Abstract
The Gram-negative bacterial lipopolysaccharide (LPS)-induced sepsis has emerged as major concern worldwide due to the pressing need to develop its effective treatment strategies which is not available yet. LPS is the major causative agent in the pathogenesis of septic shock. In macrophages, LPS interacts with cell surface TLR4 leading to reactive oxygen species (ROS), TNF-α, IL-1β production, oxidative stress and markedly activated the MAPKs and NF-kB pathway. Post cell isolation, the macrophages were subjected to administration with neutralizing antibodies to TLR4 and TNFR1 either alone or in combination prior to LPS challenge. Subsequently, we performed flow cytometric analysis along with Western blots, reactive oxygen species production, and TNF-α, IL-1β release. Outcomes suggested that the dual blockade of TLR4 and TNFR1 was indeed beneficial in shifting the LPS-induced M1 polarization towards M2. Both TLR4 and TNFR1 exhibited dependency during LPS stimulation. Furthermore, the switch towards the M2 phenotype might be responsible for the decreased levels of TNF-α, IL-1β, NO, and superoxide anion and the simultaneous elevation in the activity level of anti-oxidant enzymes like SOD, CAT (catalase), and GSH content in the isolated peritoneal macrophages. Simultaneous blocking of both TLR4 and TNFR1 also showed reduced expression of NF-kB, JNK, and COX-2 by promoting TNFR2-mediated TNF-α signaling. The increased arginase activity further confirmed the polarization towards M2. Thus it may be inferred that dual blockade of TLR4 and TNFR1 might be an alternative therapeutic approach for regulating of sepsis in future.
Similar content being viewed by others
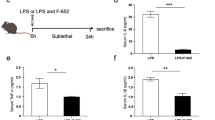
Avoid common mistakes on your manuscript.
Introduction
Lipopolysaccharide (LPS) has been recognized as a major player in the pathogenesis of sepsis and neutralization of receptor for LPS, or inhibition of its downstream signaling pathway is the new treatment strategy in preclinical experiments [1]. The pathological consequences of sepsis include an over-exuberant inflammatory response during which an unrestrained, cytokine-mediated immune exhaustion causes significant organ damage resulting in septic shock, multiple organ failure, and finally death [2]. Inflammation itself is a tightly regulated phenomenon and mainly controlled by the signal either leading to the pro-inflammatory or anti-inflammatory in nature [3]. An imbalance between these two types of signals cause inflammation to go unchecked that leads to oxidative stress and cellular damage. So, the regulation of these two opposing phenomenon is the greatest challenge to the management of LPS-induced sepsis.
The innate immune system serve as the primary defense to the host against inflammation and the macrophages are its frontline warriors [4]. They display a remarkable amount of plasticity in their physiological responses, and by inducing the cytokine storm, LPS-pattern recognition receptor (i.e., TLR4) shows profound effect to the phenotypic alteration of the activated macrophages [5]. On being exposed to inflammatory stimuli, macrophages secrete cytokines such as TNF-α, IL-1β, IL-6, and IL-8 [6]. Such events lead to excessive ROS generation that accumulates within the cellular micro-environment. This directs the macrophages towards the classically activated, pro-inflammatory M1 phenotype by promoting other inflammatory consequences. On the contrary, alternatively activated M2 macrophages express higher levels of anti-oxidant enzymes like SOD, catalase, and glutathione reductase which scavenge the accumulated ROS/NO, thus resisting cell damage and promote healing and tissue repair [7].
It was reported that LPS via binding to Toll like receptor-4 (TLR4) results in pro-inflammatory consequences by releasing TNF-α from stimulated macrophages acting through TNF receptors [8]. With a wide-range spectrum for detection of pathogens by recognizing PAMPs and DAMPs, TLR4 is an obvious target for the treatment of LPS-induced sepsis [9]. Reports confirm that TLR4 recognizes LPS and mediates innate immune responses to the pathogens by regulating the release of pro-inflammatory cytokines. Engagement of both TLRs and TNFRs leads to activation of the transcription factor NF-kB and MAPKs, leading to expression of pro-inflammatory cytokines, chemokines, and microbicidal molecules [10]. It has also been found that TLR4 knockout murine models are resistant to Gram-negative bacteria–induced septic shock [11]. The immune-stimulatory activity of LPS depends on binding to LPS-binding protein (LBP) and CD14 [12]. Upon investigation of LPS-induced sepsis, it has been found that increased TLR4 expression aggravates sepsis by promoting IFN-γ expression [13]. Literature suggests that intraperitoneal LPS administration to animals results in systemic inflammation due to the release of cytokines such as TNF-α and IL-1β by macrophages [14]. Since most of the clinical trials targeting single inflammatory cytokine in the treatment of sepsis failed, therapeutic targeting of TLR4 along with neutralization of pro-inflammatory cytokine receptor looks promising. There was no report available on the dependency of TLR4/TNFR1 at the surface of macrophages in modulating M1/M2 switching during LPS stimulation. Furthermore, TLR4 signaling pathway regulates the expression of COX-2 via Myd88 [15]. Inhibition of COX-2 impedes prostaglandin production that exerts anti-inflammatory responses. Hence, suppression of COX-2 can be considered as an indicator of inflammation resolution [16].
TNF-α has been reported to act through two different cell surface receptors TNFR1 and TNFR2 [17]. They have exhibited differential responses upon binding with its natural ligand TNF-α. As TNF-α signals through two receptors and it is assumed that most pro-inflammatory effects of TNF-α are mediated mainly by TNFR1 [18], our concept is to specifically block this receptor, leaving TNF signaling active by keeping TNFR2 intact. In contrary, binding of TNF-α to TNFR2 initiates signaling pathways that may offer protection against infectious diseases [19]. Sepsis results from an imbalance between the generation of ROS and their subsequent inadequate clearance by endogenous anti-oxidants leading to the oxidative stress [20]. The LPS-TLR4 signaling pathway is characterized by excessive ROS production that includes H2O2, superoxide anion and several pro-inflammatory cytokines. Those reactive free radicals are scavenged by anti-oxidant enzymes such as SOD, catalase, and glutathione peroxidase, thus maintaining the cellular homeostasis [21]. The resulting higher expressions of these anti-oxidants might give rise to a possible alternative treatment option for sepsis. As LPS was reported to be an inducer of M1 macrophages, our hypothesis would be either single (TLR4/TNFR1) or dual (TLR4 + TNFR1) receptor blockade before LPS stimulation in murine peritoneal macrophages might lead to the modulation of phenotypic switching towards anti-inflammatory M2. Since TLR4 expression was increased in macrophages during LPS challenge, particular attention has been made to target TLR4 receptor in LPS. It is conceivable that TLR4 antagonism along with pro-inflammatory mediator blockade (like TNF alpha) might complement each another in search of the most effective therapeutic regimen [22].
Therefore, blocking of cell surface TLR4 to prevent LPS-induced activation along with neutralization of TNFR1 in order to minimize the pro-inflammatory effects induced by its specific ligand TNF-α seems quite relevant for expecting re-polarization of M1 to M2 macrophages as an alternative therapeutic option for controlling LPS-induced inflammation. Moreover, the attenuation of free radical induced damages by modulating the anti-oxidant enzyme activities was also correlated with the phenotypic switching of peritoneal macrophages in response to the neutralization strategy.
Materials and methods
Experimental animals and isolation of murine peritoneal macrophages
All animal experiments were done in adherence to the protocols approved by the Institutional Animal Ethics Committee (IAEC), Department of Physiology, University of Calcutta, under the guidance of Committee for the Purpose of Control and Supervision of Experiments on Animals (CPCSEA) [approval number — IAEC-V/P/BB-1/2019 dated 07.08.2019], Ministry of Environment and Forest, Government of India. Male Swiss albino mice (6–8 weeks of age with body weight of 20 ± 4 g) were injected intraperitoneally with 4% sterile thioglycolate broth, and the resulting peritoneal exudates were harvested with endotoxin-free Hanks’ solution 4 to 5 days later. Peritoneal macrophages were suspended in 0.83% ammonium chloride solution containing 10% (v/v) Tris buffer (pH 7.65) to lyse erythrocytes. Then, the cells were resuspended in Roswell Park Memorial Institute Medium (RPMI) 1640 medium supplemented with 10% FBS, 100 IU/ml penicillin, and 100 μg/ml streptomycin, and allowed to adhere. Non-adherent cells had been removed [23]. Adherent cells viability was determined by Trypan blue dye exclusion technique and when more than 95% of peritoneal macrophages were found to be viable they were used for experiments. For our in vitro study, these adherent cells were pooled from a minimum of 6 mice to obtain the requisite amount of peritoneal macrophages (5 × 106 cells/ml).
