Abstract
Edible fruits of two indigenous medicinal Ficus species (Ficus sycomorus L. and Ficus burtt-davyi Hutch) collected from eight different sites in South Africa were assessed for nutritional value, elemental concentration, and the possible risk associated with their consumption. The metal concentrations in the fruits and growth soil were determined by inductively coupled plasma-optical emission spectrometry (ICP-OES). The results showed elemental concentrations in the fruits to contribute significantly to recommended dietary allowances and were found to be in decreasing order of Ca > Mg > Fe > Zn > Mn > Cu > Cr and Ca ˃ Mg ˃ Fe ˃ Mn ˃ Zn ˃ Cu for both F. sycomorus and F. burtt-davyi fruits. The results for proximate composition of F. sycomorus fruits were (in %) 55.8 for moisture, 25.3 for carbohydrates, 5.6 for protein, 8.9 for fats, 55.8 for crude fiber, and 4.4 for ash; for F. burtt-davyi fruits, it was (in %) 78.9 for carbohydrates, 5.0 for protein, 8.4 for lipids, 4.0 for crude fiber, and 3.7 for ash. The health risk assessment showed target hazard quotient, and hazard indices for all the studied heavy metals in the fruits for all the sites were to be less than one and the target carcinogenic risk values to be within the acceptable regulatory cancer risk range. This study confirms that the fruits of F. sycomorus and F. burtt-davyi are safe for human consumption due to low non-carcinogenic and carcinogenic adverse health effects.
Similar content being viewed by others
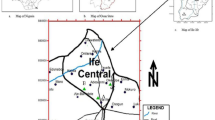
Explore related subjects
Discover the latest articles, news and stories from top researchers in related subjects.Avoid common mistakes on your manuscript.
Introduction
Trees have been an essential part of human survival from the earliest time, providing basic needs such as shelter, firewood, medicine, and food. The use of indigenous plant foods to treat medical conditions of people dates back to time immemorial. Many Southern African trees have edible fruits, most of which are yet to be domesticated and developed into commercial crops [1]. Wild fruit trees are important to rural people, especially children, as they introduce nutrient diversity to the diet in an environment where food choices are limited. In addition, the vitality of fruits to the human diet is linked to health-promoting components such as vitamins, essential minerals, antioxidants, and prebiotics (fibers) [2]. Epidemiological studies have shown an inverse correlation between the consumption of fruits and the incidence of chronic diseases such as cancer [3, 4], diabetes [5], and heart disease [6].
Food safety is a major public health concern, and increased awareness has motivated research into the risks associated with the consumption of contaminated food products, particularly, plant-based food products [7]. The occurrence of heavy metals in soils (natural geological occurrences and anthropogenic inputs) and plant-based foods has been the focus of a number of studies as soil to plant transfer is a major route of contamination [8,9,10,11].
Although the efficacy of medicinal plants as a therapeutic agent is a result of the phytochemical constituents, prolonged ingestion can result in elemental accumulation, if at elevated levels in the plant [12, 13]. Heavy metals have been linked with toxicity associated with environmental pollution due to them being nonbiodegradable, having long biological half-lives (high residence time) and their potential to accumulate in different parts of plants [14,15,16]. Based on this, elemental screening of medicinal plants is paramount for quality control and safety [17, 18].
Phytochemical studies on F. burtt-davyi have shown its fruits to contained chemotherapeutic agents [19]. Furthermore, the fruits of F. burtt-davyi and F. sycomorus are regularly utilized by rural communities in Venda and Eastern Cape, South Africa, for the management of tuberculosis [20] and as a laxative [21], respectively. However, to the best of our knowledge, there have been no reports on the elemental composition and nutritional value of F. burtt-davyi and F. sycomorus fruits. Hence, this study aimed to investigate the elemental distribution and concentrations of 13 elements in the edible fruits of F. burtt-davyi and F. sycomorus and to assess for their nutritional value. Additionally, this study also evaluated the potential health risks associated with the toxic elements via the consumption of the fruits.
Materials and Methods
Sample Collection
Tree-ripened fruit samples were randomly picked from eight different sampling sites within KwaZulu-Natal, South Africa, between February and August for F. sycomorus and F. burtt-davyi (Fig. 1A and B), respectively. Fruit samples were then placed in sealed plastic bags and taken to the laboratory for further analyses. A single flowering plant specimen each for each species was collected at each sampling site for identification. Soil samples at a depth of 15–20 cm from six points along the drip line of each tree were collected randomly from the eight different sampling sites from which the fruits were picked.
