Abstract
The concentration of radon in groundwater is mainly governed by the radium content in the rocks of the aquifer. The internal exposure to high levels of radon in water is directly associated with the radiological risk to members of public. In this work, radon concentrations were measured in groundwater of Garhwal Himalaya, India, using scintillation detector-based RnDuo and silicon detector-based RAD7 monitors. An inter-comparison exercise was carried out between RnDuo and RAD7 techniques for a few samples to validate the results. The radiation doses associated with the exposure to radon in water were estimated from measured values of activity concentrations. An attempt has been made to see the effect of geology, geohydrology and different types of sources on radon levels in Himalayan groundwater. The experimental techniques and results obtained are discussed in detail.
Similar content being viewed by others
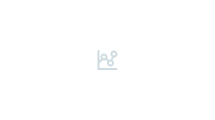
Explore related subjects
Discover the latest articles, news and stories from top researchers in related subjects.Avoid common mistakes on your manuscript.
Introduction
Drinking water is generally obtained from ground and surface water sources. Several naturally occurring radioactive elements present in groundwater contaminate the drinking water sources. The concentrations of these radioelements in water depend on physical, chemical and geological nature of the aquifer (Jobbágy et al. 2010; Przylibski and Gorecka 2014; Fonollosa et al. 2016). Radon is the most important element among these radioelements from a radiation protection point of view. It is formed in the earth by radioactive disintegration of radium. Radon enters the aquatic environment most importantly by the dissolution from the nearby geological environment such as rocks and soil. (Choubey and Ramola 1997; Moreno et al. 2014; Jobbágy et al. 2017). The concentration of radon in spring and hand pump water is influenced by 226Ra concentration in rocks and abundance of 238U minerals (Choubey et al. 2000). Although radon is little soluble in water, its activity level in groundwater can be high due to high level of radon concentration in rocks and soil. The major health risk associated with radon exposure arises from the inhalation of short-lived progeny of radon which is considered as the second most important cause of lung cancer, next to smoking (WHO 2009). Ingestion of radon through water and food has been indicated as a probable cause of stomach cancer in some studies (Todorovic et al. 2012; Ravikumar and Somashekar 2014). Alpha emissions of 214Po and 218Po (radon daughters) are major contributors to the total radiation dose due to radon exposure. The existence of high radon levels in water poses a health risk to the public through inhalation of radon and its decay products released from water into indoor atmosphere and through direct ingestion of radon in drinking water (USEPA 1999; Kendall and Smith 2002; WHO 2011). About 90% of the total dose associated with exposure to radon in drinking water is attributed to inhalation rather than ingestion (Duggal et al. 2017). For drinking water, WHO has proposed a radon reference limit of 100 Bq l−1 in its drinking water guidelines (WHO 2008). However, radon guidance level has not been incorporated in the recent WHO drinking water guidelines and no other reference level is mentioned (WHO 2011). The European Union (EU) council proposes a reference value of 100 µSv year−1 for annual effective dose received by the ingestion of various contaminants in drinking water (EU Council Directive, 98/83/EC 1998). This value does not include the dose received from 3H, 40K, 222Rn and its progeny. It is, therefore, important to carry out systematic study for assessment of doses associated with radon in water. In addition, in situ measurements of radon concentrations in groundwater are helpful in exploring uranium deposits, examining hidden faults and thrusts, etc. (Prasad et al. 2009). In this study, the measurements of radon concentrations were performed in groundwater sources of Garhwal Himalaya using scintillation detector-based RnDuo and silicon detector-based RAD7 radon monitors. The measured radon levels were used to estimate associated age-dependent ingestion doses and inhalation doses. This is the first attempt which has been made to see the variation of dose exposure rate among different age groups. An effort has also been made to see the effect of geology and geohydrology on radon levels in groundwater. The results of radon measurements obtained from different types of sources (springs, hand pumps and a few taps) were compared to see the variability of radon levels in these sources. The radon levels measured with RnDuo and RAD7 monitors were compared for a few samples validate the results. In this way, present study is important from dose assessment point of view and the results may serve as baseline data in future epidemiological studies. In addition, the observed variability of radon levels in different types of groundwater sources, the dependence of radon levels on geological and geohydrological parameters may be useful in exploring uranium deposits and in understanding other geochemical processes. The description of experimental techniques, results and conclusions is given below.