Antibody blocking and LPS stimulation
Before conducting the experiment, a dose-dependent study was undertaken and a dose response curve was plotted using different concentrations of LPS. It was found that a dose of 100 ng/ml LPS was optimum to elicit inflammatory responses in macrophages, both in terms of free radical production as well as M1 polarization. Murine peritoneal macrophages (5 × 106 cells/ml) were pre-treated with primary antibodies for TLR4 (Abcam, cat no. ab13867) and TNFR1 at a dose of 10 μg/ml (Biorbyt, cat no. orb27627) [24] either alone or in combination for 30 min prior to LPS stimulation followed by washing off the excess antibody by changing the cell culture media. Then cells were stimulated with LPS (InvivoGen, cat no. tlrl-eklps) at a dose of 100 ng/ml [25] for the time period of 60 min. Prior to performing the FACS analysis, another round of washing was conducted to remove excess LPS from the media.
Analysis and sorting of murine peritoneal M1 and M2 macrophages after LPS stimulation by FACS
Though thioglycolate-elicited macrophages have been used in our study as a control, they are metabolically more active than naïve peritoneal macrophages. Actually we have attempted to elicit the phagocytic capacity and more maturation in our in vitro cultured cells. It is well known that mature macrophages have greater functional efficiency than naïve cells. Considering this to be the baseline, we have performed all FACS M1/M2 gating. Before sorting the cells, we checked the CD1d expression in the isolated cells to ensure our cell purity and nullify the presence of monocyte-derived DCs, and they were found to be negligible.
We have examined the alteration of M1 and M2 macrophage populations by FACS after LPS stimulation to the peritoneal macrophages pre-treated with anti-TLR4 and TNFR1 antibodies either alone or in combination. FACS analysis and sorting of M1/M2 subpopulations were performed using BD-FACS Aria as described earlier [26]. At first pure macrophage population was analyzed by gating CD11b+ cells by using anti-CD11b antibody conjugated with PerCP-Cy5.5 (Thermo Fisher Scientific, cat no. 45–0112-82) and then by using M1-specific marker anti-CD86 antibody conjugated with PE (Thermo Fisher Scientific, cat no. 12–0862-81) and M2-specific marker anti-CD206 antibody conjugated with FITC (Thermo Fisher Scientific, cat no. MA5-16,870) differential analysis and sorting of M1/M2 macrophages were performed. The sorted cells (M1 and M2 macrophages) were centrifuged at 4 °C separately; supernatant and cell free lysate were prepared for further analyses.
Assay for quantification of hydrogen peroxide (H2O2) production
After time-dependent LPS stimulation, supernatants were collected and cell lysates were prepared from the pellet. H2O2 assay of the supernatant was performed. 70 μl of supernatant, 20 μl of Horse Radish peroxidase (HRP) (500 μg/ml), 70 μl of Phenol red (500 μg/ml) and 40 μl medium was added and allowed for incubation for 2 h at 37°C. The reaction was stopped by adding 25 μl of 2 N NaOH, and the absorbance reading was taken at 620 nm. Control set received 40 μl of HBSS in place of supernatant. A standard H2O2 curve was plotted, and H2O2 release in supernatants was evaluated and expressed in μM/106 cells [27].
Assay for quantification of nitric oxide (NO) production
The amount of NO release was determined by the Griess assay. Of M1/M2 supernatants, 50 μl were incubated separately in 40 μM Tris (pH 7.9) containing 40 μM of the reduced form of b-nicotinamide adenine dinucleotide phosphate, 40 μM flavin adenine dinucleotide, and 0.05 U/ml nitrate reductase at 37 °C for 15 min. Reduced samples were incubated with an equal volume of Griess reagent containing of sulphanilamide (0.25% w/v) and N-1-naphthylethylenediamine (0.025% w/v), and the mixture was incubated for 10 min and the absorbance at 550 nm was measured. The total NO release was determined by comparison to a reduced NaNO3 standard curve [28].
Assay for quantification of superoxide anion (O2 −) production
Superoxide anion release assay measures the change in color of cytochrome C (cyt C), when reduced by O2− released from the macrophages pre-treated with or without exogenous SOD (2.78 μg/ml). The difference between the amounts of cyt C reduced in the presence and absence of SOD represents the amount of superoxide anion generated during the incubation. M1 and M2 cell culture supernatants were obtained and incubated in presence of cyt C (100 μl at 2 mg/ml conc.). The production of superoxide anion was monitored spectrophotometrically at 550 nm with reference to the blank. The amount of superoxide anion production will be calculated by the following formula: nM of superoxide anion = (mean absorbance at 550 nm × 15.87) [29].
Lipid peroxidation (LPO) assay
Elevation of LPO level is considered as an indicator of oxidative stress. Lipid peroxidation of the cell supernatants was determined as thiobarbituric acid reactive substances (TBARS). Trichloroacetic acid thiobarbituric acid-hydrochloric acid (TBA-TCA-HCl) reagent was mixed thoroughly with the cell supernatants and heated for 20 min at 80 °C. The sample-containing tubes were then cooled down to room temperature. After centrifugation at 1200 × g for 10 min at room temperature, the absorbance of the pink chromogen present in the clear supernatant was measured in a UV–VIS spectrophotometer at 532 nm. Tetraethoxypropane was taken as a standard, and the values obtained were expressed in terms of nM of TBARS per mg protein [30].
GSH assay
Catalase enzymes, SOD, and glutathione (GSH) play significant roles in scavenging superoxide anion and hydrogen peroxide. These anti-oxidant enzymes resist the production of hydroxyl radical, thus protecting the cell and its constituents from damage due to oxidative stress. SOD performs rapid dismutation of the superoxide anion to H2O2. This H2O2 is then degraded by catalase to produce oxygen and water. Depletion in cellular GSH concentration occurs due to oxidative damage resulting from stress or inflammation. Hence the measurement of cellular GSH is crucial for this experimental study. Reduced glutathione content (as acid soluble sulfhydryl) was estimated by its reaction with DTNB (Ellman’s reagent) following the method of Sedlak and Lindsay with some modifications. A mixture of 0.3 ml of the sample and 0.3 ml 10% TCA was vortexed, and the mixture was centrifuged for 10 min at 40 °C at 5000 rpm. A 500 μl 0.8 M Tris–HCl was added to 250 μl of the cell supernatant. Subsequently, 25 μl of 5, 5 -dithiobis-2-nitrobenzoic acid (DTNB) was added to the mixture, and the absorbance was measured at 412 nm in an UV–VIS spectrophotometer to know the GSH content. The observations were expressed in terms of nM of GSH per mg protein [31].
Assay of superoxide dismutase (SOD) enzyme activity
The estimation of SOD enzyme activity was crucial for this experimental design as anti-oxidant balance could influence the process of polarization in macrophages through regulation of intracellular ROS by offering protection to the oxidative damage by the modulation of superoxide dismutase (SOD) or catalase activity. For this 100 μl of the cell supernatant was mixed separately with 1.5 ml of a Tris–EDTA-HCl buffer (pH 8.5). Then 100 μl of 7.2 mM/L pyrogallol was added, and the reaction mixture was incubated at 25 °C for 10 min. The reaction was stopped by adding 50 μl of 1 M HCl and absorbance measured at 420 nm. Enzyme activity was expressed in terms of U/mg protein, while one unit was taken to be the amount of enzyme that inhibited the oxidation of pyrogallol by 50% [32].
Assay of catalase enzyme activity
LPS stimulation to macrophages generates inflammatory cytokines leading to ROS production through activation of NADPH oxidase. The host’s defense mechanism is thus exhibited by the levels of anti-oxidant enzymes as well as their activity. Hence, to determine the role of these anti-oxidant enzymes in the neutralization of ROS, we estimated the anti-oxidant enzyme activity in the cell supernatant. Catalase activity in the cell supernatant was determined in a spectrophotometer by measuring the decrease in H2O2 concentration at 440 nm. At time zero, 100 μl of the supernatant was added separately to 2.89 ml of potassium phosphate buffer (pH 7.4) taken in a quartz cuvette. Next, 0.1 ml of 300 mM H2O2 was added, and absorbance was taken at 240 nm for 5 min at 1 min intervals. Catalase activity was expressed in terms of nM/min mg protein [33].
Assay of glutathione reductase enzyme activity
The glutathione reductase activity was measured following the oxidation of NADPH to NADP+ during the reduction of oxidized glutathione. The reaction was initiated by the addition of sample in a quartz cuvette containing 1.5 ml reaction mixture of 0.3 mM of NADPH and 3 mM oxidized glutathione in 0.2 M K2HPO4 buffer (pH = 7.5). The decrease in absorbance at 340 nm was recorded for 3 min in the spectrophotometer. The glutathione reductase activity was calculated using 6.22, the millimolar extinction coefficient for NADPH at 340 nm. The enzyme activity was expressed in terms of nM NADPH/min/mg of protein [34].