Sample Preparation
A botanist, Prof. H. Baijnath, authenticated the plants, and voucher specimens were deposited in the WARD Herbarium of the School of Life Sciences, University of KwaZulu-Natal, Westville Campus, South Africa. Voucher specimen numbers were Ogunlaja, O1 for F. burtt-davyi, and Ogunlaja, O2 for F. sycomorus. All fruit samples were washed thoroughly with doubly distilled water and oven-dried at 50 °C, overnight. Dried fruit samples were crushed using a food processor (Kenwood Compact Blender, BL380), and resultant powder samples were stored in a refrigerator in sealed polyethylene bags until analyzed. A thoroughly mixed representative soil sample was taken from each site and was air-dried then passed through a 2-mm mesh sieve to remove organic matter and gravel. About 10 g of this soil was crushed with a mortar and pestle to reduce particle size for microwave digestion. Samples were stored in sealed plastic bags and kept in a refrigerator until analyzed.
Reagents and Chemicals
All chemicals used were supplied by Merck (Kenilworth, USA) and Sigma-Aldrich (St. Louis, USA) Chemical Companies and were of analytical-reagent grade. Elemental calibration standards were prepared from spectroscopic grade stock standard solutions of 1000 mg L−1 (Sigma-Aldrich, Buchs, Switzerland).
Quality Control and Analytical Quality Assurance
All plastic containers were washed with laboratory liquid detergent then soaked in 1 M HNO3, overnight. Glassware and other equipment were cleaned with 6 M HNO3 and rinsed off with Millipore™ water (Billerica, MA, USA) to minimize the risk of contamination before use. Millipore™ water was used throughout the experiments. Working standards were made up with Millipore™ water and 10 mL of 70% HNO3 to match the sample matrix. Blank reagents and certified reference material (CRMs) for plant (BCR-402) certified by the European Commission under the responsibility of the Institute for Reference Materials and Measurements (IRMM, Brussels, Belgium) and for soil (D081–540, ERA, A waters Company, Milford, MA, USA) were used to verify the accuracy, precision, and efficiency of the analytical method.
Extraction of Exchangeable Metals and Total Metals
The extracting solution was prepared by diluting 38.542 g ammonium acetate (NH4CO2CH3), 25 mL acetic acid (CH3COOH, 96%), and 37.225 g ethylenediaminetetraacetic acid (EDTA) to 1 L in double-distilled water. Exactly 50 mL of extracting solution was added to 5.0 g of dry soil samples in 250 mL polyethylene bottles and shaken in a laboratory shaker for 2 h. Thereafter, solutions were filtered through Whatman No. 1 filter papers and then Millipore 0.45 μm filter membranes to permit analysis of extracted metals. All samples were stored in plastic bottles and kept in a refrigerator until analyzed.
Microwave-assisted closed-vessel technology was used to digest samples as described by Ogunlaja et al. [19] in the dry fruit, soil, and the certified reference material (CRM) samples. Dried fruit, CRM, and soil samples (0.25 g each) were placed in different 50 mL liners with 10 mL of 70% HNO3 and allowed to predigest for 1 h [19]. Digestions were performed using the CEM Microwave Accelerated Reaction System (MARS) 6, (CEM Corporation, Matthews, North Carolina, USA). Digests were transferred to 50 mL volumetric flasks, diluted to the mark with Millipore™ water, and stored in polyethylene bottles prior to elemental analysis. All digestions were performed in triplicate.
Soil pH, Cation Exchange Capacity (CEC), and Soil Organic Matter (SOM)
The pH of soil was determined by measuring the pH of the solution, 1:2.5, dry wt/v using a pH meter (Aqualytica, Model pH 17) fitted with a glass electrode. The cation exchange capacity (CEC) of soil was determined using the pH 7.0 ammonium acetate method [22], while soil organic matter (SOM) was measured according to the procedure adopted from Walkley and Black [23].
Determination of Proximate Chemical Composition
The proximate chemical composition of the fruits (moisture, crude protein, fat, fiber, and crude ash) was determined according to standard methods of analysis, as described by the Association of Official Analytical Chemists [24]. Total carbohydrate content was obtained by difference. All determinations were done in triplicate.
Elemental Analysis
All extracted and digested samples were analyzed for As, Ca, Cd, Co, Cr, Cu, Fe, Mg, Mn, Ni, Pb, Se, and Zn by inductively coupled plasma-optical emission spectrometry (ICP-OES) using the Perkin Elmer Optima™ 5300 Dual View ICP-OES (Billerica, Massachusetts, USA) due to its multielement determination capability, dynamic linear range, and low detection limits. Analytical wavelengths were chosen from the three most sensitive lines that showed no interfering elements and that had minimal spectral or matrix interferences.
Bioaccumulation Factor (BAF)
Generally, elements are persistent in the environment and tend to accumulate in plant tissues. In this study, elemental bioaccumulation was evaluated by comparing their concentration in the fruit against that in the growth soil.
Statistical Analysis
All statistical analyses were performed using the Statistical Package for the Social Sciences (PASW version 24, IBM Corporation, Cornell, NY, USA). Pearson’s correlation analysis was applied to the dataset to quantitatively analyze and confirm the relationship between soil quality parameters and heavy metal concentrations. Principal component analysis was also done [25].
