Abstract
Purpose
Soil amendment is an important method to improve soil ecology and crop quality. Field experiments of soil amendments were conducted to find a suitable solution to slow down soil acidification and to decrease the effect of heavy metal pollution in tea plantations.
Methods
A 2-year field experiment was conducted in a tea garden contamination with Pb (lead), Cd (cadmium), and soil pH below 4.5. It included five treatments with various amendments: CK (no amendment), bentonite, calcium magnesium phosphate fertilizer, biochar, and lime.
Results
Four soil amendments increased soil pH by 0.38–0.60, nutrient contents (available nitrogen, phosphorus, and potassium), as well as soil organic matter content. The contents of soil available Pb, Cd, and Cr (chromium) also decreased significantly (p < 0.05). Tea yield increased significantly by 15.13–40.89%, as tea polyphenols, free amino acids, caffeine, and water extract increased by 2.06–11.68%, 2.48–46.51%, 6.97–22.40%, and 5.46–33.76%, respectively. In addition, Pb in tea treated with biochar was decreased significantly by 61.28%. The Cd and Cr in tea treated with bentonite, calcium magnesium phosphate fertilizer, and lime decreased significantly by 15.93–41.15%, 25.27–31.87%, while As (arsenic) decreased by 9.82–18.05% (p > 0.05), respectively.
Conclusion
Four soil amendments could promote the rebalancing of soil nutrients and raise the soil pH in the acidified tea plantations. The accumulation of heavy metals in tea was reduced, and the yield of tea increased. Biochar has a better effect on improving the soil of acidified tea plantations, while its molecular mechanism of promoting tea tree growth should be focused on research.
Graphical Abstract

Similar content being viewed by others
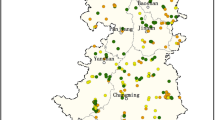
Explore related subjects
Discover the latest articles, news and stories from top researchers in related subjects.Avoid common mistakes on your manuscript.
1 Introduction
Tea (Camellia sinensis L.) is a traditional economic crop in China. In 2020, China’s tea plantation area had reached up to 3.217 million hm2, with a total output of 2.932 million tons. Its plantation area is ranked the first in the world, with a total output value of 262.66 billion yuan (Wu et al. 2020). Tea industry plays an extremely important role in China’s agricultural production and national economy. However, in recent years, soil acidification in tea plantations has been severe due to continuous crop cultivation and heavy fertilizer application, which has exacerbated soil nutrient imbalance, increased soil heavy metal content, and tea safety risk (Le et al. 2022a). Soil pH of 4.5–5.5 is favorable for tea plant growth. However, in China, the average pH in tea plantation is 4.68, whereas 46.0% of tea planting areas is lower than 4.5, seriously affecting the yield and quality of tea (Yan et al. 2020). If the soil pH is lower than 4.0, the root tip of tea plant shrinks, the growth is inhibited, and the absorption of N (nitrogen), P (phosphorus), and K (potassium) decreases sharply, affecting the yield and quality of tea (Morita et al. 2011). In addition, acidification also causes the harm to the physical and chemical properties of tea plantation soil (Le et al. 2022b). The presence of a large amount of exchangeable H+ in acidified soil will make it difficult to form a granular structure, resulting in a decrease in soil porosity (Raza et al. 2020). At the same time, with the decrease of pH, the availability of N, P, K, Ca (calcium), Mg (magnesium), S (sulfur), and other nutrients decreased, which, in turn, affect the absorption and utilization efficiency of tea plants (Yadav et al. 2020). On the other hand, acidification will reduce the adsorption capacity of soil to heavy metal ions and increase its solubility, mobility, and effectiveness (Liu et al. 2023). A previous report (Zhang and Fang 2007) found that the decrease of soil pH increased the contents of Cd, Cr, Cu (cuprum), Fe (ferrum), Ni (nickel), Mn (manganese), Pb, and Zn (zinc) in tea, the effect of which was more significant when the soil pH was lower than 4.4. Zhang et al. (2021) analyzed the spatial heterogeneity of soil pH and Cd in tea gardens in the mountainous area of Guizhou Plateau in China. They found that when the soil pH was ≤ 5.5, the effect on Cd content was the greatest. It has been reported that more than 75% of soil in Anhui tea plantations of China have excessive Cd, Hg (hydrargyrum), Pb, and Zn, the Cd of which are the highest carcinogenic risk for tea security (Tao et al. 2021), because acidification will increase the bioavailability of heavy metals in soil, while heavy metals in tea mainly come from soil (Zhang et al. 2018). Thus, we urgently need to find suitable solutions to alleviate soil acidification and repair heavy metal pollution in tea plantations.
Adding soil amendments can alleviate soil acidification and decrease the bioavailability of heavy metals. At present, the commonly used amendments are calcium, mineral soil amendments, and organic amendments such as biochar. Lime and calcium magnesium phosphate fertilizer as calcium-containing materials can increase soil pH and provide a large amount of hydroxyl ion OH− to have the effect of co-precipitation with Cd2+, reducing the availability of Cd in soil and the absorption of Cd by crops (Wu et al. 2016). The mineral amendments represented by bentonite can stabilize Cu, Zn, and Cd through cation exchange (Yu et al. 2017). In recent years, biochar as a new type of soil amendment has attracted the attention of global scholars. Previous studies have reported that the application of straw biochar can well fix soil Cd and Pb (Shen et al. 2016), and the application of biochar and compost together will reduce the availability of Cd and Zn (Tang et al. 2020). Yet the repeated application of biochar will not increase the concentration of heavy metals in soil (Lucchini et al. 2014), and biochar showed a good effect on the remediation of soil organic pollution. Liang et al. (2020) showed that the application of biochar/compost amendment in sulfamethoxazole-contaminated wetland soil could increase the activity of soil dehydrogenase and urease and improve the degradation efficiency of pollutants. At the same time, the adsorption amount of sulfamethoxazole was positively correlated with the amount of modifier (Tang et al. 2021). There are many kinds of soil amendments, but their effects in tea plantation are still limited. Thus, we need to find suitable amendments to alleviate soil acidification and repair heavy metal pollution in tea plantations.
