Abstract
Hepatocellular carcinomas (HCC) are aggressive cancers, and the prognosis of HCC patients is poor. This study investigated the roles of CD29 in epithelial–mesenchymal transition (EMT) and chemoresistance and radioresistance in HCC tumors. CD29 expression in HCC and peritumoral tissues was measured by immunohistochemistry. CD29 overexpression was established by an adenovirus-carrying CD29 gene expression cassette, while silencing of CD29 expression was established by an adenovirus-carrying shRNA. Western blot was used to measure protein expression, and MTT was used to analyze cell viability. Xenograft HCC mouse model was established by inoculating isolated CD29+ and CD29− HCC tumor cells. Significantly higher percentage of positive CD29 expression was observed in HCC tissues compared to peritumoral tissues. Xenograft CD29+ tumors grew more quickly than CD29− tumors. CD29+ tumors were more resistant to radiotherapy and cisplatin therapy than CD29− tumors. Overexpression of CD29 significantly increased the resistance of CD29− tumors to radiation and cisplatin treatment. In contrast, silencing of CD29 expression significantly sensitized CD29+ tumors to irradiation and cisplatin treatment. Overexpression of CD29 decreased E-cadherin, but increased fibronectin, vimentin, ILK activity, Akt Ser473 phosphorylation, and mTORC1 protein expression in Hep G2 and THLE-3 cells. Moreover, overexpression of CD29 significantly increased the resistance of Hep G2 and THLE-3 cells to starvation, radiation, and cisplatin treatments. This study suggests that CD29 plays a crucial role in the resistance of HCC to chemo/radiotherapy and EMT of liver epithelial cells.
Similar content being viewed by others
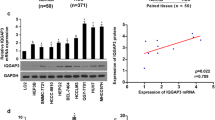
Avoid common mistakes on your manuscript.
Introduction
Liver cancer is the leading cause of cancer-related deaths in China, and the crude mortality of liver cancer in 2009 was reported to be 26.04/100,000 [1]. Hepatocellular carcinoma (HCC) is an aggressive tumor with poor prognosis. The 5-year survival rate of early detected HCC is around 70 %, and 15 % of patients experience cancer recurrence [2]. However, most patients with HCC are diagnosed at an advanced stage [3]. Patients with advanced-stage HCC have a median survival of only 6–20 months and a 5-year survival rate of 3 % [4]. These figures suggest that the mechanisms related to tumorigenesis, progression, and therapeutic resistance of HCC have not been fully elucidated.
Integrins are heterodimeric membrane glycoproteins composed of an alpha chain and a beta chain. The CD29 gene encodes an integrin β1 chain, which forms a complex with alpha chains to produce integrins, such as α3β1 and α4β1. [5]. Integrin-linked kinase (ILK) is a serine/threonine protein kinase. ILK can be activated by extracellular stimulation through interacting with the cytoplasmic domains of β1 and β3 integrins [6]. ILK can also activate Akt through phosphorylating AKT at Ser473, which subsequently activates mTOR signaling. The ILK-Akt-mTOR signaling cascade significantly contributes to β1 integrin-mediated anchorage-dependent survival of cells [7]. Integrins exhibit a broad spectrum of biological activities, and their roles in cancer cell migration, invasion, and metastasis have been intensively investigated [8]. CD29 expression has previously been detected in HCC [9, 10], and its role has been linked to the metastasis of HCC cells [11]. Studies during the past decade have found a subset of cancer cells with the ability to escape from chemo/radiotherapy. This cancer cell subpopulation, called cancer stem cells (CSCs), can initiate tumor growth and cause tumor recurrence [12]. A recent study demonstrated that CD29 is a CSC marker and is involved in epithelial–mesenchymal transition (EMT). As a stem cell marker, CD29 may be involved in tumor progression and drug resistance [13].