Human Health Risk Assessment
Health risk assessments consist of the identification of hazard, assessment of exposure, dose-response, and characterization of the risk [26]. The health risk assessment of each potentially toxic element (PTE) was quantified using the two toxicity risk indices expressed in terms of carcinogenic risk characterization using the slope factor (SF) and a non-carcinogenic risk characterization using the reference dose (RfD) [27]. In this study, elemental concentrations were used to calculate the estimated daily intake (EDI) of elements, target hazard quotients (THQ), hazard index (HI), and target cancer risk (TCR) separately for adult and children.
Estimated Daily Intake of Toxic Chemical Elements
The daily human exposure was evaluated using the estimated daily intake (EDI) of each element as expressed in Eq. 2 [28]
where, MC, CF, IR, and BW represent the metal concentrations in fruits, the conversion factor (0.208), daily fruit ingestion rate, and average body weight (adult = 60 kg and children = 16 kg), respectively. Fruit ingestion rate (g/person/day) is 50.59 (for adults) and 25.33 (for children) [28, 29].
Target Hazard Quotient
The non-carcinogenic hazard was evaluated by the THQ using Eq. 3 which represents the health risk level by the consumption of fruits.
where RfD is the oral reference dose (mg kg−1/day) for individual heavy metals that humans can be exposed to and for this study were obtained from USEPA [30,31,32]. If THQ ˂ 1 consumption of fruits is considered safe for human health (no possible health risk) but if THQ ≥ 1, there is an unacceptable risk of adverse non-carcinogenic effects on human health [27]. The risk assessments of PTE mixture were computed as the sum of individual THQs to form hazard index (HI):
Target Carcinogenic Risk
The lifetime exposure to the incremental risk of an individual developing cancer was evaluated using the lifetime target carcinogenic risk (TCR) and computed by the excess lifetime cancer risk equation:
where the cancer slope factor (CSF) (Table 1) converts the estimated daily intake (EDI) of the PTE in the body over a lifetime of exposure directly to the incremental risk of an individual developing cancer [34]. If TCR ˃ 1 × 10−4, then it is considered unacceptable and intolerable [31].
Results and Discussion
Proximate Chemical Composition
The proximate chemical composition of F. sycomorus fruits showed high levels of moisture (55.8 ± 0.3%) and carbohydrates (25.3 ± 1.1%) yet lower than that of Ficus sur fruits with 88.8% moisture and 65.6% carbohydrates [19]. The fruits of F. sycomorus also contained 5.6 ± 0.2% protein, 8.9 ± 0.5% fats, 55.8 ± 0.9% crude fiber, and 4.4 ± 0.4% ash. The values for protein and fat were similar to those previously reported [35]. The fruits of F. sur contained lower crude fiber (7.7%) compared to F. sycomorus [19]. Based on the intake level observed to protect against coronary heart disease, the American Heart Association (AHA) set the Adequate Intake (AI) for crude fiber in foods at 38 and 25 g per day for young men and women, respectively [36]. Dietary fiber may play a role in modulating the immune system, which may lead to decreased risk of cardiovascular disease, diabetes, cancer, and obesity [37,38,39]. The proximate chemical data showed that the fruits of F. sycomorus might contribute significantly towards the AI for crude fiber. Similarly, the moisture content of the fresh fruit of F. burtt-davyi was 23.7 ± 0.20%. Based on dry mass, the protein content was 5.0 ± 0.30%, 8.4 ± 0.40% for lipids, 78.9 ± 0.55% for carbohydrates, 4.0 ± 0.70% for crude fiber, and 3.7 ± 0.10% for ash. The results show the fruits of F. burtt-davyi to be high in energy and low in fats.
Elemental Concentration
Method Validation
The precision of the analytical procedure was authenticated by concurrent analysis of CRMs. The experimental mean values compared well to certified values (P ˂ 0.05) with recovery percentages being within acceptable limits (Table 2).
For the macro-elements, concentrations in the fruit of F. sycomorus ranged from 4447 ± 777 to 6963 ± 227 mg kg−1 for Ca and from 1766 ± 42.43 to 2676 ± 141 mg kg−1 for Mg. The bioaccumulation factors (BAFs) for the exchangeable form of macro-elements ranged from 6.7 to 49 and 3.5 to 23 for Ca and Mg, respectively, indicating the tendency of the plant to accumulate these metals.
Total soil Pb ranged from 4.8 ± 0.01 to 44.0 ± 0.22 mg kg−1 across the study sites. This elevated concentration is above the South African maximum permissible level of 6.6 mg kg−1 set for agricultural soil [40]. Previously, similar results for total soil Pb were also obtained, which may be due to vehicular emissions [19]. At all study sites, the concentrations of Pb in the fruit samples were found to be below the instrument detection limits.