Study area
Geology of the study area
The geological map of the study area showing the sampling locations is shown in Fig. 1. The study area comprises three regions (or zones) in Garhwal Himalaya, India, namely: Kedar (or Mandakini) valley of Rudraprayag district, Karnprayag area of Chamoli district and Tehri area of Tehri Garhwal district. The sampling locations were chosen such as to cover the three different geological zones representatively. The Kedar (Mandakini) valley lies in Vaikrita Group of Central Crystalline which comprises of the Munsiari, Joshimath, Pandukeshwar and Pindari formations (Heim and Gansser 1939). The rocks of the area are surrounded by Munsiari Thrust and Trans Himadri fault that belong to Central Crystalline Badrinath zone (Heim and Gansser 1939; Gupta 1977; Sinha 1989). The Kedarnath region in Munsiari formation is mainly composed of mica schist, calc silicate lances, quartzites and phyllonites, etc. (Valdiya et al. 1999). Granitic rocks are also found in the upper part of Kedarnath area. The regional geology of Karnprayag area belongs to Lesser Garhwal Himalaya which is composed of metamorphic rocks (Heim and Gansser 1939). The geological structure of Tehri Garhwal is complex due to recurrent tectonic disturbances in the region. The area is composed of two broad tectonic zones viz. Lesser Himalaya and Central Himalaya. A group of metamorphic rocks is exposed in the Central Himalaya (Valdiya 1980). The Tehri area of Tehri Garhwal district lies in the Lesser Himalaya.
Geohydrological framework of Himalayan springs
Geohydrology may be useful in understanding the variation of radon levels in groundwater sources. Groundwater is transported to the earth’s surface through springs via faults, fractures, joints, etc. There are two kinds of water bearing geological formations in Garhwal Himalaya: (a) fractured hard rocks and (b) Colluvial and fluvial deposits. If fractures and joints are main governing parameters; springs are referred as fracture–joint (FJ) related springs. However, if colluvial or fluvial deposits play significant part in the formation of springs, they are referred as colluvial/fluvial (CL/FD)-related springs (Valdiya and Bartarya 1991; Choubey et al. 2000). Groundwater sources (springs and hand pumps) in Mandakini valley (Kedar valley) are influenced by FJ-related rocks of Vaikrita and Munsiari unit. On the other hand, the springs and hand pumps located in Karnprayag and Tehri regions are affected by colluvial and fluvial deposits (Choubey et al. 2000).
Measurements of radon concentration in drinkable groundwater
The measurements of radon concentration in 67 groundwater samples of study area have been carried out using RAD7 and RnDuo techniques. The water supplied from all these water sources is used by the public for drinking purpose. A brief description of sampling procedure and measurement techniques is given below.
Materials and methods
Sampling procedure
Radon can easily escape from water; therefore, water samples were collected in such a manner that there is least aeration. A schematic of the sampling procedure is shown in Fig. 2. The bubbles formed in the water may result in outgassing of the dissolved radon. The fresh water samples were analyzed using RAD7 and RnDuo instantly on the spots so as to avoid decay correction due to time difference between sample collection and analysis. For better precision of results, RAD7 detector was purged for a sufficient time to minimize the relative humidity (RH) of less than 15% prior to analysis of samples. RAD7 was operated in grab mode using WAT 250 protocol for radon measurements in water (RAD7 User Manual Durridge 2013).
