU+/NeuN+ cells (white stars) in control and GSK1016790A-injected mice; ×40 objective, scale bar = 100 μM
Discussion
Neurogenesis occurs throughout adult life and is regulated by both physiological and pathological factors [1]. Ca2+ plays an important role in modulating cell growth, differentiation, and survival [3, 4]. TRPV4 is a member of the TRPV subfamily, and this receptor is abundant in the hippocampus [7, 24]. Increased [Ca2+]i caused by TRPV4 activation has been confirmed in various cell types, including hippocampal neurons [24]. Some TRPC family members that are permeable to Ca2+ are also reportedly involved in neurogenesis. For example, TRPC1 activation is required for the proliferation of adult hippocampal neuronal progenitor cells and contributes to the basic fibroblast growth factor-induced self-renewal of embryonic rat neural stem cells [11, 25]. Higher TRPC4 and TRPC7 protein levels were found in proliferating cells in an immortalized hippocampal neuronal cell line (H19-7 cells). TRPC1 and TRPC3 may also control the switch between proliferation and differentiation in H19-7 cells [26]. The present in vivo study is the first to demonstrate that TRPV4 activation can promote the proliferation of stem cell in the adult hippocampal dentate gyrus.
In the adult hippocampus, stem cells are located in the SGZ. As the first step of neurogenesis, stem cell proliferation plays a vital role in controlling the subsequent process of neurogenesis. BrdU is an analog of thymidine that can incorporate into proliferating cells, and Ki-67 is an intrinsic marker of proliferation that is expressed throughout the cell cycle in continuously dividing cells [20]. In the present study, the proliferation of stem cell was first assessed using BrdU and Ki-67 labeling. We found that 1-day-old BrdU+ and Ki-67+ cell counts were both markedly increased in mice injected with GSK1016790A. We then found that the protein level of PCNA, another intrinsic marker of proliferation, was significantly enhanced in GSK1016790A-injected mice (Fig. 1). These results confirmed that TRPV4 activation promotes the proliferation of stem cell. However, for lack of the specific markers, the present study could not identify which type of progenitor cells proliferation was enhanced by TRPV4 activation. In fact, activation of TRPV4 also enhances proliferation in other cell types. For example, application of the TRPV4 agonist 4α-PDD increased Ki-67+ cells in cultured porcine endothelial cells and promoted BrdU incorporation in esophageal epithelial cells [9, 27]. TRPV4 activation also potentiated the proliferation of human brain capillary endothelial cells [8]. Notably, the TRPV4-induced promotion of cell proliferation in the above reports is accompanied by increased [Ca2+]i. Hence, these TRPV4-induced increases in [Ca2+]i are thought to be responsible for promoting stem cell proliferation. In the hippocampus, TRPV4 activation was reported to enhance N-methyl-D-aspartate (NMDA)-mediated Ca2+ entry [28]. Therefore, the TRPV4-induced increase in [Ca2+]i in the hippocampus may result from Ca2+ influx through the receptor per se and/or through NMDA glutamate receptors.
The cell cycle is tightly regulated by CDKs and cyclins [21]. [Ca2+]i is thought to play a crucial role in regulating the cell cycle by stimulating the expression of genes associated with cell cycle progression, as well as by activating enzymes that participate in DNA replication and mitosis [4]. The role of Ca2+ influx through TRP channels in cell proliferation and cell cycle progression has been confirmed in previous studies. For example, knocking down TRPC1 induced G1 arrest and reduced the proliferation of endothelial progenitor cells [29]. Knocking down TRPM7 also led to a block in G0/G1 in pancreatic epithelial cells and slowed the G1/S transition in human retinoblastoma cells [30, 31]. In mammals, cell proliferation is predominantly controlled during the G1 phase of the cell cycle [32]. During G1, cyclin D binds to activate CDK4 or CDK6, and their complexes phosphorylate Rb to release E2F proteins, which promote the transcription of genes required for cell cycle progression, including cyclin E. Activated cyclin E-CDK2 complexes irreversibly inactivate Rb and subsequently promote the G1/S transition. CDK2 then partners with cyclin A to drive the cell through S phase, and at the end of this cell cycle phase, CDK1/cyclin B and A complexes promote the G2/M transition. In the present study, CDK6 and CDK2 protein levels were markedly increased, whereas CDK4 level was nearly unchanged in GSK1016790A-injected mice. The cyclin E1 and cyclin A2 protein levels were higher in GSK1016790A-injected mice than in controls, whereas the cyclin D1 level was unaltered. Additionally, p-Rb protein level was elevated by GSK1016790A injection (Fig. 2). Therefore, we propose that TRPV4 activation facilitates Rb phosphorylation by increasing the CDK6 and CDK2-cyclin E complexes to promote the G1/S transition and then accelerate S phase by increasing CDK2-cyclin A complexes. Therefore, this acceleration of cell cycle progression is likely responsible for the promotion of stem cell proliferation caused by TRPV4 activation.
