Abstract
Purpose
Evaluate the efficiency of Populus alba clone Villafranca in the uptake and translocation of Zn from contaminated soils.
Materials and methods
The effects of 48 days of zinc treatment (Zn t ) on the growth and the photosynthetic activities of P. alba L. clone Villafranca were studied using ZnSO4 (375 ppm per unit of soil dry weight) added in sand and peat moss substrate at the beginning of the treatment (T 0) and again after 30 days (T 1) in order to reach a target Zn concentration of 375 ppm at T 0 and 750 ppm at T 1 per unit of soil dry weight.
Results and discussion
Zn uptake in the different organs was analyzed after 30 (T 1) and 48 days (T 2) from the beginning of treatment, showing the following order: root ≫ leaves ≥ woody cutting = stem. The leaf area increased by 12 % in comparison to control plants at the end of second treatment (48 days). Cutting radial growth showed a high synchronicity in the growth rate fluctuation among control and Zn t plants, but a higher increase in radial diameter of Zn t cutting was observed starting from day 38 (after 8 days of second Zn t ) reaching after 48 days 38 % higher than control plants.
Conclusions
Although our data of leaf Zn concentration were in the range usually reported as toxic for plants, Villafranca clone in Zn t substrate were unaffected in terms of net CO2 assimilation and stomatal conductance to water vapor.
Similar content being viewed by others
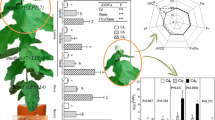
Explore related subjects
Discover the latest articles, news and stories from top researchers in related subjects.Avoid common mistakes on your manuscript.
1 Introduction
Recently, there has been an upsurge of interest on plant responses to heavy metals (HMs) because metal pollution is still increasing worldwide modifying the ecosystems’ stability (Manara 2012). Fast-growing tree species, such as those belonging to Salicaceae, have been evaluated for biomass production in heavy metal-contaminated lands (Unterbrunner et al. 2007; Guerra et al. 2011). Among Salicaceae, poplars have wide species distributions that frequently cover markedly different soil pollution ranges, making this group of species an ideal system for the study of tree adaptation to HMs. Crossing these two factors (HMs × poplars) for many years, researchers have focused on the study of the responses of poplar under high concentrations of Cu, Cd, Cr, Zn, etc., using in vitro approaches (Franchin et al. 2007), hydroponic systems, or experiments in pot/lysimeters containing soil contaminated with HMs (Di Baccio et al. 2003; Sebastiani et al. 2004, 2014; Giachetti and Sebastiani 2006; Borghi et al. 2008; Durand et al. 2010; Romeo et al. 2014a).
The range of total Zn concentrations in soils reported in the literature change around the world; in England, soils contaminated from various sources showed a mean Zn content of 97 mg kg−1 (full range 5–3548 mg kg−1) (Alloway 2009). In France, the Zn in soil starts to 17 mg kg−1 in sandy soils up to 132 mg kg−1 in very clayey soils (>50 % clay) (Baize 1997); in Australia, the range is 2–180 mg kg−1 with a mean of 34 mg kg−1 (Alloway 2009). The high concentrations of Zn found in contaminated soils may cause symptoms of toxicity like yield reduction, chlorosis, and interference with ion uptake (Sagardoy et al. 2009; Hassan and Aarts 2011). The optimum Zn concentration inside plant tissues for adequate growth is estimated in 15–150 mg Zn kg−1 dry weight (DW) (Markert 1992). The guideline for safe limits of Zn in agricultural soils is 300 ppm (as reported in Table 5 of Nagajyoti et al. 2010), but anthropogenic inputs can enrich soil. In fact, the Zn concentration in sewage sludge ranges from 91 to 49,000 ppm, in phosphatic fertilizers from 50 to 1450 ppm, and in farmyard manure from 15 to 556 ppm (Nagajyoti et al. 2010).
Populus species include several cultivars: One of them was the Populus alba L. clone ‘Villafranca’ (Romeo et al. 2014a), obtained at the Poplar Research Institute, Casale Monferrato (Italy) crossing a female P. alba from Villafranca Piemonte (Piedmont, Italy) and a male specimen of the same species from Lucca (Tuscany, Italy). This genotype showed a good biomass production and a remarkable re-sprouting ability after coppicing (Confalonieri et al. 2000). Moreover, Villafranca has been previously studied for its high Zn uptake traits in hydroponic studies aimed to compare several poplar species (Romeo et al. 2014a) and successfully used as a model plant in transformation experiments to get new varieties with traits related to herbicide tolerance and insect pest tolerance (Confalonieri et al. 2000).