Epithelial–mesenchymal transition is thought to be an early event in tumor progression comparable to invasion and metastasis. Cells that undergo EMT often lose their epithelial characteristics, and these cells exhibit a decrease in E-cadherin expression. With the loss of epithelial characteristics, cells that undergo EMT also gain mesenchymal properties, such as differentiation, proliferation, and migration with an upregulation of vimentin and fibronectin expression [14, 15]. EMT is thought to play a critical role in tumor metastasis, recurrence, and resistance to therapy [16]. Although CD29 expression has been observed in HCC, it is unclear whether CD29 is involved in the EMT of liver epithelial cells and HCC cells.
In this study, we investigated the expression of CD29 protein in HCC tumor tissues and peritumoral tissues. We further investigated the sensitivity of CD29+ and CD29− HCC cells to radiation and cisplatin treatment and the roles of CD29 in EMT of HCC and liver epithelial cells.
Materials and methods
Subjects
Fifty-eight hepatocellular carcinoma (HCC) specimens were collected at Xiangya Hospital from January 2013 to December 2014. The HCCs were diagnosed by clinical symptoms, ultrasound B screening, and histological staining. Of the 58 HCC patients, 47 were male and 11 were female with an average age of 47.2 years. Among the 58 HCC patients, two had lymph node metastasis and 13 patients had vascular invasion. No patient was given preoperative chemotherapy or radiotherapy before sampling. Twenty-four peritumoral tissues were collected from the 58 HCC patients. Peritumoral tissues were collected at ≥3 cm from the edge of tumor mass. Tumor and peritumoral tissues were routinely paraffin-embedded. This study was approved by the Human Research Ethics Committee of Xiangya Hospital.
Immunohistochemistry
The paraffin-embedded tumors and peritumoral tissues were sectioned at 5 µm, and the immunostaining was carried out using EnVision™ Detection Kit (Dako Laboratories, CA, USA) according to the manufacturer’s protocol. Briefly, the sections were deparaffinized and incubated with peroxidase inhibitor (3 % H2O2) before staining. After washing with 1×PBS, sections were incubated with rabbit anti-human CD29 antibody for 1 h followed by HRP-conjugated goat-anti-rabbit antibody for 30 min after washing with 1×PBS. Sections were then stained with DAB and counter-stained with hematoxylin. The positive controls were purchased from Beijing Zhongshan Golden Bridge Biotechnology (Beijing, China). Anti-green fluorescence protein antibody was used as negative control in place of the primary antibody. The positively stained cells were counted under 400× magnification as previously reported [17]. Both the percentage of positive cells and staining intensity were used for determining whether a sample showed positive or negative overall expression. A sample was defined as strongly positive when ≥50 % of cells showed positive staining. A sample was defined as moderately positive when ≥25 %, but <50 % of cells showed positive staining. A sample was defined as negative when <25 % of cells showed positive staining or cells showed negative staining.
Isolation of CD29+ and CD29− HCC cell subsets
HCC tumor tissues from five patients were used for cell isolation, and the mixed cells were used for animal experiments. Briefly, the tumor tissues were cut into small pieces and incubated in a working solution of collagenase (0.25 Wünsch U/mL). After washing with DMEM/F-12 K medium, the single cells were cultured for one passage in DMEM/F-12 K medium containing 10 % FCS (fetal calf serum). The trypsinized cells were then suspended at a concentration of 1 × 106/mL and incubated with FITC-anti-human CD29 mAb (BioSS, China) for 1 h in the dark. After rinsing with 1×PBS, cells were fixed and treated with permeabilization solution. CD29+ and CD29− cells were sorted with MoFlo flow cytometry (Dako, CA, USA).