Arginase enzyme activity assay
From the functional point of view, NO and ornithine, correlating to killing (M1) and repairing function (M2) of macrophages, have been regarded by some investigators as the most characteristic molecules of macrophages [35]. After time-dependent LPS stimulation following incubation of macrophages with antibodies to TLR4 and TNFR1 either alone or in combination, supernatants were collected and macrophages were lysed with the addition of 100 μl PBS containing 0.1% (vol/vol) Triton X-100. After addition of 100 μl of 25 mM Tris, 1 mM MnCl2, the collected cell supernatant was heated to 55 °C for 10 min. Once cooled, 200 μl of 0.5 M arginine in PBS (Sigma-Aldrich) was added to the sample solution, which was then incubated at 37 °C for 1 h. The arginase reaction was stopped by the addition of 900 μl of 44.6 N H2PO4, 36 N H2SO4. The reaction solution was then incubated for 30 min at 100 °C after the addition of 40 μl of 9% (vol/vol) isonitrosopropiophenone in ethanol. Absorbance was measured at 550 nm. A standard curve generated using two-fold serial dilutions of 200 mM urea was used to quantify the assay [36].
Western blot analysis of TLR4, TNFR1, TNFR2, NF-kB, SAPK/JNK, COX-2 expressions
The sorting of macrophages with or without TLR4 and TNFR1 blocking yielded variable populations of M1 and M2 in different groups. Then the equal numbers of M1 and M2 macrophages were cultured separately and lysed with radioimmunoprecipitation assay (RIPA-NP40) buffer and normalized the protein content by Lowry method. Samples containing equal amounts of protein in equal volumes of sample buffer were separated in a denaturing 10% polyacrylamide gel and transferred to a 0.1 mm pore nitrocellulose membrane. Nonspecific binding were blocked with 5% non-fat dry milk. Membrane was then incubated with primary antibodies to TLR4 (Abcam, cat no. ab13867), TNFR1 (Biorbyt, cat no. orb27627), TNFR2 (Biorbyt, cat no. orb224647), COX-2 (Biorbyt cat no. orb106537), SAPK/JNK (Biorbyt, cat no. orb14628), and NF-κB (Biorbyt, cat no. orb10182224) in TBS with 0.1% Tween 20 (TBST). Blots were washed three times in TBST, incubated for 2 h with horseradish peroxidase-conjugated secondary antibodies, developed with the Super Signal chemiluminescent substrate (Thermo Scientific, USA) and exposed to X-OMAT BT films (Kodak). Beta-tubulin was used as loading control to ensure equal loading of samples throughout the gel.
Cytokines (TNF-α, IL-1β) ELISA
TNF-α, IL-1β release were quantified from the cell culture supernatant of M1 and M2 macrophages by Sandwich ELISA technique. The supernatants from different groups were normalized to the protein content by Lowry method before ELISA and the levels of TNF-α (Cat No. ELM-TNFa) and IL-1β (Cat No. ELM-IL1b) were determined in accordance with the manufacturer’s guidelines by using BioRad ELISA Reader at 450 nm.
Statistical analysis
Isolated peritoneal macrophages (PMs), from 5 mice, were pooled together to obtain the requisite amount of cells (5 × 106 cells/ml), and the different parameters were measured. This was repeated thrice for each parameter, and the mean value of these triplicate experiments was taken for calculation. The number of cells was equal for each assay. Data was expressed as mean ± SD. Two-way ANOVA (analysis of variance) was performed for comparing all the groups. In all the cases the significance level was p < 0.05. Scheffe’s F-test had been done as a post hoc test for multiple comparisons of different group means when significant F values were found. All analyses were done using Origin Pro 8 software.
Results
LPS stimulation promotes M1 phenotype while combinatorial blocking of TLR4 and TNFR1 favors M1 to M2 switching in peritoneal macrophages
Flow cytometric sorting data of peritoneal macrophages (Fig. 1a) showed that M1 phenotypic population was significantly increased in LPS treated cells compared to the control macrophages (p < 0.05). The single pre-treatment of TLR4 Ab and TNFR1 Ab in LPS stimulated cells markedly reduced M1 population compared to the LPS stimulated cells but dual blocking (TLR4Ab + TNFR1Ab + LPS) exhibits (Fig. 1b) most potent diminution of M1 phenotype with respect to both of single pre-treatments (p < 0.05).
Flow cytometric sorting of peritoneal macrophages using CD11b-PerCP Cy5.5, CD86-PE and CD206-FITC antibodies after single or dual blocking of TLR4 and TNFR1. a Dot plot images of M1/M2 macrophage sorting. b Graphical representation of CD86+ M1 population. c Graphical representation of CD206+ M2 population in five different groups. Data were represented as mean ± SD from three independent experiments. All the differences were statistically significant at p < 0.05 level. “*” indicates significant difference in comparison to control, “#” indicates significant difference in comparison to LPS, “^” indicates significant difference with respect to TLR4Ab + LPS, “$” indicates significant difference in comparison to TNFR1Ab + LPS
In the case of M2 population opposite scenario was observed during TLR4 and TNFR1 blocking. M2 macrophage population was significantly diminished after LPS stimulation (p < 0.05). When cells were pre-treated with TLR4 Ab and TNFR1 Ab either alone (TLR4Ab + LPS, TNFR1Ab + LPS) or in combination (TLR4Ab + TNFR1Ab + LPS), a sharp augmentation of M2 macrophages was observed in all the cases compared to the LPS stimulated cells (p < 0.05). Moreover, combine pre-treated cells exhibit highest population of M2 cells with respect to the both single pre-treatments (p < 0.05) as depicted from Fig. 1c.
LPS induced higher H2O2, NO and superoxide anion production by M1 macrophages are diminished after M2 polarization
From Fig. 2a, it is seen that M1 macrophages release high amount of hydrogen peroxide on stimulation with LPS (p < 0.05). But on blocking of TLR4 and TNFR1 individually or together, H2O2 release was significantly decreased (p < 0.05). Moreover, the M2-polarized macrophages showed significantly lower levels of H2O2 compared to the M1 population (p < 0.05). Dual blocking of TLR4 and TNFR1 in the M2-polarized macrophages (TLR4Ab + TNFR1Ab + LPS) was observed to significantly diminish H2O2 production (p < 0.05).
Effect of dual blocking of TLR4 and TNFR1 on the release of H2O2 (a), NO (b), and superoxide anion (c), from both M1- and M2-polarized macrophages. Macrophages (5 × 106 cells/ml) were allowed to interact with LPS at 37 °C for 60 min in the presence or absence of anti-TLR4 and anti-TNFR1 antibody. Results were represented as mean ± SD from three independent experiments. The differences were statistically significant at p < 0.05 level. “*” indicates significant difference in comparison to control, “#” indicates significant difference in comparison to LPS, “^” indicates significant difference with respect to TLR4Ab + LPS, “$” indicates significant difference in comparison to TNFR1Ab + LPS, and “@” implies significant difference between M1- and M2-polarized cells at p < 0.05 level
M1 macrophages produced significantly higher amounts of NO and superoxide anion in response to LPS stimulation (Fig. 2b, c), but in both cases dual blocking of TLR4 and TNFR1 in M1 exhibited significantly lower (p < 0.05) amounts of those free radical production when comparing with the LPS stimulated cells (LPS) as well as single pre-treated cells (TLR4Ab + LPS, TNFR1Ab + LPS).
LPS-induced surge in the lipid peroxidation (LPO) levels in M1 macrophages plummet after M2 polarization
The level of lipid peroxidation was found to be significantly higher in the LPS treated M1 macrophages in comparison to the control group (p < 0.05) (Fig. 3a). On blocking of the receptors individually or in combination (TLR4Ab + LPS/ TNFR1Ab + LPS or TLR4Ab + TNFR1Ab + LPS) levels of lipid peroxidation showed a downfall demonstrating decreased oxidative stress (p < 0.05).
Effect of dual blocking of TLR4 and TNFR1 on the level of lipid peroxidation (LPO activity) (a) and production of reduced glutathione (b) from both M1- and M2-polarized macrophages. Macrophages (5 × 106 cells/ml) were allowed to interact with LPS at 37 °C for 60 min in the presence or absence of anti-TLR4 and anti-TNFR1 antibody. Results were represented as mean ± SD from three independent experiments. The differences were statistically significant at p < 0.05 level. “*” indicates significant difference in comparison to control, “#” indicates significant difference in comparison to LPS, “^” indicates significant difference with respect to TLR4Ab + LPS, “$” indicates significant difference in comparison to TNFR1Ab + LPS, and “@” implies significant difference between M1- and M2-polarized cells at p < 0.05 level
The amount of lipid peroxidation was significantly diminished in all respective groups of M2-polarized cells with respect to their M1 counterparts (p < 0.05). Combined pre-treatment group was found to be most effective in curtailing LPS-induced lipid peroxidation in M2 macrophages (p < 0.05).
Reduced glutathione (GSH) content in the M1 macrophages rises after the phenotypic shift towards M2
On single receptor blocking (TLR4Ab + LPS and TNFR1Ab + LPS) in the M1 population, cellular GSH content showed significant increase compared to the control group (p < 0.05). But simultaneous blocking of both the receptors together (TLR4Ab + TNFR1Ab + LPS) was found to be most effective in increasing the levels of cellular GSH (p < 0.05) (Fig. 3b).
