Abstract
In the current work, we have reported the preparation of Fe3O4/ZnO nanocomposite (NC) via co-precipitation. The prepared Fe3O4/ZnO nanocomposite were characterized by x-ray diffraction, Fourier transform infrared (FT-IR) spectroscopy, scanning electron microscopy (SEM) equipped with energy dispersive x-ray analysis (EDAX), transmission electron microscopy (TEM), Brunauer–Emmett–Teller (BET) surface area analysis, ultraviolet-diffuse reflectance spectroscopy (UV-DRS) and photoluminescence spectroscopy (PL). From the x-ray diffraction pattern, the average crystallite size of Fe3O4/ZnO NC was found to be 13 nm. UV-DRS showed an energy band gap of 2.3 eV for the prepared sample. From the Brunauer–Emmett–Teller (BET) surface area analysis, total pore volume (VP) and mean pore diameter (rp) were found to be 12.647 m2g−1, 0.024125 cm3g−1 and 7.6302 nm. The functional groups and metal to oxygen bonding in Fe3O4/ZnO NC were observed in FT-IR. SEM and TEM micrograph of Fe3O4/ZnO NC show flake- and grain-like structures, and the luminescence spectrum shows blue emission. The photocatalytic activity of Fe3O4/ZnO NC was examined using industrial effluent dyes such as Evans blue (90% in 150 min) and Rhodamine B (RB) (99% in 150 min) under UV light irradiation. Further, Fe3O4/ZnO NC also showed a superior zone of inhibition against pathogenic bacterial strains such as Escherichia coli and Staphylococcus aureus by agar well diffusion compared to Fe3O4 and ZnO nanoparticles.
Similar content being viewed by others
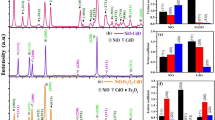
Avoid common mistakes on your manuscript.
Introduction
In recent years, the development of industries has increased pollution. Methylene blue, Evans blue, remazol red, malachite green, methyl orange etc., are colored dyes used in textile industries which leads to contamination of environmental resources (global warming, ozone layer depletion, etc.), and it also harms aquatic life and humans1 (skin diseases, lung infections, etc.). Photocatalytic degradation is one of the most effective strategies to reduce the contamination from industrial waste.
Metal oxides such as TiO2, ZnO, ZrO2, SnO2 and Fe3O4 are largely used in the degradation process.2 Among them, semiconductor ZnO is preferred as the photocatalyst due to its high photocatalytic activity, wide band gap, high quantum efficiency, and low cost.3 Magnetic core-shell nanoparticles have been used to improve the photocatalytic degradation property. Among several, Fe3O4 has important magnetic properties, low cost, chemical stability, mechanical hardness, easy separation, is non-toxic, and in the octahedral site there is a shift of electrons between Fe2+ and Fe3+ oxidation states. There are many physical and chemical methods, such as hydrothermal, co-precipitation, and sol-gel, for the synthesis of magnetic nanoparticles but co-precipitation is the most well-known synthesis route to achieve good morphology and enhanced properties.4
Several NPs inhibit the growth of bacteria by representing strong antibacterial properties which act against Staphylococcus aureus and Escherichia coli bacteria.5 Nowadays, metal oxide NC has shown fascinating properties and enormous applications within the fields of biology, medicine, sensors and the environment. Jilla et al. prepared an agglomerated Fe3O4/ZnO nanocomposite by free surface sonication and its application in photocatalytic degradation of dye.6 The visible light photocatalytic application of Fe3O4@LPH@Ag/Ag3PO4 sub microspheres was synthesized by Chao et al., where Fe3O4@LDH was prepared by a hydrothermal method and Fe3O4@LDH@PO43− by ion exchange.7 Sobia et al. prepared a microsphere Fe3O4@MIL-100(Fe) core-shell for photocatalytic degradation by a hydrothermal method.8 Jian et al. synthesized Fe3O4@ZnO nanospheres by a chemical method for photocatalytic and antibacterial activity.9 Agglomerated Fe3O4@MOR@CuO was prepared by Rajablet al., who used a hydrothermal method for a visible light driven photocatalyst.10 An Fe3O4@ZnO core-shell was prepared by Jian Wang et al. by co-precipitation for the photocatalytic property.9
In this current work, Fe3O4/ZnO nanocomposites have been synthesized by a simple co-precipitation method and its structural, optical, morphological, photocatalytic and antibacterial properties are studied.