MAPKs are a family of serine-threonine kinases that play an important role in regulating multiple biological processes, including proliferation, survival, differentiation, and transformation [22]. Previous studies in lung epithelial cells, trigeminal ganglion and dorsal root ganglion sensory neurons, capillary endothelial cells, adipocytes, and hippocampi have shown that TRPV4 activation may enhance ERK1/2 and p38 MAPK signaling [14, 15, 33–36]. Recently, we found that application of a TRPV4 agonist had no effect on JNK signaling [14]. Therefore, the present study focused on the involvement of ERK1/2 and p38 MAPK signaling in the TRPV4-induced promotion of stem cell proliferation. Consistent with previous reports, p-ERK1/2 and p-p38 MAPK protein levels were higher in GSK1016790A-injected mice (Fig. 3a, b). TRPV4 is permeable to Ca2+ and activation of TRPV4 induces an increase in [Ca2+]i. TRPV1 is another member of TRPV subfamily and also permeable to Ca2+. It has been reported that activation of TRPV1 can lead to the activation of ERK and p38 MAPK signaling pathways, dependent on TRPV1-induced Ca2+ signaling [5]. Therefore, it is deduced that TRPV4-induced Ca2+ influx may be responsible for the activation of ERK and p38 MAPK signaling pathways. Besides this, activation of NMDA receptor is related to the activation of ERK pathway. In our previous studies, NMDA receptor function can be enhanced by TRPV4 activation and blockage of NR2B subunit markedly attenuated TRPV4-induced activation of ERK signaling pathway [15, 28]. Therefore, the enhanced NMDA receptor is also likely involved in the TRPV4-induced ERK signaling pathway activation. Here, GSK1016790A-increased p-p38 MAPK protein level was markedly attenuated by U0126 (Supplementary Fig. 1b), indicating that TRPV4-activated ERK signaling pathway may facilitate p38 MAPK signaling pathway activation.
ERK1/2 signaling pathway plays a pivotal role in controlling cell cycle progression and is involved in the proliferation of neuronal progenitor cells [22]. After activation, ERK translocates to the nucleus, where it phosphorylates transcription factors, resulting in expression of genes involved in cell cycle progression (including CDKs and cyclins) [37]. Inhibition of p38 MAPK signaling blocks the proliferation of neuronal and oligodendrocyte progenitors [38, 39]. The effect of p38 MAPK in the proliferation of neuronal precursors is probably limited to G2/M phase, when p38 MAPK is phosphorylated by a specific MAPKK, PDZ-binding kinase/T-LAK cell-originated protein kinase that is activated by cyclin B/CDK1 [38]. p38 MAPK signaling-induced modulation of oligodendrocyte progenitor proliferation is cooperation with ERK signaling [39]. Activation of p38 MAPK is linked to phosphorylation of Ser-389 of glycogen synthase kinase 3β in adult hippocampal neural stem/progenitor cells, which in turn results in reduced degradation and increased intracellular level and nuclear accumulation of β-catenin [40]. Here, the GSK1016790A-induced increase in 1-day-old BrdU+ cells was markedly attenuated by either U0126 or SB203580 (Fig. 3c); the increased Ki-67+ cell counts and PCNA expression in GSK1016790A-injected mice were blocked by U0126 or SB203580 as well (Fig. 3d, e), indicating that both ERK and p38 MAPK signaling pathways are involved in TRPV4-enhanced stem cell proliferation. As discussed above, GSK1016790A-increased ERK signaling pathway activation may help to activate p38 MAPK signaling pathway. However, TRPV4-induced increase in p-ERK protein level was not affected by SB203580 (Supplementary Fig. 1a). The detailed interaction between ERK and p38 MAPK signaling pathways under the condition of TRPV4 activation needs to be studied in the future. By assessing the expression of cell cycle-related proteins, our data showed that the increased protein levels of CDK6/CDK2 and cyclin E1/cyclin A2 were attenuated by both U0126 and SB203580; GSK1016790A-induced increase in p-Rb protein level was also blocked by U0126 and SB203580 (Fig. 4). These results indicate a convergence of the downstream targets of ERK and p38 MAPK signaling pathways. Therefore, activation of TRPV4 may lead to activate ERK and p38 MAPK signaling pathways and these two pathways likely have cooperative effect on TRPV4-enhanced proliferation of stem cell through increasing the expression of proteins that regulate both the G1/S transition and S-phase progression.