Measurements using RAD7 technique
RAD7 is based on the principle of solid-state semiconductor (silicon) detector which converts the alpha radiation into an electrical pulse. It is a battery-operated transportable device consisting of an air pump and a radon monitor. It utilizes RAD H2O accessory for radon measurements in water. The schematic diagram of RAD7 with RAD H2O accessory is shown in Fig. 3. RAD7 consists of a 0.71-L hemispherical internal cell, the internal surface of which is coated with electrical conductor. At the middle of hemisphere a semiconductor (Si) α-detector is positioned which transforms α-rays to an electrical pulse. A very high potential of 2000–2500 V is applied between the internal surface of the conductor and silicon detector using high voltage–power circuit. The generated electric field repels positively charged alpha particles towards the detector. The samples were collected in special type of radon tight vials of 250 ml capacity specially designed for water measurements using RAD7. For the purpose of measuring 222Rn level in water, RAD7 detector is coupled with a water bubbling kit. The bubbling kit ejects the dissolved radon from water sample and delivers it to hemispherical cell using an air circulating pump. The radon inside the cell decays to 218Po which is collected on silicon detector with the help of applied electric field. Alpha emissions from collected 218Po atoms are detected by the silicon detector and radon concentration is estimated from the count rate of 218Po. The results are displayed after 30 min analysis with good sensitivity. The air pump initially runs for 5 min to aerate the water contained in sampling bottle and sends the released 222Rn to the hemisphere. The state of equilibrium is reached within 5 min of air circulation by pump. Effectiveness of the pump to extract radon from water is about 94% for 250 ml sample (RAD7 User Manual Durridge 2013). However, the extraction efficiency is influenced by ambient temperature but it is always above 90% (RAD7 User Manual Durridge 2013). After 5 min, the pump stops automatically and the system counts alpha emissions of 218Po for next 5 min. The acquired data along with respective bar charts and cumulative spectra of each sample can be printed by the printer associated with the device through sensor. The counting process repeats for 5 min cycle to give accurate results.
Measurements using RnDuo technique
The experimental arrangement for radon measurements in water using RnDuo technique is shown in Fig. 4. This technique utilizes the principle of detection of α-radiation produced by 222Rn disintegration series atoms by ZnS (Ag) scintillation cell and converting the measured counts to radon activity concentration using a radioactive decay–growth algorithm implemented in the micro-controller of the system (Gaware et al. 2011). The experimental arrangement involves a water bubbler with the continuous radon monitor, SMART RnDuo. The water bubbler transfers a major portion of dissolved radon from water to air volume of setup within minute time. The transfer fraction depends upon the ratio of air to water volume and transfer coefficient between air and water. The detector volume is flushed for 5 min using the inbuilt micro pump of the monitor before connecting water bubbler to the monitor. Once the connection is made, the pump is kept running again for 5 min to transfer the dissolved radon to the detector volume of the monitor. In this study SMART RnDuo was operated for 1 h with 15 min cycle for a particular water sample. The 222Rn level in water is estimated from 222Rn level measured in air using following relation:
where Cw and Ca represent radon concentrations (Bq m−3) in water and air volume of monitor, respectively. Va and Vw are volumes of air and water, respectively, and K is partition function between air and water. In present investigation, by selecting tubing of appropriate air volume, sample volume (Vw) and air volume (Va) of whole set up were adjusted in such a way to achieve air volume to sample volume ratio (Va/Vw) to be nearly 10. The value of partition coefficient (K) between water and air is approximately equal to 0.25 and may vary marginally from sample to sample depending upon the carbon content and other composition in groundwater. Also, it may vary with temperature variation in groundwater. Nevertheless, this variation is marginal and K factor is negligible compared to ratio of air volume to sample volume (Va/Vw). Thus, K factor does not affect much the estimated radon concentration in water.
Results and discussion
Descriptive statistics of radon levels in groundwater of Garhwal Himalaya
The descriptive statistics of measured values of 222Rn in drinking water of Garhwal Himalaya is shown in Table 1. 222Rn levels in drinkable groundwater of Garhwal Himalaya ranged from 1.7 to 400 Bq L−1 with a mean of 45 Bq L−1 and from 1.7 to 210 Bq L−1 with a mean of 37 Bq L−1 using RnDuo and RAD7, respectively. The mean values (45 and 37 Bq L−1) of radon levels are significantly higher than the maximum concentration limit (MCL) of 11 Bq L−1 proposed by USEPA (1991). The high radon levels are due to the fact that the analyzed water sources are groundwater sources and not the surface water sources. The ingestion of this water may result in harmful health effects. Thus, the people of the investigated region were suggested to keep groundwater in open containers for few hours so that radon gets released before the water is used for drinking purpose. Further, it is worth highlighting that radon levels are well within the reference limit of 100 Bq L−1 established by European commission (EU 2001) and World Health Organization (WHO 2004) in 94 and 97% samples measured with RnDuo and RAD7, respectively. Limited literature is available on radon levels in groundwater of Garhwal Himalayan region. A wide variation in radon concentration ranging from 0.4 to 887 Bq L−1 was reported in groundwater samples of Garhwal Himalaya (Choubey et al. 2000).
