Abstract
The contour method of residual stress measurement relies on the careful application of wire electro-discharge machining (WEDM) for the cutting stage. Changes in material removal rates during the cut lead to errors in the final calculated values of residual stress. In this study, WEDM cutting parameters have been explored to identify the optimum conditions for contour method residual stress measurements. The influence of machine parameters on the surface roughness and cutting artifacts in the contour cut is discussed. It has been identified that the critical parameter in improving the surface finish is the spark pulse duration. A typical cutting artifact and its impact on measured stress values have been identified and demonstrated for a contour cut in a welded marine steel. A procedure is presented to correct contour displacement data from the influence of WEDM cutting artifacts, and is demonstrated on the correction of a measured weld residual stress. The corrected contour method improved the residual stress magnitude up to 150 MPa. The corrected contour method results were validated by X-ray diffraction, incremental center hole drilling, and neutron diffraction.
Similar content being viewed by others
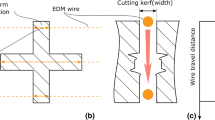
Avoid common mistakes on your manuscript.
1 Introduction
The contour method of residual stress measurement is a relatively new method that, while destructive, has significant advantages over many other techniques.[1–7] A stressed component is cut into two parts using wire electro-discharge machining (WEDM). This causes relaxation of residual stress on the cut surfaces. The resulting surface profiles are then measured to determine the displacements caused by the relaxed stresses. The measured displacements are applied as displacement boundary conditions to a model surface using the finite element method, and allowing the model to relax elastically provides a calculation of the pre-existing residual stress field.
Wire EDM is currently the best method available for application of the contour method. In wire EDM, an electrically charged wire, typically copper or brass, travels between wire guides on the top and bottom sides of the workpiece and material removal takes place by a spark erosion process. The component to be cut is placed in a temperature-controlled chamber filled with de-ionized water. The amount of material removed during WEDM is equivalent to the wire diameter and the overcut.[7] Tension is applied to the wire between the guides to keep it straight. Injection nozzles are used for flushing of cut workpiece material. The WEDM cutting process is depicted in Figure 1.
There are a number of potential types and location of wire EDM cutting errors and artifacts:[8,9] wire entry and exit artifacts; artifacts at the start of cut; wire breakage; and bowing and waviness of the cut surface. Errors can be symmetric and anti-symmetric with respect to the two cut surfaces. Cutting artifacts have significant impact on the quality of contour residual stress results. They can potentially be reduced by bonding sacrificial layers composed of a similar material at the affected locations, such as the EDM wire entry and exit points, and the start and the end of the cut.[10] The use of sacrificial layers to avoid errors and artifacts is further explored in this present study.
Reducing the conductivity of the de-ionized water used in the EDM chamber gives a slight improvement to the roughness of the cut surfaces.[11] However, reducing the conductivity of the water means that the machine filtration system may need additional maintenance which adds to the overall measurement cost. Reducing the pulse duration as well as the current has been shown to give improved surface roughness,[12] but only for machining by multiple cuts: no literature is available on the potential improvement obtained during a single cut as required for the contour method.
Noise in the displacement data at the edges of a sample may develop during WEDM machining or subsequent co-ordinate measuring machine (CMM) measurement, and it was initially suggested that stress values near edges should not be considered when applying the contour method.[7] Removal of more material near sample edges than in the center during cutting was also observed. Vibration and deflection of the wire, as well as spark intensity variations, are the main sources of dimensional inaccuracy of machined parts. Bending of the wire near the workpiece edges is often considered to be a cause of scatter in measured data, and as a remedy it is suggested to increase the wire tension.[12]
In this study, the cutting artifacts were determined and quantified for a marine steel, and the WEDM settings were investigated for minimization of these effects. A procedure is presented to remove the effects of such cutting artifacts on the final calculated residual stresses. We believe this is the first systematic study to incorporate the correction of WEDM cutting artifacts into contour method measurement results.