Total soil Cr ranged from 31.8 ± 2.25 to 110 ± 25.27 mg kg−1 and < 1.9% was in mobile form. The concentration in the fruits was within a small range of variation (0.04 ± 0.03 to 0.39 ± 0.01 mg kg−1) suggesting the plant controls Cr uptake. This is in agreement with other reports [41, 42]. The available Cr ranged from 0.39 ± 0.12 to 0.76 ± 0.11 mg kg−1, which is less than the phytotoxicity range of 1–5 mg kg−1 for available Cr in soil [41]. Chromium is an essential element to humans, and its deficiency includes impaired glucose tolerance, elevation in serum insulin, glycosuria, impaired growth, and altered immune function. Although total soil As, Cd, Co, and Ni ranged (in mg kg−1) from 7.4 ± 0.01 to 8.9 ± 0.3, 0.9 ± 0.05 to 6.7 ± 0.72, 1.8 ± 0.10 to 27.6 ± 0.50, and 4.5 ± 0.05 to 12.1 ± 1.11, respectively, concentrations in the fruits were found to be below the instrument detection limits. This showed that the fruits of F. sycomorus do not accumulate these toxic metals.
The elemental concentrations in soil (total and exchangeable) and fruits for the other microelements in F. sycomorus are summarized in Table 3. Total soil Cu ranged from 5.4–44.0 mg kg−1 which exceeded the South African maximum permissible level of 6.6 mg kg−1 for agricultural soil at most sites [40]. Except for sites 1, 4, and 5, Cu concentration in the fruits exceeded the WHO permissible limit of 10 mg kg−1 for plants [43], although the fruits did not tend to bioaccumulate Cu (BAF < 1 .0 for most sites) (Table 3). Copper is essential for humans, and it is necessary for the formation of hemoglobin and red blood cells [44]. Elevated levels in the food chain can result in diarrhea, vomiting, liver damage, fatigue, and depression. In this study, the concentrations of elements in the fruits were found to be in decreasing order of Ca > Mg > Fe > Zn > Mn > Cu > Cr, and the toxic metals (As, Cd, Co, and Pb) were below the instrument detection limits.
For the macro-elements, Ca in soil (in mg kg−1, total and exchangeable) ranged from 496 to 8405 and 245 to 4399, respectively, with highest the concentration (14,530 mg kg−1) obtained for the fruit from site 8, where 490 mg kg−1 was in exchangeable form (BAF = 29.2), and lowest concentration (7921 mg kg−1) obtained for fruit from site 3. Calcium in fruits may delay ripening and can contribute about 2% towards the total body weight of many fruits [45]. There was an accumulation of Mg in fruits at all sites with Mg in soil (total and exchangeable) and fruits (in mg kg−1) ranging from 214 to 1035, 199 to 340, and 2461 to 3728, respectively. For Ca and Mg, soil concentrations were lower than fruit concentrations, indicating the plants ability to bio-concentrate and bioaccumulate these metals to meet physiological requirement levels.
The concentrations of microelements in soil (total and exchangeable) and fruits of F. burtt-davyi are presented in Table 4. Elemental concentrations that were below the instrument detection limit were omitted from the table. The maximum concentration of Fe in the fruit (103 mg kg−1) was observed at site 8. Iron is known to play very essential role in many metabolic and synthetic pathways such as DNA synthesis, oxygen transport and storage, mitochondrial respiration, and citric acid cycle in the human body [46]. The BAFs (exchangeable) for Fe were relatively low (˂ 0.3 at all sites), suggesting that Fe uptake is controlled. This observation is similar to our previous report on the fruits of F. sur [19]. Overall, about 5.3% of total soil Fe was in exchangeable form. About 77.4% of total soil Mn was in exchangeable form, but the BAFs (exchangeable) ranged from 0.1 to 1.2 (site 8). This may be due to the lowering of the rate of Mn uptake due to the presence of Mg [47, 48]. The plant tends to regulate the uptake of Mn, based on metabolic requirements. The concentration of Mn in fruits ranged from 9.51 to 54.8 mg kg−1, which are below the maximum limits of 2000 mg kg−1 [49]. Total soil Cu ranged from 4.60 to 25.7 mg kg−1, while exchangeable concentrations ranged from 4.14 to 13.0 mg kg−1 with an average of 82.7% in exchangeable form. The high exchangeable value for Cu may be due to the high stability of the Cu complex formed with EDTA [50]. Copper concentrations in the fruits were all above the WHO permissible limit of 10 mg kg−1 [43] for plants. Elevated concentrations of Cu are known to cause anemia (via Mn depletion, which leads to Fe deficiency anemia), liver and kidney damage, and stomach and intestinal irritation in humans [51].
Generally, Zn concentration in fruits (25.3 to 49.0 mg kg−1) was higher than total and exchangeable soil concentrations. Although, about 56.9% of Zn was available for uptake by the fruit; the BAF was less than 1 for the studied sites. The concentration of Zn in the fruits at sites 1 (49.0 mg kg−1) and 5 (42.3 mg kg−1) were above the maximum levels for plants set by the Department of Health, South Africa, which is 40 mg kg−1 [52]. Data from this study showed that the elemental concentration in the fruits of F. burtt-davyi was the highest for Ca, followed by Mg, Fe, Mn, Zn, and Cu. In addition, the concentrations of trace essential elements (Co, Cr, Ni, and Se) and toxic metals (As, Cd, and Pb) were found to be below the instrument’s detection limits.