CD29 overexpression and silencing vectors
The overexpression of human CD29 gene was established by delivery of adenovirus-carrying human CD29 gene expression cassette. The adenovirus was produced using AdEasy™ Adenoviral Vector System (Agilent Technologies). Briefly, human CD29 gene (BC020057) was amplified by PCR using forward primer: 5′-GCTCGAGAAGATGAATTTACAACCAATTTTCTGG-3′ and reverse primer: 5′-GGATATCCTCATTTTCCCTCATATTCGG-3′ from a human cDNA library. The amplified fragment (2409 bp) was purified and directly cloned into pAdtrack-CMV vector at XhoI and EcoRV sites. The CD29 gene sequence was validated by directly sequencing the positive clones using six primers among the human CD29 gene (400 bp per primer). The pAdtrack-CMV vector containing correct human CD29 sequence was subjected to virus production as previously described [18]. The produced adenovirus-carrying human CD29 gene expression cassette was called Ad-CD29. To knock down CD29 gene expression, Ad-h-ITGβ1-shRNA virus expressing shRNA to knockdown of human CD29 mRNA was purchased from Vector BioLabs (Malvern, PA, USA). This virus was called AdsiCD29 in this study and amplified as previously described. An adenovirus produced directly from pAdtrack-CMV blank vector was used as negative control, called AdNC.
Tumor growth experiments
One hundred Balb/C nude mice (BALB/c, nu/nu) weighing 21–22 g were purchased from Shanghai Biological Science Institution and housed in the animal facility of Central South University. The animal protocol was approved by the Animal Use Committee of Central South University, and all experiments were performed in accordance with the animal care guidelines of the Chinese Council.
Experiment 1
Approximately 1 × 106 CD29+ HCC tumor cells were subcutaneously injected into the right hind limbs of 50 mice. After the tumors grew to 100 mm3, mice were randomly divided into five groups (N = 10 for each group): the control group receiving AdNC control virus injection, radiation group receiving AdNC injection plus radiation, cisplatin group receiving AdNC and cisplatin injection, Rad+AdsiCD29 group receiving radiation plus AdsiCD29 virus injection, and Cis+AdsiCD29 group receiving cisplatin and AdsiCD29 virus injection. Tumors were irradiated for 5 Gy using 320 kV X-rays for three continuous days, while covering the rest of the mouse’s body. Cisplatin was injected intraperitoneally at a concentration of 4 mg/kg daily for 6 days. AdNC, ADCD29, or AdsiCD29 virus (1 × 108 pfu in 50 µL saline) was intratumorally injected just after irradiation or cisplatin injection one time per week for three weeks. Animals were killed 30 days after treatments. Tumor volume was calculated with the formula (L × W 2)/2, where L is the tumor length and W is the tumor width, both of which were measured with a caliper.
Experiment 2
1 × 106 CD29− HCC tumor cells were subcutaneously injected into the right hind limbs of 50 mice. After the tumors grew to 100 mm3, mice were randomly divided into five groups (N = 10 for each group): the control group receiving AdNC control virus injection, radiation group receiving AdNC injection plus radiation, cisplatin group receiving AdNC and cisplatin injection, Rad+AdCD29 group receiving radiation plus AdCD29 virus injection, and Cis+AdCD29 group receiving cisplatin and AdCD29 virus injection. Animals were irradiated and injected as described above.
Cell culture
Hep G2, a human liver hepatocellular adenocarcinoma cell line, and THLE-3, a human liver epithelial cell line, were originally purchased from ATCC (American Type Culture Collection) and incubated in DMEM/F-12 (Invitrogen) and BEGM (Lonza/Clonetics Corporation, Walkersville, MD) medium, respectively. The complete medium contained 10 % fetal bovine serum and 1 % penicillin/streptomycin. Cells were cultured at 37 °C, 5 % CO2.
