Abstract
Excess nitrogen and phosphorus nutrients in the aquatic environment result in the growth of algal cells and water eutrophication, which adversely affect the aquatic environment and human health. Therefore, discovering a safe and efficient algae suppression method is necessary to ensure the ecological safety of water. Recently, the allelopathic effects of aquatic plants on algae have attracted extensive attention from researchers. This review demonstrates the current research hotspot of allelopathic algal inhibition in aquatic plants and lists the common aquatic plant species and allelochemicals. In addition, the inhibition mechanism of allelochemicals from aquatic plants on algae is systematically discussed. Moreover, the key factors affecting the inhibition of allelopathy in algae, such as pH, temperature, algal cell density, and concentration of allelochemicals, are summarized. The present utilization modes of allelochemicals on algae are also presented. Finally, the problems existing in the study of allelopathic algal inhibition of aquatic plants are highlighted, and suggestions for further research are proposed.
Similar content being viewed by others
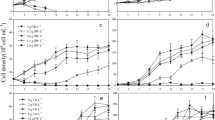
Explore related subjects
Discover the latest articles, news and stories from top researchers in related subjects.Avoid common mistakes on your manuscript.
Introduction
Due to the large amount of wastewater containing nutrients such as nitrogen and phosphorus being discharged into the water body, eutrophication occurs frequently all over the world (Suresh et al. 2023). Eutrophication, also known as algal bloom, refers to the phenomenon of water pollution caused by the rapid growth of algal cells on the water surface (Zhao et al. 2015). When certain algae, including Microcystis aeruginosa and Prymnesium parvum, reach high concentrations in a specific range of water, they cause detrimental effects on the aquatic ecosystem of the surrounding area. When the algal bloom phenomenon occurs, a large number of algal cells float on the water’s surface, which reduces the transparency of the water (Abadie et al. 2018), making it difficult for sunlight to penetrate the water and affecting the growth of aquatic plants. At the same time, algal blooms consume a large amount of oxygen in the water, resulting in the death of aquatic organisms due to anoxia. Dead aquatic organisms and other organic matter are decomposed by microorganisms under anaerobic conditions, producing an unpleasant odor (Cheng et al. 2022). Some harmful algal can even produce toxins, which can accumulate in the food chain through their toxic effects on aquatic organisms, ultimately threatening human health (Liu et al. 2022). As a consequence, the inhibition of excess algal growth has become an urgent issue in environmental protection.
The main methods currently studied that can be used for algae removal are physical, chemical, and biological methods (Xie et al. 2023). Physical methods mainly include ultrasonic treatment (Kong et al. 2019), biochar adsorption (Cheng et al. 2022), mechanical fishing, etc. The physical approach poses a minimal risk of secondary water pollution. However, it is time-consuming and expensive, so this treatment method is unsuitable for large-scale use. Chemical methods are generally used to achieve algal growth inhibition and removal by dispensing chemical agents such as oxidants (Zhang et al. 2020), fungicides (Chavan et al. 2017), flocculants (Zhang et al. 2023), and photocatalysts (Fan et al. 2023; Yang et al. 2023). The chemical method is distinguished by its abbreviated processing time, expeditious effectiveness, and superior treatment efficiency. However, secondary pollution is a risk (Sun et al. 2015), and the chemicals may also be toxic to other organisms (Zhao et al. 2018). Biological methods, however, aim to create unfavorable conditions for algal growth (Kibuye et al. 2021). Standard methods such as the use of algae-killing bacteria, zooplankton, and allelopathy in plants are considered to be more economical and friendly methods for controlling cyanobacterial blooms (Jia et al. 2010) and degrading cyanobacterial toxins (Mohamed and Alamri 2012). Usually, allelopathic algal inhibition of aquatic plants provides a new viewpoint for biological algae inhibition, which has become the preferred method to improve eutrophic water in recent years.
“Allelopathy” was first proposed by Molisch (1937). Rice (1974) expanded the definition of allelopathy in the mid-1970s. Plants (including microorganisms) produce chemical compounds that are released into the environment. These compounds showed direct or indirect harmful effects on other plants. With a mature understanding of allelopathy, Rice (1984) extended the definition of allelopathy: plants that directly or indirectly cause positive or negative effects on other plants or microorganisms by releasing certain chemical substances into the surrounding environment. Currently, allelopathy refers to any process in which organisms produce bioactive metabolites that affect other neighboring plants or fungi (Legrand et al. 2003; Fa et al. 2010; Bajwa 2014). Plants produced secondary metabolites called allelochemicals (Long et al. 2021), which are considered environmentally friendly algaecides due to their natural origin, low toxicity, high selectivity, and biodegradability (Wang et al. 2018b).
The purpose of this review is to understand the research hotspots of allelopathy by using a co-occurrence diagram. In addition, we examine the inhibition mechanisms and influencing factors of allelochemicals from aquatic plants. Moreover, this work aims to expose practical applications of allelochemicals to provide a basis for water ecological health management.
Current research hotspots
This paper used “allelopathy” and “algae” as search keywords in the Web of Science database to search for relevant documents published before December 15, 2022. A co-occurrence diagram of allelopathy was prepared in VOSviewer to analyze current international research hotspots (Fig. 1). The figure is divided into six clusters and different research topics.
In cluster 1, allelopathy involves competition. This theme covers keywords such as competition, macroalgae, and chemical defenses. Aquatic plants restrain algae growth by releasing allelochemicals, which is one of the essential method of obtaining effective competition (Qi et al. 2019b). Organisms might inhibit the growth of other organisms by secreting allelochemicals, thus contributing to their advantage to growth in the environment. Cluster 2 shows the extraction of allelochemicals. This topic covers keywords such as inhibition, gallic acid, and usnic acid. Through allelopathy, the plant can secrete a variety of bioactive compounds, i.e., allelochemicals. Researchers can determine the types and contents of allelochemicals with algae-inhibiting effects by different extraction and identification methods, which can provide a reference for future research. Cluster 3 corresponds to the control of water eutrophication. This theme covers keywords such as submerged macrophytes, blue-green algae, growth inhibition, and aquatic macrophyte. Aquatic plants have attracted increasing attention owing to their rapid growth, abundant secondary metabolites, and direct contact with water, which have great potential in algae control applications. Most current studies focus on the use of allelopathy of aquatic plants to inhibit the growth of algae, thus providing ideas for the solution to eutrophication in water bodies. Cluster 4 corresponds to mechanisms of allelochemicals to inhibit algal growth. This topic covers keywords such as oxidative stress, chlorophyll fluorescence, and protease inhibitors. Plant allelochemicals reduce algal growth by different mechanisms and by now have been extensively studied. Cluster 5 corresponds to algal cells that are inhibited by allelopathy. This topic covers keywords such as phytoplankton, harmful algae, and P. parvum. Since allelopathy has different effects on different study subjects, the subjects can be restructured according to the type of algae present in the water that urgently needs to be addressed. Common types of algae cells inhibited by allelopathy mainly include P. parvum, Heterosigma akashiwo, M. aeruginosa, etc. P. parvum (Chromophyta: Haptophyceae) has been detected in both coastal and brackish waters and inland freshwaters (Feist and Lance 2021). Its euryhaline and eurythermal properties support aquatic ecosystems with different gradients (Taylor et al. 2021). The golden-brown marine alga H. akashiwo (Chromophyta: Raphidophyceae) is frequently observed in coastal waters globally and has been increasing in incidence and distribution (Ling and Trick 2010; Zhang et al. 2018). M. aeruginosa is a prominent species of freshwater cyanobacterium with worldwide distribution and a propensity for forming blooms (Dick et al. 2021). These types of algae are deemed to be a substantial threat to the well-being of aquatic ecosystems and are commonly singled out as objectives for allelochemical suppression. Cluster 6 shows the influencing factors of allelopathic algal inhibition. This topic covers keywords such as growth, toxicity, different nutrient conditions, and cell density. The strength of allelopathic inhibition of algae has different influencing factors, such as different nutrient conditions, distinct algal cell densities, and varying temperatures. The best algae-inhibiting conditions can be found by changing different factors.
Aquatic plants and allelochemicals that inhibit algal growth
Allelochemicals
Allelochemicals are secondary metabolites secreted into the water by plants that show toxicity or deterrent effects on algae and cyanobacteria (Li et al. 2021b). Allelochemicals produced by terrestrial higher plants were divided into 14 categories by Rice (1984), as shown in Table 1. Among them, the most common allelochemicals are phenolic compounds, terpenes, and fatty acids (Li et al. 2021b). Nevertheless, the structures of allelochemicals are different in aquatic plants and lower terrestrial plants compared to those of higher terrestrial plants (Zhou et al. 2018). The use of allelochemicals secreted by aquatic plants to minimize algae growth has been suggested to be a more reliable option (Chen et al. 2012). Allelochemicals secreted by aquatic plants are generally directly delivered into the water through natural volatilization, rain and fog leaching, root secretion, and decomposition of plant residues or litter (Xuan et al. 2005). Their impact on water is more direct and effective than that of terrestrial plants. It can be seen that the discussion of the allelopathic effect of aquatic plants is of great importance. Studies of allelochemicals from aquatic plants have been conducted over the past twenty years. The study of allelopathy has shown that a large number of aquatic plants are capable of secreting allelochemicals that exert inhibitory effects on algae (Mohamed 2017). For instance, allelochemicals with inhibitory effects on algae have been collected from aquatic plants such as Vallisneria (Wang et al. 2022), Pistia stratiotes L. (Wu et al. 2013a), and Phragmites australis (Men et al. 2006). Allelochemicals produced by aquatic plants are listed in Table 2.
