Abstract
The glass particles were coated with Spirulina sp. LEB-18 and bioblends of Spirulina sp. LEB-18/chitosan by casting technique and, afterward, it was verified its potential as adsorbents for basic and acid dyes. Nine Spirulina sp. suspensions with different components were used to coat the glass particles, and in the best condition of coating were prepared the bioblends with chitosan. The coated glass particles with Spirulina sp. and its bioblends with chitosan were applied in adsorption of the allura red (acid) and methylene blue (basic) dyes in a batch operation evaluate the pH effect, and a fixed bed column operation, being evaluated to the removal percentage and adsorption capacity of the column. The glass particles coated with Spirulina sp. applied in batch adsorption showed the highest removal percentages for allura red dye (35 to 45%) at pH 4.0, and for methylene blue dye (35 to 80%) at pH 6.0 and 8.0. In fixed bed column using glass particles coated with bioblends were reached the amount dye of 54.2 mg of adsorbed allura red dye and 60.2 mg of the of adsorbed methylene blue dye, respectively. Moreover, it was found good dye adsorption capacities, around 89 mg g−1, for both dyes, in acidic and basic pH values. Based on these results, these bioblends coated glass particles can be applied as an adsorbent for different types of dyes in adsorption column.
Similar content being viewed by others
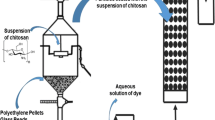
Explore related subjects
Discover the latest articles, news and stories from top researchers in related subjects.Avoid common mistakes on your manuscript.
Introduction
Spirulina sp. microalga has a height protein content, polyunsaturated fatty acids, vitamins, minerals, and pigments, and due to these biomolecules varieties in its composition, the dry biomass has several functional groups in your structure, such as amines, carboxylates, hydroxyls, aldehydes, ketones, phosphates, and sulfates (Fakher et al. 2019). Besides that, the renovation potential in the environment and great availability makes Spirulina sp. a microalga that has been extensively studied (Koru 2012). From an environmental aspect, the Spirulina sp. is outstanding in the application as an adsorbent of aqueous solutions pollutants, such as dyes. The food and textile industries are the ones that most use dyes. One of the most used dyes in the food industry is Allura red, an anionic dye with two sulfonic groups. In the textile industry, one of the widely used dyes is methylene blue, a cationic dye in an aqueous solution. These dyes are soluble in water, and their presence in effluents is visible and undesirable. The problem associated with the use of dyes is their presence in wastewater, when discarded without proper care, can cause a great environmental impact on the water body where they are discarded (Dotto et al. 2012a).
Hassan and Carr 2018 related the challenges and directions for the development of the new adsorbents, and that the adsorption is a great suitable for the dye removal of industrial effluents; however, further research is needed so that biomass can compete with ion exchange resins and activated carbon. Dotto et al. 2012a,b,c developed works applying micro and nanoparticles of Spirulina sp. in biosorption of food dyes and metal ions, through the evaluation of kinetics, equilibrium, and thermodynamics of biomass biosorption; the authors demonstrated that the microalga Spirulina sp. is a biosorbent potential for removal of dyes from aqueous solutions.
Besides all these findings, the macromolecules variety that makes Spirulina sp. can be combined with other biomaterials to form cohesive and firm matrices, such as bioblends of chitosan and Spirulina sp. (Silva et al. 2017). Chitosan is a natural polymer that has high commercial value and interesting properties in the industrial and research fields, being that an expressive number of publications were related the chitosan modification and application as adsorbent in wastewater treatment (Brion-Roby et al. 2018; Hossini et al. 2017; Ignat et al. 2016). Modified chitosan has been used to improve its chemical stability in acidic environments, its selectivity, and the adsorption capacity of metals and dyes from wastewater (Rocha et al. 2016). These characteristics were attributed to the chitosan polymer chain that contains the amino and hydroxyl groups, which allow its structural modification and use as a biomaterial in the form of gels, films, membranes, powders, flakes, spheres, sponges, fibers, and supported by inert material (Dotto et al. 2017). Moreover, bioblends are systems that originated from the physical mixture of two or more components, whose main objective is to incorporate the properties of the different compounds into the same material. Silva et al. 2017 developed bioblends films of chitosan/Spirulina sp., for application in the dyes removal from aqueous solutions; the authors observed that the films of the bioblends preserved its mechanical structures at pH ranges from 4.0 to 8.0, and they found that the bioblends films of chitosan /Spirulina sp. were suitable for removing anionic and cationic dyes from aqueous solutions.
The adsorption operation is most industrially used in a column packed with an adsorbent (fixed bed), through which the mixture will percolate having one or more adsorbed components. This fact has promoted the development of cheaper and more efficient adsorbents such as clay, zeolites, silicas, agro-industrial residues, biopolymers, and biomasses (Yagub et al. 2014, Gupta and Suhas 2009) . Glass beads coated with chitosan stand out by good mechanical resistance for application in continuous adsorption processes, overcoming problems that occur when chitosan flakes or powder are applied, such as larger pressure drop and fixed bed clogging (Vieira et al. 2018). Thus, for the application Spirulina sp. and chitosan as adsorbent in fixed bed column is required its structural modification to improve particle characteristics necessary by system hydrodynamics (Auta and Hameed 2014; Gokhale et al. 2009). Among the techniques applied for the structural modification there is the casting technique, which is used for chitosan films production (Moura et al. 2015) and bioblends of Spirulina sp./chitosan (Silva et al. 2017).