Western blot
Hep G2 and THLE-3 cells were passed 24 h and grew in 10-cm dishes at 70–80 % confluency before treatments. Cells were infected with 10 MOI (multiple of infection) of AdCD29, AdNC control virus, or no virus for 24 h. Cells were harvested and homogenized, and Western blot was performed as previously described [19]. Briefly, 10 µg of total protein was loaded onto each lane of a 4–15 % SDS-PAGE gel. After transferring the protein onto a PVDF membrane and blocking with 5 % nonfat milk for 30 min, membranes were incubated with primary antibody at room temperature for 2 h, followed by HRP-labeled secondary antibody (1:2000 dilution) for 2 h at room temperature. Immunoreactive proteins were visualized using a chemiluminescence reagent (Pierce, Rockford, IL, USA). To control for loading efficiency, the blots were stripped and re-probed with α-tubulin antibody (Sigma-Aldrich, St. Louis, MO, USA). The CD29, Akt Ser473, mTORC1, and peroxidase-labeled secondary antibodies were purchased from Cell Signaling Technology (Beverley, MA, USA). The antibodies for E-cadherin, N-cadherin, fibronectin, and vimentin were purchased from Abcam (USA).
ILK activity assay
Hep G2 and THLE-3 cells at 70–80 % confluency in 10-cm dishes were infected with 10 MOI of AdCD29, AdNC control virus, or no virus for 24 h. Cells were harvested and homogenized. ILK activity was analyzed as previously described [20].
MTT assay
Hep G2 or THLE-3 cells were seeded into each well of 96-well plates. After treatments, the 3-(4,5-dimethylthiazol-2yl)-2,5-diphenyltetrazolium bromide (MTT) assay was performed as previously described [18]. The cell viability was calculated as the percentage of absorbance in the non-virus-infected control (blank control).
Starvation experiments
1 × 104 Hep G2 or THLE-3 cells were seeded into each well of 96-well plates. After 24 h, cells were infected with 10 MOI of AdCD29 or AdNC virus. Cells without virus infection were used as blank control. Cells were then incubated with medium containing no FCS for 72 h. Cells without virus infection were incubated with medium containing 10 % FCS as a control. Cells were then subjected to MTT assay.
Irradiation of cells
Hep G2 or THLE-3 cells were seeded and infected as described above. Cells were irradiated for 0, 1, 2, 4, 6, and 8 Gy. After 72 h, cells were subjected to MTT assay.
Cisplatin treatment of cells
Hep G2 or THLE-3 cells were seeded and infected as described above. Cells were then incubated with 0, 10, 30, 100, 300, and 1000 µM of cisplatin for 72 h, followed by MTT assay.
Statistical analysis
Data were presented as mean ± standard error and analyzed using SPSS version 17.0 (SPSS Inc., Chicago, IL, USA). One-way ANOVA was used for statistical analyses and for comparison between groups. A P < 0.05 was considered statistically significant.
Results
The expression of CD29 in HCC and peritumoral tissues
Immunohistological staining showed that CD29 protein expression was mainly located in the cytoplasm and nucleus (Fig. 1a). Among the 58 HCC tumors, CD29 expression was negative in 14 (24.1 %) patients, moderately positive in 18 (31.0 %) patients, and strongly positive in 26 (44.8 %) patients. Among the 24 peritumoral tissues, CD29 expression was negative in 15 (62.5 %) samples, moderately positive in four (16.6 %) samples, and strongly positive in five (20.8 %) samples. Significant differences in the percentage of CD29 positive expression were observed between HCC and peritumoral tissues (Fig. 1b) (P < 0.001).