Aquatic plant species
Previous research on allelopathy was focused on terrestrial plants, but with the deterioration of aquatic ecosystems, algal blooms have become a global problem (Zhu et al. 2021). To solve this problem, researchers began to explore the allelochemicals from aquatic plants to inhibit algae in the late 20th century (Nakai et al. 1999). Aquatic plants have allelopathic effects on inhibiting algal cells in the aquatic environment, including submerged plants, floating plants, and emergent plants. Aquatic plants showing an inhibitory effect on algae are listed in Table 2, and the mechanisms involved in algal growth inhibition are shown in Fig. 2.
Submerged plants
Submerged plants inhibit algae mainly through three main processes: competition, shading, and allelopathy. Submerged plants can compete with algae in water for nutrient conditions, resulting in the inhibition of algal growth (Preiner et al. 2020). Additionally, submerged plants can impede incoming light and release allelochemicals, which can ultimately inhibit algae growth (Mohamed 2017). The ultrasonic cellulase extraction of Vallisneria produces benzylic acid, sorbic acid, and glycerol, which substantially inhibit the growth of M. aeruginosa (Wang et al. 2022). Similarly, Haroon and Abdel-Aal (2016) characterized allelochemicals secreted by Potamogeton crispus using gas chromatography–mass spectrometry (GC-MS) analyzer and demonstrated that fatty acids, esters, and ketones secreted by P. crispus also had inhibitory effects on cyanobacteria and diatoms.
Floating plants
Floating plants typically have larger leaf structures that attenuate light and temperature in the water, ultimately hindering the growth of algae to some degree (Guo et al. 2014). It was also found that floating plants can further influence the growth of algae through the release of allelochemicals. The floating plants Nymphaea tetragona and Nelumbo nucifera were found to have algae inhibition activity up to 75–82% on M. aeruginosa after 19 days of algae inhibition tests (Chen et al. 2012). When the concentration range of allelochemicals extracted from P. stratiotes L. was 60–100 mg/L, the allelochemicals had the most potent inhibitory effect on the growth of algae, with a relative inhibition ratio reached 50–90%. Furthermore, the chlorophyll-a (Chl-a) content in algae cell cultures was reduced by 50–75% within 3–7 days (Wu et al. 2013a). Wu et al. (2018) investigated the potential of propionamide, derived from the aquatic plant Eichhornia crassipes, for controlling cyanobacterial blooms. The results demonstrated that the inhibition rate of algae cells exceeded 90% when 2 mg/L propionamide was co-cultured with the cells for 7 days by semi-continuous addition. Additionally, they showed the gradual disintegration of algal cells.
Emergent plants
The stems and leaves of emergent aquatic plants extend out of the water, and the roots grow in the silt of the water bottom, inhibiting the growth of algae mainly through the release of allelochemicals from the roots (Qian et al. 2021). The aqueous extract of the roots of the aquatic plant Thalia dealbata could dramatically inhibit the growth of the cyanobacteria Anabaena flosaquae and M. aeruginosa. It mainly leads to a decrease in chlorophyll content and to inhibition of total dehydrogenase activity (Zhang et al. 2011). A similar situation was observed with the aqueous extract of Arundo donax L. after 7 days of cultivation. The percentage of inhibitions (PIs) was above 60% for all treatment groups, and the maximum almost reached 100%. In addition, the potential allelochemicals of A. donax L. mainly include esters, ketones, alkaloids, and phenolic acids (Hong et al. 2011).
Inhibition mechanism of allelochemicals on algae growth
Allelopathy of aquatic plants has been shown to inhibit the growth of algae cells. Current studies have found that the mechanism of allelopathy against algae mainly includes the destruction of cellular structures, causing oxidative stress in cells as well as programmed cell death (PCD) and influencing the photosynthetic system and protein synthesis. The algae-inhibiting mechanism of allelochemicals is shown in Fig. 3.
Destruction of cellular structures
As a natural barrier between cells and organelles, allelochemicals initially influence the cytomembrane (Fink 2007). Allelochemicals destroy the permeability and integrity of the cytomembrane, and disturbances in cytomembrane integrity may lead to disruptions in organelle structure and function. The allelochemical ethyl-2-methyl acetoacetate (EMA) damaged the cytomembrane of M. aeruginosa, resulting in the leakage of substances from the algal cells and the destruction of the nucleus and mitochondrial structure (Li et al. 2007). After adding an extract of the aquatic plant Vallisneria, the cell morphology of M. aeruginosa changed dramatically. Wang et al. (2022) used concentrations of 0.5 g/L and 1 g/L test groups and observed extracellular attachments and slight cell damage. Similarly, in the 10 g/L and 20 g/L experimental groups, the membrane of algae cells was destroyed, resulting in leakage of intracellular fluid and the formation of columnar structures through cell connections. When M. aeruginosa cells were exposed to 14 mg/L pyrogallic acid (PA) for 72 h, the algal cells showed nuclear membrane rupture and extravasation of intracellular material, which eventually led to vacuolation (Lu et al. 2017). Similarly, Tan et al. (2019) proposed that the shape of algal cells would change under the action of a particular concentration of allelochemicals, which manifested as protoplast contraction and cell wall separation, as well as reduced nuclear and chloroplast structures.
Causes of oxidative stress in algal cells
Oxidative stress is produced by an increase in the level of reactive oxygen species (ROS) and can induce damage to biological macromolecules (Staszek et al. 2021; Wang et al. 2022). Algae cells produce oxygen free radicals, including hydroxyl radical (HO•), hydrogen peroxide (H2O2), and superoxide anion radical (O2•−) and accumulate reactive oxygen species (ROS), under the stimulation of allelochemicals. Enzymes of the antioxidant system, superoxide dismutase (SOD), catalase (CAT) and peroxidase (POD), can reduce ROS so that the cells will continue their normal physiological activities. However, if there is an accumulation of ROS within a short time that exceeds the normal metabolic level of antioxidant enzymes, it will result in cellular demise, thus inhibiting algae growth. Kirpenko and Usenko (2013) proposed that under the influence of EMA, the concentration of ROS and lipid peroxide products in algae cells increased. At the same time, the activity of some antioxidant enzymes was inhibited. Malondialdehyde (MDA), the product of lipid peroxidation in algal cell membranes under stress, is commonly used to assess the degree of damage to cell membranes and to measure the degree of stress in algal cells (Zhu et al. 2021). In the semi-continuous co-culture system, after 108 h of exposure to allelochemicals produced by the aquatic plant Cyperus alternifolius, the MDA content of M. aeruginosa was 3.6 times higher than that of the control group and then decreased sharply (Zhu et al. 2014).
Causes of programmed cell death (PCD)
PCD is a genetically controlled biological process of cellular self-destruction that involves the transcription of specific genes and protein synthesis (Lockshin and Zakeri 2004; Bidle 2016). ROS are the bridge between allelopathy and PCD. When low-concentration allelochemicals stimulate algae cells, they act as signal molecules to regulate growth and development processes and activate defense mechanisms. In contrast, ROS trigger PCD when exposed to high concentrations of allelochemicals. Multiple caspase orthologs, such as paracaspases, metacaspases and caspases, have been shown to be involved in the PCD process in algal cells (Bidle 2016). Plants rely on caspases for oxidative stress to induce PCD (Klemenčič and Funk 2018; Qi et al. 2019a). Simultaneously, PCD-treated algae constantly show changes such as shrinkage, phosphatidylserine externalization, nuclear fragmentation, and concentration. Xu et al. (2022) conducted a study on the process of PCD induced by 5,4,-dihydroxyflavone (5,4,-DHF) in two cyanobacteria, M. aeruginosa and Phytocystis globularis. The findings revealed that 5,4,-DHF exhibited a considerable inhibitory effect on both algal species, and typical indicators of PCD, phosphatidylserine externalization and chromatin condensation, were detected in both species.
Impact on the photosynthetic system
Photosynthesis is one of the necessary conditions for the normal physiological metabolism of algae cells. The addition of allelochemicals markedly reduces pigment content by destroying the synthesis of photosynthetic pigments or stimulating the decomposition of photosynthetic pigments (Zhu et al. 2021). Furthermore, chlorophyll is one of the main pigments for photosynthesis in aquatic plants. The ethanol extract obtained by Sun et al. (2021b) from the brown microalga Sargassum fusiforme displayed the ability to substantially reduce the chlorophyll content of H. akashiwo, which negatively impacted the average growth of algae cells. When algal cells were treated with different concentrations of tannins, Chl-a inhibition in algal cells was 95% when treated with 140 mg/L tannins for 13 days, and the color of the exposure solution gradually turned yellow, suggesting that the photosynthesis of the algae was inhibited (Xie et al. 2023).