This study was planned under controlled environmental conditions with the objectives to evaluate the efficiency of P. alba clone Villafranca in the uptake and translocation of Zn from artificially polluted soil in order to simulate a monometallic pollution. With this proposal, the radial growth profile was monitored during the experiment in order to study the secondary growth in terms of biomass production in relation to Zn treatment.
2 Material and methods
2.1 Plant materials and Zn treatments
Woody stem cuttings obtained from 1-year-old cutback stems of P. alba L. clone Villafranca were planted into sand. After rooting (1 month), plants were placed in 1-dm3 pots containing sand and peat moss (25:75, v/v, pH 6.9) to allow stem bud sprouting and shoot growth. Plants were cultivated in a growth chamber at 21 ± 2 °C, 70 ± 5 % of relative humidity, and an irradiance of 1000 μmol m−2 s−1 provided during 16-h photoperiod. After 6 weeks, plants were transferred into pots containing 1.6 kg of soil DW and concomitantly pruned to second axillary bud in order to make sure that the new leaves were entirely formed under Zn exposure (day 0).
A solution of ZnSO4 × 7H2O was added to soils of treated plants (Zn t ) at the beginning of the experiment (T 0) and then again after 30 days (T 1) in order to reach a target Zn concentration of 375 ppm at T 0 and 750 ppm at T 1 per unit of soil dry weight; sulfate was balanced with K2SO4 in the case of control plants. The whole experimental period was 48 days (T 2). Plants were manually irrigated every 2 days with tap water avoiding leaching.
2.2 Growth parameters
Leaf area, leaf number, and stem length were measured every 3 days. After 30 (T 1) and 48 (T 2) days from the beginning of the experiment, six plants from each groups of treatments were collected and divided into root, woody cutting, stem (newly formed stem during the treatment), and leaves.
Leaf mass ratio (LMR), stem mass ratio (SMR), and root mass ratio (RMR) were calculated as the fraction of the total biomass allocated to the leaves, stem, and root [organ mass (g)/mass of plant (g)] to whole plant (Hunt 1978). The translocation factor (Tf) was calculated as ratio of Zn concentration in aerial part (leaves, stem, and woody cutting) to root. The Zn bio-concentration factor (BCF) of root system and aerial part (woody cutting, stem, and leaves) was calculated as the ratio of Zn concentration in specific organ to Zn soil concentration.
According to Morabito et al. (2006), individual leaf width (Lw, cm) was manually measured periodically in order to estimate not destructively the leaf area (La, cm2) using the following formula: La = (0.71 × Lw2) + (1.114 × Lw), R 2 = 0.98. Leaf area ratio (LAR, cm2 g−1) was calculated as the fraction of the total leaf area and total biomass allocated to the leaves.
2.3 Zn determination
Each plant was separated into roots, stems, woody cuttings, and leaves. Then, the plant organs were oven dried at 70 °C for 7 days. Dried plant organs were grounded with a laboratory grinder, and 200 mg (±0.5 mg) of samples were treated by an acid mixture (3 ml of 37 % hydrochloric acid and 6 ml of nitric acid 65 %) and digested with a pressurized closed-vessel microwave system (Multiwave 3000, Anton Paar GmbH, Germany). The digested samples were adjusted to 50 ml with deionized water and used for the determination of Zn by atomic absorption spectrophotometer (model 373; Perkin-Elmer, Norwalk, CT, USA).