Significantly lower levels of GSH were recorded in the LPS stimulated M2 population in comparison to the controls (p < 0.05). GSH levels were also seen to be much greater in combination blockade (TLR4Ab + TNFR1Ab + LPS) compared to the controls (p < 0.05) in the M2 macrophages. GSH expression was significantly increased on dual blocking in comparison to single receptor blocking (TLR4 + LPS and TNFR1 + LPS) (p < 0.05).
Anti-oxidant enzyme activities of SOD, catalase, and glutathione reductase were upregulated in the M2-polarized macrophages
From Fig. 4a, it was found that activity of superoxide dismutase (SOD) in the M1 macrophage population was significantly (p < 0.05) lower compared to the M2 population. On treatment with LPS, M1 macrophages showed decreased SOD activity (p < 0.05). On the other hand, LPS-stimulated M2 macrophages exhibited significantly lower levels of SOD activity compared to the control group (p < 0.05). Furthermore, dual blocking of both the receptors (TLR4Ab + TNFR1Ab + LPS) promoted significant increase in the activity of SOD (p < 0.05) compared to single receptor blocking.
Effect of dual blocking of TLR4 and TNFR1 on the activities of SOD (a), CAT (b), and glutathione reductase (c) enzymes from both M1- and M2-polarized macrophages. Macrophages (5 × 106 cells/ml) were allowed to interact with LPS at 37 °C for 60 min in the presence or absence of anti-TLR4 and anti-TNFR1 antibody. Results were represented as mean ± SD from three independent experiments. The differences were statistically significant at p < 0.05 level. “*” indicates significant difference in comparison to control, “#” indicates significant difference in comparison to LPS, “^” indicates significant difference with respect to TLR4Ab + LPS, “$” indicates significant difference in comparison to TNFR1Ab + LPS, and “@” implies significant difference between M1- and M2-polarized cells at p < 0.05 level
On LPS treatment, catalase activity was remarkably dwindled in the M1 macrophages compared to the control group (p < 0.05) (Fig. 4b). Single receptor blocking (TNFR1Ab + LPS) and combination blockade (TLR4Ab + TNFR1Ab + LPS) resulted in significant improvement of catalase enzyme activity in the M1 population (p < 0.05). In contrast, the M2 macrophages recorded elevated SOD activity than the M1 population. LPS-stimulated M2 macrophages were seen to have significantly lower SOD expression than the control group (p < 0.05). SOD activity was noticeably escalated when both the receptors were blocked (TLR4Ab + TNFR1Ab + LPS) in comparison to single receptor blocking (TLR4Ab + LPS and TNFR1Ab + LPS) (p < 0.05).
Glutathione reductase activity was also measured (Fig. 4c). Observations revealed that the expression was highly suppressed in M1 macrophages due to oxidative stress but soared up in the M2 population (p < 0.05). LPS treatment mitigated glutathione reductase activity in the M2 cells compared to the control group but blocking of both TLR4 as well as TNFR1 receptors simultaneously (TLR4Ab + TNFR1Ab + LPS) resulted in the surge of the enzyme activity particularly in the M2 macrophages(p < 0.05).
Lower arginase activity in the LPS-induced M1 macrophages is elevated after M2 polarization
From Fig. 5, it is seen that LPS stimulation significantly downregulated arginase activity in the M1 population in comparison with the control group (p < 0.05). Blocking of the receptors, individually (TLR4Ab + LPS and TNFR1Ab + LPS) or in combination (TLR4Ab + TNFR1Ab + LPS), led to the significant increase in the arginase enzyme activity (p < 0.05).
Effect of dual blocking of TLR4 and TNFR1 on the activity of arginase enzyme from both M1- and M2-polarized macrophages. Macrophages (5 × 106 cells/ml) were allowed to interact with LPS at 37 °C for 60 min in the presence or absence of anti-TLR4 and anti-TNFR1 antibody. Results were represented as mean ± SD from three independent experiments. The differences were statistically significant at p < 0.05 level. “*” indicates significant difference in comparison to control, “#” indicates significant difference in comparison to LPS, “^” indicates significant difference with respect to TLR4Ab + LPS, “$” indicates significant difference in comparison to TNFR1Ab + LPS, and “@” implies significant difference between M1- and M2-polarized cells at p < 0.05 level
On the contrary, the macrophages that polarized towards the M2 phenotype expressed higher enzyme activity compared to the M1 type (p < 0.05). LPS stimulation to the M2 macrophages caused significant lowering of arginase enzyme activity compared to the control group (p < 0.05). Single (TLR4Ab + LPS and TNFR1Ab + LPS) or dual receptor blockade (TLR4Ab + TNFR1Ab + LPS) in the M2 population resulted in increased arginase activity (p < 0.05) in the M2 cell population.
Dual blocking of TLR4 and TNFR1 mitigates LPS-induced upregulation of their individual expressions along with COX-2, NF-kB, and SAPK/JNK expressions both in M1 and M2 macrophages
From Fig. 6 it was observed that LPS stimulation significantly upregulated both of TLR4 and TNFR1 expression in M1 macrophages compared to the control M1 (p < 0.05). However, TLR4 blocking and TNFR1 blocking markedly reduced their expressions in respective single pre-treatment groups (TLR4Ab + LPS and TNFR1Ab + LPS) compared to the LPS stimulated M1. Dual blocking (TLR4Ab + TNFR1Ab + LPS) downregulated both of those receptors expression with respect to that of LPS, TLR4Ab + LPS, and TNFR1Ab + LPS groups significantly (p < 0.05).
Western blot analysis of TLR4, TNFR1, TNFR2, COX-2, NF-kB, and SAPK/JNK expression from sorted M1 macrophages. Receptor expression patterns of M1-polarized macrophages. Whole cell lysates were prepared for analysis of TLR4, TNFR1, TNFR2, COX-2, NF-kB, and SAPK/JNK expression after 60 min of LPS stimulation. All the samples were probed using β-tubulin to ensure equal protein loading. The differences were statistically significant at p < 0.05 level. “*” indicates significant difference in comparison to control, “#” indicates significant difference in comparison to LPS, “^” indicates significant difference with respect to TLR4Ab + LPS, “$” indicates significant difference in comparison to TNFR1Ab + LPS
Moreover it was also seen that TNFR2 expression significantly declined in the single pre-treatment groups (TLR4Ab + LPS and TNFR1Ab + LPS) as well as in dual blockade (TLR4Ab + TNFR1Ab + LPS) when compared to the control groups (p < 0.05). M1 macrophages further exhibited reduced expression of COX-2 on blockade of TLR4 or TNFR1 singly or both in comparison to the LPS-treated macrophages. NF-kB expression was also upregulated on LPS stimulation. But dual blockade of both TLR4 and TNFR1 together showed lower expression of the same. Furthermore, dual blocking (TLR4Ab + TNFR1Ab + LPS) was effective in reducing SAPK/JNK expression in the M1 macrophage population in comparison to the single pre-treatment (TLR4Ab + LPS and TNFR1Ab + LPS) as well as the control groups (p < 0.05).
In case of M2-polarized macrophages, the expression of TLR4 was significantly downregulated on blocking of both receptors simultaneously (TLR4Ab + TNFR1Ab + LPS) (Fig. 7). This observation was also found to be valid in case of TNFR1 expression (p < 0.05). Individual receptor blocking (TLR4Ab + LPS and TNFR1Ab + LPS) as well as simultaneous blocking of the receptors (TLR4Ab + TNFR1Ab + LPS) before LPS treatment showed higher expression of TNFR2 in the M2-polarized macrophages (p < 0.05). Dual receptor blocking (TLR4Ab + TNFR1Ab + LPS) was found to be beneficial in suppressing COX-2 expression in the M2-polarized macrophages in comparison to the blocking of TLR4 and TNFR1 individually. When compared to the control groups, NF-kB and SAPK/JNK demonstrated low levels of expression on single receptor blocking; both the receptors were significantly down regulated on simultaneous blocking of TLR4 and TNFR1 together (TLR4Ab + TNFR1Ab + LPS) that further indicating the switch towards the alternative M2 phenotype.
Western blot analysis of TLR4, TNFR1, TNFR2, COX-2, NF-kB, and SAPK/JNK expression from sorted M2 macrophages. Receptor expression patterns of M2-polarized macrophages. Whole cell lysates were prepared for analysis of TLR4, TNFR1, TNFR2, COX-2, NF-kB, and SAPK/JNK expression after 60 min of LPS stimulation. All the samples were probed using β-tubulin to ensure equal protein loading. “*” indicates significant difference in comparison to control, “#” indicates significant difference in comparison to LPS, “^” indicates significant difference with respect to TLR4Ab + LPS, “$” indicates significant difference in comparison to TNFR1Ab + LPS. All the differences were statistically significant at p < 0.05 level
Combined blocking of TLR4 and TNFR1 shows attenuation of LPS-induced TNF-α, IL-1β production in M2 macrophages compared to the single blocking
From Fig. 8, it could be said that pro-inflammatory cytokines like TNF-α and IL-1β showed almost identical patterns of free radical release assay. Briefly, the LPS-induced release of TNF-α and IL-1β was drastically reduced after dual receptor (TLR4 and TNFR1) blocking in both M1 and M2 cells. Similarly, a significant reduction of those cytokines was observed in all the groups of M2-polarized macrophages with respect to their M1 counterparts (p < 0.05). The combined blocking group (TLR4Ab + TNFR1Ab + LPS) was also proved to be most efficient in controlling pro-inflammatory cytokines production (both TNF-α and IL-1β) compared to the single blockings (TLR4Ab + LPS, TNFR1Ab + LPS).