2 Experimental Procedure
The experimental procedure is grouped into five sub-sections that cover: selection of WEDM machine; the use of sacrificial layers; selection of cutting parameters; reduction of surface roughness; and incorporation of correction for WEDM cutting artifacts into contour method measurements. Before undertaking cutting tests, the following WEDM settings were adjusted:
-
Wire tension to near the maximum allowable value.
-
Low conductivity of de-ionized water used in WEDM chamber.
-
Moderate flushing pressure from both injection nozzles.
-
Symmetric clamping.
The conductivity of the de-ionized water used in the WEDM chamber was in the range of 9 to 10 µS/m. It was verified that the material did not have an initial residual stress. Material blocks were extracted from the DH36 ship steel and stress relieved at 873 K (600 °C) in a furnace for 1 hour. X-ray diffraction (XRD) on-surface measurements were performed to examine the state of residual stress after heat treatment. The stress values were found to be less than 10 MPa.
Tests with sacrificial layers were carried out to examine the reduction of wire cutting artifacts. Two types of sacrificial layers were used. One was made of the parent material, with silver-epoxy metallic adhesive containing about 70 pct silver used to attach the sacrificial layers to the samples. The adhesive used must give a strong bond and be electrically conductive. Sacrificial layers of 6 and 10 mm thickness were used at the wire entry and exit, and start and end of cut locations.
A low-melting point (LMP) bismuth-based alloy was also used as a sacrificial layer in some cutting tests. METTLER thermoanalysis laboratory equipment and its associated software were used to measure the latent heat and melting point of the alloy. The melting point of the alloy was 349 K (76 °C). The use of LMP alloy as a sacrificial layer can help to fill gaps that it would be difficult to fill using adhesive alone because of the consequent variation in properties along the cut; and it is particularly useful on rough and uneven surfaces where it is difficult to prepare a matching sacrificial shape from steel.
3 Results
3.1 Identification of Cutting Artifacts
The initial cutting tests looked at the selection of WEDM machine from the two available, as well as an initial study of the wire diameter and the cutting parameters. Sacrificial layers were not used for this series of tests. The EDM machine and wire diameter for contour measurement of residual stress were selected by cutting tests on stress-free DH36 steel. The size of the sample extracted was 15 × 10 × 25 mm3. The chemical composition of DH36 steel as per the International Association of Classification Societies (IACS) is given in Table I.
Owing to the large number of parameters available in wire electric discharge machining, and some variations in the cutting process as well as in terminology and units between different machine manufacturers, it was not possible to adjust the parameters on the different machines available to be equivalent. Therefore, machine default settings for a single cut were selected, and the best basis of comparison was accomplished by fixing the cutting speed. It is important to note that once the cutting speed is fixed, then the machine automatically adjusts other cutting parameters such that it achieves the required speed.
The summary of cutting conditions used in the first series of tests is listed in Table II. Cutting conditions 1a and 2a looked at the selection of wire EDM machine by keeping similar cutting speed, wire diameter, and wire tension. Condition 3a examined the effect of using a smaller wire diameter and increasing the flushing nozzle injection pressure. Table II also provides the measured surface roughness, expressed as R a, on the cut surfaces.
Wire EDM machines generally set high injection pressures when using small wire diameters. High pressure at small gap widths is expected to be more effective to remove debris from the cutting area.
The surface profiles obtained from the three WEDM cutting conditions along the cutting path are shown in Figure 2. From Figure 2(a) and Table II, it can be seen that a significantly rougher surface was obtained with machine 1, and also that there was a notable artifact at the start of the cut. When the spark locations in the EDM process are uniformly distributed, a flat and smooth surface finish results.[13] If there is variation in the EDM wire gap width owing to debris, the spark locations become localized and this results in an irregular surface.
Machine 2 gave a much improved surface profile. As a result of the improvement introduced by cutting condition 2a, it was selected as the starting point for further optimization of the machine parameters. The best surface roughness obtained in these trials was with the smaller wire diameter and high flushing pressure, although in this case those conditions did still show a small artifact at the start of the cut (Figure 2c).
Machine 2 was, therefore, used for the further trials. The results indicate that, where more than one WEDM machine is available, it is likely that different results will be obtained for similar conditions.