2.4 Cutting radial growth
Cutting radial growth during the 48 days of treatment was measured every 15 min with an automatic point dendrometer using six plants per treatment. A linear motion sensor (AB Electronics Ltd., Romford, Essex, UK) was mounted and adjusted on woody cutting so that its diameter changes mechanically impinged on it, forcing the electric tension out of the variable resistance of the sensor connected as a tension divider. The variable tension was digitized by an analog-to-digital converter (Adlink Technology Inc., Taiwan) plugged into a PC-based recording data system. The software piloting this device and storing the collected data was developed in Visual Basic for Applications and integrated in Excel’s spreadsheet (Microsoft, Redmond, Washington, USA) (Morabito et al. 2006). The daily ΔR was calculated by dividing the stem radius diurnal cycle into three distinct phases: (i) contraction phase (the period between morning maximum and afternoon minimum), (ii) expansion phase (the period from the minimum to the following morning maximum), and (iii) stem radius increment phase (part of the expansion phase from the time when the stem radius exceeds the morning maximum until the subsequent maximum). The difference between maximum expansion and the beginning of the third phase represents the positive ΔR. When the maximum value of the previous cycle was not reached, negative ΔR (cm) was calculated (Giovannelli et al. 2007).
2.5 Photosynthetic activities
Net CO2 assimilation (A) and leaf stomatal conductance to water vapor (g s) were measured on the last fully expanded leaf for six plants for each treatment and were conducted with a portable photosynthetic system (Ciras-2, PP Systems, Hitchin, Herts, UK) on days 14, 20, 23, 27, 30, 35, 40, and 43 from the beginning of experiment. From these data, the water use efficiency was estimated (WUE = A/gs).
2.6 Statistical analysis
The experiment was set up in a completely randomized design (n = 5 for data at T 1; n = 6 for data at T 2). Data were subjected to statistical analyses. Two-way ANOVA for statistical evaluation of the effects produced by Zn, organ, and interaction of these two factors was applied to data regarding Zn concentration and Zn content. Means were subjected to Fisher’s LSD test at 0.05 probability level. Unpaired t test analysis at 0.05 probability level was performed on data about leaf mass ratio, stem mass ratio, root mass ratio, leaf area ratio, leaf area, stem length, leaf number, CO2 assimilation, stomatal conductance, and WUE. Statistical analysis was performed using NCSS 2000 Statistical Analysis System Software.
3 Results and discussion
In this experiment, the addition of Zn to substrate was deliberately designed to reach a concentration value two times higher than the one imposed in agricultural soil by the European Union guideline (Nagajyoti et al. 2010). As a consequence of Zn treatments, the leaves of Villafranca poplar clone did not display visual symptoms of injury (chlorosis, necrosis, or leaf senescence). Moreover, dry weight did not differ in response to Zn although an increment of RMR was observed after 30 days of Zn exposure compared to control (Table 1). As previously demonstrated by Romeo et al. (2014b), Zn excess condition can induce an increase of root branching, considered as an early strategy to facilitate water and nutrition absorption in the root system under Zn excess conditions.
In this study, it has been shown that the growth responses of Villafranca clone to Zn application differ between organs and do not correspond to Zn accumulation. The total leaf area was not affected in Zn t plants up to day 48 (T 2), when a 12 % increase occurred in comparison to control plants (Fig. 1). As also observed by Di Baccio et al. (2010), this means that increasing Zn concentration can affect the area expansion of leaves before their biomass allocation.
Leaf area (I), stem length (cm) (II), and leaf number (n) (III) of Villafranca clone. T 0 corresponds to the first Zn treatment, T 1 to the second, and T 2 is the end of experiment. Values are means ± standard error (n = 5 for data at T 1; n = 6 for data at T 2). Means were subjected to t test analysis at 0.05 probability level. *P ≤ 0.05
No effect on stem length and leaf number were found comparing the two sets of plants, that reached at the end of the experimental period an average stem length of 64 ± 1.99 cm and a leaf number equal to 27 ± 0.51 (Fig. 1).
Although plant biomass was unaffected under Zn exposure, the heavy metal was uptaken in the different plant organs in the following order: root ≫ leaves ≥ woody cutting = stem.
At the end of exposure (T 2), root Zn concentration of Zn t plants was 1808 ± 218 mg kg−1, the double compared to T 1 (907 ± 130 mg kg−1) (Fig. 2a, b). Furthermore, at each sampling time (T 1 and T 2), root Zn concentration was, on average, three times of magnitude higher than in leaves. Stems and woody cuttings of Zn t plants exhibited the lowest Zn concentrations relatively to other organs (Fig. 2a, b). Similar trend of Zn accumulation was previously found by Romeo et al. (2014a) in hydroponic experimental condition.