The aim of this work was to coat glass particles with Spirulina sp. LEB-18 and with bioblends of Spirulina sp. LEB-18/chitosan through the casting technique, and to verify their potential as adsorbent of the basic and acid dyes. The glass particles were coated with nine Spirulina sp. LEB-18 suspensions added of the different components, and in the best condition of coating were prepared the bioblends with chitosan. The plasticizer and surfactants additions, and chitosan proportion added in the bioblends about the coating percentage in cure steps (physical and chemical) were evaluated. The glass particles coated with Spirulina sp. LEB-18 and its bioblends with chitosan were applied in dyes adsorption of the allura red (acid food dye) and methylene blue (basic textile dye) in a fixed bed column. The coated glass particles performances, for both dyes, were evaluated to the removal percentage and adsorption capacity of the column.
Materials and methods
Materials
The materials used for the development of this work were adsorbents (Spirulina sp. and chitosan), adsorbates (dyes) glass particles, and analytic grade reagents. Spirulina sp. LEB 18 was donated by Biochemical Engineering Laboratory (LEB) of the School of Chemistry and Food of the Federal University of Rio Grande (FURG). Chitosan was produced from shrimp waste (obtained in local industries of Rio Grande, Brazil). The adsorbates used were the allura red (acid food dye) acquired from the Plury Química Ltda (Diadema, Brazil), and methylene blue (basic textile dye) acquired from the Sigma-Aldrich (São Paulo, Brazil). The particles used were glass beads acquired from the Nacional Esferas Ltda (Brazil).
Production and characterization of Spirulina sp. LEB 18 biomass
The biomass cultivation was realized in open outdoor photo-bioreactors with 450 L of the water, under uncontrolled conditions, according Morais et al. 2008. During the cultivation, water supplemented with 20% Zarrouk synthetic was added. The biomass was recovered by filtration, when the biomass concentration reached 0.5 g L−1, afterward, washed and pressed until it reached the moisture content of 76% (wet basis).
The wet biomass was led to a trays conventional dryer, in cylindrical pellet form with a diameter of 4 mm, on stainless steel perforated tray of 2 mm openings, using parallel air flow with the following conditions, according to Oliveira et al. 2010: air temperature 55 °C, air velocity 2.5 m s−1, absolute air humidity 0.013 kg kg−1dry air, trays thickness of 4 mm, load in tray of 4 kg m−2. The size reduction of dried biomass Spirulina sp. LEB-18 was performed using a mill (Wiley Mill Standard, No. 03, USA) and was sieved until the discrete particle size ranging from 35 to 150 mesh (Tyler standard), representing the size range between 106 and 425 µm.
Spirulina sp. LEB 18 was characterized when its centesimal composition. The moisture and ashes contents of the dry biomass Spirulina sp. LEB 18 were determined by gravimetric method (nº 950.46 and nº 920.153, respectively), the protein content was obtained by Kjeldhal method (nº 928.08), the lipid content was determined by Folch, Lee and Stanley method (Folch et al. 1957); all methods were according to Official Methods of Analysis (AOAC 1995) and carbohydrates obtained by difference.
Preparation and characterization of chitosan
Chitin was extracted of shrimp residuals (Penaeus brasiliensis) according to methodology described by Weska et al. 2007. Chitosan was obtained by chitin deacetylation alkaline. The deacetylation reaction was realized in a reactor, where it was added 45.0 g of chitin and 3.0 L of NaOH 42.1% m v−1 under constant stirring (50 rpm) and temperature of 130 ± 2 ºC (Moura et al.2011). Chitosan was purified and dried in a spouted bed according to Dotto et al. 2011, and the product presented particles size from 64 to 70 µm. Chitosan was characterized when deacetylation degree and molecular weight. The deacetylation degree was determined by potentiometric titration (Tolaimate et al. 2000) and the molecular weight was determined by viscosimetric method (Weska et al. 2007).
Dyes adsorbates
Allura red is a food dye with color index 16,045, chemical class azo, molecular formula C18H14N2Na2O8S2, molecular weight of 496.4 g mol−1 and maximum wavelength of 500 nm (Piccin et al. 2009). Methylene blue is a textile dye with color index 52,015, molecular formula C16H18ClN3S, molecular weight of 319.8 g mol−1 and maximum wavelength of 660 nm (Liu et al. 2012). The allura red molecule presented groups azo (–N = N–), sulfonated (–SO3−) and radicals (–OH), and in methylene blue molecule presents groups nitrogenated (–N+(CH3)2).
Coating suspensions production
The coating suspensions of Spirulina sp., chitosan, and bioblends were prepared by biopolymer dissolution and biomass in a suitable solvent with mechanical agitation. The coating suspensions of Spirulina sp. were prepared by addition of 0.75 g biomass (d.b.) in 75 mL of distilled water, under stirring of 25,000 rpm in a mechanical stirrer (Bosch, GS 927 Professional, Brazil) for 10 min at ambient temperature (20 ± 2 °C), according to Silva et al. 2017. Afterward, nine types of coating suspension with different compositions (from S1 to S9 assays) were prepared, as expressed in Table 1. The experimental design was carried out being evaluated in the coating suspension the plasticizer type (sorbitol and glycerol) and surfactant type (Tween 80 and magnesium stearate). The amounts of plasticizer and surfactant were, respectively, 0.2 g (wet basis to Spirulina sp.) and 0.05 g (dry basis to Spirulina sp.).