Estimated Contribution of Elements in Fruits to the Diet
Fruits are vital to the human diet as they contain micronutrients, which can contribute beneficially to recommended dietary allowances (RDAs) and may help meet the nutritional needs of impoverished rural communities where nutritionally deficient diseases are common. In this study, the elemental concentrations in the edible fruits were compared to the dietary reference intakes (DRIs) for most individuals (Table 5) to determine the contribution of the consumption of 20.0 g each of F. sycomorus and F. burtt-davyi fruits to the diet.
Consumption of 20.0 g of F. sycomorus fruits may contribute between 9.4–12.2% and 13.1–13.5% towards the RDA for Ca and Mg, respectively, and may also contribute about 4.5–10.1% towards the RDA for Fe and > 22.0% towards the RDAs for Cu and Mn. The fruits of F. sycomorus were richer in the nutrients Ca, Mg, Mn, and Zn compared to fruits of F. sur (11%, 9%, 4%, and 3%, respectively, towards the RDA) [19]. An intake of 0.024–0.035 mg of Cr per day is recommended, and consumption of approximately 20.0 g of F. sycomorus fruits may contribute approximately 0.0043 mg (12.3–19.9%) towards its RDA. Chromium is known to improve the efficiency of insulin, and it is needed in the metabolism of proteins, fats, and carbohydrates [54].
Similarly, 20.0 g of fruit of F. burtt-davyi may contribute up to 43.8% towards the RDA for Mn, thereby making the fruit a good source of Mn. Some Mn-rich, plant-based foods include pineapple (raw and juice), spinach, peanuts, sweet potatoes, brown rice, and pecan nuts [55]. Manganese is a component of the powerful antioxidant enzyme, manganese superoxide dismutase (MnSOD), which neutralizes free radicals in the human body [56,57,58]. A diet rich in Mn may prevent cancer and other diseases such as arthritis, osteoporosis, diabetes, and epilepsy [59, 60].
Principal Component Analysis (PCA)
Principal component analysis (PCA) was applied to assist in the identification of elemental sources in soil, and the results for the two species are presented in Table 6. The preliminary results of the KMO test (0.8) further validated the results of the PCA. For the F. sycomorus soil samples, three components were obtained, accounting for 86.3% of the total variance. Component 1 was dominated by Cd (0.98), Co (0.95), Cr (0.95), Fe (0.97), Mn (0.96), Ni (0.90), and Pb (0.81) accounting for 56.6% of the total variance. The high loadings of these metals suggest a common anthropogenic input. Previously, we reported similar patterns in soils from KwaZulu-Natal [19, 61]. Component 2, dominated by Ca and Mg, accounted for 20.7% of the total variance indicating that the elements originated from soil mineral forming processes. Component 3 was dominated by As, Cu, and Zn accounting for 9.0% of the total variance. A 3-D plot of the PCA loadings is presented in Fig. 2A, and the relationships among the 12 metals are clearly seen.
The growth soil from F. burtt-davyi was dominated by four principal components, which were extracted based on eigenvalues ˃ 1 and explained over 80% of the total variability, which described the overall elemental pattern, signifying different sources (Table 6). The first component explained 32.9% of the total variance; high loadings were obtained for Cd, Co, Cr, and Fe (Table 6) which could suggest common anthropogenic sources (vehicular emissions). Elevated levels of these trace metals have been reported in areas with high traffic density in South Africa [62]. The second principal component was strongly represented by Ca, Cu, Mg, and Zn, contributing 30.8% of the total variance. A quasi-independent behavior was also observed within the group due to Se having a loading of 0.55, which was further corroborated by a large distance in the 3-D PCA loading plot (Fig. 2B), which may suggest different sources. The third principal component contributed 14.4% to the total variance and had high loading of As and Ni, suggesting a common origin, while the fourth principal component was dominated by Mn (0.90), accounting for 9.3% of the total variance, suggesting that it came from a different source. A similar occurrence was reported previously [61].
Human Health Risk Assessment: Target Hazard Quotient (THQ)
Table 7 summarizes the non-carcinogenic risk, expressed as target hazard quotient (THQ) for Cr, Cu, Mn, and Zn for children. The ranges of THQ values for the fruits of F. sycomorus were 00.001 to 0.011 for Cr, 0.014 to 0.067 for Cu, 0.002 to 0.41 for Mn, and 0.005 to 0.066 for Zn. Similarly, the ranges of THQ estimates for the fruits of F. burtt-davyi were 0 to 1.0 × 10−5 for Cr, 0.012 to 0.093 for Cu, 0.006 to 0.059 for Mn, and 0.007 to 0.054 for Zn (Table 7). Elemental THQ values in the fruits of F. sycomorus were in the decreasing order of Cu ˃ Zn ˃ Mn ˃ Cr, and for fruits of F. burtt-davyi, it was in decreasing order of Cu ˃ Mn ˃ Zn ˃ Cr.