3.2 Optimization of Cutting Conditions
The second series of cutting tests looked at additional cutting parameters, and the effect of using sacrificial layers adhered to the sample. To examine the influence of single parameter on cut quality, it is preferable to change one parameter at a time. The conditions used are shown in Table III. During the phase of adjusting/setting machine parameters, it was found that the cutting speed achieved with default parameters for this particular material, and the cross-section is 2.2 mm/minutes. For condition 1b, the cutting speed was restricted to 0.5 mm/minutes to examine the effect of low cutting speed on surface quality. No sacrificial layers were used for this cutting condition. Conditions 2b, 3b, and 4b looked at the improvement introduced by using steel sacrificial layers. Conditions 5b and 6b examined the improvement with sacrificial layers made of low-melting point alloy material which was used at the wire entry and exit sides only, whereas for the start and end of cut locations no sacrificial layers were used. A dam was built on the sample to allow pouring of the LMP alloy in liquid form. For conditions 2b, 4b, and 6b, lower pulse durations were used to examine the effect on the quality of surface produced.
From the roughness measurements on samples 2b, 3b, and 4b, it is seen that there is improvement in surface finish with a decrease in pulse duration time: for condition 3b, an average Ra value of 1.8 µm was obtained, which decreased further for condition 4b to 1.6 µm. It is important to mention that for the pulse duration value there is a limit below which a cut could not be made. The roughness value associated with the machine default setting is about 2.6 µm. Longer exposure of the EDM spark to the sample surface may impart more deterioration in the form of a rougher surface, hence the benefit of using the reduced pulse duration.
The surface profiles presented here are averaged data from the mating surfaces. The surface profiles were measured using a CMM with a 3-mm-diameter touch probe with spacing between measurements points of 0.5 mm. The closest measurements to the sample edge were taken at 0.5 mm.
Condition 1b demonstrates the potential effects of a non-optimal cut on the final stress calculations. Condition 1b did not have the best surface roughness of the conditions tested (that was condition 4b). Condition 1b showed no artifact at the start or end of the cut; however, a wire entry artifact is seen over the first ~1 mm from the wire entry position. Also, and most importantly, there is a curved surface profile along the wire travel path as shown in Figure 3. Such a surface profile would result in an apparent residual stress in the material, despite it being stress-free: in this case, the residual stress would appear to be tensile near the surfaces and compressive in the center.
A three-dimensional, rectangular FE model of the cut surface pertaining to cutting condition 1b was created, and the averaged displacement data as shown in Figure 3 were smoothed using a cubic spline with 7 × 7 mm2 knot spacing before applying them as displacement boundary conditions to the model surface. A linear elastic FE analysis was performed to extract the stresses associated with this displacement data. The FE analysis was performed with Abaqus software using 8-node brick elements (C3D8R). Two boundary nodes were constrained to avoid rigid body motion of the model. The distance between element nodes in both X and Y directions is 1 mm. As can be seen from Figure 4, the surface profile generates the spurious result that tensile stresses are present near the surface on both wire entry and exit sides. This fictitious stress associated with this cutting condition will cause local offsets in the actual residual stress profile when applying the contour method. Such detrimental effects are further magnified with an increase in magnitude of the displacement variation on the cut surface.
Condition 2b used machine default settings along with steel sacrificial layers. An irregular surface profile as well as an artifact at the end of cut is associated with this condition. Large scatter in the data is seen along the cutting direction, with a curved profile as depicted in Figure 5.
Condition 4b used significantly reduced WEDM pulse duration and resulted in the best surface profile among all the tests in respect of low scatter, flatness of cut, and no significant artifact at the start or end of cut. This condition employed a steel sacrificial layer and also gave best surface along the wire travel path among all tests in respect of no wire entry or exit artifacts. The averaged surface profiles pertaining to this cutting condition along the cut path and sample thickness are given in Figure 6.