Many allelochemicals produced by aquatic plants are able to inhibit photosystem II (PSII). PSII is a highly conserved multiprotein pigmented membrane complex that catalyzes light-driven water splitting, oxygen evolution, and plastoquinone reduction, which is crucial for photosynthesis (Li et al. 2023b). While the physiological state of PSII can be measured by chlorophyll fluorescence (Henriques 2009), it primarily includes maximum photochemical efficiency (Fv/Fm), effective photochemical quantum yield (YPSII), ETR, IK, α, and other photosynthetic parameters. Fv/Fm can measure the photosynthetic efficiency of PSII. With the increase in α-linolenic acid concentration (4, 6, 8 mg/L), the Fv/Fm ratios of H. akashiwo decreased dramatically (6.8–29.4%), which suggested that PSII was the potential mechanism for the inhibition effect (Sun et al. 2021b). The pyrogallic acid (PA) and gallic acid (GA) in the aquatic plant Myriophyllum spicatum inhibited the PSII activities of M. aeruginosa by 71.43% and 70.95%, respectively (Zhu et al. 2010). Analogously, Nakai et al. (2014) also found that allelochemicals produced by M. spicatum markedly inhibited PSII activity. Chlorophyll fluorescence parameters were restricted by the capacity of the electron transport chain and Calvin activity in photosynthesis (Xu et al. 2020). Toxicological studies have revealed that linoleic acid significantly impairs photosynthetic pigment content in M. aeruginosa, ultimately leading to the blockage of electron transport in the photosynthetic electron transfer chain, as reported by Ni et al. (2018). Leu et al. (2002) found that β-1,2,3-tri-O-galloyl-4,6-(S)-hexahydroxydiphenoyl-D-glucose, known as tellimagrandin II, dramatically disrupted the electron transport chain in the cyanobacteria Anabaena sp. and Synechococcus sp. by enhancing the redox midpoint potential of nonheme iron.
PSII is often the primary target when algae cells are under pressure from the surrounding environment, and oxygen production is impacted by allelochemicals (Zhu et al. 2021). For instance, the respiration rate of algal cells began to decrease when the allelochemicals isolated from the terrestrial plant Aloe vera came into contact with the algal cells (Hongying and Hong 2008). Shao et al. (2010) also observed a 34% reduction in the rate of oxygen evolution in M. aeruginosa upon exposure to a concentration of 4 mg/L nonanoic acid. Furthermore, allelochemicals extracted from the aquatic plant M. spicatum adversely affected the respiration of the microalga Selenastrum capricornutum and the cyanobacterium M. aeruginosa (Zhu et al. 2010).
Influence on protein synthesis
Protein is a significant supporter of life functions and an essential component of cells. Allelochemicals are able to negatively affect algae growth by affecting protein synthesis. Chao (2013) discovered that allelochemicals reduced the protein content in approximately 80% of M. aeruginosa cells. Expression of the psbA gene encoding the D1 protein was markedly inhibited by pyrogallic acid, resulting in the failure of protein synthesis (Wu et al. 2013b). Similarly, Shao et al. (2010) investigated the effect of pyrogallic acid on gene expression in M. aeruginosa and found that protein was an important target site for pyrogallic acid inhibition, which affected the growth of M. aeruginosa by disrupting protein synthesis.
Factors affecting the inhibition of allelopathy in algae
As an effective competition strategy, the allelopathic effects of aquatic plants are certainly affected by the surrounding biotic and abiotic factors. It is of great value to discuss the allelopathy of aquatic plants under different influencing factors to reveal the regulation of allelopathy in aquatic ecological systems (Li et al. 2021b).
Abiotic factors represent all physicochemical factors in an ecosystem that have an impact on a particular biotic population and are behaviors from non-living to living. In contrast, biological factors are related to the activities of organisms and have an impact on the distribution of plant and animal species in a given biological community (Chaïb et al. 2021).
Abiotic factors
Light intensity
Light may cause changes in allelochemicals due to oxidation, polymerization, or degradation (Gross et al. 2007). It has been shown that light intensity substantially affects the production of allelochemicals. Through field manipulation experiments, Cronin and Lodge (2003) observed that leaf phenolics of the aquatic plant Potamogeton amplifolius were markedly increased by 72% by high light (35% of surface irradiance). The concentration of total phenolic compounds in Potamogeton perfoliatus and M. spicatum branches under sunlight was also higher than that in the shade (Choi et al. 2002). In addition, Bauer et al. (2012) proved that light and microbial treatment reduced the growth rate of cyanobacteria more than dark treatment, but the differences in algal growth were minor. In contrast, under low light intensity, the total phenolic content in the top tissue of M. spicatum was only 3.09% higher than that under high light intensity, but the content of phenolics in the stem was 112.77% higher than that under high light intensity (Ge et al. 2012). The biomass of M. spicatum was dramatically reduced with the decrease in light intensity. This may be due to the decomposition of some allelochemicals under light conditions, resulting in differences in algal inhibition (Nakai et al. 2014).
pH
The existing state of allelochemicals can be altered by a variety of pH levels (Li et al. 2021b). Hence, pH is a crucial influencing factor. The inhibitory effect of Chrysochromulina polylepis on Heterocapsa triquetra was stronger in alkaline growth medium than in neutral or acidic medium (Schmidt and Hansen 2001). Additionally, Noaman et al. (2004) noted that the pH level of the culture medium strongly influenced the growth of Synechococcus leopoliensis as well as the production of secondary metabolites. The allelopathic effect of Corallina pilulifera was most significant when the pH value was 10 (Wang and Tang 2016). In the same way, Yamasaki et al. (2007) also proposed the influence of pH level on allelochemical activity.
Nutritional conditions
Allelopathy is regarded as a novel competitive mechanism among species, and aquatic plants tend to discharge a higher concentration of allelochemicals during times of nutrient scarcity, thus improving their competitiveness against algae (Rice 1984). Mjelde and Faafeng (1997) observed that when essential nutrients are lacking in aquatic systems, phytoplankton may be inhibited by aquatic plants. Similarly, Cagle et al. (2021) determined different parts of P. parvum culture under balanced N:P conditions, unbalanced N:P conditions, and P deficiency conditions. The results showed that the yield of allelochemicals was meager under balanced N:P conditions. However, the allelochemical concentration increases under the conditions of imbalance and P reduction. Similar to this test result, Graneli and Salomon (2010) found that allelopathy may be reduced or completely stopped if the cells of P. parvum grow under balanced N:P conditions. Within 24 h of supplying deficient nutrients to N-deficient or P-deficient cells, allelochemical production of P-deficient cells was reduced to less than half, the same phenomenon as adding inorganic N to N-deficient cells. An increase in the concentration of allelochemicals from the submerged plant M. spicatum could be observed under low phosphorus conditions (Gross 2003).
These studies suggest that nutrient availability in the environment can influence the production of allelochemicals by aquatic organisms and ultimately affect their allelopathic potential.
Temperature
The impact of temperature on the allelopathy of aquatic plants cannot be overstated. It is generally accepted that temperature plays a key role in the production and release of plant allelochemicals, primarily by influencing the enzymatic activity within the plants themselves as well as the rate of diffusion of these chemicals through the water (Li et al. 2021b). The effect of coumarin on M. aeruginosa at varying temperatures was studied by Hong et al. (2018). Compared with the control group (day/night, 25 °C/20 °C), the inhibition rate of algae cells decreased by 63.27% when the temperature increased by 2 °C during the day and decreased by 12.24% when the temperature increased by 2 °C at night. The results of the study demonstrated that the photosynthesis of M. aeruginosa and its resistance to allelochemicals were dramatically enhanced with an increase in temperature. Correspondingly, Nakai et al. (2014) studied the effect of temperature on the inhibitory effect of eight allelochemicals (comprising five polyphenols and three fatty acids) produced by M. spicatum on M. aeruginosa. They revealed that the degree of growth inhibition in M. aeruginosa varied within a temperature range of 20–30 °C, with more significant growth inhibition observed at lower temperatures: at 20 °C, the number of algae cells decreased by 1.9 times as much as that at 30 °C.
Metal ions
Metal ions have specific effects on the allelopathic and algae-inhibiting activities of aquatic plants. Zuo et al. (2012a) observed that heavy metals (Ni, Pb, Cr, and Cu) in aquatic ecosystems reduced the algae-inhibiting potential of the aquatic plant Alternanthera philoxeroides. However, some elements other than heavy metals, i.e., inorganic anions F−, S2−, Cl−, SO42−, and metal cations K+, Na+, Ca2+, Mg2+, Zn2+, total Fe, and total Mn, could improve the algae inhibition potential of A. philoxeroides. The results showed a significant relationship between the allelopathic algae inhibition effect of A. philoxeroides and the concentrations of metal cations, but not inorganic anions, in the aquatic environment.
Freshness
Freshness will affect the activity of allelochemicals secreted by aquatic plants. When freshly harvested aqueous extract of the aquatic plant A. donax L. was co-cultured with M. aeruginosa for 7 days, the maximum percentage inhibitions (PIs) approached 100%. However, the allelopathic activity of the extracts decreased markedly after 2 months of storage, resulting in inhibition rates below 60% in all test groups (Hong et al. 2011). Kang et al. (2020) investigated the effect of extracts from reed (P. australis) and cattail (Typha angustifolia) on the growth of M. aeruginosa in different decomposition stages (fresh and dead). The researchers quantified the effective concentration 50 (EC50) of the extract based on the concentration of dissolved organic carbon (C). The EC50 value of fresh cattail extracts for 100 days was 19.3 mg C/L. According to the results derived from the EC50 and the comparison of growth percentage at the maximum concentration of extracts, it can be concluded that the inhibitory effect of live plants on algae was comparatively more pronounced than that of dead plants.