The present study showed that the ratio of 7-day-old/1-day-old BrdU+ cells was decreased by GSK1016790A (Fig. 5b), suggesting that although TRPV4 activation promotes stem cell proliferation, more newborn cells die during neurogenesis. Here, GSK1016790A was injected for five consecutive days and was supposed to be absent 7 days after injection. The ratio of 14-day-old/7-day-old BrdU+ cells in GSK-injected mice was similar to that in control mice, consistent with our hypothesis. Therefore, the GSK1016790A-induced increase in 7- and 14-day-old BrdU+ cells was more likely a residual effect of the increased proliferation (Fig. 5a). It is known that apoptosis and proliferation are intimately coupled. Activation of TRPV4 has been proven to induce apoptosis in various types of cells [14, 41, 42]. In our recent study, activation of TRPV4 results in the apoptosis in hippocampus, which is mediated, at least partially, through activation of p38 MAPK signaling pathway [14]. Therefore, the present enhanced proliferation of stem cell in SGZ is probably a secondary response to TRPV4-induced neurotoxicity. Besides this, apoptotic newborn cells may also exist upon TRPV4 activation. As the ratio of 7-day-old/1-day-old BrdU+ cells decreased markedly by GSK1016790A treatment, therefore, it is deduced that apoptosis of newborn cells may be prominent in the early stage of the neurogenesis in the present drug administration protocol. More experiments are needed to clarify this in the future study. Prior evidence suggests that TRPV4 activation facilitates nerve growth factor-induced neurite growth in peripheral neurons [43]. However, our data showed that neither the density nor the length of DCX+ fiber was affected by GSK1016790A (Fig. 5c), indicating that TRPV4 activation did not affect neurite growth. Moreover, the numbers of 28-day-old BrdU+, BrdU+/NeuN+, and BrdU+/GAPDH+ cells in GSK1016790A-injected mice were the same as those in control mice (Fig. 5d), indicating that TRPV4 activation did not affect differentiation or survival of newborn cells. As discussed above, these negative results were probably due to the GSK1016790A injection protocol used in this study. More experiments are needed to study the direct effect of TRPV4 activation on neurite growth, differentiation, or survival of newborn cells during neurogenesis.
Besides hippocampal SGZ, another brain region that contains the stem cells is the subventricular zone (SVZ) of the lateral ventricles. However, there are differences across species in the quantity of progenitor cells in SGZ vs. SVZ [44]. Changes in the number of proliferating stem cells in SGZ and SVZ in the pathological conditions (such as Alzheimer’s disease and cerebral ischemia) have been reported [45, 46]. Whether the proliferation of stem cells in SVZ could be affected by TRPV4 activation remains to be illuminated.
Our study provides in vivo evidence that TRPV4 activation promotes the proliferation of stem cells in the adult hippocampal DG, and this effect is likely mediated through activation of ERK1/2 and p38 MAPK signaling to increase the expression of CDKs (CDK6 and CDK2) and cyclins (cyclin E1 and A2), phosphorylate Rb consequently, and accelerate the cell cycle ultimately. Therefore, TRPV4 may be a new target for the modulation of adult neurogenesis under pathological conditions.