Conditions 5b and 6b used low-melting point alloy as a sacrificial layer at wire entry and exit locations only. No sacrificial layer was used at the start of the cut and an artifact at that location was seen. Condition 6b gave a better surface profile in respect of low scatter; however, through the sample thickness, there is a slight curved profile mainly caused by scatter in the data at the wire entry and exit sides as shown in Figure 7.
It is concluded that condition 4b gave the best surface in respect of no significant artifacts during the cutting. The cut surface was sufficiently flat, and the data scatter range was the lowest as compared to other conditions. This condition also gave the best surface finish. The low duration of the EDM spark helps to prevent degradation of the surface that can result in increased roughness. The results of this study suggest that a contour cut should be performed at the smallest workable pulse duration, which needs to be determined for each material and cross-section. One way to achieve this is to use machine default settings and then reduce the pulse durations setting to a minimum workable value.
A slot was made in stress-free DH36 steel with wire EDM using cutting condition 1b and examined using an optical microscope for the variation of size of the slot along the cutting path. The cut was made with a wire diameter of 0.25 mm. The slot size was seen to be greater at the start of the cut by around 6 to 10 µm compared to the middle of the cut location. More material removal took place at the start of the cut and it stabilized after about 1 mm cut into the material. A higher slot size at the start of cut recommends the use of sacrificial layers at this location to stabilize the cut. However, this is a local approach that works at the perimeter of the slot only. A complete picture of cut surface can be provided by the displacement data obtained from the CMM.
3.3 Effect of Reduced Pulse Duration
From the second series of cutting tests, the importance of reduced pulse duration setting on the quality of the surface was seen for DH36 steel in respect of the roughness level achieved (full results are given in Section III–B). To explore the application of this particular setting adjustment to other materials, further cutting tests were defined, as indicated in Table IV. Three materials were tested: another class of ship steel, DH275; 316L steel; and Al7475-T7351 aluminum. These three materials were tested in comparison with the WEDM single cut default setting.
The results clearly show that a reduced pulse duration gives an improvement in surface finish for all the materials investigated. Figure 8 shows the surface displacement line profile pertaining to cutting conditions 1c and 2c. The displacement profile was measured using Taylor Hobson Surtronic 3+ roughness tester using its 5 µm diameter diamond tip. The acquired data were afterward flattened to place zero origin in the middle of whole dataset. Flattening removes the planar component from the data by shearing the points and places the axis in the middle of the dataset.[16]
Condition 2c shows improvement in surface profile compared to condition 1c. During measurements of the surface contour using a co-ordinate measurement machine (CMM), the surface displacement peaks shown in Figure 8 play an important role as the CMM probe may measure that position after being deflected from that position. Thus, errors in CMM measured displacement data occur owing to high roughness levels and therefore good surface finish reduces error in the measured displacement data for the contour method.
The surface displacement profiles of 316L and Al-7475 show a similar trend, with improved surface profile with reduced pulse duration settings. This can be taken as a general conclusion from this study.
3.4 Effect and Mitigation of Macroscopic Curvature
The fourth series of tests moved on from studying the surface roughness alone, for ensuring that the cut obtained is macroscopically flat. Even with low surface roughness, a flat cut still remains the basic requirement for the contour method, and as good practice it should be verified before undertaking the contour cut. Tests were conducted with sacrificial layers attached at the EDM wire entry and exit points, and at the start and end of the cut. Table V shows the various cutting conditions tested.
The surface profiles from these cutting conditions all showed a convex shape profile through the thickness of the sample. The level of scatter was the lowest in the case of condition 2d, as a consequence of the reduced pulse duration. The profile around the wire that leads to this result is shown in Figure 9. As shown in Section III–A, such a convex profile introduces errors in the final stress values. The averaged isometric surface profile from condition 2d is plotted in Figure 10, and shows the convex shape profile through the thickness of the sample.
Cutting condition 3d used a slow cutting speed to reduce the impact of possible wire vibration; however, the results showed no improvement. The wire tension and injection nozzle pressure were set to the upper limit of the machine for condition 2d. Slowing the wire travel speed further and increasing its tension above the recommended level gave no additional improvement. From these cutting tests, condition 2d was selected as it gave the best surface profile. Figure 11 shows the surface displacement profile obtained from conditions 1d, 2d, and 3d, and it can be seen that condition 2d has the lowest range of scatter.