Effect of dual blocking of TLR4 and TNFR1 on the production of TNF-α and IL-1β, from both M1- and M2-polarized macrophages. Macrophages (5 × 106 cells/ml) were allowed to interact with LPS at 37 °C for 60 min in the presence or absence of anti-TLR4 and anti-TNFR1 antibody. Results were represented as mean ± SD from three independent experiments. The differences were statistically significant at p < 0.05 level. “*” indicates significant difference in comparison to control, “@” indicates significant difference in comparison to LPS, “#” indicates significant difference with respect to TNFR1Ab + LPS, and “$” indicates significant difference in comparison to TLR4Ab + LPS at p < 0.05 level
Discussions
LPS stimulation leads to the induction of systemic inflammation that mimics a number of clinical features of sepsis including extensive production of pro-inflammatory cytokines such as TNF-α, and IL-1β. Despite significant advancements in the healthcare sector in the modern era, sepsis still poses a major threat to medical professionals and researchers alike. Epidemiological studies have demonstrated that sepsis accounts for more patient admissions than those for diseases like myocardial infarction and stroke [37]. Sepsis, further, is one of the leading causes of death in intensive care units of hospitals around the world due to its high morbidity and mortality [38]. Thus it is absolutely necessary to develop effective strategies against sepsis. Administration of widely accepted broad spectrum antimicrobials have resulted in multiple side effects such as kidney damage among other complicacies [39]. The use of conventional antibiotics, anti-endotoxin antibodies, and other therapeutic options were found to be insufficient for septic patients [40] which opens room for further investigation in this area in search of an alternative therapeutic approach to treat and cure LPS-induced fatal diseases. The paramount role of macrophages is well known in sepsis. LPS stimulation is capable of causing heterogeneous phenotypic alterations in macrophage populations [41]. The classically activated M1 macrophages are characterized by the inflammatory responses resulting to their destructive nature, while, on the other hand, the M2 phenotype is considered to be the “repair” type macrophages involved in tissue repair and resolving inflammation.
TLR4 recognizes LPS and is involved in the regulation of pro-inflammatory cytokines like TNF-α. Signaling of TNF-α via TNFR1 is believed to mediate majority of the pro-inflammatory effects, while TNFR2 diminishes inflammatory responses and promotes survival [42]. We did not find any report on dependency of TLR4/TNFR1 or any cross talking at the surface of macrophages and its role on M1/M2 polarization of LPS-stimulated macrophages. However, both of TLR4 and TNFR1 are involved in LPS-mediated signaling in macrophages and might have some roles on M1/M2 switching in macrophages. In our study, we attempted to regulate the severity of LPS-induced inflammation by in vitro blocking of cell surface TLR4 and TNFR1 in murine peritoneal macrophages. We also analyzed whether single (TLR4/TNFR1) or dual (TLR4 + TNFR1) receptor blocking could facilitate the switching of the LPS-induced M1 macrophages to the immunomodulatory M2 phenotype by significantly suppressing the production of additional inflammatory mediators like cytokines and free radicals. LPS binding to TLR4 activates NF-kB causing the generation of several inflammatory molecules [43]. Since most of the clinical trials targeting single inflammatory cytokine in the treatment of sepsis failed, therapeutic targeting of Toll-like receptor 4 looks promising [44, 45]. However, analysis of mice with targeted disruptions in their tlr2 or tlr4 genes revealed that TLR4 knockouts, but not TLR2 knockouts, are LPS unresponsive again suggesting in favor of targeting TLR4 in LPS sepsis [46]. One possible explanation for the discrepancies related to TLR2 use by LPS has also been reported [47]. Therefore, our approach towards neutralization of TLR4 in macrophages during LPS stimulation and its effects on M1/M2 polarization is relevant in the sense of regulating LPS-TLR4 signaling in macrophages. It is well-established that cell surface CD86 is a M1 marker, while CD206 is an exclusive marker for M2 macrophages [48]. Flow cytometric sorting data implied that LPS stimulation promotes CD86+ M1 switching possibly by increasing the free radicals like NO, superoxide anion production. Moreover, the higher levels of TNF-α, IL-1β also supported the inflammatory cellular micro-environment [49]. Elevated levels of H2O2 in the M1 macrophages and higher lipid peroxidation (LPO) levels indicate the ROS generation due to the bactericidal inflammatory reaction brought about by the M1 macrophages [50]. NF-kB plays a vital role in inflammatory responses which makes it a potential target for the development of anti-inflammatory drugs and therapies. In fact, it has been reported that small molecules that inhibit NF-kB activation are potential targets for therapeutic interventions [51]. LPS binding to TLR4 initiates downstream signaling pathways including c-Jun N-terminal kinase (JNK) besides NF-kB which is evident from the increased expressions of these proteins in the Western blot analysis data obtained from the CD86+ population. Depletion in the anti-oxidant enzyme levels like SOD, catalase, and glutathione reductase in M1 macrophages further indicates increased oxidative stress in the cell population proving the inflammatory circumstances.
Binding of TNF-α to TNFR1 activates the NF-kB and JNK pathways, leading to transcriptional activation of genes encoding pro-inflammatory proteins [52,53,54], thereby suggesting an implication of TNFR1/ TNF-α signaling in the modulation of M1/M2 polarization in LPS-induced sepsis. Although TNF-α is produced during early LPS sepsis and TNF-α can contribute to M1/M2 switching via the TNFR1 signaling pathway, but whether blockade of TNFR1 signaling or TNFR1 deficiency could also affect LPS-induced polarization of M1/M2 macrophages in mice has not been done so far. Therefore blocking of TLR4 to prevent its activation by the signature ligand LPS, along with neutralization of TNFR1 in order to minimize the pro-inflammatory effects induced by its specific ligand TNF alpha, seems quite relevant for studying M1/M2 switching during LPS sepsis and might not demand alternative options. However, in ongoing studies, some relevant alternative options will be definitely taken care of for better understanding the underlining mechanism. By blocking the TLR4 and TNFR1 receptors individually or together, we observed a rise in the CD206+ cell population. This is a clear indication of the switching towards the M2 phenotype [55]. The data obtained from the flow-cytometric sorting further establishes the fact that dual blocking of the receptors (TLR4Ab + TNFR1Ab) results in the polarization of M2 at the optimum level. The blocking of TLR4 and TNFR1, individually or together, aids the polarization of the macrophages towards M2 is also evident from the protein expressions obtained by the Western blot analysis. TNFR2, which is known to be involved in immunosuppression, showed significantly elevated expressions in the CD206+ M2 population on single or dual blocking of the receptors. The shift towards the immunomodulatory M2 type can also be approved by the reduced expression of pro-inflammatory indicators like SAPK/JNK and COX-2 which are highly suppressed in the macrophages subjected to combined blockade. The anti-oxidative enzymes showed increased concentrations in the CD206+ population indicating reduction in the oxidative stress that led to the fall in the cellular lipid peroxidation. Measuring the arginase enzyme activity in both macrophage populations, the M1 macrophage cells exhibited lower levels compared to the M2-polarized cells which establishes further the switch over to M2 [55]. Literature suggests that the metabolism of L-arginine is a key player in macrophage polarization. That the anti-inflammatory M2-type macrophages produce significant levels of ornithine via arginase [50] can be predicted from the increased arginase activity in the M2-polarized cell population. L-arginine is the sole physiological substrate for nitric oxide synthase (NOS) reaction which makes the regulation of its availability as a determining factor in the cellular production of NO [56]. Though we have not measured the levels of IFN-γ in M1 or M2-polarized macrophages, it was reported that in vitro stimulation of LPS along with IFN-γ could lead to the classically activated (M1) macrophages [57]. However, Gordon’s group proved that microbial LPS and IFN-γ polarize macrophages to M1 [58]. We have found lower levels of TNF-α and IL-1β after the dual blockade of TLR4 and TNFR1 that might be responsible for the phenotypic switching towards anti-inflammatory M2. So, it could be speculated that IFN-γ level might also be diminished in M2 macrophages compared to the classically activated M1 type. It was also reported that LPS and IFN-γ probably works synergistically in favor of M1 switching [59]. Therefore, in our current study dual receptors block might able to attenuate the LPS induced excessive production of IFN-γ like TNF-α and IL-1β to facilitate the M2 switching.