THQ values for heavy metals in the fruits for all sites were less than 1, indicating an acceptable non-carcinogenic risk to human health which confirms that consumption of the fruits by rural communities is safe. This result is consistent with the mean elemental concentrations as well as the multivariate results from this study. These results showed that consumption of the fruits of F. sycomorus and F. burtt-davyi by children is safe and will not lead to non-carcinogenic exposure or adverse health effects, which would make it safe for the rest of the population.
Furthermore, the elemental additive effect for non-carcinogenic risk was also used to predict the possible effects on human health. The elemental hazard indices (HI) of the studied heavy metals in fruits were less than 1, indicating no additive adverse non-carcinogenic health risk to humans through the consumption of metals in the fruits of both species.
The average lifetime target carcinogenic risk (TCR) for Cr through the consumption of fruits of the two species from the study area ranged from 7.9 × 10−8 to 1.7 × 10−5 which were within the USEPA recommended safe limit for cancer risk (1.0 × 10−4) [63, 64] confirming no carcinogenic risk to the exposed population.
Conclusion
Many rural communities, especially in South Africa, often consume the fruits from Ficus species. This study provides information on the nutritive value of the fruits of F. sycomorus and F. burtt-davyi. The results indicate that the fruits are good for health and do not tend to accumulate toxic elements (As, Cd, and Pb); therefore, vulnerable communities would not be exposed to non-carcinogenic and carcinogenic adverse health risks through their consumption. The fruits were also found to be rich in Mn, which may be beneficial in maintaining a healthy immune system if consumed by people living in impoverished communities. The results showed site to influence elemental distribution; however, statistical analyses showed uptake of elements to be controlled by the plant to meet physiological needs as evidenced by bioaccumulation factors.
References
Van Wyk B, Van Wyk P, Van Wyk B-E (2000) Photographic guide to trees of South Africa 1st edn. Briza Publications, Pretoria
Itanna F (2002) Metals in leafy vegetables grown in Addis Ababa and toxicological implications. Ethiop J Health Dev 16:295–302
Block G (1992) A role for antioxidants in reducing cancer risk. Nutr Rev 50:207–213
Block G, Patterson B, Subar A (1992) Fruit, vegetables, and cancer prevention: a review of the epidemiological evidence. Nutr Cancer 18:1–29
Ford ES, Mokdid AH (2001) Fruit and vegetable consumption and diabetes mellitus incidence among U.S adults. Prev Med 3:33–39
Rimm EB, Ascherio A, Giovanucci E, Speigelman D, Stampfer MJ, Willett WC (1996) Vegetable, fruit and cereal fibre intake and risk of coronary heart diseases among men. J Am Med Ass 275:447–451
D’Mello JPF (2003) Food safety: contaminants and toxins. 480. CABI Publishing, Wallingford
Gebrekidan A, Weldegebriel Y, Hadera A, Bruggen BV (2013) Toxicological assessment of heavy metals accumulated in vegetables and fruits grown in Ginfel River near Sheba tannery, Tigray, northern Ethiopia. Ecotoxicol Environ Saf 95:171–178
Nabulo G, Oryem-Origab H, Diamond M (2010) Assessment of lead, cadmium, and zinc contamination of roadside soils, surface films, and vegetables in Kampala City, Uganda. Environ Res 101:42–52
Nabulo G, Young SD, Black CR (2010) Assessing risk to human health from tropical leafy vegetables grown on contaminated urban soils. Sci Total Environ 408:5338–5351
Wang Q-R, Cui Y-S, Liu X-M, Dong Y-T, Christie P (2003) Soil contamination and plant uptake of heavy metals at polluted sites in China. J Environ Sci Health A 38:823–838
World Health Organization (1992) Cadmium. Environmental Health Criteria. Vol. 134, Geneva
Sharma KR, Agrawal M, Marshall MF (2009) Heavy metals in vegetables collected from production and market sites of a tropical urban area of India. Food Chem Toxicol 47:583–591
Nabulo G, Black CR, Young SD (2011) Trace metal uptake by tropical vegetables grown on soil amended with urban sewage sludge. Environ Pollut 159:368–376
Sathawara NG, Parikh DJ, Agarwal YK (2004) Essential heavy metals in environmental samples from Western India. Bull Environ Contam Toxicol 73:756–761
Singh A, Sharma RK, Agrawal M, Marshall FM (2010) Risk assessment of heavy metal toxicity through contaminated vegetables from waste water irrigated area of Varanasi, India. Trop Ecol 51:375–387
Liang J, Wang QQ, Huang BL (2004) Concentration of hazardous heavy metals in environmental samples collected in Xiaman, China as determined by vapor generation non-dispersive atomic fluorescence spectrometry. Anal Sci 20:85–88