CD29 expression in tumor and peritumoral tissues. a Representative immunohistochemical staining of CD29 expression. HCC hepatocellular carcinomas, PTT peritumoral tissues. b Percentage of positive CD29 expression in HCC or peritumoral tissues. NW negative or weak expression, MP moderately positive, SP strongly positive
CD29+ HCC tumors are more resistant to chemo- and radiotherapy in xenograft animal models
The roles of CD29 in radioresistance and chemoresistance were investigated using xenograft CD29+ and CD29− HCC tumor models. Tumors that grew from inoculation of CD29+ HCC cells took 15 days to grow to 100 mm3 in volume (Fig. 2a). In contrast, tumors in mice inoculated with CD29− HCC cells took 27 days to grow to 100 mm3 (Fig. 2a). After tumors grew to 100 mm3, mice were treated as described in the Methods section above. A significant difference in tumor growth between the radiation and cisplatin injection group and the control group was observed 15 days after first radiation or cisplatin injection in mice inoculated with CD29− HCC cells (Fig. 2b). Significant differences between treatment groups and control groups can only be seen 24 days post-treatments in mice inoculated with CD29+ HCC cells (Fig. 2c). CD29+ tumors were more resistant to irradiation and cisplatin treatment than CD29− tumors (P < 0.01). To further validate the role of CD29, CD29 expression was silenced in mice with CD29+ tumors by intratumoral injection of AdsiCD29, and CD29 was overexpressed in mice with CD29− tumors by intratumoral injection of AdCD29 virus. Injection of AdCD29 significantly increased the resistance of CD29− tumors to irradiation and cisplatin treatment (Fig. 2b). In contrast, injection of AdsiCD29 virus significantly increased the sensitivity of CD29+ tumors to irradiation and cisplatin treatment (Fig. 2c).
Tumor growth and CD29 expression. a Comparison of tumor growth between CD29+ and CD29− tumors. b Growth of CD29− tumors with different treatments. CD29− tumors were sensitive to irradiation and cisplatin treatment, while AdCD29 virus injection significantly increased the resistance to irradiation and cisplatin treatments. c Growth of CD29+ tumors under different treatments. CD29+ tumors were resistant to irradiation and cisplatin treatment, while AdsiCD29 virus injection significantly sensitized tumors to irradiation and cisplatin treatments. d Representative CD29 protein expression in CD29− tumors. Injection of AdCD29 virus resulted in overexpression of CD29. e Representative CD29 expression in CD29+ tumors. Injection of AdsiCD29 virus obviously inhibited CD29 expression, N = 10
The expression of CD29 in xenograft tumor tissues was measured by Western blot. CD29 was weakly expressed in CD29− tumors, and injection of AdCD29 virus caused a high expression of CD29 in tumor tissues (Fig. 2d). CD29 protein levels were highly expressed in CD29+ tumors, and injection of AdsiRNA significantly decreased CD29 expression in CD29+ tumors (Fig. 2e).
Overexpression of CD29 enhanced EMT in HCC tumor cells and liver epithelial cells through a CD29-ILK-Akt-mTORC1 pathway
Hep G2 (Fig. 3a) and THLE-3 (Fig. 3b) cells at 80 % confluency were infected with AdCD29, AdNC virus, or no virus infection for 24 h. Cells were harvested for Western blot assay. AdCD29 virus infection caused an overexpression of CD29 protein in both Hep G2 and THLE-3 cells. The protein expression of E-cadherin was low in Hep G2 (Fig. 3a) and high in THLE-3 (Fig. 3b) cells. However, AdCD29 infection significantly decreased E-cadherin protein expression in both cell lines. In contrast, the protein expression of fibronectin and vimentin was significantly increased in AdCD29-infected Hep G2 (Fig. 3a) and THLE-3 (Fig. 3b) cells. No changes in N-cadherin expression were observed. These results suggested that: (1) high CD29 expression can further decrease N-cadherin expression, but increase fibronectin and vimentin expression in HCC tumor cells, which suggested EMT in HCC tumor cells, and (2) high CD29 expression caused EMT in liver epithelial cells. To further investigate the downstream signaling of CD29, the ILK-Akt-mTOR signaling was studied. AdCD29 infection significantly increased ILK activity, Akt Ser473 phosphorylation, and mTORC1 protein expression in both Hep G2 (Fig. 3a) and THLE-3 (Fig. 3b) cells, but the role of AdCD29 infection was more significant in THLE-3 cells. These results suggested that the activated EMT may be mediated by CD29-ILK-Akt-mTOR signaling.