Effect of geology on radon levels in groundwater
Radon is a member of uranium series which is formed by the decay of radium. Although radium is normally present everywhere in the soil, its concentration changes with geology of different regions. The regions with high levels of radium and/or uranium bearing granitic rocks are expected to result in occurrence of elevated levels of radon in groundwater. However, the regions with sedimentary rocks or soil would have relatively lower levels of radon in groundwater. To see the effect of geology on radon levels in groundwater, radon measurements were performed in groundwater of three different regions, namely Kedar (or Mandakini) valley of Rudraprayag district, Alaknanda and Pindar valleys of Chamoli district, and Chamba (New Tehri) area of Tehri Garhwal district in Uttarakhand state of India. The geographical variability of radon concentrations in groundwater samples of Garhwal Himalaya is shown in the Table 2. A wide variation of radon levels in groundwater has been seen over these regions. The mean values of radon concentrations measured with RnDuo in the three regions were found to be 39, 15 and 31 Bq L−1, respectively. The mean values of radon concentrations measured with RAD7 monitor in the three regions were found to be 42, 24 and 37 Bq L−1, respectively. The relatively higher values of radon concentrations were observed in Kedar valley of Rudraprayag district. On the other hand, lower values of radon concentrations were observed in Pindar valley of Chamoli district. The comparatively higher levels of radon in groundwater of Kedar valley may be due to richness of radium-bearing minerals in this region. The radium content in the soil of Ukhimath region (nearby to investigated region in Kedar valley) is reported to be varying from 38.4 ± 6.1 Bq kg−1 to 141.7 ± 11.9 Bq kg−1 with an average of 80.5 Bq kg−1 (Rautela et al. 2012). The investigated region of Mandakini valley is a granitic terrain which is close to two important faults known as Vaikrita Thrust and Pindari Thrust. These geological peculiarities may help to explain high radon levels in groundwater of this region since such tectonically active region presents a greater surface for radon emission from rocks. In addition, granitic rock aquifers exhibit higher radon content compared to sedimentary rock aquifers because uranium is accumulated in such rocks (Fonollosa et al. 2016). These reasons justify the higher radon levels observed in groundwater leaving from granitic rocks of Mandakini valley compared to the samples originating from other geological structures. On the other hand, the relatively lower levels of radon in groundwater of Karnprayag area of Chamoli district and Chamba (New Tehri) region of Tehri Garhwal district may be attributed to sedimentary rock formation of these regions.
Geohydrological explanation of radon variability in groundwater
Radon levels in groundwater were found to be influenced by geohydrology of the area. The high radon levels were found in the fault–lineament (FL) and fracture–joint (FJ) type springs in Mandakini valley (Kedar valley) compared to those in colluvial (CL)- and fluvial (FD)-related springs in Pindar and Alaknanda valleys of Chamoli district and Tehri Garhwal district. The extremely fractured and jointed rocks surrounding a FJ-related spring present an increased rock surface area to water volume ratio, thereby increasing radon emission rate (Lawrence et al. 1991; Choubey et al. 2000). Nashine (1982) identified uranium mineralization in the form of uraninite and fluorapatite in Bhatwari thrust where most of the FL-related springs are found. On the other hand, the relatively lower levels of radon in colluvial (CL)- and fluvial (FD)-related springs may be associated with higher water carrying capability, higher permeability and higher porousness of colluvial and fluvial deposits. The high porosity of colluvial and fluvial deposits results in migration of radon into atmosphere. Thus, radon does not accumulate to considerable level in CL and FD related springs. The high transmissivity (flow rate per unit width per unit gradient) of colluvial and fluvial deposits results in decreasing emanation efficiency of 222Rn due to lesser surface area of rock to water volume ratio for a large volume of water. However, a large variation in radon levels has also been observed in the some springs with identical geohydrological background. The relatively lower levels of radon have also been found in few FL- and FJ-related springs. This is attributed to the fact that these samples were collected at locations considerably away from the spring sources located on hills and water flows downward. The radon gets released into the atmosphere on the way before reaching the sample collection point. The high radon values in few colluvial and fluvial springs may be due to sedimentation of 238U and/or 226Ra minerals that are transported from the catchment area.