Experimental
Preparation of Fe3O4/ZnO Nanocomposite
Analytical grade reagents were purchased from Fluka, Sigma-Aldrich and Acros and used as supplied. The preparation of Fe3O4/ZnO NC involves two steps.
Step I: Preparation of Fe3O4
FeCl3.6H2O (9.4 g) and FeCl2.4H2O (3.46 g) were dissolved in 100 mL of double distilled water in a round bottom flask at 70–80°C for 30 min. Then the solution was heated in a pre-heated oil bath at 85°C by stirring until the solution turned yellow. After 30 min, 20 mL NH4OH was added dropwise into the solution. The reaction mixture was then left in the heated oil bath for 30 min. Once the reaction was completed, the reaction mixture was removed from the oil bath and cooled. Then the prepared magnetic nanoparticles were washed with double distilled water until neutral pH was reached from basic medium and further washed with methanol (30 mL). The obtained black powder of Fe3O4 NPs was vacuum dried and stored.
Step II: Preparation of Fe3O4/ZnO Nanocomposite
The Fe3O4/ZnO NC was attained by later deposition of ZnO on the surface of iron nanoparticles through thermal decomposition. Fifty milliliters of ethyl alcohol was added to the mixture of 0.49 g of Fe3O4 NPs and 3.93 g of Zn(CH3COO)2.4H2O. The mixture was stirred at 70°C for 1 h. Dropwise addition of 1.58 mL of diethanol amine and 5 mL of ethyl alcohol was done under stirring until a dark brown colour was obtained. Stirring was continued for 2 h until the solution turned to a gel. Fe3O4/ZnO NC was then collected by simple magnetic decantation and ultimately washed with water (40 mL) and methanol (40 mL×4) then dried under vacuum at 90°C for 24 h (Scheme 1).11
Characterization
Powder x-ray diffraction (XRD) of prepared Fe3O4/ZnO NC was used to observe the crystalline phases using a Rigaku Ultima-IV x-ray diffractometer using Cu-Kα radiation (λ = 1.5406 Å). FTIR spectra were recorded by a Bruker spectrophotometer using KBr pellets. TLC silica gel 60 F254 (Merck) detected UV light (254 nm) and iodine vapors. Scanning electron microscope (SEM) analysis was done using a JEOL-JSM 7100F, and transmission electron microscopy (TEM) was done by a JEOL-JEM 2100. The surface area and pore size distribution was studied by Brunauer–Emmett–Teller (BET) using a Belsorp Max instrument (Japan). Photoluminescence studies (PL) were carried out using an Agilent Technologies Cary-60 Eclipse spectrophotometer.
Photocatalytic Setup
The photoreactor assembly includes a cylindrical tube, the inside of which is made transparent to irradiate the light. A tungsten vapour lamp (300 W) of medium pressure was placed at the centre of the system and cooling jacket was used for refrigeration. Fans were afixed to the four corners of the photoreactor in order to cool the reactor. As the lamp produces heat, continuous circulation of cold water through the cooling jacket is maintained. A set of quartz tubes of 100 mL capacity were placed around the lamp and filled with organic pollutants. Using an air pump, bubbling of the solution was maintained in order to distribute the catalyst uniformly.
Photocatalytic Activity
The photocatalytic property of the Fe3O4/ZnO NC was examined by the photodegradation of Evans blue (EB) and Rhodamine B (RB) dye aqueous solution under UV light illumination. Fifty milligrams of the catalyst (Fe3O4, ZnO, Fe3O4/ZnO NC) was added to different glass tubes containing 5 ppm of two different dyes (three tubes for each dye). After mixing, the solution was kept in the dark and air bubbled for 30 min to obtain the adsorption/desorption equilibrium between the photocatalyst and dye before illumination. After attaining equilibrium, UV light was focused on the solution with the circulation water. At 30-min intervals, 4 mL of the solution was taken out and used to test the absorbance. An Agilent Technologies Cary-60 UV-Visible spectrophotometer was used.