Biotic factors
Species of aquatic plants and algae
Allelopathy is a phenomenon that is specific to certain species. In other words, the same species of plant or allelochemicals may produce diverse effects on various algal species (Hilt and Gross 2008). Generally, donor organisms may have an impact on multiple target organisms, although not necessarily all of them. Additionally, target organisms possess sensitivity to a range of allelochemicals released by donor species, although not to all of them. Many studies have found that the allelopathy intensity depends on the taxonomic characteristics of donor and recipient species (Poulson-Ellestad et al. 2014; Konarzewska et al. 2020). Sylvers and Gobler (2021) studied the inhibitory effect of three culturable macroalgae, Irish moss (Chondrus crispus), sugar kelp (Saccharina latissima), and Ulva spp., on Alexandrium catenella. Co-culture experiments showed that with the exception of low levels of C. crispus, all species instigated cell lysis and resulted in a decrease in the number of A. catenella compared to the control group, with densities reduced by 17–74% in 2–3 days and 42–96% in similar to 1 week. Among the three seaweed species, S. latissima exhibited the strongest inhibitory effect on A. catenella, followed by Ulva spp. and C. crispus, respectively. The study highlights that different aquatic plant species display varying inhibition abilities against algae. Li and Hu (2005) isolated allelochemicals from the aquatic plant Phragmites communis Trin. that displayed potent inhibition of Chlorella pyrenoidosa and M. aeruginosa but no inhibition of Chlorella vulgaris. The allelopathic effects of freshwater stonewort, Chara australis, and P. crispus on microalgae were examined by Pakdel et al. (2013). The study analyzed the growth of Anabaena variabilis and Scenedesmus quadricauda in the presence and absence of macrophyte extracts, exudates, and live material. The results showed that all treatments had no significant impact on the growth of S. quadricauda but had a significant adverse effect on A. variabilis, with the most substantial effect on C. australis. This phenomenon confirms earlier findings that allelochemicals from macrophytes have specific receptor species. In another study, when the concentration of allelochemicals was 100 mg/L, the algae-inhibiting activity of Ceratophyllum demersum on M. aeruginosa was more potent than that of Vallisneria spiralis (Xian et al. 2006).
Concentration of allelochemicals
Allelopathy of plants can inhibit or promote the growth of algae, which mainly depends on the concentration of secreted allelochemicals. In the study by Xie et al. (2023), the inhibitory effects of polyphenols with different concentration gradients (1 mg/L, 20 mg/L, 50 mg/L, 80 mg/L, 110 mg/L, 140 mg/L) on M. aeruginosa were investigated. The results showed that the algal cell density in the experimental group dramatically decreased with increasing initial polyphenol concentration. The allelopathic effects of aquatic plants on algae were observed to exhibit a relationship where low concentrations promoted growth and high concentrations inhibited growth. Wang et al. (2022) designed two concentration gradients, low-concentration (0.5 g/L and 1 g/L) and high-concentration (5 g/L, 10 g/L, and 20 g/L) extracts, to inhibit M. aeruginosa using the aquatic plant V. spiralis as the source of allelochemicals. Algal cell growth showed completely different trends between the low- and high-concentration treatments, indicating that allelochemicals from V. spiralis had low-concentration promotion and high-concentration inhibition on M. aeruginosa.
Growth phase
The sensitivity of algae to the allelopathy of aquatic plants is subject to variation based on the growth phase. It was observed that the allelochemicals of the aquatic plant P. communis inhibited M. aeruginosa more strongly at the initial growth stage (lag phase) than at the rapid growth stage (logarithmic phase) (Li and Hu 2005). Similarly, the growth stage of aquatic plants affects their allelopathy. A study indicates that aquatic plants show higher allelopathic activity during seedling stage (Sun et al. 2021a).
Different organs and tissues of aquatic plants
The variations in the distribution of allelochemicals among aquatic plants lead to disparate types and quantities of allelochemicals present within distinct plant tissues and organs. This, in turn, gives rise to differences in the effectiveness of algae inhibition. Chen et al. (2012) found that all tissues from eight tested macrophytes could markedly inhibit the growth of M. aeruginosa, in which the leaves of the aquatic plant N. tetragona and N. nucifera, two of the tested plants, exhibited higher activity than petioles.
Different extraction solvents
Extracting the same parts of aquatic plants with different solvents can also result in differences in the type and amount of allelochemicals. Ghobrial et al. (2015) employed aqueous extracts containing solvents of 50% and 100% acetone and ethanol to investigate the impact on M. aeruginosa and Oscillatoria tenuis of Potamogeton pectinatus and C. demersum. The results revealed that the aqueous extracts of 50% and 100% acetone and ethanol produced different effects on the growth of algal cells. The ethanol extract showed a more substantial inhibitory effect than the 50% acetone extract. The strongest inhibitory effect of the 50% acetone extract of Ceratophyllum against O. tenuis was 1.05 mg/L, with the highest percentage rate (R) of 169.4. The highest inhibitory rate of the 50% ethanolic extract of Ceratophyllum against O. tenuis was 0.0083 mg/L, with an R of 74.32. In a study by Haroon and Abdel-Aal (2016), the chemical composition and anti-algal activity of crude extracts obtained from aquatic plants, namely, P. crispus and M. spicatum, were investigated. In this regard, various organic solvents with different polarities, such as petroleum ether, methylene chloride, chloroform, acetone, and methanol, were employed for the extraction process. The findings of the study indicated that the phytochemical composition and mass fraction varied significantly based on the extraction solvents and plant species. Among the different extracts evaluated, it was observed that the mixed extracts and chloroform extracts of the two plants demonstrated the most promising anti-algal activity.
Utilizing modes of allelochemicals for algae control
Growing aquatic plants
The rapid increase in nitrogen and phosphorus in water will cause eutrophication of the water, which poses a great threat to aquatic ecosystems and human health. Planting aquatic plants can effectively inhibit algal reproduction. Planting aquatic plants in water is often used for the construction of ecological floating beds (EFBs).
EFBs refer to an ecological technology for purifying water bodies floating on the surface of the water, mainly consisting of aquatic plants and taking macromolecule materials or inorganic nonmetallic materials as carriers and bases (Samal et al. 2019). Aquatic plants in the EFBs provide attachment sites for microorganisms through developed roots (Bi et al. 2019), secrete allelochemicals and purify eutrophic water bodies through absorption, competition, and shading. A 3-month experiment was conducted in a 326 m2 lake located in the northwest corner of Xi’an (34°27′ N, 108°93′ E) Fengqing Park. The EFBs system uses calamus, iris, lythum, and Hydrocotyle vulgaris as vegetation and zeolite and sponge iron as fillers. The results showed a noteworthy decrease in the mean concentration of Chl-a and the density of algae (Wang et al. 2018a). Simultaneously, Svanys et al. (2014) observed that M. spicatum had consistent negative effects on M. aeruginosa biomass through mesocosm experiments in the Curonian Lagoon. Although planting aquatic plants in EFBs may effectively control the growth of algae, aquatic plants are vulnerable to low-temperature weather, their growth state is difficult to control, and dead plants are liable to cause secondary pollution to the water.
Addition of allelochemicals sustained-release microspheres (AC-SMs)
To solve the shortcomings of direct planting of aquatic plants, a method of extracting allelochemicals from aquatic plants and adding them artificially was proposed. In natural waters, aquatic plants continuously release allelochemicals into the water (Bauer et al. 2009). The use of AC-SMs aims to simulate the natural release process of allelochemicals to provide the possibility for the practical application of allelochemicals.
AC-SMs are prepared from allelochemicals and natural materials. The most commonly used preparation method is to mix allelochemicals and sodium alginate in distilled water as the basic material, then use an aqueous acetic acid solution containing chitosan and calcium chloride as the coagulation fluid, and finally add the basic material dropwise into the coagulation fluid to obtain beads. Chitosan and sodium alginate are two nontoxic and biodegradable natural polysaccharides (Rescignano et al. 2015). Li et al. (2023a) discovered that AC-SMs not only effectively reduced the toxicity of allelochemicals but also enhanced the ingestion, incorporation, and digestion abilities of Daphnia magna Straus. Moreover, the combination of chitosan and sodium alginate guarantees high encapsulation efficiency and good release performance of AC-SMs. The SMs of DHF prepared by Huang et al. (2016) showed a high drug-loading rate (47.18%) and encapsulation efficiency (67.65%). The theoretical release time was approximately 120 days, which has a long-term inhibitory effect on M. aeruginosa (>30 days). Linoleic acid SMs not only has high encapsulation efficiency (up to 62%) but also has good thermal stability (decomposition temperature of 236 °C) (Ni et al. 2015). In the process of algae inhibition by AC-SMs, Ni et al. (2013) observed that artemisinin SMs reduced the content of Chl-a and soluble protein in 30 days and increased the superoxide dismutase activity of M. aeruginosa. Additionally, AC-SMs dramatically reduced the content of phycobiliprotein in M. aeruginosa, but the stressed algae cells still had complete cell membrane structure, which indicated that the algae cells may undergo PCD (Sang et al. 2022). Additionally, according to the comparative proteomic analysis conducted by Li et al. (2021a), the SMs of luteolin markedly upregulated photosynthesis and protein homeostasis while downregulating the stress response, transcription, translation, transport, cell shaping, and cell division. This disruption negatively impacts the physiological and metabolic processes of the organism and impedes its growth. The primary inhibitory mechanism of artemisinin algaecides involves irreversible damage to the cell membrane. Jiang et al. (2022) noted that lipid peroxidation occurred in algal cell membranes after treatment with artemisinin SMs, and the permeability of the membrane increased. Similarly, Ni et al. (2018) noted that disruption of algal cell membranes was the main mechanism of linoleic acid SMs. The algae-inhibiting effect of AC-SMs may be related to particle size. The inhibitory effect was found to be more significant when the particle size of artemisinin sustained-release microspheres (ASMs) was reduced to 2/10000 in comparison to artemisinin sustained-release granules (ASGs). It is possible that the particulate dimensions of ASMs are comparatively inferior to those of ASGs, which dramatically increases the effective contact area with M. aeruginosa and reduces the swelling rate of the sustained-release algal inhibitor (Jiang et al. 2022). Researchers have found that using AC-SMs before recruiting cyanobacteria would be more effective (Huang et al. 2016; Ni et al. 2020).