In this study, a 2-year field experiment was conducted with commonly used amendments (biochar, lime, calcium magnesium phosphate fertilizer, and bentonite) in a tea plantation with soil pH of 4.28 and Pb and Cd pollution. We aimed to assess the effects of the application of amendments on the physical and chemical properties of soil, the yield and quality of tea, and the contents of Pb, Cd, Cr, and As in soil and tea, providing scientific basis and references for the application of amendments in acidified tea plantations. We proposed the following hypothesis: (i) the application of amendments could improve tea yield and quality; (ii) the amendments increase soil pH to promote the growth of tea plants; and (iii) the amendments reduce the content of heavy metals in soil and tea.
2 Materials and methods
2.1 Experiment area and soil properties
The experimental site is located in a tea plantation in Beilun District, Ningbo City, Zhejiang Province, China (121° 52′ 51.59′′ E, 29° 48′ 16.37′′ N). The experiment area is a subtropical monsoon climate, facing the East China Sea. The climate is mild and humid with the annual average temperature of 16.5 ℃ and the annual average rainfall of 1316.8 mm. Its four seasons are distinct, and the frost-free period is long. Tea variety is ‘Longjing 4’, the plant age of which was 6 years with double row planting, line spacing 1.5 m, and management methods for hand picking tea. The soil type was Inceptisols, the physical–chemical properties of which were soil pH of 4.28, available N of 150 mg/kg, available P of 81.26 mg/kg, available K of 97.45 mg/kg, organic matter of 32.17 g/kg, available Pb of 20.45 mg/kg, available Cd of 0.07 mg/kg, available Cr of 0.41 mg/kg, and available As of 0.06 mg/kg. The experiment site was selected for the reason that total soil Pb and Cd levels of 150 mg/kg and 0.53 mg/kg, respectively. It exceeded the Chinese Soil Environmental Quality Risk Control Standard for Soil Contamination of Agricultural Land (GB 15618–2018) (Cd < 0.3 mg/kg and Pb < 70 mg/kg). Meanwhile, the Pb content of some tea samples in the area exceeded the Chinese national food safety standard (GB 2762–2017) (Pb < 5 mg/kg).
2.2 Experiment material
We selected the biochar, calcium magnesium phosphate fertilizer, bentonite, and lime as experiment amendments. Biochar was purchased from Biochar Environmental Material Co., Ltd. (Pingdingshan, Henan, China). The raw material was rice straw, which was prepared by pyrolysis at 550 ℃ for 2 h. Its basic properties were as follows: pH of 9.84, C of 54.21%, H of 2.34%, N of 0.95%, P of 0.24%, S of 0.41%, and organic carbon of 364 g/kg. Calcium magnesium phosphate fertilizer was purchased from Gaoyuan Phosphate Fertilizer Co., Ltd. (Jingmen, Hubei, China), and its basic properties were pH of 9.46, P2O5 of 13.41%, CaO of 26.31%, MgO of 3.26%, and SiO2 of 11.43%. Bentonite was purchased from Zhongsen Perlite Application Co., Ltd. (Xinyang, Henan, China), and its basic properties were pH of 9.61, SiO2 of 69.32%, Al2O3 of 14.27%, MgO of 2.69%, and K2O of 1.04%. Lime was purchased from Shunfa Lime Production Co., Ltd. (Wuhu, Anhui, China). The basic properties were pH of 10.24, SiO2 of 8.65%, Al2O3 of 2.5%, CaO of 55.64%, and MgO of 2.14%. The heavy metal contents in the experiment materials (Table 1) were all lower than the “Requirements for the Limits of Toxic and Hazardous Substances in Fertilizers” (GB 38400–2019) in China.
2.3 Experimental design
There were 5 treatments: no soil amendment (CK), bentonite (P1), calcium magnesium phosphate fertilizer (P2), biochar (P3), and lime (P4). A randomized block design was used in the experiment. Each treatment was repeated three times. The plot area was 60 m2 (30 m in length and 2 m in width), and its protective rows with an interval of 1.5 m were settled in different plots. The application of soil amendments was carried out in November 2019 and 2020. The dosage of each treatment is shown in Table 2, and the amount of material was determined from our previous studies. The amount of tea chemical fertilizer was 400 N kg/hm2, 160 P2O5 kg /hm2, and 420 K2O kg/hm2, respectively. In November (before spring tea), 70% of N fertilizer and 100% of P and K fertilizer were applied. In February of the second year, 30% of N fertilizer was applied. The fertilizer was applied in strips along tea plant row in the plot, from the rhizosphere of the tea plant to the 2/3 edge of the canopy (about 0.3 m away from the tea plant), while a 20-cm-wide and 15-cm-deep fertilization ditch was opened. Other field management measures (weeding, watering, spraying pesticides, pruning, etc.) were consistent with the local practices.