Comparison of radon levels on the basis of water sources
Radon measurements were carried out in water samples collected from three types of sources; springs, hand pumps and taps. Radon levels in different sources of water are shown in the Table 3. The mean values of radon concentration in spring water samples were found to be 56 and 35 Bq L−1 using RnDuo and RAD7, respectively. In tap water samples, mean radon levels were found to be 38 and 25 Bq L−1 using RnDuo and RAD7, respectively. The mean values of radon concentration in hand pump water samples were found to be 44 and 44 Bq L−1 using RnDuo and RAD7, respectively. The high values of radon levels were observed in spring and hand pump water, whereas low levels of radon were found in tap water samples. The radon gets released in the way between water source and point of sample collection resulting in a lower radon concentration in tap water compared to springs and hand pumps. The spring water contains 222Rn escaping from about few meters from earth’s surface. However, the water is not generated at a particular point but it is flows from a considerably large volume around springs or hand pumps.
Inter-comparison of radon levels measured with RAD7 and RnDuo
An inter-comparison test was performed between RnDuo and RAD7 techniques for 33 samples to insure the reliability and correctness of results. The measured values of radon concentration in these samples were found in the range of 8–58 Bq L−1 with a mean of 31 Bq L−1 and from 18 to 61 Bq L−1 with a mean of 35 Bq L−1 using RnDuo and RAD7, respectively. The mean radon levels measured with RnDuo and RAD7 were found comparable with each other. A linear regression with its equation for measured values of radon levels using both methods is shown in the Fig. 5. A positive correlation (Slope = 0.98 and R2 = 0.80) was observed between radon levels measured with RnDuo and RAD7 monitors. It can, therefore, be inferred that both the techniques are well comparable with one another for radon measurements in water.
Estimation of radiation doses due to radon in water
The radon (in water) enters human body via ingestion of drinking water and via inhalation of radon released from water to indoor air. In this way, stomach and lungs both are exposed to radon in water.
Assessment of age-dependent ingestion dose
The ingestion dose from the exposure to radon in drinking water for individuals of various age categories was calculated using equation (UNSCEAR 1993, Annex A, Section III, Paragraph 133, Page No. 54):
where DIng is annual effective dose due to ingestion of radon in drinking water (mSv year−1), Cw is radon concentration in water (Bq L−1), DWI is daily water consumption, DCF is dose conversion factor (10−8 Sv Bq−1) and t is exposure time (365 days year−1). The numerical values of DWI for the people of different age groups such as infants, children, males, females, pregnancy and lactation are given in Table 4 (NRC 1999). The estimated annual ingestion dose for the people of different age groups is shown in Table 4. The mean values ingestion doses from the exposure to radon in groundwater are greater than the reference value of 100 µSv year−1 (from all sources including radon) proposed by World Health Organization (WHO 2004).
Assessment of inhalation dose
The radiation dose attributed to inhalation of 222Rn which has escaped from household water to indoor air was assessed using UNSCEAR’s relation (UNSCEAR 2000, Annex B, Section III, Paragraph 157, Page No. 108):
where DInh is the annual effective dose due to inhalation of 222Rn released from the household water, ARn is the activity level (Bq m−3) of 222Rn in water, \( \frac{{R_{\text{a}} }}{{R_{\text{w}} }} = 10^{ - 4} \), Ra and Rw being the concentrations of 222Rn in air and water, respectively. EF is the equilibrium factor for 222Rn (0.42) for Garhwal Himalaya (Prasad et al. 2016), tI is the time an individual spends inside the house (0.82) for Garhwal Himalaya (Ramola et al. 1997) and DCF is the dose conversion factor (9 nSv Bq−1 h−1 m3). The estimated value of annual effective dose attributed to inhalation from varied 5–1085 µSv year−1 with a mean of 122 and 5–570 µSv year−1 with a mean of 100 µSv year−1 using RnDuo and RAD7, respectively.