Western blot analysis also confirmed the successful blocking of individual receptors and indicated interdependency between TLR4 and TNFR1 by attenuating each other’s expression during LPS stimulation as supported by a previous literature indicating dynamic crosstalk between TLR4, TNFR1, and IL-1R signaling via NF-kB [60]. The downregulation of TLR4 and TNFR1 receptors warranted protection in terms of reduced H2O2, NO, and superoxide anion release as well as lower cytokine levels. The diminished levels of these pro-inflammatory cytokines upholds the fact that the M1 macrophages shifted towards M2 delivering protection against LPS-induced inflammation [61]. Previous investigations also confirm inverse functions of iNOS and arginase towards inflammatory responses. High levels of ornithine production via arginase promote cell proliferation and heal tissue damage by repair type responses. Thus dominant ornithine production can be predicted from increased arginine enzyme activity in the M2 macrophages [62]. Low levels of SOD and catalase in the LPS-induced M1 population and the upregulated expression of the same in the CD206+ population were also observed during dual receptor blocking which further confirms that a shifting took place causing a rise in the levels of these anti-oxidant enzymes [26]. High NO levels further depleted cellular GSH levels and glutathione reductase activity due to increased oxidative stress [63], but these effects were mitigated, and remarkably higher levels of GSH and glutathione reductase were confirmed that blocking of the receptors (TLR4, TNFR1) led to the phenotypic switching from the pro-inflammatory M1 towards the anti-inflammatory M2 type. In a nutshell, the M1 macrophages exhibited lower expression of anti-oxidants like SOD and CAT which failed to control the accumulation of ROS due to its excessive production compared to the anti-oxidant enzymes. As a consequence, the lipid peroxidation in the cell membrane hiked leading to cellular damage as well as stimulation of inflammatory pathways mediated through NF-kB which is evident from the Western blot analyses. On the contrary, we found a higher M2-polarized cell population following dual blockade of both receptors (TLR4 + TNFR1). It leads to increased cellular arginase activity that inhibits the NO pathway probably by the suppression of iNOS. This further triggers the activity of glutathione reductase that converts oxidized glutathione to its reduced form (GSH).
Simultaneous blocking of both TLR4 and TNFR1 also showed reduced expression of NF-kB, JNK, and COX2 by promoting TNFR2 mediated TNF-α signaling. All these events together ultimately maintains a redox balance within the cellular environment (Fig. 9). Therefore the polarization of M2 macrophages was found to be beneficial in terms of LPS-induced regulation of inflammation and in protecting the host from sepsis. Figure 9 (schematic representation) describes the possible mechanism of macrophage M2 switching after neutralization of TLR4 and TNFR1 prior to the LPS stimulation. During LPS stimulation, it binds to the surface TLR4 leading to the release of TNF-α from the macrophages. The TNF-α then binds to the TNFR1 as well as the TNFR2 receptors. This LPS-TLR4, TNF-α-TNFR1 bindings lead to the excessive ROS and pro-inflammatory cytokine production within the cell. Such events trigger inflammatory consequences ultimately leading to the activation of the transcription factor NF-kB. The expression of TNFR2 is found to be diminished. However, the accumulation of ROS/NO in the cellular micro environment increases the level of lipid peroxidation due to the lower anti-oxidant scavenging of H2O2, NO and superoxide (O2−) anion. Moreover, the resulting oxidative stress could promote M1 switching and causes cellular damage which ultimately leads to septic shock. On the other hand, the binding of LPS and TNF-α in peritoneal macrophages is hampered by the neutralization of TLR4 and TNFR1. The TNFR2 pathway is kept functional and showed increased activity via binding with TNF-α. The dual blocking of receptors lead to the higher activities of anti-oxidant enzymes like SOD, CAT, and GRx which efficiently scavenge the ROS and resulting in reduced oxidative stress. Lipid peroxidation level thus falls. The arginase enzyme activity is also increased, thereby suppressing NO production as well as NF-kB activation. All these anti-inflammatory events confer protection via triggering macrophage M2 switching which might be crucial for the treatment of LPS sepsis.
Schematic representation of the experimental study. From the figure, it is seen that isolated peritoneal macrophages are stimulated with LPS. Two phenotypically distinct cell populations arise -M1 and M2. The TLR4 and TNFR1 receptors in the M2 macrophages are blocked with their respective antibodies, while those in the M1 macrophages are left undisturbed. The M1 macrophages exhibit inflammatory responses causing excessive free radicals and inflammatory cytokine production leading to increased lipid peroxidation levels and ROS generation and ultimately promoting inflammation via the NF-kB pathway. On the contrary, receptor blocking on the M2 macrophages suppresses ROS generation and rise in the activities of anti-oxidant enzymes which scavenge the free radicals and decrease oxidative stress by promoting cell survival. Furthermore, simultaneous blocking of both TLR4 and TNFR1 also showed reduced expression of NF-kB, JNK, and COX2 by promoting TNFR2-mediated TNF-α signaling leading to the shifting towards M2 polarization
There might be other alternative option to block IFN-γ/IFN-γR interaction along with TLR4 blocking as a regulatory mechanism of sepsis. As IFN-γ and LPS have some synergistic interaction in the promotion of M1 phenotype [59], blocking of these two important receptors (TLR4 and IFN-γR) might able to potentiate M2 switching. However, the degree or nature of M2 polarization may differ from our current study. In our future study we will definitely consider the IFN-γ-mediated immunomodulation in terms of macrophage polarization. Moreover, we are trying to develop an in vivo sepsis model to validate this IFN-γ/IFN-γR-related aspect in future.
Gram-negative bacterial infection caused accumulation of substantial amounts of IFN-γ within a 3-h time frame in the sera of mice in a TLR4-dependent manner. Moreover, TLR4-specific blockade impedes IFN-γ release from human monocytes upon Gram-negative bacterial infection. It has been suggested that time-dependent accumulation of inflammatory TLR signals encompassing one signal that “ switches on” second-line TLR2-specific sensitivity, which might depend on first-line TLR4 activation upon a Gram-negative bacterial insult, again suggesting initial blocking of TLR4 [64]. Therefore, effective interference with pattern recognition concomitant with mediator blockade might be possible in LPS sepsis after infection. It is conceivable that TLR4 antagonism (as hypothesized in our study), as well as mediator blockade (for TNF-α) and other concepts of LPS sepsis pathology inhibition might have to match with each other or complement one another in search of the most effective therapy.
Hence our study demonstrates a redox balance that might be incorporated as a therapeutic strategy in terms of receptor neutralization. The overall reduction of the pro-inflammatory mediators might be responsible for M1 to M2 switching in macrophages and ultimately confers protection from LPS induced inflammation. This M1/M2 polarization may be considered to be the alternative therapeutic approach in contrast to the existing remedies. Hence, it can be concluded that blocking of TLR4 along with TNFR1 might be an alternative option for regulating LPS-induced inflammation via targeting M1 to M2 switching.
Conclusions
As discussed above, the M1/M2 paradigm also applies to immune regulation, with M1 inducing and M2 preventing inflammatory diseases, respectively. An imbalance of macrophage M1–M2 polarization has been reported to be associated with LPS sepsis. Therefore, from the in vitro study, we are interested to figure out the molecules associated with the dynamic changes of M1/M2 polarization and to understand the effects of dual receptor blocking particularly for TLR4 (for LPS sensor) and TNFR1 (for TNF-α) before LPS stimulation. Then to further elucidate the effects of M1/M2 switching in LPS sepsis progression, we will definitely test this hypothesis in an animal model of sepsis for designing novel macrophage-mediated therapeutic strategies against sepsis. Limited information is available regarding the exact pathways and causative factors promoting one phenotype over the other which might be exploited for the therapeutic gain of septic shock patients. Though we have not analyzed the additional parameters like IL-10, iNOS activity/expression outcomes of these parameters would be helpful in determining the inflammatory as well as its regulatory mechanisms more clearly. Further studies might be required in in vivo sepsis model post the neutralization of TLR4 and alternate blocking of TNFR1/TNFR2 receptors to extrapolate our current findings.
References
Wang HD, Lu DX, Qi RB. Therapeutic strategies targeting the LPS signalling and cytokines. Pathophysiology. 2009;16(4):291–6. https://doi.org/10.1016/j.pathophys.2009.02.006.
Hotchkiss RS, Moldawer LL, Opal SM, Reinhart K, Turnbull IR, Vincent JL. Sepsis and septic shock. Nat Rev Dis Primers. 2016;2:45. https://doi.org/10.1038/nrdp.2016.45.
Bennett JM, Reeves G, Billman GE, Sturmberg JP. Inflammation-nature’s way to efficiently respond to all types of challenges: implications for understanding and managing “the epidemic” of chronic diseases. Front Med (Lausanne). 2018;5:316. https://doi.org/10.3389/fmed.2018.00316.
Hirayama D, Iida T, Nakase H. The phagocytic function of macrophage-enforcing innate immunity and tissue homeostasis. Int J Mol Sci. 2017;19(1):92. https://doi.org/10.3390/ijms19010092.