The best condition of coating with Spirulina sp. was used in the bioblends preparation with chitosan. The bioblends were carried out by addition of the chitosan solution in the Spirulina sp. suspension. Chitosan coating solutions were prepared by chitosan dissolution (0.25, 0.50 or 0.75 g, d.b.) in 100 mL of acetic acid solution 1.0% (v v−1), under stirring of 600 rpm for 2 h, at ambient temperature (20 ± 2 °C) according to Vieira et al. 2018. The bioblends were nominated as B1, B2, and B3, and its proportions of Spirulina sp. (g): chitosan (g) were, respectively, B1 (0.75: 0.25), B2 (0.75: 0.50) and B3 (0.75: 0.75).
Characterization and coating of glass particles
First, the glass particles were characterized to the mean diameter, sphericity, specific mass, and surface morphology. A sample of 30 glass particles was used for these measurements. The particles mean diameter (Dm) was determined by pachymetry. The esfericity shape factor (ø) and density (ρp) were determined according to Geankoplis 2003. For these measurements, 30 particles were used Then, the glass particles coating was realized through the sequential steps of surface preparation, coating application, physical cure, and chemical cure according to preview work (Vieira et al. 2018).
The particles used were glass beads, placed in flasks and cleaned with acid acetic solution 3,0% (v v−1) for 6 h at home temperature (25 ± 2 °C). In the next step, the particles were separated by filtration and coated by the casting technique. This procedure was carried in plastic petri dishes, where the particles (75.0 g) were immersed on (75.0 mL) coating suspensions for 24 h at temperature of 40 ± 2 °C. The petri dishes remain open for solvent evaporation and film formation on particles surface (physical cure). After this step, the particles were immersed on coagulant solution for 4 h (chemical cure). At each step, the particles are weighed to calculate the coating percentage (Eq. (1)). The covering assays were carried out in three repetitions (n = 3). The coating quantification was performed according to Vieira et al. 2019. The coated glass particles (25.0 g) were subjected to high agitation (900 rpm) in acetic acid solution (3.0%) to coating separation. The coating percentage (%C) was determined by Eq. (1) where mICB (g) is the initial mass of coated glass particles, mFIB (g) is the final mass of glass particles without coating, mISOL is the solids mass contained in the coating solution (g).
The coating percentage was calculated in two steps of the coating procedure: after physical cure step (%C1), and after chemical cure step (%C2). In addition, the solids mass adhered on glass particles (mcoa) was expressed as a reason of the mgsolids g−1particles. Thus, it is possible to determine adsorbent mass contained on batch experiments and adsorption column. The coated glass particles surface was analyzed by scanning electron microscopy (SEM) (Jeol, JSM-6060, Japan).
Application coated glass particles on adsorption
The coated glass particles with Spirulina sp. obtained from S1 to S9 assays were applied in batch adsorption to evaluate the pH effect (4, 6, and 8) and the dye type (acid and basic). The dyes used were allura red and methylene blue. In the sequence, the coated glass particles with Spirulina sp. (best condition of coating and batch adsorption) and bioblends obtained from B1 to B3 were applied in fixed bed adsorption to evaluate the dye type (acid and basic). The adsorption on coated glass particles was verified with dispersive X-ray spectrometry (EDS) (Jeol, JSM–6610LV, Tokyo, Japan).
Batch adsorption assays
The batch adsorption assays were realized with agitation of 200 rpm using an orbital shaker (Nova Ética, 109–1 TCM, Brazil) to determine dye removal percentage (%R). The adsorbate solutions were prepared in volumetric flask using 10 mL of dye solution (1.0 g L−1), 10 mL of disodium phosphate / citric acid buffer (0.1 mol kg−1) and completed with distilled water until 100 mL. The dye concentration solutions obtained was 100 mg L−1 at the different pH values. These solutions were transferred for becker with 25 g of Spirulina sp. coated glass particles, and remained on the shaker for 24 h at temperature of 20 ± 2 °C. The remaining dye concentration in the solution was determined on a spectrophotometer (Quimis, Q108 DRM, São Paulo, Brazil) at a maximum wavelength of 500 nm for allura red and 660 nm for methylene blue. The dye removal percentage %R was determined by Eq. (2), where C0 and Ce are the initial and equilibrium dye concentrations in the liquid phase (mg L−1), respectively.
Fixed bed adsorption assays
The fixed bed adsorption assays were realized in acrylic column with 3.4 cm of internal diameter and 30 cm of height, added of equalizer at input (7.0 cm) and output (3.0 cm). First, blank tests were performed in the column packed with 30 cm of uncoated glass particles. After, the column was packed of 125-g coated glass particles for assays of Spirulina sp. (with the best condition) and bioblends (B1, B2, and B3). These coated glass particles composed 10 cm in the column middle. The base (10 cm) and the top (10 cm) of the column were placed uncoated glass particles.
The dye solutions used were allura red (pH = 4.0) and methylene blue (pH = 8.0) in concentration of 100 mg L−1. The solutions were pumped upward through the column a flow rate of 3.0 mL min−1 with use of a peristaltic pump (Master Flex, 07,553–75, Ottawa, Canada). In the column top, samples were collected at regular intervals until the column saturation, when the column concentration output (Ct) equaled the column concentration input (C0). The dye concentration in samples was determined by a spectrophotometry (Quimis, Q108 DRM, São Paulo, Brazil). The assays were carried out in replicate (n = 3) and blank assays using uncoated glass particles were performed.
The collected data were expressed as breakthrough curves. The column times of breakthrough (tb) and exhaustion(te) were considered when the dye concentration at column top reached about 5.0 mg L−1 and 95.0 mg L−1, respectively. These values correspond at 5.0% and 95.0% of the initial dye concentration (C0) (Lemus et al. 2017).