Western blot of protein expression. a Western blot of protein expression in Hep G2 cells. b Western blot of protein expression in THLE-3 cells. Cells were infected with negative control virus (AdNC), CD29 expression virus (AdCD29), or no virus (blank). Western blot was performed after cells were infected for 24 h, N = 5
Overexpression of CD29 increased the resistance of cells to starvation, radiation, and cisplatin treatments
Hep G2 (Fig. 4a) and THLE-3 (Fig. 4b) cells infected with AdCD29 or AdNC virus were cultured in medium containing no FCS for 72 h. MTT assay showed that AdCD29 infection significantly increased the resistance of Hep G2 (P < 0.05) and THLE-3 cells (P < 0.001) to starvation. The effect was more significant in THLE-3 cells. We further observed the effect of CD29 in chemoresistance and radioresistance. AdCD29 infection significantly increased the resistance of Hep G2 cells to 4, 6, and 8 Gy irradiation (Fig. 4c) and 30–1000 µM cisplatin treatments (Fig. 4d). Similarly, AdCD29 infection significantly increased the resistance of THLE-3 cells to 4, 6, and 8 Gy irradiation (Fig. 4e) and 30–1000 µM cisplatin treatments (Fig. 4f).
Survival analysis. a MTT assay of cell viability of Hep G2 cells after starvation for 72 h. Stv starvation. # P < 0.05 versus NC (negative control: cells grew up in medium containing 10 % FCS). * P < 0.05 versus AdNC, N = 5. b MTT assay of cell viability of THLE-3 cells after starvation for 72 h. ** P < 0.001 versus NC or Stv+AdCD29, N = 5. c MTT assay in Hep G2 cells 72 h after irradiation for 0, 1, 2, 4, 6, and 8 Gy. * P < 0.01, ** P < 0.001 versus AdNC, N = 5. d MTT assay in Hep G2 cells after treatments for 0, 10, 30, 100, 300, and 1000 µM of cisplatin for 72 h. * P < 0.01, ** P < 0.001 versus AdNC, N = 5. e MTT assay in THLE-3 cells 72 h after irradiation for 0, 1, 2, 4, 6, and 8 Gy. * P < 0.01, ** P < 0.001 versus AdNC, N = 5. f MTT assay in THLE-3 cells after treatments for 0, 10, 30, 100, 300, and 1000 µM of cisplatin for 72 h. * P < 0.01, ** P < 0.001 versus AdNC, N = 5
Discussion
Previous studies reported increased CD29 (integrin β1) expression in HCC tissues and suggested that CD29 is a stem cell marker involved in tumor progression and drug resistance. Also, CD29 has been revealed to participate in EMT. However, (1) the role of CD29 in EMT of HCC tumors, (2) the role of CD29 in the tumorigenesis of HCC, (3) the role of CD29 in chemo/radioresistance of HCC, (4) the role of CD29 in cell survival of HCC under a circumstance of nutrition deficiency, (5) the role of EMT in HCC chemo/radioresistance, and (6) the signal pathway of CD29-mediated EMT in HCC and liver epithelial cells have not been examined. These questions have been answered in this study through experiments with xenograft CD29+ and CD29− HCC models in vivo and HCC tumor cells and normal liver epithelial cells in vitro. Our study suggests that high CD29 expression is involved in EMT of both the HCC tumor cells and liver epithelial cells though CD29-ILK-Akt-mTOR signaling, which confers chemoresistance and radioresistance to HCC tumors.
Consistent with previous studies [9, 10], this study demonstrated that CD29 was highly expressed in HCC tumor tissues (75.9 % positive) compared to the peritumoral tissues (37.4 % positive). To identify the biological activities of CD29 in HCC, CD29+ and CD29− tumor cells were isolated from human HCC tumors and inoculated in nude mice. Our results showed that xenograft CD29+ HCC tumors grew more quickly than CD29− tumors. Importantly, these CD29+ tumors were more resistant to both irradiation and cisplatin treatment than CD29− tumors. The resistance of CD29+ tumor to chemo/radiotherapy was reversed by silencing of CD29 expression via an adenovirus-carrying shRNA. Conversely, the CD29− tumor became resistant when CD29 was overexpressed through an adenovirus-carrying CD29 expression cassette. These observations provide solid evidence that HCC tumor cells with high expression of CD29 are more aggressive and high CD29 expression renders the HCC tumor cells resistant to chemo/radiotherapy. A previous study had demonstrated that CD29 is a CSC marker [13]. We propose that CD29 may also a CSC marker in HCC.