2.4 Sampling
In April 2022, sampling was conducted during the spring tea picking season. The picking standard was one bud and two leaves of fresh tea. Immediately after picking, the harvested material was taken back to the laboratory and placed in a preheated oven at 105 ℃ for 10–15 min. After drying at 80 ℃, the dry samples were stored in a refrigerator (4 ℃) until tested.
The surface soil (0–20 cm) was collected from the root system of tea plant (10 cm) using wood shovel with 5-point sampling method. After removing debris, all samples (1 kg) were fully mixed, each sample mass of which was naturally dried and then grinded through 2 mm and 0.149 mm sieves to determine soil physical and chemical properties and soil heavy metals.
2.5 Determination of soil physical and chemical properties
Soil pH was measured using a pH meter (Orion 3 Star, Thermo Ltd., Waltham MA, USA) (soil:water = 1 g:2.5 mL). Available N was determined by alkaline hydrolysis diffusion method (Kwon et al. 2009). Soil available P was extracted with HCl-NH4F and then determined by molybdenum blue method (Bray and Kurtz 1945). Available K was analyzed by with ammonium acetate-flame photometer method (Leaf 1958), while organic carbon was determined by potassium dichromate oxidation capacity method-external heating method and soil organic matter = soil organic carbon × 1.724 (Schollenberger 1945). Total Pb and Cd in soil were extracted by HNO3-HF-HClO4 method and available Cd, Pb, and Cr in soil were extracted with 0.1 M HCl (soil/liquid = 1 g:5 mL, extracted for 2 h) and determined by atomic absorption spectrophotometry (AA-7000, Shimadzu, Japan), and available As was determined by atomic fluorescence spectrophotometry using 0.05 M NH4H2PO4 extraction (soil/solution = 1 g:25 mL, extraction 16 h) (AFS-230E, Haiguang, Beijing, China) (Zeng et al. 2011). The analytical quality control was carried out with Chinese national standard substance GBW07442 (GSF-2), and the blank samples and parallel samples were determined. The results showed that the contents of Cd, Pb, Cr and As met the allowable error values.
2.6 Determination of tea yield, quality, and heavy metals
We followed the previous method (Yang et al. 2021) for budding density by 0.1 m2 sample frame 5-point sampling survey method (33 cm × 33 cm), from top to bottom picking box one bud and two leaves, while each plot repeated 6 times. For determination of 100-bud weight (fresh tea) by picking sufficient new shoots (one bud and two leaves) in each plot, 100 new shoots were randomly and quickly selected and weighed, being repeated 6 times (Yang et al. 2021). Tea yield was measured by recording the yield of the whole plot (excluding the protection line), and the yield per hectare (kg/hm2) was calculated by conversion (Yang et al. 2021). Tea polyphenols were determined by Folin-Ciocalteu colorimetric method; total free amino acids were determined by ninhydrin colorimetric method; water extract content was determined by water bath extraction drying method; and caffeine content was determined by ultraviolet spectrophotometry (Du et al. 2012). Determination of heavy metals in tea was followed by HNO3-H2O2 microwave digestion (ETHOS UP, Milestone, Sorisole, Italy). Pb, Cr, Cd, and As were determined by inductively coupled plasma mass spectrometry (iCAP-Q ICP-MS, Thermo Scientific, Waltham, MA, USA) (Rashid et al. 2016).
2.7 Statistical analysis
Non-parametric statistics and one-way analysis of variance (one-way ANOVA) were performed using IBM SPSS Statistics 23 software. The Duncan method was used to make multiple comparisons within the experimental data. The significance level for the differences was p < 0.05. The figures production was used by Origin 2021. The test data are expressed as mean ± standard deviation (SD).
3 Results
3.1 Soil physical and chemical properties
Two years of the field trial proved that all four soil amendments raised soil pH compared to the CK treatment (Table 3). Among them, P1 treatment had the most obvious improvement effect, increasing by 0.60 units (p < 0.05), while P2, P3, and P4 treatments increased by 0.42, 0.38, and 0.48 units, respectively. Compared with CK treatment, the soil available N content of the treatments increased by 4.56–21.13 mg/kg, of which P1, P2, P3, and P4 treatments increased by 10.47%, 12.32%, 9.47%, and 2.66%, respectively (p > 0.05). The content of soil available K in P1 treatment was 130.66 mg/kg, which was the highest among all treatments. Compared with CK treatment, it increased by 20.61% (p < 0.05). Soil organic matter content of four amendments treatment was 32.30–38.57 g/kg and increased by 2.28–22.13% (p > 0.05) compared with CK treatment, while this increase was the most in P3 treatment with the content of 6.99 g/kg.
3.2 Soil available heavy metals
Figure 1a shows that the application of amendments significantly reduced the content of soil available Pb. The lowest content of available Pb was 8.75 mg/kg in P2 treatment, which decreased by 57.07% compared with CK treatment. P1, P3, and P4 treatments significantly decreased by 44.06%, 30.42%, and 23.95%, respectively (p < 0.05). The content of available Cd in soils decreased significantly by 73.03–84.18% (p < 0.05), with the lowest level of 0.01 mg/kg in the P1 treatment (Fig. 1b). P2, P3, and P4 amendment decreased significantly by 73.22%, 73.03%, and 74.08%, respectively. The available Cr content in the soil was significantly reduced as seen in Fig. 1c, compared to the CK treatment and P4 treatment was the lowest (0.078 mg/kg) with a decrease of 80.80% (p < 0.05), yet P1 and P2 treatments were similar, which decreased by 66.04% and 67.51%, respectively (p < 0.05), while the soil available As content of P3 treatment was the lowest, which decreased by 80.65% (p < 0.05) (Fig. 1d).