References
Alvarez-Buylla A, Garcia-Verdugo JM (2002) Neurogenesis in adult subventricular zone. J Neurosci 22:629–634
Balu DT, Lucki I (2009) Adult hippocampal neurogenesis: regulation, function implications and contribution to disease pathology. Neurosci Biobehav Rev 33:232–252
Berridge MJ, Lipp P, Bootman MD (2000) The versatility and universality of calcium signaling. Nat Rev Mol Cell Biol 1:11–21
Capiod T (2013) The need for calcium channels in cell proliferation. Recent Pat Anticancer Drug Discov 8:4–17
Guo Z, Shi F, Zhang L, Zhang H, Yang J, Li B, Jia J, Wang X, Wang X (2010) Critical role of L-type voltage-dependent Ca2+ channels in neural progenitor cell proliferation induced by hypoxia. Neurosci Lett 478:156–160
Bunk EC, König HG, Prehn JH, Kirby BP (2014) Effect of the N-methyl-D-aspartate NR2B subunit antagonist ifenprodil on precursor cell proliferation in the hippocampus. J Neurosci Res 92:679–691
Garcia-Elias A, Mrkonjić S, Jung C, Pardo-Pastor C, Vicente R, Valverde MA (2014) The TRPV4 channel. Handb Exp Pharmacol 222:293–319
Hatano N, Suzuki H, Itoh Y, Muraki K (2013) TRPV4 partially participates in proliferation of human brain capillary endothelia cells. Life Sci 92:317–324
Troidl C, Troidl K, Schierling W, Cai WJ, Nef H, Möllmann H, Kostin S, Schimanski S, Hammer L, Elsässer A, Schmitz-Rixen T, Schaper W (2009) Trpv4 induces collateral vessel growth during regeneration of the arterial circulation. J Cell Mol Med 13:2613–2621
Martin E, Dahan D, Cardouat G, Gillibert-Duplantier J, Marthan R, Savineau JP, Ducret T (2012) Involvement of TRPV1 and TRPV4 channels in migration of rat pulmonary arterial smooth muscle cells. Pflugers Arch 464:261–272
Li M, Chen C, Zhou Z, Xu S, Yu Z (2012) A TRPC1-mediated increase in store-operated Ca2+ entry is required for the proliferation of adult hippocampal neuronal progenitor cells. Cell Calcium 51:486–496
Thoppil RJ, Adapala RK, Cappelli HC, Kondeti V, Dudley AC, Gary Meszaros J, Paruchuri S, Thodeti CK (2015) TRPV4 channel activation selectively inhibits tumor endothelia cell proliferation. Sci Rep 5:14257
Song Y, Zhan L, Yu M, Huang C, Meng X, Ma T, Zhang L, Li J (2014) TRPV4 channel inhibits TGF-β1-induced proliferation of hepatic stellate cells. PLoS One 9:e101179
Jie P, Hong Z, Tian Y, Li Y, Lin L, Zhou L, Du Y, Chen L, Chen L (2015) Activation of transient receptor potential vanilloid 4 induces apoptosis in hippocampus through down-regulating PI3K/Akt and up-regulating p38 MAPK signaling pathways. Cell Death Dis 6:e1775
Jie P, Lu Z, Hong Z, Li L, Zhou L, Li Y, Zhou R, Zhou Y, Du Y, Chen L, Chen L (2016) Activation of transient receptor potential vanilloid 4 is involved in neuronal injury in middle cerebral artery occlusion in mice. Mol Neurobiol 53:8–17
Badger AM, Bradbeer JN, Votta B, Lee JC, Adams JL, Griswold DE (1996) Pharmacological profile of SB 203580, a selective inhibitor of cytokine suppressive binding protein/p38 kinase, in animal models of arthritis, bone resorption, endotoxin shock and immune function. J Pharmacol Exp Ther 279:1453–1461
Duncia JV, Santella JB 3rd, Higley CA, Pitts WJ, Wityak J, Frietze WE, Rankin FW, Sun JH, Earl RA, Tabaka AC, Teleha CA, Blom KF, Favata MF, Manos EJ, Daulerio AJ, Stradley DA, Horiuchi K, Copeland RA, Scherle PA, Trzaskos JM, Magolda RL, Trainor GL, Wexler RR, Hobbs FW, Olson RE (1998) MEK inhibitors: the chemistry and biological activity of U0126, its analogs, and cyclization products. Bioorg Med Chem Lett 8:2839–2844