A previous study observed the role of CD29 in EMT of cancer cells [12], but its roles in HCC have not been reported. This study demonstrated that overexpression of CD29 decreased E-cadherin protein expression, while increasing fibronectin and vimentin protein expression in Hep G2 HCC tumor cells. E-cadherin is an epithelial marker, and fibronectin and vimentin are mesenchymal markers [14, 15]. This suggests that high CD29 expression could lead to EMT in HCC tumor cells. Moreover, overexpression of CD29 significantly increased the resistance of Hep G2 cells to starvation, irradiation, and cisplatin treatment. Therefore, the CD29-mediated EMT is involved in the survival and chemo/radioresistance of HCC tumor cells. A novel finding in this study is that overexpression of CD29 caused more significant changes in E-cadherin, fibronectin, and vimentin expression in THLE-3 normal liver epithelial cells, suggesting that high CD29 expression could induce EMT in normal liver epithelial cells. Furthermore, overexpression of CD29 also significantly increased the resistance of THLE-3 cells to starvation, irradiation, and cisplatin treatment. This further suggests that liver epithelial cells could gain characteristics of cancer cells through EMT and CD29 may be involved in the tumorigenesis of liver cancer.
A previous study demonstrated that constitutive activation of ILK can lead to oncogenic phenotypes in epithelial cells through downregulation of E-cadherin expression and subsequent epithelial to mesenchymal transformation [6]. Our study demonstrated that overexpression of CD29 increased ILK activation, Akt Ser473 phosphorylation, and mTOR protein expression in THLE-3 normal liver epithelial cells. The activation of ILK-Akt-mTOR signaling parallels the downregulation of E-cadherin and upregulation of fibronectin and vimentin expression. Moreover, the normal epithelial cells overexpressing CD29 are resistant to starvation and chemo/radiotherapy. This suggests that high CD29 expression can result in EMT in liver epithelial cells through activation of ILK-Akt-mTOR signaling. The observation in THLE-3 cells was confirmed in Hep G2 HCC tumor cells. Therefore, our study highlighted the role of CD29 in HCC tumorigenesis, HCC cell survival, and resistance to therapies.
In conclusion, this study suggests that high CD29 expression activates ILK-Akt-mTOR signaling, which subsequently results in EMT in both normal liver epithelial cells and HCC tumor cells. CD29-mediated EMT is involved in the tumorigenesis of HCC and survival and chemo/radioresistance of HCC cells.
References
Chen WQ, Zheng RS, Zhang SW. Liver cancer incidence and mortality in China, 2009. Chin J Cancer. 2013;32:162–9.
Siegel R, Ma J, Zou Z, et al. Cancer statistics, 2014. CA Cancer J Clin. 2014;64:9–29.
Chen X, Liu HP, Li M, et al. Advances in non-surgical management of primary liver cancer. World J Gastroenterol. 2014;20:16630–8.
Yang N, Ekanem NR, Sakyi CA, Ray SD. Hepatocellular carcinoma and microRNA: new perspectives on therapeutics and diagnostics. Adv Drug Deliv Rev. 2014;81:62–74.
Roussel E, Gingras MC, Ro JY, Branch C, Roth JA. Loss of α1β1 and reduced expression of other beta 1 integrins and CAM in lung adenocarcinoma compared with pneumocytes. J Surg Oncol. 1994;56:198–208.
Oloumi A, McPhee T, Dedhar S. Regulation of E-cadherin expression and beta-catenin/Tcf transcriptional activity by the integrin-linked kinase. Biochim Biophys Acta. 2004;1691:1–15.