The percentage degradation of the dye was calculated using the following relation.
where η is the percentage of degradation, the initial concentration of the dye is given by c0 and the concentration of the dye after irradiation is given by ct.12
Evaluation of Antibacterial Activity
ZnO, Fe3O4 and Fe3O4/ZnO NC were examined for antibacterial activity by Agar well diffusion13,14 against gram negative bacteria—Escherichia coli [NCIM-5051] and gram positive bacterial strains Staphylococcus aureus [NCIM-5022] were sub-cultured in sterile nutrient agar medium and poured into sterile petri dishes for solidifying. Using a sterilized L-shaped glass rod, matured broth culture of individual pathogenic bacterial strains was spread uniformly over the surface of agar plates using a sterile cork borer. In each individual agar plate about 6-mm wells were made. Different concentrations of ZnO, Fe3O4, Fe3O4/ZnO NC (150, 300 and 450 µg/well) were dispersed in sterile distilled water and dispersed into the well using micropipettes. For positive control, a standard antibiotic (ciprofloxacin) was used. The zone of inhibition of each well was measured once the plates were incubated at 37°C for 24 h.
Result and Discussion
XRD Studies
The XRD patterns of ZnO, Fe3O4, and Fe3O4/ZnO NC are shown in Fig. 1a, b, and c. Six characteristic peaks for Fe3O4 NPs (2θ = 30.16°, 35.48°, 43.13°, 53.49°, 56.91° and 62.71°) are marked by their indices (220), (311), (400), (422), (511) and (440), respectively. These peaks are well indexed to the JCPDS card no. 01-076-0704. The eight peaks for ZnO NPs (2θ = 31.84°, 34.52°, 36.33°, 47.63°, 56.71°, 62.96°, 68.13°, 69.18°) are marked by their indices (100), (002), (101), (102), (110), (103), (112) and (201) with JCPDS card no. (01-088-0315). The XRD pattern of Fe3O4/ZnO nanocomposite confirmed the presence of Fe3O4 NPs and ZnO NPs combined together which formed a composite. The marking of peaks with * (ZnO) and # (Fe3O4) can be observed in the Fe3O4/ZnO NC without any additional impurities. Hence, no extra peaks were absorbed which confirmed that Fe3O4 nanoparticles were coated with ZnO.9,14
FTIR Studies
The prepared Fe3O4/ZnO NC were characterized by FTIR and are shown in Fig. 2. The strong absorption band at 450 cm−1 is due to the different stretching modes of the Zn-O bonding in zinc oxide. The stretching vibrations of the Fe-O bonding fall near to each other at 563 cm−1. Furthermore, the peak at 1607 cm−1 shows the C-H bending vibration and the C-O stretching vibration attributed to 1102 cm−1 and 1409 cm−1. This confirms that all the characteristic peaks of Fe3O4 and ZnO are present in Fe3O4/ZnO NC. Because of the increased concentration of ZnO in the Fe3O4/ZnO NC system, a broad band is observed between 450 (v1) and 557 (v2) cm−1 which is attributed to the Zn-O vibrations. This increased concentration of ZnO makes resolution of the v1 and v2 peaks difficult. However, a peak is observed around 563 cm−1 which can be attributed to the vibrations between oxygen and iron. The strong absorption peak at 3425 cm−1 shows the O-H stretching vibration. The absorption peaks at 2914 cm−1 are attributed to the C-H stretching vibrations of the -CH2 functional group.15
Morphological Studies
Figure 3a and b shows the SEM images of Fe3O4 and ZnO. From analysis of SEM micrographs, almost uniform-sized particles with flake- and grain-like structure were observed and conjointly marked tendency to make giant clusters. Further, Fig. 3c and d indicates the composite structure of ZnO and Fe3O4 magnetic nanoparticles. Figure 4 shows the EDAX of Fe3O4/ZnO NC. From EDAX, iron, zinc and oxygen peaks (Pt is excluded as it from platinised carbon tape) clearly confirm the presence of Fe3O4 and ZnO in the prepared nanocomposite.16
HRTEM with SAED Analysis
Figure 5a, b, c, and d clearly represents the TEM images of Fe3O4/ZnO NC and the overall size and structure of the prepared nanocomposite. We can also identify the spherical and hexagonal nano-flakes forming the clusters by combining each other having low dispersion which tends to the magnetic property of the synthesized nanocomposite.17,18 During electron irradiation there is difference in electron scattering; hence, Fe3O4 appears darker because of higher electron density when compared to ZnO nanoparticles in Fig. 5c.19 HRTEM shown in Fig. 5e gives a d spacing value of 0.19 nm (Fe3O4) and 0.24 nm (ZnO) in the nanocomposite20 which supports the XRD studies. SAED pattern of core shell nanoparticles is represented in Fig. 5f with an (hkl) value of (101) ZnO and (220) Fe3O4 which corresponds to the JCPDS card number of the XRD.21,22
BET Studies
For determining the surface properties, the N2 adsorption-desorption isotherm was analysed and is shown in Fig. 6. Fe3O4/ZnO NC shows isotherm hysteresis type (IV). BET surface area, total pore volume and mean pore diameter were found to be 12.647 m2g−1, 0.024125 cm3g−1 and 7.6302 nm.23 Because of its high surface area, good pore volume and pore diameter, surface active sites available for the photocatalytic reactions also increases which slows down the recombination rate of electron hole pairs. In general, high surface area will enhance the catalytic reactions.