Sha S, Xu J, ZH L, Hong J, WJ Q, Zhou JW, Chen L (2016) Lack of JWA enhances neurogenesis and long-term potentiation in hippocampal dentate gyrus leading to spatial cognitive potentiation. Mol Neurobiol 53:355–368
Wang C, Chen T, Li G, Zhou L, Sha S, Chen L (2015) Simvastatin prevents β-amyloid(25-35)-impaired neurogenesis in hippocampal dentate gyrus through α7nAChR-dependent cascading PI3K-Akt and increasing BDNF via reduction of farnesyl pyrophosphate. Neuropharmacology 97:122–132
Schutte B, Tinnemans MM, Pijpers GF, Lenders MH, Ramaekers FC (1995) Three parameter flow cytometric analysis for simultaneous detection of cytokeratin, proliferation associated antigens and DNA content. Cytometry 21:177–186
Cheffer A, Tárnok A, Ulrich H (2013) Cell cycle regulation during neurogenesis in the embryonic and adult brain. Stem Cell Rev 9:794–805
Miloso M, Scuteri A, Foudah D, Tredici G (2008) MAPKs as mediators of cell fate determination: an approach to neurodegenerative diseases. Curr Med Chem 15:538–548
Rao MS, Shetty AK (2004) Efficacy of doublecortin as a marker to analyse the absolute number and dendritic growth of newly generated neurons in the adult dentate gyrus. Eur J Neurosci 19:234–246
Shibasaki K, Suzuki M, Mizuno A, Tominaga M (2007) Effects of body temperature on neural activity in the hippocampus: regulation of resting membrane potentials by transient receptor potential vanilloid 4. J Neurosci 27:1566–1575
Fiorio Pla A, Maric D, Brazer SC, Giacobini P, Liu X, Chang YH, Ambudkar IS, Barker JL (2005) Canonical transient receptor potential 1 plays a role in basic fibroblast growth factor (bFGF)/FGF receptor-1-induced Ca2+ entry and embryonic rat neural stem cell proliferation. J Neurosci 25:2687–2701
Wu X, Zagranichnaya TK, Gurda GT, Eves EM, Villereal ML (2004) A TRPC1/TRPC3-mediated increase in store-operated calcium entry is required for differentiation of H19-7 hippocampal neuronal cells. J Biol Chem 279:43392–43402
Ueda T, Shikano M, Kamiya T, Joh T, Ugawa S (2011) The TRPV4 channel is a novel regulator of intracellular Ca2+ in human esophageal epithelial cells. Am J Physiol Gastrointest Liver Physiol 301:G138–G147
Li L, Qu W, Zhou L, Lu Z, Jie P, Chen L, Chen L (2013) Activation of transient receptor potential vanilloid 4 increases NMDA-activated current in hippocampal pyramidal neurons. Front Cell Neurosci 7
Kuang CY, Yu Y, Wang K, Qian DH, Den MY, Huang L (2012) Knockdown of transient receptor potential canonical-1 reduces the proliferation and migration of endothelial progenitor cells. Stem Cells Dev 21:487–496
Hanano T, Hara Y, Shi J, Morita H, Umebayashi C, Mori E, Sumimoto H, Ito Y, Mori Y, Inoue R (2004) Involvement of TRPM7 in cell growth as a spontaneously activated Ca2+ entry pathway in human retinoblastoma cells. J Pharmacol Sci 95:403–419
Yee NS, Zhou W, Liang IC (2011) Transient receptor potential ion channel Trpm7 regulates exocrine pancreatic epithelial proliferation by Mg2+-sensitive Socs3a signaling in development and cancer. Dis Model Mech 4:240–254
Duronio RJ, Xiong Y (2013) Signaling pathways that control cell proliferation. Cold Spring Harb Perspect Biol 5:a008904
Nayak PS, Wang Y, Najrana T, Priolo LM, Rios M, Shaw SK, Sanchez-Esteban J (2015) Mechanotransduction via TRPV4 regulates inflammation and differentiation in fetal mouse distal lung epithelial cells. Respir Res 16:60
Chen Y, Kanju P, Fang Q, Lee SH, Parekh PK, Lee W, Moore C, Brenner D, Gereau RW 4th, Wang F, Liedtke W (2014) TRPV4 is necessary for trigeminal irritant pain and functions as a cellular formalin receptor. Pain 155:2662–2672