Riaz A, Zeller KS, Johansson S. Receptor-specific mechanisms regulate phosphorylation of AKT at Ser473: role of RICTOR in β1 integrin-mediated cell survival. PLoS One. 2012;7:e32081.
Lahlou H, Muller WJ. β1-integrins signaling and mammary tumor progression in transgenic mouse models: implications for human breast cancer. Breast Cancer Res. 2011;13:229.
Liu LX, Jiang HC, Liu ZH, Zhou J, Zhang WH, Zhu AL, Wang XQ, Wu M. Integrin gene expression profiles of human hepatocellular carcinoma. World J Gastroenterol. 2002;8:631–7.
Zhao G, Cui J, Qin Q, Li SS, Yin T, Chen LB, Wu HS, Wang CY. The expression of integrin beta 1 in normal hepatic tissues, hepatic cirrhosis tissues and hepatocellular carcinoma]. Chin J Hepatol. 2010;18:353–6.
Tian J, Tang ZY, Ye SL, Liu YK, Lin ZY, Chen J, Xue Q. New human hepatocellular carcinoma (HCC) cell line with highly metastatic potential (MHCC97) and its expressions of the factors associated with metastasis. Br J Cancer. 1999;81:814–21.
Tong CM, Ma S, Guan XY. Biology of hepatic cancer stem cells. J Gastroenterol Hepatol. 2011;26:1229–37.
Geng S, Guo Y, Wang Q, Li L, Wang J. Cancer stem-like cells enriched with CD29 and CD44 markers exhibit molecular characteristics with epithelial–mesenchymal transition in squamous cell carcinoma. Arch Dermatol Res. 2013;305:35–47.
Scanlon CS, Van Tubergen EA, Inglehart RC, D’Silva NJ. Biomarkers of epithelial–mesenchymal transition in squamous cell carcinoma. J Dent Res. 2013;92:114–21.
Morra L, Moch H. Periostin expression and epithelial–mesenchymal transition in cancer: a review and an update. Virchows Arch. 2011;459:465–75.
Satpute PS, Hazarey V, Ahmed R, Yadav L. Cancer stem cells in head and neck squamous cell carcinoma: a review. Asian Pac J Cancer Prev. 2013;14(10):5579–87.
Liu DC, Yang ZL. Overexpression of EZH2 and loss of expression of PTEN is associated with invasion, metastasis, and poor progression of gallbladder adenocarcinoma. Pathol Res Pract. 2011;207:472–8.
Zhang X, Hu K, Li CY. Protection against oxidized low-density lipoprotein-induced vascular endothelial cell death by integrin-linked kinase. Circulation. 2001;104:2762–6.
Zhang Y, Zhu X, Bai M, Zhang L, Xue L, Yi J. Maternal deprivation enhances behavioral vulnerability to stress associated with miR-504 expression in nucleus accumbens of rats. PLoS One. 2013;8:e69934.
Li M, Dai FR, Du XP, Yang QD, Zhang X, Chen Y. Infusion of BDNF into the nucleus accumbens of aged rats improves cognition and structural synaptic plasticity through PI3 K-ILK-Akt signaling. Behav Brain Res. 2012;231:146–53.
Acknowledgments
This study was supported by The Foundation of Major International Cooperation Projects of The Science and Technology (2010DFB30300) and the National Natural Science Foundation of China (81272193), National Basic Research Program of China (2010CB833605).
Conflict of interest
All authors declared no conflict of interest.
Author information
Authors and Affiliations
Corresponding authors
Rights and permissions
About this article
Cite this article
Jiang, X., Wang, J., Zhang, K. et al. The role of CD29-ILK-Akt signaling-mediated epithelial–mesenchymal transition of liver epithelial cells and chemoresistance and radioresistance in hepatocellular carcinoma cells. Med Oncol 32, 141 (2015). https://doi.org/10.1007/s12032-015-0595-x
Received:
Accepted:
Published:
DOI: https://doi.org/10.1007/s12032-015-0595-x