Conclusions
It can be concluded from this study that radon levels in most groundwater sources located in Garhwal Himalaya, India, exceed the reference limit of 11 Bq L−1 established by the USEPA. However, the levels of 222Rn are mostly under the reference value of 100 Bq L−1 suggested by the European commission (EU) and the World Health Organization (WHO). Radon levels in groundwater depend on geological and geohydrological parameters of the area. The source-wise comparison shows that radon concentration in water depends on the volume surrounding the water source. The scintillation detector-based recently developed RnDuo technique is well comparable with silicon detector-based RAD7 technique for radon measurements in water. The estimated mean values of total radiation dose from the exposure to radon in drinkable groundwater are lesser than recommended reference limit of 1 mSv year−1 set by UNSCEAR and WHO. The variability of radon levels observed in different types of groundwater sources, the dependence of radon levels on geological and geohydrological parameters may be useful in exploring in uranium deposits and examining hidden faults and thrusts, etc.
References
Choubey VM, Ramola RC (1997) Correlation between geology and radon levels in groundwater, soil and indoor air in Bhilangana Valley, Garhwal Himalaya, India. Environ Geol 32:258–262
Choubey VM, Bartarya SK, Ramola RC (2000) Radon in Himalayan springs: a geohydrological control. Environ Geol 39:523–530
Duggal V, Sharma S, Mehra R (2017) Radon levels in drinking water of Fatehabad district of Haryana, India. Appl Radiat Isot 123:36–40
Durridge Radon Instrument (2013) RAD7 radon detector, user manual, 2013. Durridge Co. USA. http://www.durridge.com/documentation/RAD7%20Manual.pdf
EU (1998) European drinking water directive 98/83/EC of 3rd November 1998 on the quality of water intended for human consumption. Off J Eur Comm (European Commission)
EU (2001) Commission recommendation of 20th December 2001 on the protection of the public against exposure to radon in drinking water. Off J Eur Comm (European Commission)
Fonollosa E, Penalver A, Borrull F, Aguilar C (2016) Radon in spring waters in the south of Catalonia. J Environ Radioact 151:275–281
Gaware JJ, Sahoo BK, Sapra BK, Mayya YS (2011) Indigenous development and networking of online radon monitors in the underground uranium mine. Radiat Prot Environ 34:37
Gupta SK (1977) Geology and petrology of part of Upper Bhagirathi Valley Central crystalline, M. Tech Dissertation Report, Dist. Uttarkashi, UP
Heim A, Gansser A (1939) Central Himalaya: geological observations of the Swiss expedition 1936. Denkschriften der Schwieizerishen Naturforschenden Gasellschaft
Jobbágy V, Kávási N, Somlai J et al (2010) Radiochemical characterization of spring waters in Balaton Upland, Hungary, estimation of radiation dose to members of public. Microchem J 94:159–165
Jobbágy V, Altzitzoglou T, Malo P et al (2017) A brief overview on radon measurements in drinking water. J Environ Radioact 173:18–24
Kendall GM, Smith TJ (2002) Doses to organs and tissues from radon and its decay products. J Radiol Prot 22:389
Lawrence E, Poeter E, Wanty R (1991) Geohydrologic, geochemical, and geologic controls on the occurrence of radon in ground water near Conifer, Colorado, USA. J Hydrol 127:367–386
Moreno V, Bach J, Baixeras C, Font L (2014) Radon levels in groundwaters and natural radioactivity in soils of the volcanic region of La Garrotxa, Spain. J Environ Radioact 128:1–8
Nashine SK (1982) Uranium occurrences close to the main central thrust around Sileth, Dhargaon, Chamyala Balganga valley, Tehri Garhwal. UP Him Geol 12:305–316
NRC (1999) Risk assessment of radon in drinking water. National Research Council Report. National Academy Press, Washington, DC