Through the breakthrough curves were calculated the amount of dye adsorbed, mad (mg), the equilibrium adsorption capacity qeq (mg g−1) and the total amount of dye mtotal (g) presents in the column, according to Eqs. (3)–(5), respectively; where, Q is the volumetric flow rate (mL min– 1); Cad is the final dye concentration on column (mg L−1); t is the time (min); m is the adsorbent mass on column (g); and C0 is the initial dye concentration (mg L−1) (Brion-Roby et al. 2018; Esfandian et al. 2017).
Results and discussion
Spirulina sp. LEB 18 was characterized by centesimal composition (w w−1): moisture content of 8.6 ± 0.6, ashes content 5.4 ± 0.2, lipid content of 8.3 ± 0.3, protein content of 67.2 ± 0.8, and carbohydrates content of 10.5 ± 0.4 (obtained by difference). The centesimal composition for the Spirulina LEB-18 sp. biomass was like that found by Silva et al. 2017. According to Morais et al. 2008, the Spirulina sp. dry biomass is mostly composed of proteins, which vary from 64.0 to 74.0% (w w−1). Chitosan was characterized by a deacetylation degree of 85.0 ± 1.0% and a molecular weight of 146.0 ± 5.0 kDa, and these values are like to found by Vieira et al. 2018. The glass particles were characterized by a mean diameter of 3.0 ± 0.2 mm, sphericity of 0.98 ± 0.01 and density of 2300 ± 50 kg m−3. The glass particles surface was verified by EDS analysis, and the results showed that the major elements on surface were oxygen (54.5 ± 2.6%) and silicon (45.6 ± 1.9%), these being constituents of vitreous silica.
Glass particles coating
The glass particles coating was realized with suspensions of Spirulina sp. and Spirulina sp./chitosan bioblends. In the coating with Spirulina sp. (from S1 to S9 assays) were verified the effects of the plasticizer and surfactant additions. In the best condition of coating with Spirulina sp. were produced the bioblends with chitosan (from B1 to B3), and the results of the mass adhered and the coating percentage after steps of physical cure (PC) and chemical cure (CC) are shown in Table 1, together with the components respective for each assay.
The results of coating with Spirulina sp. for the physical cure (PC) step presented in Table 1 show a large variation for coating percentage (%C1), with values from 38 to 75%. This variation can be explained by the different components in the coating suspension and the interaction between them. The presence of surfactants (Tween 80 and Magnesium Stearate) on the suspension favorited the Spirulina sp. adhesion on glass particles, due to values above 70% for coating percentage in S2 and S3 assays. The surfactant has the function of reducing the surface tension, allowing the liquid in contact with the surface to have a better spreading, with this, the Spirulina sp. suspension must have reached a larger area on glass particles surface and, consequently, the contact with a greater number of active sites available on surface. However, the simultaneous use of surfactant and plasticizer reduced the coating percentage on glass particles, according to the results found in S5, S6, S8, and S9 assays, all below 61%.
The Spirulina sp. mass retained in the glass particles varied from 5.2 to 8.6 mgsolids g−1particles. However, only the drying of the Spirulina sp. suspension (film) on glass particles (physical cure) did not guarantee full coating adherence. Therefore, in the subsequent adhesion step (chemical cure), a coagulating agent was used to ensure that the adsorption application is successful, without solubilization problems during the adsorption process. The quantifications for the chemical cure (CC) step are shown in Table 1, which shows that the results of coating percentage (%C2) after the step chemical cure stayed between 5 and 62%. Comparing these results (%C2) with those presented in the first step (% C1), there is a reduction in the percentage coated with Spirulina sp. is evident in all assays. According to the values found for the coating percentage (%C2) of the S7, S8, and S9 assays, it can be noted that the addition of glycerol or glycerol plus the surfactants (Tween 80 or MgS) favored the biomass adhesion in the glass particles after chemical cure (CC). Also, the use of Tween 80 (S2 assay) showed good results. In the other assays, a large part of the biomass was leached into the coagulant solution, leaving %C2 below 15%, making its application unfeasible.
Regarding the use of sorbitol and glycerol plasticizers, its additions in the coating suspension did not affect the coating percentage (p > 0.05), with values around 68% for the S1, S4, and S7 assays; this effect had been observed in the glass particles coating whit chitosan. The function of plasticizers is to make the film more malleable, both glycerol and sorbitol have a hydrophilic character; however, glycerol has a lower molar mass than sorbitol. As for the surfactants analyzed in the study, Tween 80 has hydrophilic groups without charges, while magnesium stearate has a hydrophobic nature. In this context, the glycerol addition (S7 assay), Tween 80 (S2 assay), or both (S8 assay) in the suspension favored the coating, which may be related to the hydrophilic character presented by these components. Given the exposed results, one can choose the condition of the S7 assay to compose the bioblends of Spirulina sp. and chitosan, following the coating studies.
The bioblends were composed of Spirulina sp., chitosan, and glycerol. The Spirulina sp. mass contained in the bioblends was 0.75 g for all bioblends, and the chitosan mass values for B1, B2, and B3 bioblends were, respectively, 0.25, 0.50, and 0.75 g. After the physical cure and chemical cure steps, it has carried out the quantifications of the coating percentage and adhered mass according to results presented in Table 1. It can observe a decrease in the coating percentage (%C1) as the total mass of the bioblend has been increased (from B1 to B3). Demonstrating that for the coating percentage to remain above 50%, there is a limit to the solids present in the suspension. In the case of this study, it was 1.25 gsolids for 75 gparticles to be coated, used for B2 bioblend. In chemical cure (CC), there was a decrease in the coating percentages of the bioblends, due to the leaching of part of the coating to the coagulant solution. However, the increase in chitosan mass from B1 bioblend (0.25 g) to B2 (0.50 g) provided an increase in the coating percentage from 17 to 25%. In the chemical cure (CC), there was the contact of the glass particles coated with a basic solution, with this, the higher amount of chitosan brought benefits for the adhesion of the bioblend to the glass particles. A further increase in the chitosan mass from B2 bioblend (0.50 g) to B3 (0.75 g) did not demonstrate a significant increase (p > 0.05) in the coating percentage. Therefore, the amount of solids present in the coating suspension should be limited.