Summary and outlook
Allelopathy of aquatic plants has certain advantages in limiting water eutrophication caused by algal blooms, which provides a new idea for the remediation of eutrophication. Previous studies have mainly focused on the sources and types of allelochemicals, the separation of allelochemicals, the factors affecting allelochemicals, and the inhibition mechanism of allelochemicals from aquatic plants on algae, in addition to achieving some research results. However, there are currently numerous research endeavors that remain to be conducted with a view to achieving significant breakthroughs:
-
(1)
Currently, research on the effects of aquatic plants on algae remains mostly at the cellular level; while genetic level research mostly focuses on protein synthesis, other levels of research still need to be explored and discovered.
-
(2)
Most of the current studies are only at the laboratory level. However, in practical applications, the allelochemicals released by plants are located in complex and diverse environments. It is not possible to determine whether allelochemicals would still exert algal inhibition under multiple factors in the same or similar way as under laboratory conditions.
-
(3)
Allelochemicals with algae inhibitory activity have been widely recognized by research scholars, but their manufacture has not yet been scaled up, thus leading to high costs in application, which would be combined with other treatments, such as photocatalysis and UV irradiation, to make future growth and manufacture more scaled up to reduce application costs.
In conclusion, it is an eco-friendly water treatment method to use allelopathy in aquatic plants to inhibit algae. Currently, the research has made some achievements, but it still needs to be systematically studied in multiple aspects and at a deep level to be applied in the current environment.
Data availability
Not applicable.
References
Abadie E, Chiantella C, Crottier A, Rhodes L, Masseret E, Berteaux T, Laabir M (2018) What are the main environmental factors driving the development of the neurotoxic dinoflagellate Vulcanodinium rugosum in a Mediterranean ecosystem (Ingril lagoon, France)? Harmful Algae 75:75–86. https://doi.org/10.1016/j.hal.2018.03.012
Bajwa AA (2014) Sustainable weed management in conservation agriculture. Crop Protect 65:105–113. https://doi.org/10.1016/j.cropro.2014.07.014
Bauer N, Blaschke U, Beutler E, Gross EM, Jenett-Siems K, Siems K, Hilt S (2009) Seasonal and interannual dynamics of polyphenols in Myriophyllum verticillatum and their allelopathic activity on Anabaena variabilis. Aquat Bot 91(2):110–116. https://doi.org/10.1016/j.aquabot.2009.03.005
Bauer N, Zwirnmann E, Grossart HP, Hilt S (2012) Transformation and allelopathy of natural dissolved organic carbon and tannic acid are affected by solar radiation and bacteria. J Phycol 48(2):355–364. https://doi.org/10.1111/j.1529-8817.2012.01134.x
Bi R, Zhou C, Jia Y, Wang S, Li P, Reichwaldt ES, Liu W (2019) Giving waterbodies the treatment they need: a critical review of the application of constructed floating wetlands. J Environ Manage 238:484–498. https://doi.org/10.1016/j.jenvman.2019.02.064
Bidle KD (2016) Programmed cell death in unicellular phytoplankton. Curr Biol 26(13):R594–R607. https://doi.org/10.1016/j.cub.2016.05.056
Cagle SE, Roelke DL, Muhl RW (2021) Allelopathy and micropredation paradigms reconcile with system stoichiometry. Ecosphere 12(2). https://doi.org/10.1002/ecs2.3372
Chaïb S, Pistevos JCA, Bertrand C, Bonnard I (2021) Allelopathy and allelochemicals from microalgae: an innovative source for bio-herbicidal compounds and biocontrol research. Algal Res 54:102213. https://doi.org/10.1016/j.algal.2021.102213
Chao Z (2013) Algicidal activity of Salvia miltiorrhiza Bung on Microcystis aeruginosa--towards identification of algicidal substance and determination of inhibition mechanism. Chemosphere 93(6):997–1004. https://doi.org/10.1016/j.chemosphere.2013.05.068
Chavan P, Kumar R, Kirubagaran R, Venugopalan VP (2017) Comparative toxicological effects of two antifouling biocides on the marine diatom Chaetoceros lorenzianus: damage and post-exposure recovery. Ecotoxicol Environ Saf 144:97–106. https://doi.org/10.1016/j.ecoenv.2017.06.001
Chen J, Zhang H, Han Z, Ye J, Liu Z (2012) The influence of aquatic macrophytes on Microcystis aeruginosa growth. Ecol Eng 42:130–133. https://doi.org/10.1016/j.ecoleng.2012.02.021
Cheng R, Hou S, Wang J, Zhu H, Shutes B, Yan B (2022) Biochar-amended constructed wetlands for eutrophication control and microcystin (MC-LR) removal. Chemosphere 295:133830. https://doi.org/10.1016/j.chemosphere.2022.133830
Choi C, Bareiss C, Walenciak O, Gross EM (2002) Impact of polyphenols on the growth of the aquatic herbivore Acentria ephemerella (Lepidoptera: Pyralidae). J Chem Ecol 28(11):2245–2256. https://doi.org/10.1023/A:1021049332410
Cronin G, Lodge DM (2003) Effects of light and nutrient availability on the growth, allocation, carbon/nitrogen balance, phenolic chemistry, and resistance to herbivory of two freshwater macrophytes. Oecologia 137(1):32–41. https://doi.org/10.1007/s00442-003-1315-3
Dick GJ, Duhaime MB, Evans JT, Errera RM, Godwin CM, Kharbush JJ, Nitschky HS, Powers MA, Vanderploeg HA, Schmidt KC, Smith DJ, Yancey CE, Zwiers CC, Denef VJ (2021) The genetic and ecophysiological diversity of Microcystis. Environ Microbiol 23(12):7278–7313. https://doi.org/10.1111/1462-2920.15615
El-Darier SM, Metwally A-F K, Nasser AW, Taha HM (2021) Biointerference relationship between the macroalga Ulva lactuca and two green microalgae. Egypt J Aquat Res 47(2):163–169. https://doi.org/10.1016/j.ejar.2021.03.002
Fa M, Molinillo JM, Varela RM, Galindo JC (2010) Allelopathy--a natural alternative for weed control. Pest Manage Sci 63(4):327–348. https://doi.org/10.1002/ps.1342
Fan G, Cai C, Chen Z, Luo J, Du B, Yang S, Wu J (2023) Visible-light-drivenself-floating Ag2MoO4/TACN@LF photocatalyst inactivation of Microcystis aeruginosa: performance and mechanisms. J Hazard Mater 441:129932. https://doi.org/10.1016/j.jhazmat.2022.129932
Feist SM, Lance RF (2021) Genetic detection of freshwater harmful algal blooms: a review focused on the use of environmental DNA (eDNA) in Microcystis aeruginosa and Prymnesium parvum. Harmful Algae 110:102124. https://doi.org/10.1016/j.hal.2021.102124
Fink P (2007) Ecological functions of volatile organic compounds in aquatic systems. Mar Freshwater Behav Physiol 40(3):155–168. https://doi.org/10.1080/10236240701602218
Gao YN, Liu BY, Xu D, Zhou QH, Hu CY, Ge FJ, Zhang LP, Wu ZB (2011) Phenolic compounds exuded from two submerged freshwater macrophytes and their allelopathic effects on Microcystis aeruginosa. Pol J Environ Stud 20(5):1153–1159. https://doi.org/10.1080/01919512.2011.604764
Ge F, Liu B, Lu Z, Gao Y, Wu Z (2012) Effects of light on growth and phenolic contents of Myriophyllum spicatum. Environ Sci Technol 35(3):30–34. https://doi.org/10.3969/j.issn.1003-6504.2012.03.007
Ghobrial MG, Nassr HS, Kamil AW (2015) Bioactivity effect of two macrophyte extracts on growth performance of two bloom-forming cyanophytes. Egypt J Aquat Res 41(1):69–81. https://doi.org/10.1016/j.ejar.2015.01.001
Graneli E, Salomon PS (2010) Factors influencing allelopathy and toxicity in Prymnesium parvum. J Am Water Resour Assoc 46(1):108–120. https://doi.org/10.1111/j.1752-1688.2009.00395.x
Gross EM (2003) Differential response of tellimagrandin II and total bioactive hydrolysable tannins in an aquatic angiosperm to changes in light and nitrogen. Oikos 103(3):497–504. https://doi.org/10.1034/j.1600-0706.2003.12666.x
Gross EM, Hilt S, Lombardo P, Mulderij G (2007) Searching for allelopathic effects of submerged macrophytes on phytoplankton - state of the art and open questions. Hydrobiologia 584:77–88. https://doi.org/10.1007/s10750-007-0591-z