Box-and-whiskers representation of Zn concentration in root, woody cutting, stem, and leaves of Populus alba clone Villafranca at time T 1 (a) and T 2 (b) (n = 5 for data at T 1; n = 6 for data at T 2). Whiskers represent maximum and minimum values. Results were analyzed by two-way ANOVA. Different letters are significantly different according to Fisher’s LSD test at 0.05 probability level
The capability of Villafranca clone to extract and accumulate Zn in the plant was evaluated calculating the plant/soil concentration quotients (bio-concentration factor) as reported in Fig. 3a, b. The BCF ranged between 0.38 and 3.83 at T 1 and between 0.30 and 4.06 at T 2. The BCF indicated that Villafranca poplar clone had the same capability to accumulate Zn in different organs independently of timing of exposure. Moreover, in Villafranca clone, the root BCF represented approximately 55 and 62 % of the whole plant Zn uptaken from the soil at time T 1 and T 2, respectively.
Bio-concentration factor calculated on Populus alba clone Villafranca at time T 1 (a) and T 2 (b), respectively (n = 5 for data at T 1; n = 6 for data at T 2). Results were analyzed by one-way analysis of variance (ANOVA) with Tukey’s multiple comparison test (P < 0.05). Different letters represent significant differences among organs
The root system plays a key role in the interaction between heavy metal and plants. In fact, the Tf, that is indicative of the plant capability to accumulate Zn in the aerial part, was lower than 1 (Fig. 4a, b) at both time of sampling. In particular, a reduction of 30 % of Tf was observed at the end of experiment (T 2) as a consequence of an increase in root concentrations. Similar behavior was observed in Populus nigra and P. alba under Zn treatment in a hydroponic system (Dos Santos Utmazian et al. 2007).
Translocation factor calculated on Populus alba clone Villafranca at time T 1 (a) and T 2 (b), respectively (n = 5 for data at T 1; n = 6 for data at T 2). Results were analyzed by one-way analysis of variance (ANOVA) with Tukey’s multiple comparison test (P < 0.05). Different letters represent significant differences among organs
Villafranca clone did not show a metal indicator phenotype under Zn supply, and the highest Zn concentration was found at root level. Zn distribution in plant is also in agreement with data found in our previous experiment (Romeo et al. 2014a), confirming that this clone regulates Zn uptake independently to the substrate and experimental conditions applied.
As expected, the Zn content increased more in treated plants in comparison with the control ones, following the order: leaves > woody cutting = root ≥ stem (Fig. 5). Similar results were obtained by Langer et al. (2009) on Populus x canescens grown under different Zn doses and by Hermle et al. (2006) in Populus tremula.
Zn content in root, woody cutting, stem, and leaves of Populus alba clone Villafranca after 30 (a) and 48 (b) days from the beginning of the experiment. Data, n = 5 for (a) and n = 6 for (b) were analyzed by two-way ANOVA, and values having different letters are significantly different according to Fisher’s LSD test at 0.05 probability level
In leaves, Zn increased from T 1 to T 2 reaching values in the range 0.28 to 2.71 mg (Fig. 5a, b). Considering the theoretical harvestable parts (stem + woody cutting + leaves), the Zn content was about five times higher than the root content at each sampling time: 1.5 vs. 0.3 and 5.0 vs. 1.1 mg (T 1 and T 2, respectively).
The Zn concentration did not follow the same trend of Zn content, showing that the Zn accumulation was majorly influenced by biomass production. Considering Zn concentration of the harvestable portion (stem) of poplar plants and hypothesizing a plantation density of 10,000 plants ha−1 (short rotation forestry), producing 119 t of dry biomass ha−1 in an 11-year cycle (Bonari 2001), our experimental values correspond to 27.61 kg ha−1of Zn exported.
Combining different measurements of Villafranca clone performance provided a more accurate view about the net response of this poplar under Zn conditions. As reported by Körner (2003), secondary growth shows higher sensitivity to environmental conditions than photosynthesis, and so, the radial growth profile was monitored during the experiment in order to give information about secondary growth in terms of biomass production in relation to Zn t . Cutting radius changes show a high synchronicity in the growth rate fluctuation among control and Zn t plants (Fig. 6a), although after the 38th day, Zn t plants show an increase in radial diameter compared to control plants. This increase reached a value 38 % higher than in control plants after 48 days of treatment, as well as showed comparing the diurnal cycle of stem radius variation from 38 to 48 days (Fig. 6b).