3.3 Tea yield
It can be seen from Table 4 that compared with CK treatment, the 100-bud weight of tea treated with amendments significantly increased by 26.35–51.38%, and the maximum of P3 treatment was 27.40 g. The tea budding density of P3 treatment was the highest (238.67 ind./m2), which increased significantly by 26.95% (p < 0.05). The tea budding density of P1, P2, and P3 treatments increased significantly by 12.41%, 24.11%, and 26.95%, respectively (p < 0.05). The fresh leaf yield of P3 treatment was the highest with 591.87 kg/hm2, which was significantly increased by 40.89% compared with CK treatment (p < 0.05). P1, P2, and P4 treatments significantly increased by 15.13%, 29.92%, and 31.98%, respectively (p < 0.05).
3.4 Tea quality
In Table 5, the tea polyphenol content of P3 treatment was the highest, which was 197.63 mg/g, followed by P2 treatment with 93.59 mg/g. There was no significant difference in tea polyphenol content between all amendments and CK treatment (p > 0.05). Compared with CK treatment, the content of free amino acids in P3 and P4 treatments increased significantly to 47.91 mg/g and 38.25 mg/g (p < 0.05), respectively. The ratio between tea polyphenols and free amino acids in P3 was the smallest with 4.13, while it was the largest with 5.52 in the P2 treatment. The caffeine content of P2 and P3 treatments was similar, which was significantly increased by 22.40% and 20.74% compared with CK treatment (p < 0.05), respectively. Compared with CK treatment, the water extract content of the four amendments treatments increased by 20.42–126.16 mg/g, while the highest water extract content in P3 treatment was 499.90 mg/g.
3.5 Tea heavy metals
Figure 2a shows that the Pb content in tea treated with either of amendments (P1, P2, P3, and P4) decreased significantly (p < 0.05) compared with CK treatment, with a decrease of 52.31–61.28%. Among them, P3 treatment had the largest decrease with 1.78 mg/kg, and P1, P2, and P4 treatments decreased by 52.31%, 53.31%, and 57.00%, respectively. In Fig. 2b, tea Cd content with P1, P3 and P4 was similar. Compared with CK treatment, tea Cd content decreased significantly (p < 0.05), with a decrease by 38.37%, 37.01%, and 41.45%, respectively. In Fig. 2c, compared with CK treatment, the Cr content in tea of P1 treatment decreased by 31.87%; P2, P3, and P4 treatments decreased significantly by 26.37%, 28.57%, and 25.27% respectively (p < 0.05). Figure 2d shows that the As content in tea of each treatment was not significantly different (p > 0.05), and the content of P4 treatment was the lowest, which decreased by 18.05% compared with CK treatment.
4 Discussion
Soil pH decreases severely with increasing years of cultivation tea plant (Chen et al. 2021). Our results showed that after 2 years of amendment application, the soil pH increased and promoted tea plant growth (4.60–4.82), compared with to 4.28 prior to the experiment. Since four amendments are alkaline substances, they can increase soil pH. Among them, biochar, calcium magnesium phosphate fertilizer, bentonite, and lime, as common soil amendments, have been widely used in paddy fields and have proved to have a beneficial effect on increasing soil pH (Lwin et al. 2018). Compared with CK, the application of amendments also increased soil organic matter content. The reason is mainly because of its special cultivation mode of pruning; the pruned tea branches, leaves, and other substances are used to cover the surface of the soil (Liu et al. 2022). These plant residues are decomposed into organic matter into soil under the decomposition of microorganisms (Bora et al. 2022). Most bacteria and actinomycetes related to the decomposition of plant residues had the most suitable pH of 6.5–7.5 (Krishna and Mohan 2017). The application of amendments increased the pH of acidified soil, which would accelerate the decomposition of plant residues (Wu et al. 2021). The results showed that the soil organic matter content of biochar was the highest among the four amendments. The reason for this result is mainly due to the protection matrix generated by the passivation of the aromatic structure on the surface of biochar in acidic soil, which improves the stability of organic matter (Joseph et al. 2020). At the same time, biochar can also improve the activity of soil microorganisms and promote the decomposition of soil humus and ultimately increase the content of soil organic matter (Weng et al. 2022). These wastes can be decomposed under the action of microorganisms, whereas biochar with the alkaline carbon substances accelerates the decomposition of litter (Minamino et al. 2019), improving the soil organic matter content. Our results showed that the application of calcium magnesium phosphate fertilizer, bentonite, and biochar amendment also increased soil nutrient content. Calcium magnesium phosphate fertilizer is a common P fertilizer; the content of P2O5 in the tested materials is 13.41%, so its application can increase the content of available P in soil. Most tea plants grow in warm and rainy subtropical regions, where soil N is easily leached. However, adding biochar can increase soil N retention and also improve its availability (Zheng et al. 2013). Since, montmorillonite, the main component of bentonite, has a crystal structure in which high-valent Si (silicon) and Al (aluminum) ions can be replaced unequally by other low-valent cations; K+ is adsorbed in the interlayer structure in the form of electrostatic attraction; and this exchangeable state increases the amount of available K in the soil (Mi et al. 2021).