In the coating process, the adhered mass values obtained was 5.6 mgsolids g−1particles. Based on these results, the glass particles coated with bioblends have the potential to be applied in the adsorption of dyes in a fixed bed column.
Figure 1 shows the glass particles surfaces coated with bioblends, which were analyzed by scanning electron microscopy (SEM). The coating was different for each bioblend as shown in the coated particles images. The glass particle coated with B1 bioblend has a rough appearance largely, and in B2 bioblend, it is observed that the roughness is in a part of the particle; in the coating with B3 bioblend, the rough parts are more distributed along the particles. For images augmented 500 times, one can observe more clearly the surface of coated glass particles with bioblends. In this case, a pattern of agglomerates was identified for the coating with B1 bioblend, which is more dispersed in the coatings of B2 and B3 bioblends. This behavior can be explained by the progressive increase of chitosan in each bioblend; thus, the chitosan contributed to the uniformity of surface coated.
Application of the coated particles in dyes adsorption assays
The dyes presence on coated glass particles surface was verified by EDS analysis. The images and sulfur distribution for coated glass particles with bioblends and adsorbed with the allura red and methylene blue dyes can be seen in Fig. 2. The batch adsorptions assays were carried out to verify the pH effect (4, 6, and 8) on removal percentages of allura red and methylene blue dyes, by glass particles coated with Spirulina sp. from S1 to S9 assays, as are shown in Figs. 3 and 4.
The fixed bed column adsorption assays were performed to verify the column adsorption capacity; however, some delimitations were made based on the coating and the batch adsorption assays. During coating assays with Spirulina sp., it was found that in S7 assay was the composition with the highest coating percentage (around 62%). This was the criterion for choosing the S7 assay composition as a basis for bioblends, as well as in applying Spirulina sp. for coated glass particles in column adsorption. In the batch adsorption assays, the glass particles coated in S7 assays had higher removal percentages of allura red dye at pH 4, and methylene blue dye at pH 6 and 8. Thus, for the column experiments, extreme pH was chosen (pH values of 4.0 and 8.0) according to adsorption parameters shown in Table 2 and the breakthrough curves in Figs. 5 and 6.
The particles coated with bioblends of chitosan and Spirulina sp. and adsorbed with the allura red and methylene blue dyes were subjected to energy-dispersive X-ray spectroscopy, as shown in Fig. 2. The left of Fig. 2 shows the surface elemental spectrum (EDS) of the glass particles coated with a chitosan/Spirulina sp. bioblend (B3 assay) adsorbed with the allura red and methylene blue dyes. The right of Fig. 2 shows the distribution of the sulfur element over the coated glass particles surface.
In Fig. 2, the elements identified in higher proportion were carbon, oxygen, and silicon. Oxygen and silicon are elements related to glass particle composition (glass: tetrahedral units of Si–O–Si). The presence of the carbon and oxygen elements is related to the groups of hydroxyls, carboxyls, aldehydes, and ketones present in Spirulina sp. biomass, while carbon and nitrogen elements characterize the presence of chitosan biopolymer. In addition, the presence of the sulfur element in the spectrum can be noted and its distribution is shown in the right of Fig. 2. This element comes from molecules of allura red and methylene blue dyes, which confirms the presence of dyes adsorbed on the coated surface.
Dyes adsorption assays in batch
The results of dyes adsorption assays in batch demonstrated that the glass particles coated with Spirulina sp. had the best adsorptive capacities at different pH values for each dye. The removal of the methylene blue dye by glass particles coated with Spirulina sp. reached the best percentages at neutral and basic pH, with values from 35 to 80%, while the allura red dye had removal percentages from 35 to 45% at pH acid (Table 3).
In Fig. 3, the methylene blue dye adsorption by glass particles coated with Spirulina sp. reached high removal percentages at pH 6.0 and pH 8.0. In an alkaline medium, the methylene blue dye is a neutral molecule and can interact with the numerous groups present on the surface of Spirulina sp., while at pH 4, the dye removal percentage was below 20% for all assays of glass particles coated with Spirulina sp. This fact can be attributed to the repulsion between the dye positive charges and the Spirulina sp. charges in acid medium. Among the compositions with the best results were S8 and S9 assays, which removed about 80% of the dye at pH 6, while S3 and S7 assays had dye removal percentages above 70% at pH 6 and pH 8. Thus, it can be inferred that the assays with glycerol led to greater versatility to the coating and its application in adsorption.
In Fig. 4, the allura red dye adsorption by glass particles coated with Spirulina sp. reached high removal percentages at pH 4.0. In acid medium, the allura red dye is negatively charged and can interact with –OH, –NH2, = C = O and –COO groups of the Spirulina sp. that have a positive charge in acidic conditions. When the adsorption occurred at pH 6 and pH 8, the dye removal was below 35%, and this fact can be explained by the sulfonated groups deprotonation of the allura red dye in basic medium, hindering the chemical interactions between the dye and groups present in Spirulina sp. Among the compositions with the best results were S2, S3, and S4 assays, with a removal percentage above 40% of the dye at pH 4. Thus, it can be inferred that the addition of each component individually, surfactant or plasticizer, contributed more effectively to the dye allura red adsorption than assays with the presence of both components. In the S2 (Tween 80) and S3 (magnesium stearate) assays, there was a more pronounced effect in reducing the cohesion between the molecules, which allowed the interaction with the liquid (dye in solution) that surrounds the coated glass particles, while in the S4 assay (sorbitol), the presence of –OH groups protonated in acidic medium helped in the adsorption of the dye with negative charge.