Data about cutting radial growth were usually used for long-term experiments under different stress conditions such as heavy metals (Fedorkov 2007) or climatic change (Oberhuber et al. 2014), but high-resolution measurements of cutting radial growth variation could also provide a means to study short-term dynamics of tree growth. Radial growth is usually proportionally attributed to leaf area (Yang and Midmore 2005) and stem length, as also demonstrated under our experimental condition (Fig. 7a, b). In particular, both treatments showed similar linear correlation between these parameters indicating that under Zn t , plants follow the trend of control plants.
Moreover, the analyses of the positive (ΔR+) and negative (ΔR−) frequency distribution (ΔR), between treatments were performed (data not showed), and the occurrence of ΔR+ and ΔR− did not change significantly between Zn t and control plants (X 2 test, P < 0.05). ΔR+ and ΔR− were 92 and 88 % and 7 and 11 % for control and Zn t , respectively.
The performances of the whole plant evaluated by cutting radial growth are indicative of a plant morphological adjustment in response to applied Zn and could be used as an important parameter able to determine the outcomes of plant–environment interactions.
Several authors reported that photosynthesis is usually affected by high heavy metal concentrations differing among heavy metal applied, plant species, and cultivar (Borghi et al. 2008; Azzarello et al. 2012; Fernàndez et al. 2012; Mukhtar et al. 2013). As reported by Marschner (1995), Zn toxicity occurs when leaf concentration range is between 400 and 500 mg kg−1 dry weight. Although our data of leaf Zn concentration were in the range 358–535 mg kg−1 (T 1 and T 2, respectively), leaves of plants grown in Zn t substrate were unaffected for CO2 assimilation (Fig. 8a–c). These results are in agreement with previous data reported by Durand et al. (2010) for P. tremula × P. alba genotype under Zn excess (265 mg kg−1 in soil) that also did not exhibit a dry mass reduction like Villafranca clone in our experimental condition. Under 300 ppm of ZnCl2, the ecophysiological response of five Salix clones showed the absence of reductions in gas exchange parameters indicating an efficient plant homeostasis aimed to maintain the metal content within phytotoxic limits (Bernardini et al. 2015). The inhibitory effects of Zn on photosynthetic processes were observed by the authors in all clones analyzed only when Zn concentrations reached values of 750 and 1500 mg kg−1 in the soil.
Time course of CO2 assimilation (a) stomatal conductance g s (b), and water use efficiency (WUE) (c) of Populus alba clone Villafranca submitted to control conditions (empty circles) and Zn t (filled circles). Means ± standard error for control and Zn t (n = 6) were compared. For each time, results were subjected to t test analysis at 0.05 probability level. Asterisks indicate significant difference between control and Zn t . *P ≤ 0.05, **P ≤ 0.01
Comparing the results obtained in this experiment to hybrid Populus deltoids × P. nigra (Di Baccio et al. 2003), Salix viminalis, and P. tremula (Hermle et al. 2006), Villafranca clone could be considered able to tolerate Zn stress. When data about gas exchange were correlated to Zn concentration at leaf levels, we found that g s could be used as an integrative indicator able to give information on Zn uptake. In fact, although the net CO2 assimilation was not affected by Zn exposure, we observed significant negative correlation between g s and Zn concentration only when optimum plants’ Zn uptake was exceeded (Fig. 9).
Linear correlation between Zn concentration and g s (stomatal conductance) in leaves of Populus alba clone Villafranca at T 1 and T 2 sampling data. Open symbols represent control data at T 1 (empty circles) and T 2 (empty squares). Close symbols represent Zn t data at T 1 (filled circles) and T 2 (filled squares)
4 Conclusions
Taking into account all data accumulated during this experiment, but also previous results obtained on different experimental condition (Romeo et al. 2014a), P. alba Villafranca could be considered as a Zn-resistant plant. In fact, although the foliar Zn concentration was above the normal ranges for plants, Villafranca clone did not show any toxicity symptoms like necrosis or chlorosis. More sensitive analyses like cutting radial growth measurements revealed that this clone continues to improve its growth although under Zn supply. Applying data of Zn uptake observed in this experimental conditions to a hypothetical model of short rotation forestry application, the clone Villafranca will be able to accumulate up to 2.5 kg ha−1 year−1 Zn in the harvestable portion. These results suggest that this clone could be a suitable tree for in-field application of phytoremediation approach on contaminated land polluted by high Zn concentration.