The contents of Pb and Cd in the soil of our experiment site exceeded the relevant standards of China. The contents of available Pb, Cd, and Cr in the soil decreased significantly after the application of amendments (Fig. 1). Previous reported that the addition of the bentonite, biochar, and chitosan (all three at 1%) has been shown to reduce available Cd by 22–36% and available Pb by 6–87% in soil, it being consistent with our results (Shaheen and Rinklebe 2015). Lime and calcium magnesium phosphate fertilizer, as calcium-containing soil amendments, can increase soil pH, promote the formation of carbonate and hydroxide precipitation of Cd, and reduce its bioavailability to inhibit the absorption of Cd by plants (Sharma and Nagpal 2018). Since the main component of bentonite is montmorillonite, previous reports have indicated that montmorillonite may release some dissolved Si to precipitate Cd, forming stable Cd particles, thereby reducing its availability. Biochar can adsorb heavy metals because of its rich pore space (Chen et al. 2022). In addition, it is also capable of ion exchange or redox reactions with heavy metal ions to produce stable heavy metal precipitates (Duan et al. 2019) or convert toxic elements in the high valence state to the low valence state (Zama et al. 2018). It was shown that biochar in sheep and earthworm manure reduces Pb activity in soil by increasing Pb content in the residual state (Boostani et al. 2019), which was consistent with our experimental results.
Tea yield and quality are important indicators of the economic value of tea. The application of soil amendments significantly increased the weight of tea buds, budding density, and fresh leaf yield (Table 4). Biochar treatment has the most obvious effect on the increase of tea yield, for the reason that its more developed pore structure, which can adsorb N, P, K, and other nutrients, reducing the leaching and fixed loss of soil nutrients, improving the adsorption capacity of soil to mineral nutrients, and prolonging the release time of mineral nutrients (Kim et al. 2014). On the other hand, plants can absorb P from biochar carried into the soil and increase crop yields (Farrell et al. 2014). Kätterer et al. (2019) found that soil fertility and yield increased for 10 consecutive years in maize-soybean rotation after just one application of biochar in silty and clay soil. Uzoma et al. (2011) also found that the yield of maize in sandy soils increased by 150% and 98% (p < 0.05) when the amount of biochar was 15 and 20 tons/hm2, respectively, in agreement with our results. Biochar increased the content of tea polyphenols, free amino acids, and aqueous extracts in tea leaves, and the ratio of phenols to ammonia was reduced, which closely related to the aroma and taste of tea soup. N is an important component of substances such as amino acids and caffeine in tea (Bittner 2006). The increase of soil P and K content is beneficial to increase tea polyphenols and water extracts (Tang et al. 2023). This study shows that the application of biochar can increase the content of available N, P, and K in the soil, thereby improving the quality of tea. This is mainly due to that biochar reduces the leaching of mineral nutrients by enhancing the nutrient adsorption, and the rebalancing of soil nutrients improved the nutrient utilization efficiency of tea in the later stages (Ding et al. 2016).
Obviously, with the acidification of tea plantation soil, the safety risk of tea gradually increases as the soil heavy metal activity increases, increasing the risk of human consumption. Our study showed that the application of biochar, lime, and bentonite can significantly reduce the content of Pb, Cd, and Cr in tea. Because the negative charge on the surface of biochar combines with heavy metal cations, its application reduce the activity of heavy metals in the soil, and as a result, the accumulation of heavy metals in tea is reduced (Mohamed et al. 2017). It has been shown that biochar produced at 700 ℃ can reduce the available state of Pb by 88.1% (Ahmad et al. 2016). Lime reduces the heavy metals in tea, which is mainly due to the increase of soil pH, which increases the amount of negative charge on the surface of colloids, improves the adsorption capacity of soil for heavy metal ions, and facilitates the formation of precipitates such as carbonates (Guo et al. 2006). However, long-term use of lime decreases soil pH and increases the concentration of heavy metal ions in the soil (Goulding 2016). As a clay mineral, bentonite has a relatively large specific surface area. It mainly reduces the concentration and activity of heavy metal ions in soil solution by adsorption, coprecipitation, coordination, and ion exchange, thus reducing the content of heavy metals in plants (Sun et al. 2015). However, effectiveness of bentonite on the treatment of heavy metals in soil depends on factors, such as the type of contaminated soil, remediation of soil environment, the degree of complex pollution, and the type of clay mineral. Yet bentonite is more often used in wastewater treatment projects. In comparison, biochar is increasingly used as an effective material for reducing heavy metals in tea and has a positive impact on the physical and chemical properties of the soil.
5 Conclusions
Our results showed that four soil amendments (biochar, lime, calcium-magnesium phosphate fertilizer, and bentonite) could promote the rebalancing of soil nutrients and raise the soil pH in acidified tea plantations. As the effective state of soil heavy metals was limited, the accumulation in tea was reduced, and the quality of tea was ensured. The yield of tea also increased which greatly improved the economic value of tea. Biochar has a better effect on improving the soil of acidified tea plantations. Further efforts need to be made to reveal the molecular mechanism of biochar for promoting tea tree growth.
Data availability
The data underlying this article will be shared on reasonable request to the corresponding author.