UV-Visible DRS Studies
Figure 7a shows the UV-Visible diffused reflectance spectra (DRS) of synthesized Fe3O4/ZnO NC. It shows an absorption point at around 464 nm. The Kubelka–Munk (K–M) theory was used to calculate the band gap of Fe3O4/ZnO NC, given by the following relation.
where R∞ is the reflection coefficient, λ is the absorption wavelength.
For synthesized Fe3O4/ZnO NC, the energy gap was found to be around 2.3 eV, as shown in Fig. 7b.24 Radon et al. found that the optical energy band gap of Fe3O4 nanoparticles synthesized by co-precipitation was 3.01 eV. Devi et al. synthesized ZnO nanoparticles by co-precipitation and obtained an energy band gap of 3.30 eV. When compared to these two values in the literature, Fe3O4/ZnO NC prepared by co-precipitation in the present work showed a narrow band gap. The obtained band gap of 2.3 eV confirmed that Fe3O4/ZnO nanocomposite is a visible active material.25,26
Photocatalytic Activity
Figure 8 shows the photocatalytic degradation of Fe3O4/ZnO NC against RB and EB as a targeted pollutant under UV light illumination. The decolonization of RB and EB dyes over ZnO, Fe3O4 and Fe3O4/ZnO NC was recorded by UV-Visible spectroscopy. Before irradiation, the solution mixture was kept in the dark to obtain adsorption/desorption equilibrium. Initially, there were no changes observed for either dye, but after irradiation, it shows that the dye undergoes degradation. The comparison of Fe3O4, ZnO and Fe3O4/ZnO NC using both RB and EB dyes was carried out. Fe3O4/ZnO NC shows 9% degradation at 30 min and 99% degradation at 150 min using RB as a dye, but in EB at 30 min it shows 43% degradation and at 150 min 90% degradation was observed. In RB dye Fe3O4/ZnO NC shows the maximum degradation but ZnO has degraded well compared to Fe3O4 which is shown in Fig. 8b. Comparison of degradation activity of Fe3O4, ZnO, Fe3O4/ZnO NC using EB dye is Fe3O4/ZnO NC > Fe3O4 > ZnO (Fig. 8d).9,21,23. The comparative study of degradation of dyes in the presence of ZnO, Fe3O4, Fe3O4/ZnO NC against RB is shown in Table I.
Mechanism of Photocatalytic Degradation
Photocatalytic degradation of RB and EB dye under UV light illumination using Fe3O4/ZnO NC is represented in the following reaction mechanism.
The schematic representation of the photodegradation of RB and EB using Fe3O4/ZnO NC under UV light is shown in Scheme 2. The photodegradation was initiated only when the Fe3O4/ZnO NC photocatalyst interacted with the UV light irradiation, the arrangement of e− (electrons) in conduction band CB and h+ (holes) in the valence band VB takes place during photoexcitation. To generate anionic superoxide radical (·O2−) by reduction, the photogenerated electron in the CB reacts with molecular oxygen. e− gets attracted to the photogenerated hole in VB from hydroxyl ions or water molecule to yield the most reactive hydroxyl radical (·OH) over oxidation. The H2O2 generated by reacting with superoxide anion radical with e−/h+ pairs. On the surface of Fe3O4/ZnO NC, the organic molecules are attacked by these reactive species which act as strong oxidizing agents. Hence, these organic dye molecules easily attacked by ions leads to byproducts. This process is called a redox reaction on the surface of the photocatalyst by the excitation of the catalyst.