Dyes adsorption assays in fixed bed column
The glass particles coated with Spirulina sp. (S7 assay) and with bioblends of chitosan/Spirulina sp. (B1, B2, B3) were applied in the methylene blue and allura red adsorptions in a fixed bed column. The column of 10 cm was packed with 125 g of coated glass particles, which contained the adsorbent mass of 980 mg for S7 assay, 340 mg for B1 assay, 600 mg for B2 assay, and 700 mg for B3 assay. The experimental conditions were dye initial concentration of 100 mg L−1, flow rate of 3 mL min−1, and temperature of 293 K. It is worth mentioning that no changes were observed in the concentration of dye at the exit of the column for the blank tests performed with uncoated particles. The adsorption breakthrough curves for methylene blue (pH 8.0) and allura red (pH 4.0) are presented in Figs. 5 and 6, respectively. The values of the breakthrough time (tb) and exhaustion time (te), amount of dye adsorbed (mad), dye equilibrium adsorption capacity (qeq), and removal percentage (%R) are shown in Table 2.
The best performance of the fixed bed column was found when packed of glass particles coated with B3 bioblend (Figs. 5 and 6), showing the amount of dye adsorbed (mad) of 54.2 mg for allura red dye and 60.2 mg for methylene blue (Table 2). However, the breakthrough expressed a different behavior for the dyes adsorption. The methylene blue adsorption had low breakthrough times (tb), and thus exhibited a curve with a more elongated shape; thus, the time until saturation was higher (te). In the allura red adsorption, it is observed that the breakthrough times were higher, and the curves had smaller declivities, making them closer to ideality, showing that saturation of the fixed bed column was faster.
In Table 2, the breakthrough time reached the highest values for the methylene blue and allura red dyes adsorption in column filled with glass particles coated by B3 bioblend. This fact may be associated with the amount of chitosan present in B3 bioblend. The glass particles coated with Spirulina sp. (S7 assay) showed the same rupture and exhaustion times, and in addition to these parameters, it is observed that the adsorbed dye mass and the adsorption capacity were equal (p > 0.05) for both dyes, while in bioblends, as the chitosan mass increased, breakage times, exhaustion, and adsorbed dye masses also increased. In contrast, the values found for the dyes adsorption capacity at equilibrium tripled when the bed was filled with glass particles coated with B1 bioblend (about 90 mg g−1) compared to the bed with glass particles coated with Spirulina sp. S7 (about 30 mg g−1). This increase in adsorption capacity can be attributed to the adsorbent mass present in the column, which was 980 mg for S7 assays and 340 mg for B1 assays. Increasing the adsorbent mass, the reflects in the adsorption capacity values due to the inversely proportional relationship between the adsorption capacity and the adsorbent mass. As for the dye removal percentages, these were between 30 and 45%. These values are usually low as they consider the operating time to saturation.
Conclusions
The application of the chemical cure after the physical cure is a determining factor for the adhesion on glass particles coating, leading to the coated particles being suitable for application as an adsorbent. Furthermore, the glycerol addition as a plasticizer provided malleability to the coating and contributed to the high coating percentage of glass particles with Spirulina sp. LEB-18. The bioblend did not reach the same values of coating percentage as the glass particles coated only with Spirulina sp. However, the increase in chitosan mass added to the bioblend with Spirulina sp. contributed to improving the coating percentage and, also, changed the surface characteristics, leaving the glass particles with a more uniform coating. The glass particles coated with Spirulina sp. applied in batch adsorption of dyes reached the highest removal percentages of methylene blue at neutral and basic pH. These same particles, when applied in column adsorption, reached the same equilibrium capacity for both dyes. Furthermore, the increase in chitosan mass in the bioblends favored the adsorption of the methylene blue dye at basic pH. With the bioblend, it was possible to reach the same levels of dye adsorption capacity, both in acidic and basic pH. The best performance of the fixed bed column was found when packed with glass particles coated with B3 bioblend, with 54.2 mg of adsorbed allura red dye and 60.2 mg of adsorbed methylene blue dye. This evidence enables the bioblend application as an adsorbent for dyes of different types in a fixed bed column, and it brings with it a competitive advantage over Spirulina sp. or chitosan when applied individually, because Spirulina sp. is recommended for the basic dyes adsorption and chitosan for the acid dyes adsorption.
Data availability
The datasets used and/or analyzed during the current study are available from the corresponding author on reasonable request.