Symmetric WEDM cutting errors are critical as they do not average out during data analysis stage.[14] These errors can be removed by subtracting the surface profile obtained from a stress-free form of the material from the actual measured profile. An easy way to obtain the stress-free profile is by cutting a small slice from the contour cut part.
Figure 11 shows the surface displacement profile of cutting conditions 1d, 2d, and 3d. From Section III–C, the cutting condition 2d gave the best surface profile, and therefore, was used for further analysis.
The specific cut shape profile shown in Figure 9 needs therefore to be accounted for by displacement data correction for contour method measurements. For this purpose, an approximation of the averaged and flattened displacement data of condition 2d was made by a cubic polynomial function, and the fit was extended to the edges of the sample (Figure 12). The extracted data from the polynomial fit were then subtracted from the actual contour displacement data, which was also averaged and flattened before correction.
The procedure for the data correction to deal with the wire EDM cutting artifacts is as follows:
-
(1)
Prior to making the actual contour cut, make a WEDM reference cut with the following requirements:
-
(a)
A small, stress-free form of the same material. Smaller samples will retain less residual stress and if there is retained stress, the corresponding surface displacements will be small.
-
(b)
Similar thickness as for the actual contour cut.
-
(c)
Sacrificial layers made of similar material.
-
(d)
Optimized cutting conditions with regard to surface roughness.
-
(a)
-
(2)
Measure the surface contour from the stress-free coupon.
-
(a)
Average and flatten the displacement data of the reference cut surfaces.
-
(b)
Approximate the averaged and flattened data of the reference cut with a polynomial function. It is important that the fitting routine should follow the trend in the data.
-
(a)
-
(3)
The optimized cutting conditions should be applied for the actual contour cut(s). Any clamping arrangement should be as similar as possible between the two cases, as should be any sacrificial layers used.
-
(4)
For CMM measurements, the distance between measurement points as well as from edges of the sample should be identical for both reference and actual cut. The fitted polynomial data from the reference cut should be extracted such that it matches the CMM measurement density of the actual contour cut.
-
(5)
During data analysis, subtract the extracted data from the polynomial fitting of the reference cut from the actual contour cut data, which should also be averaged and flattened before correction.
-
(6)
Use the corrected displacement data to obtain the final contour stress map.
4 Validation Experiment
This correction scheme was applied to a laser peened butt-welded sample at the weld crown toe location. Full details of this sample are given in Reference 15. The laser peened sample was 16 mm thick and 90 mm wide. Laser peening was performed across the weld and on both sides of plate. It's material was ship steel DH275, and Section III–D shows the WEDM trial cuts performed for this material. A convex shape cutting artifact as seen from trial cuts was encountered along the WEDM wire travel, i.e., thickness of specimen. An improvement in stress distribution was achieved with the correction process as shown in Figure 13. The contour method corrected results are validated by surface X-ray diffraction, near-surface incremental center hole drilling, and through-thickness neutron diffraction measurements.
As in this case, the cutting artifact mainly affected the surface profile along the wire travel direction— i.e., through the thickness of the sample—therefore, the analysis proposed was sufficient to deal with it. For situations where cutting artifacts are present along both wire travel and cutting directions, a surface fit to the data is required which is then subtracted point-by-point from the surface profile fit to the measured contour. Other than that, the correction procedure remains the same.
5 Conclusions
-
1.
A correction method has been developed for application to the contour method of residual stress measurement in cases where a flat cut cannot be obtained. Artifacts during EDM cutting can occur at various locations, including the wire entry and exit points, and at the start and the end of the cut. The width of the cut slot can also vary. If not prevented or corrected for, these artifacts will manifest as errors in the final calculated values of residual stress.
-
2.
Surface roughness increases noise in the contour displacement data, so the cut settings that provide the best possible surface finish should be applied. In this study, we have found that improved surface roughness from the EDM cutting process is obtained by decreasing the pulse duration setting.