References
Ahmad M, Ok YS, Kim BY, Ahn JH, Lee YH, Zhang M, Moon DH, Al-Wabel MI, Lee SS (2016) Impact of soybean stover- and pine needle-derived biochars on Pb and As mobility, microbial community, and carbon stability in a contaminated agricultural soil. J Environ Manage 166:131–139. https://doi.org/10.1016/j.jenvman.2015.10.006
Bittner S (2006) When quinones meet amino acids: chemical, physical and biological consequences. Amino Acids 30:205–224. https://doi.org/10.1007/s00726-005-0298-2
Boostani HR, Najafi-Ghiri M, Hardie AG, Khalili D (2019) Comparison of Pb stabilization in a contaminated calcareous soil by application of vermicompost and sheep manure and their biochars produced at two temperatures. Appl Geochemistry 102:121–128. https://doi.org/10.1016/j.apgeochem.2019.01.013
Bora SS, Hazarika DJ, Gogoi R, Dullah S, Gogoi M, Barooah M (2022) Long-term pruning modulates microbial community structure and their functional potential in Tea (Camellia sinensis L.) soils. Appl Soil Ecol 176:104483. https://doi.org/10.1016/j.apsoil.2022.104483
Bray RH, Kurtz LT (1945) Determination of total, organic, and available forms of phosphorus in soils. Soil Sci 59
Chen DD, Liu WH, Wang YX, Lu P (2022) Effect of biochar aging on the adsorption and stabilization of Pb in soil. J Soils Sediments 22:56–66. https://doi.org/10.1007/s11368-021-03059-x
Chen PF, Liu YZ, Mo CY, Jiang ZH, Yang JP, Lin JD (2021) Microbial mechanism of biochar addition on nitrogen leaching and retention in tea soils from different plantation ages. Sci Total Environ 757:143817. https://doi.org/10.1016/j.scitotenv.2020.143817
Ding Y, Liu YG, Liu SB, Li ZW, Tan XF, Huang XX, Zeng GM, Zhou L, Zheng BH (2016) Biochar to improve soil fertility. A Review Agron Sustain Dev 36:36. https://doi.org/10.1007/s13593-016-0372-z
Du WH, Peng SM, Liu ZH, Shi L, Tan LF, Zou XQ (2012) Hypoglycemic effect of the water extract of pu-erh tea. J Agric Food Chem 60:10126–10132. https://doi.org/10.1021/jf302426w
Duan Y, Shen J, Zhang X, Wen B, Ma Y, Wang Y, Fang W, Zhu X (2019) Effects of soybean–tea intercropping on soil-available nutrients and tea quality. Acta Physiol Plant 41:140. https://doi.org/10.1007/s11738-019-2932-8
Farrell M, Macdonald LM, Butler G, Chirino-Valle I, Condron LM (2014) Biochar and fertiliser applications influence phosphorus fractionation and wheat yield. Biol Fertil Soils 50:169–178. https://doi.org/10.1007/s00374-013-0845-z
Goulding KWT (2016) Soil acidification and the importance of liming agricultural soils with particular reference to the United Kingdom. Soil Use Manag 32:390–399. https://doi.org/10.1111/sum.12270
Guo GL, Zhou QX, Ma LQ (2006) Availability and assessment of fixing additives for the in situ remediation of heavy metal contaminated soils: a review. Environ Monit Assess 116:513–528
Joseph S, Pow D, Dawson K, Rust J, Munroe P, Taherymoosavi S, Mitchell DRG, Robb S, Solaiman ZM (2020) Biochar increases soil organic carbon, avocado yields and economic return over 4 years of cultivation. Sci Total Environ 724:138153. https://doi.org/10.1016/j.scitotenv.2020.138153
Kätterer T, Roobroeck D, Andrén O, Kimutai G, Karltun E, Kirchmann H, Nyberg G, Vanlauwe B, Röing de Nowina K (2019) Biochar addition persistently increased soil fertility and yields in maize-soybean rotations over 10 years in sub-humid regions of Kenya. Field Crops Res 235:18–26. https://doi.org/10.1016/j.fcr.2019.02.015
Kim P, Hensley D, Labbé N (2014) Nutrient release from switchgrass-derived biochar pellets embedded with fertilizers. Geoderma 232–234:341–351. https://doi.org/10.1016/j.geoderma.2014.05.017
Krishna MP, Mohan M (2017) Litter decomposition in forest ecosystems: a review. Energy Ecol Environ 2:236–249. https://doi.org/10.1007/s40974-017-0064-9
Kwon HY, Hudson RJM, Mulvaney RL (2009) Characterization of the organic nitrogen fraction determined by the Illinois Soil Nitrogen Test. Soil Sci Soc Am J 73:1033–1043. https://doi.org/10.2136/sssaj2008.0233
Le VS, Herrmann L, Hudek L, Nguyen TB, Bräu L, Lesueur D (2022a) How application of agricultural waste can enhance soil health in soils acidified by tea cultivation: a review. Environ Chem Lett 20:813–839. https://doi.org/10.1007/s10311-021-01313-9
Le VS, Laetitia H, Lee H, Binh NT, Lambert B, Didier L (2022b) How application of agricultural waste can enhance soil health in soils acidified by tea cultivation: a review. Environ Chem Lett 20. https://doi.org/10.1007/s10311-021-01313-9
Leaf AL (1958) Determination of available potassium in soils of forest plantations. Soil Sci Soc Am J 22:458–459. https://doi.org/10.2136/sssaj1958.03615995002200050024x