References
Alloway BJ (2009) Soil factors associated with zinc deficiency in crops and human. Environ Geochem Health 31:537–548
Azzarello E, Pandolfi C, Giordano C, Rossi M, Mugnai S, Mancuso S (2012) Ultramorphological and physiological modifications induced by high zinc levels in Paulownia tomentosa. Environ Exp Bot 81:11–17
Baize D (1997) Teneurs totales en elements traces metalligues dans les sols (France). INRA Editions, Paris, p 409
Bernardini A, Salvatori E, Di Re S, Fusaro L, Nervo G, Manes F (2015) Natural and commercial Salix clones differ in their ecophysiological response to Zn stress. Photosynthetica. doi:10.1007/s11099-015-0155-9
Bonari E (2001) Potenzialità e problematiche agronomiche della silvicoltura a breve rotazione come coltura da energia negli ambienti mediterranei. Riv Agron 35:188–199
Borghi M, Tognetti R, Monteforti G, Sebastiani L (2008) Responses of two poplar species (Populus alba and Populus x canadensis) to high copper concentrations. Environ Exp Bot 62:290–299
Confalonieri M, Belenghi B, Balestrazzi A, Negri S, Facciotto G, Schenone G, Delledonne M (2000) Transformation of elite white poplar (Populus alba L.) cv. ‘Villafranca’ and evaluation of herbicide resistance. Plant Cell Rep 19:978–982
Di Baccio D, Tognetti R, Sebastiani L, Vitagliano C (2003) Responses of Populus deltoides × Populus nigra (Populus × euramericana) clone I-214 to high zinc concentrations. New Phytol 2:443–452
Di Baccio D, Minnocci A, Sebastiani L (2010) Leaf structural modifications in Populus × euramericana subjected to Zn excess. Biol Plant 54(3):502–508
Dos Santos Utmazian MN, Wieshammer G, Vega R, Wenzel WW (2007) Hydroponic screening for metal resistance and accumulation of cadmium and zinc in twenty clones of willows and poplars. Environ Pollut 148:155–165
Durand TC, Hausman JF, Carpin S, Alberic P, Baillif P, Label P, Morabito D (2010) Zinc and cadmium effects on growth and ion distribution in Populus tremula × Populus alba. Biol Plant 54:191–194
Fedorkov A (2007) Effect of heavy metal pollution of forest soil on radial growth of Scots pine. For Pathol 37:136–142
Fernàndez J, Zacchini M, Fleck I (2012) Photosynthetic and growth responses of Populus clones eridano and I-214 submitted to elevated Zn concentrations. J Geochem Explor 123:77–86
Franchin C, Fossati T, Pasquini E, Lingua G, Castiglione S, Torrigiani P (2007) High concentrations of Zn and Cu induce differential polyamine responses in micropropagated white poplar (Populus alba L. ‘Villafranca’). Physiol Plant 130:77–90
Giachetti G, Sebastiani L (2006) Metal accumulation in poplar plant grown with industrial wastes. Chemosphere 64:446–454
Giovannelli A, Deslauriers A, Fragnelli G, Scaletti L, Castro G, Rossi S, Crivellaro A (2007) Evaluation of drought response of two poplar clones (Populus x canadensis Monch ‘I-214’ and P. deltoides Marsh. ‘Dvina’) through high resolution analysis of stem growth. J Exp Bot 58:2673–2683
Guerra F, Gainza F, Pérez R, Zamudio F (2011) Phytoremediation of heavy metals using poplars (Populus spp): a glimpse of the plant responses to copper, cadmium and zinc stress. In: Ivan A. (ed) Handbook of Phytoremediation. Golubev© Nova Science Publishers, Inc. ISBN: 978-1-61728-753-4
Hassan Z, Aarts MGM (2011) Opportunities and feasibilities for biotechnological improvement of Zn, Cd or Ni tolerance and accumulation in plants. Environ Exp Bot 72:53–63
Hermle S, Günthardt-Goerg MS, Schulin R (2006) Effects of metal-contaminated soil on the performance of young trees growing in model ecosystems under field conditions. Environ Pollut 144:703–714