Guo Y, Liu Y, Zeng G, Hu X, Li X, Huang D, Liu Y, Yin Y (2014) A restoration-promoting integrated floating bed and its experimental performance in eutrophication remediation. J Environ Sci 26(5):1090–1098. https://doi.org/10.1016/S1001-0742(13)60500-8
Haroon AM, Abdel-Aal EI (2016) Chemical composition and in vitro anti-algal activity of Potamogeton crispus and Myriophyllum spicatum extracts. Egypt J Aquat Res 42(4):393–404. https://doi.org/10.1016/j.ejar.2016.12.002
Henriques FS (2009) Leaf chlorophyll fluorescence: background and fundamentals for plant biologists. Bot Rev 75(3):249–270. https://doi.org/10.1007/s12229-009-9035-y
Hilt S, Gross EM (2008) Can allelopathically active submerged macrophytes stabilise clear-water states in shallow lakes? Basic Appl Ecol 9(4):422–432. https://doi.org/10.1016/j.baae.2007.04.003
Hong WX, Zuo SP, Ye LT, Qin BQ (2018) Asymmetric warming enhances Microcystis aeruginosa resistance to macrophytic anti-cyanobacterial coumarin. Allelopathy J 43(2):265–274. https://doi.org/10.26651/allelo.j./2018-43-2-1146
Hong Y, Hu HY, Sakoda A, Sagehashi M (2011) Straw preservation effects of Arundo donax L. on its allelopathic activity to toxic and bloom-forming Microcystis aeruginosa. Water Sci Technol 63(8):1566–1573. https://doi.org/10.2166/wst.2011.209
Hongying HU, Hong Y (2008) Algal-bloom control by allelopathy of aquatic macrophytes—a review. Front Environ Sci Eng 2(4):18. https://doi.org/10.1007/s11783-008-0070-4
Huang H, Xiao X, Lin F, Grossart H-P, Nie Z, Sun L, Xu C, Shi J (2016) Continuous-release beads of natural allelochemicals for the long-term control of cyanobacterial growth: preparation, release dynamics and inhibitory effects. Water Res 95:113–123. https://doi.org/10.1016/j.watres.2016.02.058
Huang HM, Xiao X, Ghadouani A, Wu JP, Nie ZY, Peng C, Xu XH, Shi JY (2015) Effects of natural flavonoids on photosynthetic activity and cell integrity in Microcystis aeruginosa. Toxins 7(1):66–80. https://doi.org/10.3390/toxins7010066
Jia Y, Han G, Wang C, Guo P, Jiang W, Li X, Tian X (2010) The efficacy and mechanisms of fungal suppression of freshwater harmful algal bloom species. J Hazard Mater 183(1):176–181. https://doi.org/10.1016/j.jhazmat.2010.07.009
Jiang ZY, Ni LX, Li XL, Xu C, Chen XQ, Li SY (2022) Mechanistic insight into the inhibitory effect of artemisinin sustained-release inhibitors with different particle sizes on Microcystis aeruginosa. Environ Sci Pollut Res 29(58):87545–87554. https://doi.org/10.1007/s11356-022-21534-x
Kang PG, Hong J, Kim E, Kim B (2020) Effects of extracts of reed and cattail on the growth of a cyanobacterium, Microcystis aeruginosa. J Freshwater Ecol 11:1–12. https://doi.org/10.1080/02705060.2020.1748128
Kibuye FA, Zamyadi A, Wert EC (2021) A critical review on operation and performance of source water control strategies for cyanobacterial blooms: part II-mechanical and biological control methods. Harmful Algae 109:102119. https://doi.org/10.1016/j.hal.2021.102119
Kirpenko NI, Usenko OM (2013) Influence of higher aquatic plants on microalgae (a review). Hydrobiol J 49(2):57–74. https://doi.org/10.1615/HydrobJ.v49.i2.60
Klemenčič M, Funk C (2018) Structural and functional diversity of caspase homologues in non-metazoan organisms. Protoplasma 255(1):387–397. https://doi.org/10.1007/s00709-017-1145-5
Konarzewska Z, Śliwińska-Wilczewska S, Felpeto AB, Vasconcelos V, Latała A (2020) Assessment of the allelochemical activity and biochemical profile of different phenotypes of picocyanobacteria from the genus Synechococcus. Mar Drugs 18(4):175. https://doi.org/10.33390/md18040179
Kong Y, Peng Y, Zhang Z, Zhang M, Zhou Y, Duan Z (2019) Removal of Microcystis aeruginosa by ultrasound: inactivation mechanism and release of algal organic matter. Ultrason Sonochem 56:447–457. https://doi.org/10.1016/j.ultsonch.2019.04.017
Legrand C, Rengefors K, Fistarol GO, Granéli E (2003) Allelopathy in phytoplankton - biochemical, ecological and evolutionary aspects. Phycologia 42(4):406–419. https://doi.org/10.2216/i0031-8884-42-4-406.1
Leu E, Krieger-Liszkay A, Goussias C, Gross EM (2002) Polyphenolic allelochemicals from the aquatic angiosperm Myriophyllum spicatum inhibit photosystem II. Plant Physiol 130(4):2011–2018. https://doi.org/10.1104/pp.011593
Li B, Li J, An G, Zhao C, Wang C (2021a) Long-term and strong suppression against Microcystis growth and microcystin-release by luteolin continuous-release microsphere: optimal construction, characterization, effects and proteomic mechanisms. Water Res 202:117448. https://doi.org/10.1016/j.watres.2021.117448
Li B, Yin Y, Kang L, Feng L, Liu Y, Du Z, Tian Y, Zhang L (2021b) A review: application of allelochemicals in water ecological restoration——algal inhibition. Chemosphere 267:128869. https://doi.org/10.1016/j.chemosphere.2020.128869
Li B, Yin Y, Zhou X, Feng L, Liu Y, Du Z, Tian Y, Zhang L (2023a) Effect of allelochemicals sustained-release microspheres on the ingestion, incorporation, and digestion abilities of Daphnia magna Straus. J Environ Sci 124:205–214. https://doi.org/10.1016/j.jes.2021.10.020
Li FM, Hu HY (2005) Isolation and characterization of a novel antialgal allelochemical from Phragmites communis. Appl Environ Microbiol 71(11):6545–6553. https://doi.org/10.1128/AEM.71.11.6545-6553.2005
Li F-M, Hu H-Y, Chong Y-X, Men Y-J, Guo M-T (2007) Effects of allelochemical EMA isolated from Phragmites communis on algal cell membrane lipid and ultrastructure. Environ Sci 28(7):1534–1538. https://doi.org/10.13227/j.hjkx.2007.07.022
Li W, Guo J, Han X, Da X, Wang K, Zhao H, Huang S-T, Li B, He H, Jiang R, Zhou S, Yan P, Chen T, He Y, Xu J, Liu Y, Wu Y, Shou H, Wu Z et al (2023b) A novel protein domain is important for photosystem II complex assembly and photoautotrophic growth in angiosperms. Mol Plant 16(2):374–392. https://doi.org/10.1016/j.molp.2022.12.016
Ling C, Trick CG (2010) Expression and standardized measurement of hemolytic activity in Heterosigma akashiwo. Harmful Algae 9(5):522–529. https://doi.org/10.1016/j.hal.2010.04.004
Liu F, Zhang C, Wang Y, Chen G (2022) A review of the current and emerging detection methods of marine harmful microalgae. Sci Total Environ 815:152913. https://doi.org/10.1016/j.scitotenv.2022.152913
Lockshin RA, Zakeri Z (2004) Apoptosis, autophagy, and more. Int J Biochem Cell Biol 36(12):2405–2419. https://doi.org/10.1016/j.biocel.2004.04.011
Long M, Peltekis A, González-Fernández C, Hégaret H, Bailleul B (2021) Allelochemicals of Alexandrium minutum: kinetics of membrane disruption and photosynthesis inhibition in a co-occurring diatom. Harmful Algae 103:101997. https://doi.org/10.1016/j.hal.2021.101997
Lu Z, Sha J, Tian Y, Zhang X, Liu B, Wu Z (2017) Polyphenolic allelochemical pyrogallic acid induces caspase-3(like)-dependent programmed cell death in the cyanobacterium Microcystis aeruginosa. Algal Res 21:148–155. https://doi.org/10.1016/j.algal.2016.11.007
Men Y, Hu H, Li F (2006) Effects of an allelopathic fraction from Phragmites communis Trin on the growth characteristics of Scenedesmus obliquus. Ecol Environ Sci 05:925–929. https://doi.org/10.16258/j.cnki.1674-5906.2006.05.007
Mjelde M, Faafeng BA (1997) Ceratophyllum demersum hampers phytoplankton development in some small Norwegian lakes over a wide range of phosphorus concentrations and geographical latitude. Freshwater Biol 37(2):355–365. https://doi.org/10.1046/j.1365-2427.1997.00159.x
Mohamed ZA (2017) Macrophytes-cyanobacteria allelopathic interactions and their implications for water resources management—a review. Limnologica 63:122–132. https://doi.org/10.1016/j.limno.2017.02.006
Mohamed ZA, Alamri SA (2012) Biodegradation of cylindrospermopsin toxin by microcystin-degrading bacteria isolated from cyanobacterial blooms. Toxicon 60(8):1390–1395. https://doi.org/10.1016/j.toxicon.2012.10.004