Liang J, Tang SQ, Gong JL, Zeng GM, Tang WW, Song B, Zhang P, Yang ZX, Luo Y (2020) Responses of enzymatic activity and microbial communities to biochar/compost amendment in sulfamethoxazole polluted wetland soil. J Hazard Mater 385:121533. https://doi.org/10.1016/j.jhazmat.2019.121533
Liu SQ, Yang R, Peng XD, Hou CL, Ma JB, Guo JR (2022) Contributions of plant litter decomposition to soil nutrients in ecological tea gardens. Agriculture
Liu WB, Cui SY, Ma JW, Wu DT, Ye ZQ, Liu D (2023) Effects of shellfish and organic fertilizer amendments on soil nutrients and tea yield and quality. 11:262. https://doi.org/10.3390/toxics11030262
Lucchini P, Quilliam RS, DeLuca TH, Vamerali T, Jones DL (2014) Does biochar application alter heavy metal dynamics in agricultural soil? Agric Ecosyst Environ 184:149–157. https://doi.org/10.1016/j.agee.2013.11.018
Lwin C, Seo B, Kim H, Owens G, Kim K (2018) Application of soil amendments to contaminated soils for heavy metal immobilization and improved soil quality—a critical review. Soil Sci Plant Nutr 64:156–167. https://doi.org/10.1080/00380768.2018.1440938
Mi JZ, Gregorich EG, Xu ST, McLaughlin NB, Ma B, Liu JH (2021) Changes in soil biochemical properties following application of bentonite as a soil amendment. Eur J Soil Biol 102:103251. https://doi.org/10.1016/j.ejsobi.2020.103251
Minamino Y, Fujitake N, Suzuki T, Yoshitake S, Koizumi H, Tomotsune M (2019) Effect of biochar addition on leaf-litter decomposition at soil surface during three years in a warm-temperate secondary deciduous forest. Japan Sci Rep 9:16961. https://doi.org/10.1038/s41598-019-53615-2
Mohamed BA, Ellis N, Kim CS, Bi XT (2017) The role of tailored biochar in increasing plant growth, and reducing bioavailability, phytotoxicity, and uptake of heavy metals in contaminated soil. Environ Pollut 230:329–338. https://doi.org/10.1016/j.envpol.2017.06.075
Morita A, Yanagisawa O, Maeda S, Takatsu S, Ikka T (2011) Tea plant (Camellia sinensis L.) roots secrete oxalic acid and caffeine into medium containing aluminum. Soil Sci Plant Nutr 57:796–802. https://doi.org/10.1080/00380768.2011.629176
Rashid MH, Fardous Z, Chowdhury MAZ, Alam MK, Bari ML, Moniruzzaman M, Gan SH (2016) Determination of heavy metals in the soils of tea plantations and in fresh and processed tea leaves: an evaluation of six digestion methods. Chem Cent J 10:7. https://doi.org/10.1186/s13065-016-0154-3
Raza S, Miao N, Wang PZ, Ju XT, Chen ZJ, Zhou JB, Kuzyakov Y (2020) Dramatic loss of inorganic carbon by nitrogen-induced soil acidification in Chinese croplands. Glob Chang Biol 26:3738–3751. https://doi.org/10.1111/gcb.15101
Schollenberger CJ (1945) Determination of soil organic matter. Soil Sci 59
Shaheen SM, Rinklebe J (2015) Impact of emerging and low cost alternative amendments on the (im)mobilization and phytoavailability of Cd and Pb in a contaminated floodplain soil. Ecol Eng 74:319–326. https://doi.org/10.1016/j.ecoleng.2014.10.024
Sharma A, Nagpal AK (2018) Soil amendments: a tool to reduce heavy metal uptake in crops for production of safe food. Rev Environ Sci Biotechnol 17:187–203. https://doi.org/10.1007/s11157-017-9451-0
Shen X, Huang DY, Ren XF, Zhu HH, Wang S, Xu C, He YB, Luo ZC, Zhu QH (2016) Phytoavailability of Cd and Pb in crop straw biochar-amended soil is related to the heavy metal content of both biochar and soil. J Environ Manage 168:245–251. https://doi.org/10.1016/j.jenvman.2015.12.019
Sun YB, Li Y, Xu YM, Liang XF, Wang L (2015) In situ stabilization remediation of cadmium (Cd) and lead (Pb) co-contaminated paddy soil using bentonite. Appl Clay Sci 105–106:200–206. https://doi.org/10.1016/j.clay.2014.12.031
Tang JY, Zhang LH, Zhang JC, Ren LH, Zhou YY, Zheng YY, Luo L, Yang Y, Huang HL, Chen AW (2020) Physicochemical features, metal availability and enzyme activity in heavy metal-polluted soil remediated by biochar and compost. Sci Total Environ 701:134751. https://doi.org/10.1016/j.scitotenv.2019.134751
Tang S, Zhou JJ, Pan WK, Sun T, Liu MJ, Tang R, Li ZJ, Ma QX, Wu LH (2023) Effects of combined application of nitrogen, phosphorus, and potassium fertilizers on tea (Camellia sinensis) growth and fungal community. Appl Soil Ecol 181:104661. https://doi.org/10.1016/j.apsoil.2022.104661
Tang SQ, Liang J, Gong JL, Song B, Yang ZX, Fang SY, Zhang P, Cao WC, Li J, Luo Y (2021) The effects of biochar/compost for adsorption behaviors of sulfamethoxazole in amended wetland soil. Environ Sci Pollut Res 28:49289–49301. https://doi.org/10.1007/s11356-021-13959-7
Tao CJ, Song YX, Chen Z, Zhao W, Ji JF, Shen NP, Ayoko GA, Frost RL (2021) Geological load and health risk of heavy metals uptake by tea from soil: what are the significant influencing factors? Catena 204:105419. https://doi.org/10.1016/j.catena.2021.105419