References
Alinezhad H, Zabihi M, Kahfroushan D (2020) Design and fabrication the novel polymeric magnetic boehmite nanocomposite (boehmite@ Fe3O4@PLA@SiO2) for the remarkable competitive adsorption of methylene blue and mercury ions. J Phys Chem Solids 144:109515. https://doi.org/10.1016/j.jpcs.2020.109515
Alkahtani SA, Abu-Alrub SS, Mahmoud AM (2017) Adsorption of food coloring allura red dye (E129) from aqueous solutions using activated carbon. Int J Food Allied Sci 3:10–19. https://doi.org/10.21620/ijfaas.2017110-19
AOAC Association of Official Analytical Chemists (1995) 14th ed., v. 1, Oficial Methods of Analysis, Washington, DS
Auta M, Hameed BH (2014) Chitosan–clay composite as highly effective and low–cost adsorbent for batch and fixed–bed adsorption of methylene blue. Chem Eng J 237:352–361. https://doi.org/10.1016/j.cej.2013.09.066
Ávila-Martínez AK, Roque-Ruiz JH, Torres-Pérez J, Medellín-Castillo NA, Reyes-López SY (2020) Allura Red dye sorption onto electrospun zirconia nanofibers. Environ Technol Innov 18:100760. https://doi.org/10.1016/j.eti.2020.100760
Badri A, Alvarez-Serrano I, López ML, Amara MB (2020) Sol-gel synthesis, magnetic and methylene blue adsorption properties of lamellar iron monophosphate KMgFe(PO4)2. Inorg Chem Commun 121:108217. https://doi.org/10.1016/j.inoche.2020.108217
Brion-Roby R, Gagnon J, Deschênes J-S, Chabot BJ (2018) Investigation of fixed bed adsorption column operation parameters using a chitosan material for treatment of arsenate contaminated water. J Environ Chem Eng 6:505–511. https://doi.org/10.1016/J.JECE.2017.12.032
Çathoglu F, Akay S, Turunç E, Gozmen B, Anastopoulos I, Kayan B, Kalderis D (2021) Preparation and application of Fe-modified banana peel in the adsorption of methylene blue: process optimization using response surface methodology. Environ Nanotechnol Monit Manag 16:100517. https://doi.org/10.1016/j.enmm.2021.100517
Dotto GL, Souza VC, Moura JM, Moura CM, Pinto LAA (2011) Influence of drying techniques on the characteristics of chitosan and the quality of biopolymer films. Drying Technol 29:1784–1791. https://doi.org/10.1080/07373937.2011.602812
Dotto GL, Cadaval TRS, Pinto LAA (2012a) Use of Spirulina platensis micro and nanoparticles for the removal synthetic dye from aqueous solutions by biosorption. Process Biochem 47:1335–1343. https://doi.org/10.1016/j.procbio.2012.04.029
Dotto GL, Esquerdo VM, Vieira MLV, Pinto LAA (2012b) Optimization and kinetic analysis of food dyes biosorption by Spirulina platensis. Colloids Surf B: Biointerfaces 91:234–241. https://doi.org/10.1016/j.colsurfb.2011.11.008
Dotto GL, Lima EC, Pinto LAA (2012c) Biosorption of food dyes onto Spirulina platensis nanoparticles: equilibrium isotherm and thermodynamic analysis. Bioresour Technol 103:123–130. https://doi.org/10.1016/j.biortech.2011.10.038
Dotto GL, Campana-Filho SP, Pinto LAA (2017) Chitosan based materials and its applications. Bentham and Books Press, Sharjah. https://doi.org/10.2174/97816810848551170301
Esfandian H, Samadi-Maybodi A, Khoshandam B, Parvini NJ (2017) Experimental and CFD modeling of diazinon pesticide removal using fixed bed column with Cu – modified zeolite nanoparticle. J Taiwan Inst Chem Eng 75:164–173. https://doi.org/10.1016/j.jtice.2017.03.024
Fakher A, Lima EC, Sakly A, Mejri H, Ben Lamine A (2019) Modeling of adsorption isotherms of reactive red RR-120 on Spirulina sp. platensis by statistical physics formalism involving interaction effect between adsorbate molecules. Prog Biophys Mol Biol 141:47–59. https://doi.org/10.1016/j.pbiomolbio.2018.07.004
Folch J, Lees M, Stanley GHS (1957) A simple method for isolation and purification of total lipids from animal tissues. J Biol Chem 226:497–509. https://doi.org/10.1016/s0021-9258(18)64849-5
Geankoplis CJ (2003) Transport processes and separation process principles (includes unit operations), 4th ed., PTR Prentice Hall, New York, ISBN 013101367X
Gokhale SV, Jyoti KK, Lele SS (2009) Modeling of chromium (VI) biosorption by immobilized Spirulina platensis in packed column. J Hazard Mat 170:735–743. https://doi.org/10.1016/j.jhazmat.2009.05.005
Gupta VK, Suhas, (2009) Application of low-cost adsorbents for dye removal - a review. J Environ Manage 90:2313–2342. https://doi.org/10.1016/j.jenvman.2008.11.017
Hai X, Pan Z, Shao-Yan Z, Qiong J (2020) Fullerene functionalized magnetic molecularly imprinted polymer: synthesis, characterization and application for efficient adsorption of methylene blue. Chinese J Anal Chem 48:20107–20113. https://doi.org/10.1016/S1872-2040(20)60045-7
Hassan MM, Carr CM (2018) A critical review on recent advancements of the removal of reactive dyes from dyehouse effluent by ion-exchange adsorbents. Chemosphere 209:201–219. https://doi.org/10.1016/j.chemosphere.2018.06.043
Hossini H, Soltani RDC, Safari M, Maleki A, Rezaee R, Ghanbari R (2017) The application of natural chitosan/bone char composite in adsorbing textile dye from water. Chem Eng Commun 204:1082–1093. https://doi.org/10.1080/00986445.2017.1340274