Photoluminescence Spectra
To observe the recombination efficiency of generated hole and electron pairs, photoluminescence (PL) spectra was used and is shown in Fig. 9. As depicted in Fig. 9, the intensity of peaks is different which is due to the varying recombination behavior of charge carriers for different materials. PL of Fe3O4, Fe3O4/ZnO NC, and ZnO NPs were recorded at room temperature. The excitation peak shown at 238 nm was observed at emission peaks of 284 and 392 nm.9,17,27,28 Photocatalytic activity of a semiconductor photocatalyst is majorly depending on electron and hole. The effect of photocatalytic activity reduces when both the electron and hole recombine to emit a photon. Hence, the lower PL intensity shows the higher photocatalytic activity. The PL of Fe3O4/ZnO NC shows the lowest intensity peak when compared to Fe3O4 and ZnO. Therefore, photocatalytic performance of Fe3O4/ZnO NC is higher than pure Fe3O4 and ZnO. Figure 10 shows the X and Y colour coordinates and is known as the CIE (Commission International De I’Eclairage) diagram. From the above figure, the colour of the emitted light from the Fe3O4/ZnO NC can be observed and was found to be in the blue region.
Antibacterial Activity
Antibacterial activity of all the three materials was examined against pathogenic bacterial strains such as Escherichia coli and Staphylococcus aureus by agar well diffusion. The zone of inhibition of prepared NPs with varying concentration of ZnO, Fe3O4, Fe3O4/ZnO NC (150, 300 and 450 µg/well) with respect to the positive control (ciprofloxacin) was recorded. The results with respect to zone of inhibition were estimated with vernier calipers in millimeters, and its values are shown in Table II where we can observe that the bacterial activity of Fe3O4/ZnO is greater when compared to Fe3O4 and ZnO individually. This result also indicates that the bacterial activity is completely concentration dependent on the significant effect of nanoparticles against pathogenic bacterial strains. The Fe3O4/ZnO NC shows highly significant antibacterial activity against pathogenic strains such as Escherichia coli and Staphylococcus aureus strains as shown in the Fig. 11. The mechanism for the antibacterial activity of Fe3O4/ZnO NC is shown in Scheme 3.37,38 The ROS (reactive oxygen species) and release of heavy metal ions, i.e. iron and zinc, were remarkably effective, where these two metals are active sites releasing hydroxide and super oxide radicles which act on the bacteria. Hence, this is the major reason for effective role of Fe3O4/ZnO NC, whereas in Fe3O4 and ZnO NPs individually have a single metal and less reactive oxygen species when compared to the composite39,40 where the light irradiation leads to oxidative stress in the cell membrane of the bacteria which finally leads to the death of the bacterial cell. The bacterial death due to the smaller particle size provides larger surface for the interaction with the bacteria which results in effective antibacterial activity. The opposite charge of the prepared nanoparticles and the cell membrane mutually attracts and the Fe3O4/ZnO NC enters the bacterial cell membrane reacts with thr thiol group (-SH) present on the surface of the cell which finally leads to the death of the bacterial cell. The comparative study of antibacterial activity in the presence of ZnO, Fe3O4, Fe3O4/ZnO NC against pathogenic strains is shown in the Table III.
Conclusion
Fe3O4@ZnO core-shell NPs were successfully prepared by co-precipitation and were characterized by XRD, FTIR, SEM with EDAX, TEM, BET and UV-Visible spectroscopy. The XRD pattern confirms the formation of Fe3O4/ZnO NC by comparing it with Fe3O4 and ZnO patterns. Flake- and grain-like morphology was observed in SEM and TEM micrographs. The vibrational modes and M-O bonding confirm the formation of Fe3O4/ZnO NC. BET analysis shows the surface area of 12.6 m2g−1. The bang gap was observed at 2.3 eV from UV DRS which is visible active material. From the PL spectra; Fe3O4/ZnO NC is useful for optoelectronic applications. The prepared Fe3O4/ZnO NC shows significant photocatalytic activity for the degradation of Evans blue and Rhodamine B dyes. The antibacterial activity of Fe3O4/ZnO NC demonstrated effective bactericidal activity against gram positive and gram negative bacterial strains. These results show that the prepared Fe3O4/ZnO NC synthesis is a very simple, eco-friendly and cost-effective material. It offers potential applications in the field biomedical applications and Fe3O4/ZnO nanocomposite is visible active material where amount of visible rays in sun light is around 42% hence Fe3O4/ZnO nanocomposite shows possible application in waste water treatment.