Arceusz A, Radecka I, Wesolowski M (2010) Identification of diversity in elements content in medicinal plants belonging to different plant families. Food Chem 120:52–58
Ogunlaja OO, Moodley R, Baijnath H, Jonnalagadda SB (2017) Nutritional evaluation, bioaccumulation and toxicological assessment of heavy metals in edible fruits of Ficus sur (Moraceae). J Environ Sci Health B 52:84–91
Arnold HJ, Gulumian M (1984) Pharmacopoeia of traditional medicine in Venda. J Ethnopharmacol 12:35–74
Burrows JE, Burrows SM (2003) Figs of southern and south-Central Africa. Umdaus Press Hatfield, South Africa
Chapman HD (1965) Cation exchange capacity. In: Black CA (ed) Methods of soil analysis part 2 – chemical and microbiological properties. American Society of Agronomy, Madison, pp 891–901
Walkley A, Black IA (1934) An examination of the Degjareff method for determining SOM and a proposed modification of the chromic acid titration method. Soil Sci 37:29–38
Association of Official Analytical Chemists (2000) Official methods of analysis, 17th edn. Association of Official Analytical Chemists; AOAC, Gaithersburg
Dı’az RV, Aldape J, Flores M (2002) Identification of airborne particulate sources, of samples collected in Ticoma ´n, Mexico, using PIXE and multivariate analysis. Nucl Instrum Methods Phys Res B 189:249–253
Lee J-S, Chon H-T, Kim K-W (2005) Human risk assessment of as, cd, cu and Zn in the abandoned metal mine site. Environ Geochem Health 27:185–191
Lim HS, Lee JS, Chon HT, Sager M (2008) Heavy metal contamination and health risk assessment in the vicinity of the abandoned Songcheon au–Ag mine in Korea. J Geochem Explor 96:223–230
Islam MS, Ahmed MK, Habibullah-Al-Mamun M, Raknuzzaman M (2015) The concentration, source and potential human health risk of heavy metals in the commonly consumed foods in Bangladesh. Ecotoxicol Environ Saf 122:462–469
HIES (Household Income and Expenditure Survey) (2011) Preliminary report on household income and expenditure survey-2010. Bangladesh Bureau of Statistics, Statistics Division, Ministry of Planning, Dhaka
USEPA IRIS (US Environmental Protection Agency's Integrated Risk Information System) (2011) http://www.epa.gov/iris/, Accessed date: 27 August 2019
USEPA (2015) Risk based screening table. Composite table: summary tab 0615. Accessible at http://www2.epa.gov/risk/riskbasedscreeningtablegenerictables. Accessed 25 Sept 2018
Islam MS, Ahmed MK, Habibullah-Al-Mamun M, Masunaga S (2014) Trace metals in soil and vegetables and associated health risk assessment. Environ Monit Assess 186:8727–8739
Department of Environmental Affairs (2010) The framework for the Management of Contaminated Land, South Africa. Available online: http://sawic.environment.gov.za/documents/562.pdf. Accessed 28 Aug 2019
U.S. Environmental Protection Agency (1989) Risk assessment guidance for superfund volume 1: human health evaluation manual (part a). Washington, Office of Emergency and Remedial Response
Wilson AL, Downs CT (2012) Fruit nutritional composition and non-nutritive traits of indigenous south African tree species. S Afr J Bot 78:30–36
American Heart Association (AHA) (1983) AHA committee report. Diet in the healthy child Task force committee of the nutrition committee and cardiovascular disease in the young council of American Heart Association. Circulation 67:1411–1414
Streppel MT, Ocké MC, Boshuizen HC, Kok FJ, Kromhout D (2008) Dietary fiber intake in relation to coronary heart disease and all-cause mortality over 40 y: the Zutphen study. Am J Clin Nutr 88:1119–11125
Chuang SC, Norat T, Murphy N, Olsen A, Tjønneland A, Overvad K, Boutron-Ruault MC, Perquier F, Dartois L, Kaaks L (2012) Fiber intake and total and cause-specific mortality in the European prospective investigation into Cancer and nutrition cohort. Am J Clin Nutr 96:164–174
Cho SS, Qi L, Fahey GC Jr, Klurfeld DM (2013) Consumption of cereal fiber, mixtures of whole grains and bran, and whole grains and risk reduction in type 2 diabetes, obesity, and cardiovascular disease. Am J Clin Nutr 113:1–26. https://doi.org/10.3945/ajcn.067629
Water Research Commission (1997) Permissible utilisation and disposal of sewage sludge. . 1st edition TT85–97. Water Research Commission, Pretoria
Adrino DC (2001) Trace elements in terrestrial environments: biochemistry, bioavailable and risk of metals, 2nd edn. Springer-Veralag, New York
Golovatyj SE, Bogatyreva EN (1999) Effect of levels of chromium content in a soil on its distribution in organs of corn plants. In: Bodevich IM, Lapa VV, Levitan TV (eds) Soil research and use of Fertilisers. BRISSA, Minsk
World Health Organization (2005) Quality control methods for medicinal plant materials. WHO, Geneva