Murad S. Toll-like receptor 4 in inflammation and angiogenesis: a double-edged sword. Front Immunol. 2014;5:313. https://doi.org/10.3389/fimmu.2014.00313.
Arango Duque G, Descoteaux A. Macrophage cytokines: involvement in immunity and infectious diseases. Front Immunol. 2014;5:491. https://doi.org/10.3389/fimmu.2014.00491.
Tan HY, Wang N, Li S, Hong M, Wang X, Feng Y. The reactive oxygen species in macrophage polarization: reflecting its dual role in progression and treatment of human diseases. Oxid Med Cell Longev. 2016;2016:2795090. https://doi.org/10.1155/2016/2795090.
Parameswaran N, Patial S. Tumor necrosis factor-α signaling in macrophages. Crit Rev Eukaryot Gene Expr. 2010;20(2):87–103. https://doi.org/10.1615/critreveukargeneexpr.v20.i2.10.
Mogensen TH. Pathogen recognition and inflammatory signalling in innate immune defenses. Clin Microbiol Rev. 2009;22(2):240–73. https://doi.org/10.1128/CMR.00046-08.
Akira S, Uematsu S, Takeuchi O. Pathogen recognition and innate immunity. Cell. 2006;124(4):783–801. https://doi.org/10.1016/j.cell.2006.02.015.
Roger T, Froidevaux C, Le Roy D, Reymond MK, Chanson AL, Mauri D, Burns K, Riederer BM, Akira S, Calandra T. Protection from lethal gram-negative bacterial sepsis by targeting Toll-like receptor 4. Proc Natl Acad Sci U S A. 2009;106(7):2348–52. https://doi.org/10.1073/pnas.0808146106.
Park BS, Lee JO. Recognition of lipopolysaccharide pattern by TLR4 complexes. Exp Mol Med. 2013;45(12):e66. https://doi.org/10.1038/emm.2013.97.
Li Q, Wu C, Liu Z, Zhang H, Du Y, Liu Y, Song K, Shi Q, Li R. Increased TLR4 expression aggravates sepsis by promoting IFN-γ expression in CD38-/- Mice. J Immunol Res. 2019;2019:3737890. https://doi.org/10.1155/2019/3737890.
Alves-Rosa F, Vulcano M, Beigier-Bompadre M, Fernández G, Palermo M, Isturiz MA. Interleukin-1beta induces in vivo tolerance to lipopolysaccharide in mice. Clin Exp Immunol. 2002;128(2):221–8. https://doi.org/10.1046/j.1365-2249.2002.01828.x.
Fukata M, Chen A, Klepper A, Krishnareddy S, Vamadevan AS, Thomas LS, Xu R, Inoue H, Arditi M, Dannenberg AJ, Abreu MT. Cox-2 is regulated by Toll-like receptor-4 (TLR4) signaling: role in proliferation and apoptosis in the intestine. Gastroenterology. 2006;131(3):862–77. https://doi.org/10.1053/j.gastro.2006.06.017.
Lee S, Shin S, Kim H, Han S, Kim K, Kwon J, Kwak JH, Lee CK, Ha NJ, Yim D, Kim K. Anti-inflammatory function of arctiin by inhibiting COX-2 expression via NF-κB pathways. J Inflamm (Lond). 2011;8(1):16. https://doi.org/10.1186/1476-9255-8-16.
Wajant H, Siegmund D. TNFR1 and TNFR2 in the control of the life and death balance of macrophages. Front Cell Dev Biol. 2019;7:91. https://doi.org/10.3389/fcell.2019.00091.
Van Hauwermeiren F, Vandenbroucke RE, Libert C. Treatment of TNF mediated diseases by selective inhibition of soluble TNF or TNFR1. Cytokine Growth Factor Rev. 2011;22(5–6):311–9. https://doi.org/10.1016/j.cytogfr.2011.09.004.
Yang S, Wang J, Brand DD, Zheng SG. Role of TNF-TNF receptor 2 signal in regulatory T cells and its therapeutic implications. Front Immunol. 2018;9:784. https://doi.org/10.3389/fimmu.2018.00784.
Schieber M, Chandel NS. ROS function in redox signaling and oxidative stress. Curr Biol. 2014;24(10):R453–62. https://doi.org/10.1016/j.cub.2014.03.034.
Lü JM, Lin PH, Yao Q, Chen C. Chemical and molecular mechanisms of antioxidants: experimental approaches and model systems. J Cell Mol Med. 2010;14(4):840–60. https://doi.org/10.1111/j.1582-4934.2009.00897.x.
Kuzmich NN, Sivak KV, Chubarev VN, Porozov YB, Savateeva-Lyubimova TN, Peri F. TLR4 signalling pathway modulators as potential therapeutics in inflammation and sepsis. Vaccines (Basel). 2017;5(4):34. https://doi.org/10.3390/vaccines5040034.
Meltzer MS. Peritoneal mononuclear phagocytes from small animals. In: Adams DO, Edelson PJ, Koren HS, editors. Methods for studying mononuclear phagocytes. New York: Academic Press; 1981. p. 63–8.
Dutta P, Sultana S, Dey R, Bishayi B. Regulation of Staphylococcus aureus-induced CXCR1 expression via inhibition of receptor mobilization and receptor shedding during dual receptor (TNFR1 and IL-1R) neutralization. Immunol Res. 2019;67(2–3):241–60. https://doi.org/10.1007/s12026-019-09083-x.
Watanabe K, Jose PJ, Rankin SM. Eotaxin-2 generation is differentially regulated by lipopolysaccharide and IL-4 in monocytes and macrophages. J Immunol. 2002;168(4):1911–8. https://doi.org/10.4049/jimmunol.168.4.1911.
Jablonski KA, Amici SA, Webb LM, Ruiz-Rosado Jde D, Popovich PG, Partida-Sanchez S, Guerau-de-Arellano M. Novel markers to delineate murine M1 and M2 macrophages. PLoS ONE. 2015;10(12):e0145342. https://doi.org/10.1371/journal.pone.0145342.
Leigh PCJ, Van Furth R, Zwet TL. In vitro determination of phagocytosis and intracellular killing by polymorphonuclear neutrophils and mononuclear phagocytes. In: Weir DM, editor. Handbook of Experimental Immunology. Oxford: Blackwell Scientific Publication; 1986. p. 46.1-46.19.
Watanabe I, Ichiki M, Shiratsuchi A, Nakanishi Y. TLR2-mediated survival of Staphylococcus aureus in macrophages: a novel bacterial strategy against host innate immunity. J Immunol. 2007;178(8):4917–25. https://doi.org/10.4049/jimmunol.178.8.4917.
Absolom DR. Basic methods for the study of phagocytosis. Methods Enzymol. 1986;132:95–180. https://doi.org/10.1016/s0076-6879(86)32005-6.
Buege JA, Aust SD. Microsomal lipid peroxidation. Methods Enzymol. 1978;52:302–10. https://doi.org/10.1016/s0076-6879(78)52032-6.
Sedlak J, Lindsay RH. Estimation of total, protein-bound, and nonprotein sulfhydryl groups in tissue with Ellman’s reagent. Anal Biochem. 1968;25(1):192–205. https://doi.org/10.1016/0003-2697(68)90092-4.
Paoletti F, Aldinucci D, Mocali A, Caparrini A. A sensitive spectrophotometric method for the determination of superoxide dismutase activity in tissue extracts. Anal Biochem. 1986;154(2):536–41. https://doi.org/10.1016/0003-2697(86)90026-6.
Aebi H, Wyss SR, Scherz B, Skvaril F. Heterogeneity of erythrocyte catalase II. Isolation and characterization of normal and variant erythrocyte catalase and their subunits. Eur J Biochem. 1974;48(1):137–45. https://doi.org/10.1111/j.1432-1033.1974.tb03751.x.
Goldberg DM, Spooner RJ. Assay of glutathione reductase. In: Bergmeyen HV, editor. Methods of Enzymatic Analysis. 3rd ed. Verlog Chemie: Deerfiled Beach; 1983. p. 258–65.
Weisser SB, McLarren KW, Kuroda E, Sly LM. Generation and characterization of murine alternatively activated macrophages. Methods Mol Biol. 2013;946:225–39. https://doi.org/10.1007/978-1-62703-128-8_14.
Corraliza IM, Campo ML, Soler G, Modolell M. Determination of arginase activity in macrophages: a micromethod. J Immunol Methods. 1994;174(1–2):231–5. https://doi.org/10.1016/0022-1759(94)90027-2.
Wang Q, Zhou X, Yang L, Luo M, Han L, Lu Y, Shi Q, Wang Y, Liang Q. Gentiopicroside (GENT) protects against sepsis induced by lipopolysaccharide (LPS) through the NF-κB signaling pathway. Ann Transl Med. 2019;7(23):731. https://doi.org/10.21037/atm.2019.11.126.
Sin WX, Yeong JP, Lim TJF, Su IH, Connolly JE, Chin KC. IRF-7 mediates type I IFN responses in endotoxin-challenged mice. Front Immunol. 2020;11:640. https://doi.org/10.3389/fimmu.2020.00640.