Change history
20 April 2021
A Correction to this paper has been published: https://doi.org/10.1007/s11664-021-08931-7
References
R. Saravanan, S. Karthikeyan, V.K. Gupta, G. Sekaran, V. Narayanan, and A.J.M.S. Stephen, Mater. Sci. Eng., C 33, 91 (2013).
K. Karthik, S. Dhanuskodi, C. Gobinath, S. Prabukumar, and S. Sivaramakrishnan, J. Mater. Sci.: Mater. Electron. 29, 5459 (2018).
R.Y. Hong, J.H. Li, L.L. Chen, D.Q. Liu, H.Z. Li, Y. Zheng, and J. Ding, Powder Technol. 189, 426 (2009).
J. Xia, A. Wang, X. Liu, and Z. Su, Appl. Surf. Sci. 257, 9724 (2011).
Q.L. Feng, J. Wu, G.Q. Chen, F.Z. Cui, T.N. Kim, and J.O. Kim, J. Biomed. Mater. Res. 52, 662 (2000).
J. Saffari, N. Mir, D. Ghanbari, K. Khandan-Barani, A. Hassanabadi, and M.R. Hosseini-Tabatabaei, J. Mater. Sci.: Mater. Electron. 26, 9591 (2015).
J. Sun, H. Fan, B. Nan, and S. Ai, Sep. Purif. Technol. 130, 84 (2014).
S. Aslam, J. Zeng, F. Subhan, M. Li, F. Lyu, Y. Li, and Z. Yan, J. Colloid Interface Sci. 505, 186 (2017).
J. Wang, J. Yang, X. Li, D. Wang, B. Wei, H. Song, X. Li, and S. Fu, Physica E 75, 66 (2016).
S.K. Rajabi, and S.H. Sohrabnezhad, Microporous Mesoporous Mater. 242, 136 (2017).
J. Wan, H. Li, and K. Chen, Mater. Chem. Phys. 114, 30 (2009).
M.M. Naik, H.S.B. Naik, G. Nagaraju, M. Vinuth, K. Vinu, and S.K. Rashmi, J. Mater. Sci.: Mater. Electron. 29, 20395 (2018).
C. Perez, Acta Biol. Med. Exp. 15, 113 (1990).
X. Sun, G. Ma, X. Lv, M. Sui, H. Li, F. Wu, and J. Wang, Materials 11, 780 (2018).
S.D. Kulkarni, S.M. Kumbar, S.G. Menon, K.S. Choudhari, and C. Santhosh, Adv. Sci. Lett. 23, 1724 (2017).
C. Karunakaran, and P. Vinayagamoorthy, New J. Chem. 40, 1845 (2016).
R.Y. Hong, S.Z. Zhang, G.Q. Di, H.Z. Li, Y. Zheng, J. Ding, and D.G. Wei, Mater. Res. Bull. 43, 2457 (2008).
X. Huang, J. Lu, D. Yue, Y. Fan, C. Yi, X. Wang, M. Zhang, and J. Pan, Nanotechnology 26, 125101 (2015).
V. Madhubala, and T. Kalaivani, Appl. Surf. Sci. 449, 584 (2018).
Y.-J. Chen, F. Zhang, G.-G. Zhao, X.-Y. Fang, H.-B. Jin, P. Gao, C.-L. Zhu, M.-S. Cao, and G. Xiao, J. Phys. Chem. C 114, 9239 (2010).