Hunt R (1978) Plant growth analysis. Camelot Press Ltd Southampton, UK
Körner C (2003) Alpine plant life: functional plant ecology of high mountain ecosystems. Springer, Berlin
Langer I, Krpata D, Fitz WJ, Wenzel WW, Schweiger PF (2009) Zinc accumulation potential and toxicity threshold determined for a metal-accumulating Populus canescens clone in a dose–response study. Environ Pollut 157:2871–2877
Manara A (2012) Plant responses to heavy metal toxicity. In: Furini A (ed) Plants and heavy metals. Springer briefs in biometals. Springer, New York, pp 27–53
Markert B (1992) Presence and significance of naturally occurring chemical elements of the periodic system in the plant organism and consequences for future investigations on inorganic environmental chemistry in ecosystems. Vegetatio 103:1–30
Morabito D, Caruso A, Carpin S, Carli C, Laurans F, Depierreux C et al (2006) Cambial activity of Populus tremula x Populus alba clone 717-1B4 in hydroponic culture. Can J Forest Res 36:719–724
Mukhtar N, Hameed M, Ashraf M, Ahmed R (2013) Modifications in stomatal structure and function in Cenchrus ciliaris L. and Cynodon dactylion (L.) Pers. In response to cadmium stress. Pak J Bot 45:351–357
Nagajyoti PC, Lee KD, Sreekanth TVM (2010) Heavy metals, occurrence and toxicity for plants: a review. Environ Chem Lett 8:199–216
Oberhuber W, Gruber A, Kofler W, Swidrak I (2014) Radial stem growth in response to microclimate and soil moisture in a drought-prone mixed coniferous forest at an inner Alpine site. Eur J For Res 133:467–479
Romeo S, Francini A, Ariani A, Sebastiani L (2014a) Phytoremediation of Zn: identify the diverging resistance, uptake and biomass production behaviours of poplar clones under high zinc stress. Water Air Soil Pollut 225:1813–1825
Romeo S, Trupiano D, Ariani A, Renzone G, Scippa GS, Scaloni A, Sebastiani L (2014b) Proteomic analysis of Populus × euramericana (clone I-214) roots to identify key factors involved in zinc stress response. J Plant Physiol 171:1054–1063
Sagardoy R, Morales F, Lόpez-Millán AF, Abadía A, Abadía J (2009) Effects of zinc toxicity on sugar beet (Beta vulgaris L.) plants grown in hydroponics. Plant Biol 11:339–35
Sebastiani L, Scebba F, Tognetti R (2004) Heavy metal accumulation and growth responses in poplar clones Eridano (Populus deltoides x maximowiczii) and I-214 (P. x euramericana) exposed to industrial waste. Environ Exp Bot 52:79–88
Sebastiani L, Francini A, Romeo S, Ariani A, Minnocci A (2014) Heavy metals stress on poplar: molecular and anatomical modifications. In: Gaur RK, Sharma P (eds) Approaches to plant stress and their management. Springer, India, pp 267–279
Unterbrunner R, Puschenreiter M, Simmer P, Wieshammer G, Tlustos P, Zupan M, Wenzel WW (2007) Heavy metal accumulation in trees growing on contaminated sites in Central Europe. Environ Pollut 148:107–114
Yang Z, Midmore DJ (2005) Modelling plant resource allocation and growth partitioning in response to environmental heterogeneity. Ecol Model 181:59–7
Author information
Authors and Affiliations
Corresponding author
Additional information
Responsible editor: Jaume Bech
Stefania Romeo and Alessandra Francini contributed equally to this work.
Rights and permissions
About this article
Cite this article
Romeo, S., Francini, A., Sebastiani, L. et al. High Zn concentration does not impair biomass, cutting radial growth, and photosynthetic activity traits in Populus alba L.. J Soils Sediments 17, 1394–1402 (2017). https://doi.org/10.1007/s11368-015-1251-y
Received:
Accepted:
Published:
Issue Date:
DOI: https://doi.org/10.1007/s11368-015-1251-y