-
3.
Sacrificial layers minimize very-near-surface cutting irregularities along the perimeter of the cut. This is particularly important if the method is being applied for the determination of near-surface residual stress.
-
4.
Data correction of the measured contour is necessary for those cutting artifacts that are not eliminated with the use of sacrificial layers. A series of steps has been presented for correcting the data when the reference cut is not flat.
-
5.
The method was applied to a welded sample with high near-surface stresses. The correction method increased the measured near-surface stress by around −150 MPa, a significant change. The results were validated by other experimental stress analysis techniques, showing the efficacy of the correction technique proposed.
References
D.W. Brown, T.M. Holden, B. Clausen, M.B. Prime, T.A. Sisneros, H. Swenson, J. Vaja: Acta Materialia, 2011, vol. 59, pp. 864–73.
P. Pagliaro, M.B. Prime, H. Swenson, B. Zuccarello: Experimental Mechanics, 2010, vol. 50, pp. 187–94.
M.E. Kartal, C.D.M. Liljedahl, S. Gungor, L. Edwards, M.E. Fitzpatrick: Acta Materialia, 2008, vol. 56, pp. 4417–28.
A.T. DeWald, M.R. Hill: Experimental Mechanics, 2006, vol. 46, pp. 473–90.
Y. Zhang, S. Ganguly, L. Edwards, M.E. Fitzpatrick: Acta Materialia, 2004, vol. 52, pp. 5225–32.
M.B. Prime, R.J. Sebring, J.M. Edwards, D.J. Hughes, P.J. Webster: Experimental Mechanics, 2004, vol. 44, pp. 176–84.
M.B. Prime: J. Eng. Mater. Technol., 2000, vol. 123, pp. 162–68.
F. Hosseinzadeh, P. Ledgard, P.J. Bouchard: Experimental Mechanics, 2013, vol. 53, pp. 829–39.
F. Hosseinzadeh, J. Kowal, P.J. Bouchard: Journal of Engineering, 2014, http://digital-library.theiet.org/content/journals/10.1049/joe.2014.0134.
A. Kundu, P.J. Bouchard, S. Kumar, K. Venkata, J. Francis, A. Paradowska, G. Dey, C. Truman:, Sci. Technol. Weld. Join., 2013, vol. 18, pp. 70–75.
C. Arunachalam, M. Aulia, B. Bozkurt, P.T. Eubank: J. Appl. Phys., 2001, vol. 89, pp. 4255–62.
O. Dodun, A.M. Gonçalves-Coelho, L. Slătineanu, G. Nagîţ: Intl J. Advanced Manufacturing Technol, 2009, vol. 41, pp. 858–64.
M. Kunieda, B. Lauwers, K.P. Rajurkar, B.M. Schumacher: CIRP Annals - Manufacturing Technology, 2005, vol. 54, pp. 64–87.
M.B. Prime, A.L. Kastengren: in T. Proulx (Ed.) Experimental and Applied Mechanics, Volume 6, Springer New York, 2011, pp. 233–50.
B. Ahmad and M. E. Fitzpatrick: The Journal of Engineering, 2015, DOI: 10.1049/joe.2015.0084, Online ISSN 2051–3305.
G. Johnson. PhD Thesis, University of Manchester, 2008.
Acknowledgments
We wish to thank to Peter Ledgard and Damien Flack at The Open University for performing the EDM cutting. The authors are grateful for funding from the Lloyd’s Register Foundation, a charitable foundation helping to protect life and property by supporting engineering-related education, public engagement, and the application of research.
Author information
Authors and Affiliations
Corresponding author
Additional information
Manuscript submitted June 5, 2015.
Rights and permissions
About this article
Cite this article
Ahmad, B., Fitzpatrick, M.E. Minimization and Mitigation of Wire EDM Cutting Errors in the Application of the Contour Method of Residual Stress Measurement. Metall Mater Trans A 47, 301–313 (2016). https://doi.org/10.1007/s11661-015-3231-7
Published:
Issue Date:
DOI: https://doi.org/10.1007/s11661-015-3231-7