Molisch H (1937) Der einfluss einer pflanze auf die andere allelopathie. Nature 141:493. https://doi.org/10.1038/141493a0
Nakai S, Asaoka S, Okuda T, Nishijima W (2014) Growth inhibition of microcystis aeruginosa by allelopathic compounds originally isolated from Myriophyllum spicatum: temperature and light effects and evidence of possible major mechanisms. J Chem Eng Jpn 47(6):488–493. https://doi.org/10.1252/jcej.13we253
Nakai S, Inoue Y, Hosomi M, Murakami A (1999) Growth inhibition of blue–green algae by allelopathic effects of macrophytes. Water Sci Technol 39(8):47–53. https://doi.org/10.1016/S0273-1223(99)00185-7
Ni L, Acharya K, Ren G, Li S, Li Y, Li Y (2013) Preparation and characterization of anti-algal sustained-release granules and their inhibitory effects on algae. Chemosphere 91(5):608–615. https://doi.org/10.1016/j.chemosphere.2012.12.064
Ni L, Jie X, Wang P, Li S, Hu S, Li Y, Li Y, Acharya K (2015) Characterization of unsaturated fatty acid sustained-release microspheres for long-term algal inhibition. Chemosphere 120:383–390. https://doi.org/10.1016/j.chemosphere.2014.07.098
Ni L, Rong S, Gu G, Hu L, Wang P, Li D, Yue F, Wang N, Wu H, Li S (2018) Inhibitory effect and mechanism of linoleic acid sustained-release microspheres on Microcystis aeruginosa at different growth phases. Chemosphere 212:654–661. https://doi.org/10.1016/j.chemosphere.2018.08.045
Ni LX, Yue FF, Zhang JH, Rong SY, Liu XY, Wang YF, Wang PF, Li DY, Wang N, Wu HQ, Li SY (2020) Cell membrane damage induced by continuous stress of artemisinin sustained-release microspheres (ASMs) on Microcystis aeruginosa at different physiological stages. Environ Sci Pollut Res 27(11):12624–12634. https://doi.org/10.1007/s11356-020-07813-5
Noaman NH, Fattah A, Khaleafa M, Zaky SH (2004) Factors affecting antimicrobial activity of Synechococcus leopoliensis. Microbiol Res 159(4):395–402. https://doi.org/10.1016/j.micres.2004.09.001
Pakdel FM, Sim L, Bearda J, Davis J (2013) Allelopathic inhibition of microalgae by the freshwater stonewort, Chara australis, and a. submerged angiosperm, Potamogeton crispus. Aquat Bot 110:24–30. https://doi.org/10.1016/j.aquabot.2013.04.005
Poulson-Ellestad KL, Jones CM, Roy J, Viant MR, Fernandez FM, Kubanek J, Nunn BL (2014) Metabolomics and proteomics reveal impacts of chemically mediated competition on marine plankton. P Proc Natl Acad Sci USA 111(24):9009–9014. https://doi.org/10.1073/pnas.1402130111
Preiner S, Dai Y, Pucher M, Reitsema RE, Schoelynck J, Meire P, Hein T (2020) Effects of macrophytes on ecosystem metabolism and net nutrient uptake in a groundwater fed lowland river. Sci Total Environ 721:137620. https://doi.org/10.1016/j.scitotenv.2020.137620
Qi F, Zhang C, Jiang S, Wang Q, Kuerban K, Luo M, Dong M, Zhou X, Wu L, Jiang B, Ye L (2019a) S-ethyl ethanethiosulfinate, a derivative of allicin, induces metacaspase-dependent apoptosis through ROS generation in Penicillium chrysogenum. Biosci Rep 39(6):BSR20190167. https://doi.org/10.1042/BSR20190167
Qi X, Xin J, Li W, Tian R (2019b) Research advance on allelopathy and its inhibitory effects of algae photosynthetics. Environ Sci Technol 42(04):43–52. https://doi.org/10.19672/j.cnki.1003-6504.2019.04.008
Qian K, Ma Z, Li R, Chen B, Wang M, Zhu S, Rong R, Qin W (2021) Progress in the study of allelopathy in plants: a case study of inhibiting Microcystis aeruginosa growth. Biotechnol Bull 37(04):177–193. https://doi.org/10.13560/j.cnki.biotech.bull.1985.2020-1158
Qian Y-P, Li X-T, Tian R-N (2019) Effects of aqueous extracts from the rhizome of Pontederia cordata on the growth and interspecific competition of two algal species. Ecotoxicol Environ Saf 168:401–407. https://doi.org/10.1016/j.ecoenv.2018.10.086
Rescignano N, Fortunati E, Armentano I, Hernandez R, Mijangos C, Pasquino R, Kenny JM (2015) Use of alginate, chitosan and cellulose nanocrystals as emulsion stabilizers in the synthesis of biodegradable polymeric nanoparticles. J Colloid Interface Sci 445:31–39. https://doi.org/10.1016/j.jcis.2014.12.032
Rice EL (1974) Allelopathy. Academic Press
Rice EL (1984) Allelopathy 2nd. Academic Press, San Diego
Samal K, Kar S, Trivedi S (2019) Ecological floating bed (EFB) for decontamination of polluted water bodies: design, mechanism and performance. J Environ Manage 251:109550. https://doi.org/10.1016/j.jenvman.2019.109550
Sang WL, Du CH, Liu XG, Ni LX, Li SY, Xu JW, Chen XQ, Xu J, Xu C (2022) Effect of artemisinin sustained-release algaecide on the growth of Microcystis aeruginosa and the underlying physiological mechanisms. RSC Adv 12(25):16094–16104. https://doi.org/10.1039/d2ra00065b
Schmidt LE, Hansen PJ (2001) Allelopathy in the prymnesiophyte Chrysochromulina polylepis: effect of cell concentration, growth phase and pH. Mar Ecol: Prog Ser 216:67–81. https://doi.org/10.3354/meps216067
Shao JH, Wu XQ, Li RH (2010) Physiological responses of Microcystis aeruginosa PCC7806 to nonanoic acid stress. Environ Toxicol 24(6):610–617. https://doi.org/10.1002/tox.20462
Staszek P, Krasuska U, Ciacka K, Gniazdowska A (2021) ROS metabolism perturbation as an element of mode of action of allelochemicals. Antioxidants. https://doi.org/10.3390/antiox10111648
Sun LH, Chang YJ, Han SQ, Cui J, Liu XJ, Yao DR (2021a) Metabolomics analysis of bioactive compounds in culture water planting floating bed water dropwort in different development stages. Res Environ Sci 34(08):1860–1875. https://doi.org/10.13198/j.issn.1001-6929.2021.03.04
Sun P, Hui C, Bai N, Yang S, Wan L, Zhang Q, Zhao Y (2015) Revealing the characteristics of a novel bioflocculant and its flocculation performance in Microcystis aeruginosa removal. Sci Rep 5(1):17465. https://doi.org/10.1038/srep17465
Sun S, Hu S, Zhang B, Sun X, Xu N (2021b) Allelopathic effects and potential allelochemical of Sargassum fusiforme on red tide microalgae Heterosigma akashiwo. Mar Pollut Bull 170:112673. https://doi.org/10.1016/j.marpolbul.2021.112673
Suresh K, Tang T, Van Vliet MTH, Bierkens MFP, Strokal M, Sorger-Domenigg F, Wada Y (2023) Recent advancement in water quality indicators for eutrophication in global freshwater lakes. Environ Res Lett 18(6). https://doi.org/10.1088/1748-9326/acd071
Svanys A, Paskauskas R, Hilt S (2014) Effects of the allelopathically active macrophyte Myriophyllum spicatum on a natural phytoplankton community: a mesocosm study. Hydrobiologia 737(1):57–66. https://doi.org/10.1007/s10750-013-1782-4
Sylvers LH, Gobler CJ (2021) Mitigation of harmful algal blooms caused by Alexandrium catenella and reduction in saxitoxin accumulation in bivalves using cultivable seaweeds. Harmful Algae 105:102056. https://doi.org/10.1016/j.hal.2021.102056
Tan KT, Huang ZQ, Ji RB, Qiu YT, Wang ZH, Liu JX (2019) A review of allelopathy on microalgae. Microbiol-SGM 165(6):587–592. https://doi.org/10.1099/mic.0.000776
Tang YZ, Gobler CJ (2011) The green macroalga, Ulva lactuca, inhibits the growth of seven common harmful algal bloom species via allelopathy. Harmful Algae 10(5):480–488. https://doi.org/10.1016/j.hal.2011.03.003
Taylor RB, Hill BN, Langan LM, Chambliss CK, Brooks BW (2021) Sunlight concurrently reduces Prymnesium parvum elicited acute toxicity to fish and prymnesins. Chemosphere 263:127927. https://doi.org/10.1016/j.chemosphere.2020.127927
Techer D, Fontaine P, Personne A, Viot S, Thomas M (2016) Allelopathic potential and ecotoxicity evaluation of gallic and nonanoic acids to prevent cyanobacterial growth in lentic systems: a preliminary mesocosm study. Sci Total Environ 547:157–165. https://doi.org/10.1016/j.scitotenv.2015.12.164
Wang F, Zhao W, Chen J, Zhou Y (2022) Allelopathic inhibitory effect on the growth of microcystis aeruginosa by improved ultrasonic-cellulase extract of Vallisneria. Chemosphere 298:134245. https://doi.org/10.1016/j.chemosphere.2022.134245