Segond von Banchet G, Boettger MK, König C, Iwakura Y, Bräuer R, Schaible HG (2013) Neuronal IL-17 receptor upregulates TRPV4 but not TRPV1 receptors in DRG neurons and mediates mechanical but not thermal hyperalgesia. Mol Cell Neurosci 52:152–160
Ye L, Kleiner S, Wu J, Sah R, Gupta RK, Banks AS, Cohen P, Khandekar MJ, Boström P, Mepani RJ, Laznik D, Kamenecka TM, Song X, Liedtke W, Mootha VK, Puigserver P, Griffin PR, Clapham DE, Spiegelman BM (2012) TRPV4 is a regulator of adipose oxidative metabolism, inflammation, and energy homeostasis. Cell 151:96–110
Chambard JC, Lefloch R, Pouysségur J (2007) ERK implication in cell cycle regulation. Biochim Biophys Acta 1773:1299–1310
Dougherty JD, Garcia AD, Nakano I, Livingstone M, Norris B, Polakiewicz R, Wexler EM, Sofroniew MV, Kornblum HI, Geschwind DH (2005) PBK/TOPK, a proliferating neural progenitor-specific mitogen-activated protein kinase kinase. J Neurosci 25:10773–10785
Baron W, Metz B, Bansal R, Hoekstra D, de Vries H (2000) PDGF and FGF-2 signaling in oligodendrocyte progenitor cells: regulation of proliferation and differentiation by multiple intracellular signaling pathways. Mol Cell Neurosci 15:314–329
Zhang D, Guo M, Zhang W, XY L (2011) Adiponectin stimulates proliferation of adult hippocampal neural stem/progenitor cells through activation of p38 mitogen-activated protein kinase (p38MAPK)/glycogen synthase kinase 3β (GSK-3β)/β-catenin signaling cascade. J Biol Chem 286:44913–44920
Landouré G, Zdebik AA, Martinez TL, Burnett BG, Stanescu HC, Inada H, Shi Y, Taye AA, Kong L, Munns CH, Choo SS, Phelps CB, Paudel R, Houlden H, Ludlow CL, Caterina MJ, Gaudet R, Kleta R, Fischbeck KH, Sumner CJ (2010) Mutations in TRPV4 cause Charcot-Marie-Tooth disease type 2C. Nat Genet 42:170–174
Fecto F, Shi Y, Huda R, Martina M, Siddique T, Deng HX (2011) Mutant TRPV4-mediated toxicity is linked to increased constitutive function in axonal neuropathies. J Biol Chem 286:17281–17291
Jang Y, Jung J, Kim H, Oh J, Jeon JH, Jung S, Kim KT, Cho H, Yang DJ, Kim SM, Kim IB, Song MR, Oh U (2012) Axonal neuropathy-associated TRPV4 regulates neurotrophic factor-derived axonal growth. J Biol Chem 287:6014–6024
Curtis MA, Low VF, Faull RL (2012) Neurogenesis and progenitor cells in the adult human brain: a comparison between hippocampal andsubventricular progenitor proliferation. Dev Neurobiol 72:990–1005
Jin K, Peel AL, Mao XO, Xie L, Cottrell BA, Henshall DC, Greenberg DA (2004) Increased hippocampal neurogenesis in Alzheimer’s disease. Proc Natl Acad Sci U S A 101:343–347
Jin K, Minami M, Lan JQ, Mao XO, Batteur S, Simon RP, Greenberg DA (2001) Neurogenesis in dentate subgranular zone and rostral subventricular zone after focal cerebral ischemia in the rat. Proc Natl Acad Sci U S A 98:4710–4715
Acknowledgments
This work was supported by the National Natural Science Foundation of China (81571270 and 31271206 to Lei Chen and 81470421 to Yimei Du) and Qing Lan Project of Jiangsu province (2014–2017) to Lei Chen.
Author information
Authors and Affiliations
Corresponding author
Ethics declarations
Conflict of Interest
The authors declare that they have no conflict of interest.
Electronic supplementary material
ESM 1
(GIF 36 kb)
Rights and permissions
About this article
Cite this article
Tian, Y., Qi, M., Hong, Z. et al. Activation of Transient Receptor Potential Vanilloid 4 Promotes the Proliferation of Stem Cells in the Adult Hippocampal Dentate Gyrus. Mol Neurobiol 54, 5768–5779 (2017). https://doi.org/10.1007/s12035-016-0113-y
Received:
Accepted:
Published:
Issue Date:
DOI: https://doi.org/10.1007/s12035-016-0113-y