M. Dehghani-Dashtabi, H. Hekmatara, and J. Seyed-Yazdi, Phys. B 553, 11 (2019).
C. Karunakaran, P. Vinayagamoorthy, and J. Jayabharathi, Langmuir 30, 15031 (2014).
V. Panwar, P. Kumar, A. Bansal, S.S. Ray, and S.L. Jain, Appl. Catal. A 498, 25 (2015).
X. Zhang, J. Wu, G. Meng, X. Guo, C. Liu, and Z. Liu, Appl. Surf. Sci. 366, 486 (2016).
A. Radoń, A. Drygała, Ł Hawełek, and D. Łukowiec, Mater. Charact. 131, 148 (2017).
P.G. Devi, and A.S. Velu, J. Theor. Appl. Phys. 10, 223 (2016).
S. Chidambaram, B. Pari, N. Kasi, and S. Muthusamy, J. Alloy Compd. 665, 404 (2016).
J.C.B. Huarac, M.S. Tomar, S.P. Singh, O. Perales-Perez, L. Rivera, and S. Pena, InNSTI-Nanotech. 3, 405 (2010).
Q.I. Rahman, M. Ahmad, S.K. Misra, and M. Lohani, Mater. Lett. 91, 170 (2013).
O.S. Bello, K.A. Adegoke, S.O. Fagbenro, and O.S. Lameed, Appl. Water Sci. 9, 189 (2019).
Y. Lai, M. Meng, Y. Yu, X. Wang, and T. Ding, Appl. Catal. B 105, 335 (2011).
J. Zhai, X. Tao, Y. Pu, X.-F. Zeng, and J.-F. Chen, Appl. Surf. Sci. 257, 393 (2010).
C.-C. Lin, and J.-M. Ho, Ceram. Int. 40, 10275 (2014).
S. Sun, X. Yang, Y. Zhang, F. Zhang, J. Ding, J. Bao, and C. Gao, Prog. Nat. Sci.: Mater. Int. 22, 639 (2012).
C. Singh, S. Jauhar, V. Kumar, J. Singh, and S. Singhal, Mater. Chem. Phys. 156, 188 (2015).
X. Cao, L. Gu, X. Lan, C. Zhao, D. Yao, and W. Sheng, Mater. Chem. Phys. 106, 175 (2007).
M.M. Naik, H.S.B. Naik, N. Kottam, M. Vinuth, G. Nagaraju, and M.C. Prabhakara, J. Sol-Gel. Sci. Technol. 91, 578 (2019).
M.M. Naik, H.S.B. Naik, G. Nagaraju, M. Vinuth, K. Vinu, and R. Viswanath, Nano-Struct. Nano-Objects 19, 100322 (2019).
C. Jayaseelan, A.A. Rahuman, A.V. Kirthi, S. Marimuthu, T. Santhoshkumar, A. Bagavan, K. Gaurav, L. Karthik, and K.V.B. Rao, Spectrochim. Acta Part A Mol. Biomol. Spectrosc. 90, 78 (2012).
Y.T. Prabhu, K.V. Rao, B.S. Kumari, V.S.S. Kumar, and T. Pavani, Int. Nano Lett. 5, 85 (2015).
S. Singh, K.C. Barick, and D. Bahadur, Powder Technol. 269, 513 (2015).
Acknowledgments
M. Shashank thanks Kuvempu University, Shivamogga, Siddaganga Institute of Technology, Tumkur and CNMS, Jain University, for constant support and encouragement. One of the authors Nagaraju Ganganagappa thanks DST Nanomission, Govt. of India, New Delhi (Project No. SR/NM/NS-1262/2013 dated 18-03-2015) and VGST, Govt. of Karnataka (VGST/SMYSR/2015-2016//GRD-498) for financial support to carry out the research work. Dr. M. Madhukara Naik thanks the Principal and the Management for supporting the research activity.
Author information
Authors and Affiliations
Corresponding author
Ethics declarations
Conflict of interest
The authors declared that they have no conflict of interest.
Additional information
Publisher's Note
Springer Nature remains neutral with regard to jurisdictional claims in published maps and institutional affiliations.
The original online version of this article was revised: M. Shashank’s family name was corrected.
Rights and permissions
About this article
Cite this article
Shashank, M., Naik, H.S.B., Nagaraju, G. et al. Facile Synthesis of Fe3O4/ZnO Nanocomposite: Applications to Photocatalytic and Antibacterial Activities. J. Electron. Mater. 50, 3557–3568 (2021). https://doi.org/10.1007/s11664-021-08816-9
Received:
Accepted:
Published:
Issue Date:
DOI: https://doi.org/10.1007/s11664-021-08816-9