Prasad Y, Prasad G, Choubey VM, Ramola RC (2009) Geohydrological control on radon availability in groundwater. Radiat Meas 44:122–126
Prasad M, Rawat M, Dangwal A et al (2016) Variability of radon and thoron equilibrium factors in indoor environment of Garhwal Himalaya. J Environ Radioact. https://doi.org/10.1016/j.jenvrad.2015.10.017
Przylibski TA, Gorecka J (2014) 222 Rn activity concentration differences in groundwaters of three Variscan granitoid massifs in the Sudetes (NE Bohemian Massif, SW Poland). J Environ Radioact 134:43–53
Ramola RC, Kandari MS, Rawat RBS (1997) Assessment of health risk due to exposure of radon and its daughter products in the lower atmosphere. Curr Sci 73:771–774
Rautela BS, Yadav M, Bourai AA et al (2012) Study of natural radionuclide and absorbed gamma dose in Ukhimath area of Garhwal Himalaya, India. Radiat Prot Dosim 152:58–61
Ravikumar P, Somashekar RK (2014) Determination of the radiation dose due to radon ingestion and inhalation. Int J Environ Sci Technol 11:493–508
Sinha AK (1989) Geology of the higher Central Himalaya. Wiley, New York
Todorovic N, Nikolov J, Forkapic S et al (2012) Public exposure to radon in drinking water in Serbia. Appl Radiat Isot 70:543–549
UNSCEAR (United Nations Scientific Committee on the Effects of Atomic Radiation) (1993) Sources and effects of ionizing radiation. Report to the General Assembly, with scientific annexes. United Nations sales publication Annex A, Section III, Paragraph 133, Page No. 54. United Nations, New York
UNSCEAR (United Nations Scientific Committee on the Effects of Atomic Radiation) (2000) Sources and effects of ionizing radiation. Report to General Assembly with Scientific annexes. United Nations sales publication, Annex B, Section III, Paragraph 157, Page No. 108. United Nations, New York
USEPA (United States Environmental Protection Agency) (1991) Federal Register 40 Parts 141 and 142: national primary drinking water regulations; radionuclides: proposed rule. US Government Printing Office, Washington, DC
USEPA (United States Environmental Protection Agency) (1999) Raadon in drinking water, factsheet. EPA815-F-99-007. http://water.epa.gov/scitech/drinkingwater/dws/radon/qal.cfm
Valdiya KS (1980) Geology of kumaun lesser Himalaya. Wadia Institute of Himalayan Geology, Dehradun
Valdiya KS, Bartarya SK (1991) Hydrogeological studies of springs in the catchment of the Gaula river, Kumaun Lesser Himalaya, India. Mt Res Dev 11:239–258
Valdiya KS, Paul SK, Chandra T et al (1999) Tectonic and lithological characterization of Himadri (Great Himalaya) between Kali and Yamuna rivers, central Himalaya. Himal Geol 20:1–17
WHO (2008) World health organization guidelines for drinking water quality. In: Incorporating first and second addenda, third ed. WHO Press, Geneva, 3rd edn. World Health Organisation, Geneva
WHO (2009) WHO handbook on indoor radon: a public health perspective. World Health Organization, Geneva
WHO (World Health Organization) (2004) Guidelines for drinking-water quality. Vol. 1. Recommendations, 3rd edn. World Health Organization, Geneva
WHO (World Health Organization) (2011) Guidelines for drinking-water quality. Vol. 1. Recommendations, 4th edn. World Health Organization, Geneva
Acknowledgements
The author (MP) is also thankful to Science and Engineering Research Board, Department of Science and Technology, Govt. of India, for providing financial assistance under the scheme of National Post Doctoral Fellowship (File no: PDF/2016/000565).
Author information
Authors and Affiliations
Corresponding author
Rights and permissions
About this article
Cite this article
Prasad, M., Kumar, G.A., Sahoo, B.K. et al. A comprehensive study of radon levels and associated radiation doses in Himalayan groundwater. Acta Geophys. 66, 1223–1231 (2018). https://doi.org/10.1007/s11600-018-0135-0
Received:
Accepted:
Published:
Issue Date:
DOI: https://doi.org/10.1007/s11600-018-0135-0