Dellinger RP, Levy MM, Rhodes A, Annane D, Gerlach H, Opal SM, Sevransky JE, Sprung CL, Douglas IS, Jaeschke R, Osborn TM, Nunnally ME, Townsend SR, Reinhart K, Kleinpell RM, Angus DC, Deutschman CS, Machado FR, Rubenfeld GD, Webb S, Beale RJ, Vincent JL, Moreno R. Surviving sepsis campaign: international guidelines for management of severe sepsis and septic shock, 2012. Intensive Care Med. 2013;39(2):165–228. https://doi.org/10.1007/s00134-012-2769-8.
Rello J, Valenzuela-Sánchez F, Ruiz-Rodriguez M, Moyano S. Sepsis: a review of advances in management. Adv Ther. 2017;34(11):2393–411. https://doi.org/10.1007/s12325-017-0622-8.
Yang Z, Ming XF. Functions of arginase isoforms in macrophage inflammatory responses: impact on cardiovascular diseases and metabolic disorders. Front Immunol. 2014;5:533. https://doi.org/10.3389/fimmu.2014.00533.
Ebach DR, Riehl TE, Stenson WF. Opposing effects of tumor necrosis factor receptor 1 and 2 in sepsis due to cecal ligation and puncture. Shock. 2005;23(4):311–8. https://doi.org/10.1097/01.shk.0000157301.87051.77.
Liu T, Zhang L, Joo D, Sun SC. NF-κB signaling in inflammation. Signal Transduct Target Ther. 2017;2:17023. https://doi.org/10.1038/sigtrans.2017.23.
Wittebole X, Castanares-Zapatero D, Laterre PF. Toll-like receptor 4 modulation as a strategy to treat sepsis. Mediators Inflamm. 2010;2010:568396. https://doi.org/10.1155/2010/568396.
Medzhitov R, Preston-Hurlburt P, Janeway CA Jr. A human homologue of the Drosophila Toll protein signals activation of adaptive immunity. Nature. 1997;388(6640):394–7. https://doi.org/10.1038/41131.
Takeuchi O, Hoshino K, Kawai T, Sanjo H, Takada H, Ogawa T, Takeda K, Akira S. Differential roles of TLR2 and TLR4 in recognition of gram-negative and gram-positive bacterial cell wall components. Immunity. 1999;11(4):443–51. https://doi.org/10.1016/s1074-7613(00)80119-3.
Hirschfeld M, Ma Y, Weis JH, Vogel SN, Weis JJ. Cutting edge: repurification of lipopolysaccharide eliminates signaling through both human and murine toll-like receptor 2. J Immunol. 2000;165(2):618–22. https://doi.org/10.4049/jimmunol.165.2.618.
Trombetta AC, Soldano S, Contini P, Tomatis V, Ruaro B, Paolino S, Brizzolara R, Montagna P, Sulli A, Pizzorni C, Smith V, Cutolo M. A circulating cell population showing both M1 and M2 monocyte/macrophage surface markers characterizes systemic sclerosis patients with lung involvement. Respir Res. 2018;19(1):186. https://doi.org/10.1186/s12931-018-0891-z.
Turner MD, Nedjai B, Hurst T, Pennington DJ. Cytokines and chemokines: at the crossroads of cell signalling and inflammatory disease. Biochim Biophys Acta. 2014;1843(11):2563–82. https://doi.org/10.1016/j.bbamcr.2014.05.014.
Gupta SC, Sundaram C, Reuter S, Aggarwal BB. Inhibiting NF-κB activation by small molecules as a therapeutic strategy. Biochim Biophys Acta. 2010;1799(10–12):775–87. https://doi.org/10.1016/j.bbagrm.2010.05.004.
Walton RG, Kosmac K, Mula J, Fry CS, Peck BD, Groshong JS, Finlin BS, Zhu B, Kern PA, Peterson CA. Human skeletal muscle macrophages increase following cycle training and are associated with adaptations that may facilitate growth. Sci Rep. 2019;9(1):969. https://doi.org/10.1038/s41598-018-37187-1.
Pfeffer K, Matsuyama T, Kündig TM, Wakeham A, Kishihara K, Shahinian A, Wiegmann K, Ohashi PS, Krönke M, Mak TW. Mice deficient for the 55 kd tumor necrosis factor receptor are resistant to endotoxic shock, yet succumb to L. monocytogenes infection. Cell. 1993;73(3):457–67. https://doi.org/10.1016/0092-8674(93)90134-c.
Hehlgans T, Pfeffer K. The intriguing biology of the tumour necrosis factor/tumour necrosis factor receptor superfamily: players, rules and the games. Immunology. 2005;115(1):1–20. https://doi.org/10.1111/j.1365-2567.2005.02143.x.
Wright TW, Pryhuber GS, Chess PR, Wang Z, Notter RH, Gigliotti F. TNF receptor signaling contributes to chemokine secretion, inflammation, and respiratory deficits during Pneumocystis pneumonia. J Immunol. 2004;172(4):2511–21. https://doi.org/10.4049/jimmunol.172.4.2511.
Rath M, Müller I, Kropf P, Closs EI, Munder M. Metabolism via arginase or nitric oxide synthase: two competing arginine pathways in macrophages. Front Immunol. 2014;5:532. https://doi.org/10.3389/fimmu.2014.00532.
Wang WW, Jenkinson CP, Griscavage JM, Kern RM, Arabolos NS, Byrns RE, Cederbaum SD, Ignarro LJ. Co-induction of arginase and nitric oxide synthase in murine macrophages activated by lipopolysaccharide. Biochem Biophys Res Commun. 1995;210(3):1009–16. https://doi.org/10.1006/bbrc.1995.1757.
Orecchioni M, Ghosheh Y, Pramod AB, Ley K. Macrophage polarization: different gene signatures in M1(LPS+) vs. classically and M2(LPS-) vs. alternatively activated macrophages. Front Immunol. 2019;10:1084. https://doi.org/10.3389/fimmu.2019.01084.
Gordon S, Martinez FO. Alternative activation of macrophages: mechanism and functions. Immunity. 2010;32(5):593–604. https://doi.org/10.1016/j.immuni.2010.05.007.
Müller E, Christopoulos PF, Halder S, Lunde A, Beraki K, Speth M, Øynebråten I, Corthay A. Toll-like receptor ligands and interferon-γ synergize for induction of antitumor M1 macrophages. Front Immunol. 2017;8:1383. https://doi.org/10.3389/fimmu.2017.01383.
Yang SK, Wang YC, Chao CC, Chuang YJ, Lan CY, Chen BS. Dynamic cross-talk analysis among TNF-R, TLR-4 and IL-1R signalings in TNFalpha-induced inflammatory responses. BMC Med Genomics. 2010;3:19. https://doi.org/10.1186/1755-8794-3-19.
Parisi L, Gini E, Baci D, Tremolati M, Fanuli M, Bassani B, Farronato G, Bruno A, Mortara L. Macrophage polarization in chronic inflammatory diseases: killers or builders? J Immunol Res. 2018;2018:8917804. https://doi.org/10.1155/2018/8917804.
Mills CD. M1 and M2 macrophages: oracles of health and disease. Crit Rev Immunol. 2012;32(6):463–88. https://doi.org/10.1615/critrevimmunol.v32.i6.10.
Butzer U, Weidenbach H, Gansauge S, Gansauge F, Beger HG, Nussler AK. Increased oxidative stress in the RAW 264.7 macrophage cell line is partially mediated via the S-nitrosothiol-induced inhibition of glutathione reductase. FEBS Lett. 1999;445(2–3):274–8. https://doi.org/10.1016/s0014-5793(99)00139-8.
Spiller S, Elson G, Ferstl R, Dreher S, Mueller T, Freudenberg M, Daubeuf B, Wagner H, Kirschning CJ. TLR4-induced IFN-gamma production increases TLR2 sensitivity and drives Gram-negative sepsis in mice. J Exp Med. 2008;205(8):1747–54. https://doi.org/10.1084/jem.20071990.
Acknowledgements
The authors are indebted to Department of Science and Technology, Government of India, for providing us with the instruments procured under the DST-PURSE programme to the Department of Physiology, University of Calcutta. The author is indebted to CRNN (BD Bioscience Room), Kolkata, for allowing us to use the FACS facilities.
Author information
Authors and Affiliations
Corresponding author
Ethics declarations
Conflict of interest
The authors declare that they have no conflict of interest.
Additional information
Publisher’s note
Springer Nature remains neutral with regard to jurisdictional claims in published maps and institutional affiliations.
Rights and permissions
About this article
Cite this article
Sawoo, R., Dey, R., Ghosh, R. et al. TLR4 and TNFR1 blockade dampen M1 macrophage activation and shifts them towards an M2 phenotype. Immunol Res 69, 334–351 (2021). https://doi.org/10.1007/s12026-021-09209-0
Received:
Accepted:
Published:
Issue Date:
DOI: https://doi.org/10.1007/s12026-021-09209-0