Davis GK, Mertz W (1987) Copper. In: Mertz W (ed) Trace elements in human and animal nutrition. Academic Press, Orlando
Valvi SR, Rathod VS (2011) Mineral composition of some wild edible fruits from Kolhapur District. Int J Appl Biol Pharm Technol 2:392–396
Borzoei M, Zanjanchi MA, Sadeghi-aliabadi H, Saghaie L (2019) Trace determination of Iron in real waters and fruit juice samples using rapid method: optimized dispersive liquid-liquid microextraction with synthesized nontoxic chelating agent. Biol Trace Elem Res 192:319–329
Kies C (1994) Bioavailability of manganese. In: Klimis-Tavantzis DL (ed) Manganese in health and disease. CRC Press, Inc, Boca Raton
Maas EV, Moore DP, Mason BJ (1969) Influence of calcium and magnesium on manganese absorption. Plant Physiol 44:796–800
Kabata-Pendias A, Pendias H (1989) Trace elements in the soil and plants. CRC Press, Boca Raton
Madrid F, Biasioli M, Ajmone-Marsan F (2008) Availability and bioaccessibility of metals in fine particles of some urban soil. Arch Environ Contam Toxicol 55:21–32
Raymond AW, Felix EO (2011) Heavy metals in contaminated soils: a review of sources, chemistry, risks and best available strategies for remediation. ISRN Ecol 1–20
Department of Health (2004) Regulations relating to maximum levels for metals in foodstuffs: Amendment to Foodstuffs, Cosmetics and Disinfectants Act 54 of 1972. Government Gazette 26279. South Africa
Food and Nutrition Board (2011) Dietary reference intakes: tolerable upper intake levels and recommended dietary allowance of elements. Institute of Medicine. National Academies
Chowdhury S, Pandit K, Roychowdury P, Bhattacharya B (2003) Role of chromium in human metabolism, with special reference to type 2 diabetes. J Assoc Physicians India 51:701–705
US Department of Agriculture, Agricultural Research Service (2009) USDA National Nutrient Database for Standard Reference, Release 22. Available at: http://ndb.nal.usda.gov//. Accessed 6/15/2017
Ademuyiwa O, Odusoga OL, Adebawo OO, Ugbaja RN (2007) Endogenous antioxidant defenses in plasma and erythrocytes of pregnant women during different trimesters of pregnancy. Acta Obstet Gynecol Scand 86:1175–1180
Mistry HD, Williams PJ (2011) The importance of antioxidant micronutrients in pregnancy. Oxid Med Cell Longev 1–12. https://doi.org/10.1155/2011/841749
Paynter DJ (1980) Changes in activity of the manganese superoxide dismutase enzyme in tissues of the rat with changes in dietary manganese. J Nutr 110:437–447
Ekmekcioglu C, Prohaska C, Pomazal K, Steffan I, Schernthaner G, Marktl W (2001) Concentrations of seven trace elements in different hematological matrices in patients with type 2 diabetes as compared to healthy controls. Biol Trace Elem Res 79:205–219
Osterode W, Holler C, Ulberth F (1996) Nutritional antioxidants, red cell membrane fluidity and blood viscosity in type 1 (insulin dependent) diabetes mellitus. Diabetic Med 13:1044–1050
Mahlangeni NT, Moodley R, Jonnalagadda SB (2016) The distribution of macronutrients, anti-nutrients and essential elements in nettles, Laportea peduncularis susp. Peduncularis (river nettle) and Urtica dioica (stinging nettle). J Environ Sci Health B 51:160–169
Olowoyo JO, Van Heerden E, Fischer JL (2012) Concentrations of trace metals from soils in Pretoria, South Africa. J Ecosyst Ecograph 2:1–5. https://doi.org/10.4172/2157-7625.1000113
United States Environmental Protection Agency (USEPA) (2011) Screening level (RSL) for chemical contaminant at superfund sites. U.S. Environmental Protection Agency, Washington, DC
USEPA (2012) Edition of the Drinking Water Standards and Health Advisories. EPA 822-S-12-001, 2012 Edition of the drinking water standards and health advisories, Office of Water. U.S. Environmental Protection Agency, Washington, DC
Acknowledgments
The authors would like to acknowledge the School of Chemistry and Physics, University of KwaZulu-Natal, for the research facilities.
Author information
Authors and Affiliations
Corresponding author
Ethics declarations
Conflict of Interest
The authors declare no conflict of interest.
Additional information
Publisher’s Note
Springer Nature remains neutral with regard to jurisdictional claims in published maps and institutional affiliations.
Rights and permissions
About this article
Cite this article
Ogunlaja, O.O., Moodley, R., Baijnath, H. et al. Elemental Distribution and Health Risk Assessment of the Edible Fruits of Two Ficus Species, Ficus sycomorus L. and Ficus burtt-davyi Hutch. Biol Trace Elem Res 198, 303–314 (2020). https://doi.org/10.1007/s12011-020-02048-4
Received:
Accepted:
Published:
Issue Date:
DOI: https://doi.org/10.1007/s12011-020-02048-4