Uzoma KC, Inoue M, Andry H, Fujimaki H, Zahoor A, Nishihara E (2011) Effect of cow manure biochar on maize productivity under sandy soil condition. Soil Use Manag 27:205–212. https://doi.org/10.1111/j.1475-2743.2011.00340.x
Weng Z et al (2022) Microspectroscopic visualization of how biochar lifts the soil organic carbon ceiling. Nat Commun 13:5177. https://doi.org/10.1038/s41467-022-32819-7
Wu LP, Zheng HN, Wang XJ (2021) Effects of soil amendments on fractions and stability of soil organic matter in saline-alkaline paddy. J Environ Manage 294:112993. https://doi.org/10.1016/j.jenvman.2021.112993
Wu QY, Sun CF, Yang JF (2020) Economic analysis of the change of tea production layout in China. J Phys Conf Ser 1629:012048. https://doi.org/10.1088/1742-6596/1629/1/012048
Wu YJ, Zhou H, Zou ZJ, Zhu W, Yang WT, Peng PQ, Zeng M, Liao BH (2016) A three-year in-situ study on the persistence of a combined amendment (limestone+sepiolite) for remedying paddy soil polluted with heavy metals. Ecotoxicol Environ Saf 130:163–170. https://doi.org/10.1016/j.ecoenv.2016.04.018
Yadav DS, Jaiswal B, Gautam M, Agrawal M (2020) Soil acidification and its impact on plants. In: Singh P, Singh SK, Prasad SM (eds) Plant responses to soil pollution. Springer Singapore, Singapore, pp 1–26
Yan P, Wu LQ, Wang DH, Fu JY, Shen C, Li X, Zhang LP, Zhang L, Fan LC, Wenyan H (2020) Soil acidification in Chinese tea plantations. Sci Total Environ 715:136963. https://doi.org/10.1016/j.scitotenv.2020.136963
Yang WH, Li CJ, Wang SS, Zhou BQ, Mao YL, Rensing C, Xing S (2021) Influence of biochar and biochar-based fertilizer on yield, quality of tea and microbial community in an acid tea orchard soil. Appl Soil Ecol 166:104005. https://doi.org/10.1016/j.apsoil.2021.104005
Yu K, Xu J, Jiang XH, Liu C, McCall W, Lu JL (2017) Stabilization of heavy metals in soil using two organo-bentonites. Chemosphere 184:884–891. https://doi.org/10.1016/j.chemosphere.2017.06.040
Zama EF, Reid BJ, Arp HPH, Sun GX, Yuan HY, Zhu YG (2018) Advances in research on the use of biochar in soil for remediation: a review. J Soils Sediments 18:2433–2450. https://doi.org/10.1007/s11368-018-2000-9
Zeng FR, Ali S, Zhang HT, Ouyang YN, Qiu BY, Wu FB, Zhang GP (2011) The influence of pH and organic matter content in paddy soil on heavy metal availability and their uptake by rice plants. Environ Pollut 159:84–91. https://doi.org/10.1016/j.envpol.2010.09.019
Zhang J, Yang RD, Chen R, Peng YS, Wen XF, Gao L (2018) Accumulation of heavy metals in tea leaves and potential health risk assessment: a case study from Puan County, Guizhou Province, China, Int J Environ Res Public Health
Zhang LP, Li MH, Li X, Yan P, Zhang L, Han WY (2021) Summer pruning improves the branch growth and tea quality of tea trees (Camellia sinensis). Acta Physiol Plant 43:65. https://doi.org/10.1007/s11738-021-03226-0
Zhang MK, Fang LP (2007) Tea plantation–induced activation of soil heavy metals. Commun Soil Sci Plant Anal 38:1467–1478. https://doi.org/10.1080/00103620701378417
Zheng H, Wang ZY, Deng X, Herbert S, Xing BS (2013) Impacts of adding biochar on nitrogen retention and bioavailability in agricultural soil. Geoderma 206:32–39. https://doi.org/10.1016/j.geoderma.2013.04.018
Funding
This work was financed by the National Natural Science Foundation of China (31670617), the Key Research and Development Project of Science and Technology Department of Zhejiang Province (2022C02022), the Zhejiang High-level Talents Special Support Program (2020R52026), and Jiaxing Science and Technology Plan Project (2021AZ10009).
Author information
Authors and Affiliations
Corresponding authors
Ethics declarations
Ethical approval
This research does not involve studies with human participants and/or animals.
Conflict of interest
The authors declare no competing interests.
Additional information
Responsible editor: Zhaoliang Song
Publisher's Note
Springer Nature remains neutral with regard to jurisdictional claims in published maps and institutional affiliations.
Rights and permissions
Springer Nature or its licensor (e.g. a society or other partner) holds exclusive rights to this article under a publishing agreement with the author(s) or other rightsholder(s); author self-archiving of the accepted manuscript version of this article is solely governed by the terms of such publishing agreement and applicable law.
About this article
Cite this article
Liu, W., Tang, Y., Ma, J. et al. Effects of biochar and inorganic amendments on soil fertility, tea yield, and quality in both Pb–Cd-contaminated and acidified tea plantations. J Soils Sediments 23, 3275–3284 (2023). https://doi.org/10.1007/s11368-023-03523-w
Received:
Accepted:
Published:
Issue Date:
DOI: https://doi.org/10.1007/s11368-023-03523-w