Wang RJ, Tang XX (2016) Allelopathic effects of macroalga Corallina pilulifera on the red-tide forming alga Heterosigma akashiwo under laboratory conditions. Chin J Oceanol Limnol 34(2):314–321. https://doi.org/10.1007/s00343-015-4336-y
Wang W-H, Wang Y, Li Z, Wei C-Z, Zhao J-C, Sun L-Q (2018a) Effect of a strengthened ecological floating bed on the purification of urban landscape water supplied with reclaimed water. Sci Total Environ 622:1630–1639. https://doi.org/10.1016/j.scitotenv.2017.10.035
Wang Y, Liu Q, Wei Z, Liu N, Li Y, Li D, Jin Z, Xu X (2018b) Thiazole amides, a novel class of algaecides against freshwater harmful algae. Sci Rep 8(1):8555. https://doi.org/10.1038/s41598-018-26911-6
Wu X, Wu H, Chen J, Ye J (2013a) Effects of allelochemical extracted from water lettuce (Pistia stratiotes Linn.) on the growth, microcystin production and release of Microcystis aeruginosa. Environ Sci Pollut Res 20(11):8192–8201. https://doi.org/10.1007/s11356-013-1783-x
Wu X, Wu H, Wang S, Wang Y, Zhang R, Hu X, Ye J (2018) Effect of propionamide on the growth of Microcystis flos-aquae colonies and the underlying physiological mechanisms. Sci Total Environ 630:526–535. https://doi.org/10.1016/j.scitotenv.2018.02.217
Wu Z, Shi J, Yang S (2013b) The effect of pyrogallic acid on growth, oxidative stress, and gene expression in Cylindrospermopsis raciborskii (Cyanobacteria). Ecotoxicology 22(2):271–278. https://doi.org/10.1007/s10646-012-1023-z
Xian Q, Chen H, Zou H, Yin D (2006) Allelopathic activity of volatile substance from submerged macrophytes on Microcystin aeruginosa. Acta Ecol Sinica 26(11):3549–3554. https://doi.org/10.1016/S1872-2032(06)60054-1
Xie L, Ma Z, Yang G, Huang Y, Wen T, Deng Y, Sun J, Zheng S, Wu F, Huang K, Shao J (2023) Study on the inhibition mechanism of eucalyptus tannins against Microcystis aeruginosa. Ecotoxicol Environ Saf 249:114452. https://doi.org/10.1016/j.ecoenv.2022.114452
Xu C, Huang S, Huang Y, Effiong K, Yu S, Hu J, Xiao X (2020) New insights into the harmful algae inhibition by Spartina alterniflora: cellular physiology and metabolism of extracellular secretion. Sci Total Environ 714:136737. https://doi.org/10.1016/j.scitotenv.2020.136737
Xu C, Yu S, Hu J, Effiong K, Ge Z, Tang T, Xiao X (2022) Programmed cell death process in freshwater Microcystis aeruginosa and marine Phaeocystis globosa induced by a plant derived allelochemical. Sci Total Environ 838:156055. https://doi.org/10.1016/j.scitotenv.2022.156055
Xuan TD, Shinkichi T, Khanh TD, Chung IM (2005) Biological control of weeds and plant pathogens in paddy rice by exploiting plant allelopathy: an overview. Crop Prot 24(3):197–206. https://doi.org/10.1016/j.cropro.2004.08.004
Yamasaki Y, Nagasoe S, Matsubara T, Shikata T, Shimasaki Y, Oshima Y, Honjo T (2007) Allelopathic interactions between the bacillariophyte Skeletonema costatum and the raphidophyte Heterosigma akashiwo. Mar Ecol: Prog Ser 339:83–92. https://doi.org/10.3354/meps339083
Yang Y, Chen H, Lu J (2023) Inactivation of algae by visible-light-driven modified photocatalysts: a review. Sci Total Environ 858:159640. https://doi.org/10.1016/j.scitotenv.2022.159640
Zhang C, Wang Y, Guo C, Chen G, Kan G, Cai P, Zhou J (2018) Comparison of loop-mediated isothermal amplification with hyperbranched rolling circle amplification as a simple detection method for Heterosigma akashiwo. Harmful Algae 73:1–11. https://doi.org/10.1016/j.hal.2018.01.005
Zhang H, Li X, Wu D, Yu B, Lu S, Wang J, Ding J (2023) A novel strategy for efficient capture of intact harmful algal cells using Zinc oxide modified carbon nitride composites. Algal Res 69:102932. https://doi.org/10.1016/j.algal.2022.102932
Zhang T-T, Wang L-L, He Z-X, Zhang D (2011) Growth inhibition and biochemical changes of cyanobacteria induced by emergent macrophyte Thalia dealbata roots. Biochem Syst Ecol 39(2):88–94. https://doi.org/10.1016/j.bse.2011.01.004
Zhang X, Ma Y, Tang T, Xiong Y, Dai R (2020) Removal of cyanobacteria and control of algal organic matter by simultaneous oxidation and coagulation - comparing the H2O2/Fe(II) and H2O2/Fe(III) processes. Sci Total Environ 720:137653. https://doi.org/10.1016/j.scitotenv.2020.137653
Zhao Y, Fang Y, Jin Y, Huang J, Ma X, He K, He Z, Wang F, Zhao H (2015) Microbial community and removal of nitrogen via the addition of a carrier in a pilot-scale duckweed-based wastewater treatment system. Bioresour Technol 179:549–558. https://doi.org/10.1016/j.biortech.2014.12.037
Zhao ZH, Jun-Qing ZI, Huang BS, Branch ND (2018) Preliminary study on algae removal test of plant flocculants in Erhai Lake. Environ Sci Survey 37(01):15–16. https://doi.org/10.13623/j.cnki.hkdk.2018.01.006
Zhou L, Zi-Shi FU, Chen GF, Pan Q, Song XF, Zou GY, Liu YQ (2018) Research advance in allelopathy effect and mechanism of terrestrial plants in inhibition of Microcystis aeruginosa. Chinese J Appl Ecol 29(05):1715–1724. https://doi.org/10.13287/j.1001-9332.201805.038
Zhu J, Liu B, Wang J, Gao Y, Wu Z (2010) Study on the mechanism of allelopathic influence on cyanobacteria and chlorophytes by submerged macrophyte (Myriophyllum spicatum) and its secretion. Aquat Toxicol 98(2):196–203. https://doi.org/10.1016/j.aquatox.2010.02.011
Zhu X, Dao G, Tao Y, Zhan X, Hu H (2021) A review on control of harmful algal blooms by plant-derived allelochemicals. J Hazard Mater 401:123403. https://doi.org/10.1016/j.jhazmat.2020.123403
Zhu Z, Liu Y, Zhang P, Zeng G, Hu X, Li H, Guo Y, Guo X (2014) Co-culture with Cyperus alternifolius induces physiological and biochemical inhibitory effects in Microcystis aeruginosa. Biochem Syst Ecol 56:118–124. https://doi.org/10.1016/j.bse.2014.05.008
Zuo S, Mei H, Ye L, Wang J, Ma S (2012a) Effects of water quality characteristics on the algicidal property of Alternanthera philoxeroides (Mart.) Griseb. in an aquatic ecosystem. Biochem Syst Ecol 43:93–100. https://doi.org/10.1016/j.bse.2012.03.003
Zuo S, Yongqing MA, Shinobu I (2012b) Differences in ecological and allelopathic traits among Alternanthera philoxeroides populations. Weed Biol Manage 12(3):123–130. https://doi.org/10.1111/j.1445-6664.2012.00443.x
Funding
This work was supported by the Postgraduate Research & Practice Innovation Program of Jiangsu Province (SJCX22_1571).
Author information
Authors and Affiliations
Contributions
H.L., the corresponding author, developed the idea, as well as contributed to the sourcing of relevant literature and critically revised the work. T.W. performed the literature search and data analysis and finally wrote it.
Corresponding author
Ethics declarations
Ethics approval
Not applicable.
Consent to participate
Not applicable.
Consent for publication
All authors have reviewed and approved the manuscript for publication.
Competing interests
The authors declare no competing interests.
Additional information
Responsible Editor: Thomas Hein
Publisher’s note
Springer Nature remains neutral with regard to jurisdictional claims in published maps and institutional affiliations.
Rights and permissions
Springer Nature or its licensor (e.g. a society or other partner) holds exclusive rights to this article under a publishing agreement with the author(s) or other rightsholder(s); author self-archiving of the accepted manuscript version of this article is solely governed by the terms of such publishing agreement and applicable law.
About this article
Cite this article
Wang, ., Liu, H. Aquatic plant allelochemicals inhibit the growth of microalgae and cyanobacteria in aquatic environments. Environ Sci Pollut Res 30, 105084–105098 (2023). https://doi.org/10.1007/s11356-023-29994-5
Received:
Accepted:
Published:
Issue Date:
DOI: https://doi.org/10.1007/s11356-023-29994-5