Ignat M, Amoila P, Cojocaru C, Sacarescu L, Harabagiu V (2016) Novel synthesis route for chitosan-coated zinc ferrite nanoparticles as potential sorbents for wastewater treatment (Chitosan-ZnFe2O4 sorbent for wastewater treatment). Chem Eng Commun 203:1591–1599. https://doi.org/10.1080/00986445.2016.1185711
Koru E (2012) Earth Food Spirulina (Arthrospira): production and quality standarts, food additive. In El-Samragy Y (ed) InTech. Available from: http://www.intechopen.com/books/food-additive/earth-food-spirulina-arthrospira-production-andqualitystandarts
Lemus J, Moya C, Gilarranz MA, Rodriguez JJ, Palomar J (2017) Fixed-bed adsorption of ionic liquids onto activated carbon from aqueous phase. J Environ Chem Eng 5:5347–5351. https://doi.org/10.1016/j.jece.2017.10.014
Liu T, Li Y, Du Q, Sun Y, Jiao Y, Yang G, Wang Z, Xia Y, Zhang W, Wang K, Zhu H, Wu D (2012) Adsorption of methylene blue from aqueous solution by graphene. Colloid Surf B 90:197–203. https://doi.org/10.1016/j.colsurfb.2011.10.019
Morais MG, Reichert CC, Dalcaton F, Durane AJ, Marins LF, Costa JAV (2008) Isolation and caracterization of a new Arthrospira strain. Z Naturforsch 63:144–150. https://doi.org/10.1515/znc-2008-1-226
Moura CM, Moura JM, Soares NM, Pinto LAA (2011) Evaluation of molar weight and deacetylation degree of chitosan during chitin deacetylation reaction: used to produce biofilm. Chem Eng Process 50:351–355. https://doi.org/10.1016/j.cep.2011.03.003
Moura JM, Farias BS, Rodrigues DAS, Moura CM, Dotto GL, Pinto LAA (2015) Preparation of chitosan with different characteristics and its application for biofilms production. J Polym Environ 23:470–477. https://doi.org/10.1007/s10924-015-0730-y
Oliveira EG, Duarte JH, Moraes K, Crexi VT, Pinto LAA (2010) Optimisation of Spirulina sp. platensis convective drying: evaluation of phycocyanin loss and lipid oxidation. Int J Food Sci Technol 45:1572–1578. https://doi.org/10.1111/j.1365-2621.2010.02299.x
Piccin JS, Vieira MLG, Gonçalves JO, Dotto GL, Pinto LAA (2009) Adsorption of FD&C Red No. 40 by chitosan: isotherms analysis. J Food Eng 95:16–20. https://doi.org/10.1016/j.jfoodeng.2009.03.017
Rocha LS, Almeida A, Nunes C, Henriques B, Coimbra MA, Lopes CB, Silva CM, Duarte AC, Pereira E (2016) Simple and effective chitosan-based films for the removal of Hg from waters: equilibrium, kinetic and ionic competition. Chem Eng J 300:217–229. https://doi.org/10.1016/J.CEJ.2016.04.054
Saha TK, Bishwas RK, Karmaker S, Islam Z (2020) Adsorption characteristics of Allura Red AC onto sawdust and hexadecylpyridinium bromide-treated sawdust in aqueous solution. ACS Omega 5:13358–13374. https://doi.org/10.1021/acsomega.0c01493
Salem MA, Elsharkawy RG, Ayad MI, Elgendy MY (2019) Silver nanoparticles deposition on silica, magnetite, and alumina surfaces for effective removal of Allura red from aqueous solutions. J Sol-Gel Sci Technol 91:523–538. https://doi.org/10.1007/s10971-019-05055-7
Silva JM, Farias BS, Grundmann DDR, Cadaval TRS, Moura JM, Dotto GL, Pinto LAA (2017) Development of chitosan/ Spirulina sp. bio-blend films and its biosorption potential for dyes. J Applied Polym Sci 134:1–8. https://doi.org/10.1002/app.44580
Tolaimate A, Desbrières J, Rhazi M, Alagui A, Vincendon M, Vottero P (2000) On the influence of deacetylation process on the physicochemical characteristics of chitosan from squid chitin. Polymer 41:2463–2469. https://doi.org/10.1016/S0032-3861(99)00400-0
Tran HV, Hoang LT, Huynh CD (2020) An investigation on kinetic and thermodynamic parameters of methylene blue adsorption onto graphene-based nanocomposite. Chem Phys 535:110793. https://doi.org/10.1016/j.chemphys.2020.110793
Ullah R, Iftikhar FJ, Ajmal M, Shah A, Akhter MS, Ullah H, Waseem A (2020) Modified clays as an efficient adsorbent for brilliant green, ethyl violet and allura red dyes: kinetic and thermodynamic studies. Pol J Environ Stud 29:3831–3839. https://doi.org/10.15244/pjoes/112363
Vieira MLG, Martinez MS, Santos GB, Dotto GL, Pinto LAA (2018) Azo dyes adsorption in fixed bed column packed with different deacetylation degrees chitosan coated glass beads. J Environ Chem Eng 6:3233–3241. https://doi.org/10.1016/j.jece.2018.04.059
Vieira MLG, Pinheiro CP, Keli A, Silva KA, Lutke SF, Cadaval TRS, Dotto GL, Pinto LAA (2019) Chitosan and cyanoguanidine-crosslinked chitosan coated glass beads and its application in fixed bed adsorption. Chem Eng Commun 206:1474–1486. https://doi.org/10.1080/00986445.2019.1581618
Weska RF, Moura JM, Batista LM, Rizzi J, Pinto LAA (2007) Optimization of deacetylation in the production of chitosan from shrimp wastes: use of response surface methodology. J Food Eng 80:749–753. https://doi.org/10.1016/j.jfoodeng.2006.02.006
Yagub MT, Sen TK, Afroze S, Ang HM (2014) Dye and its removal from aqueous solution by adsorption: a review. Adv Colloid Interface Sci 209:172–184. https://doi.org/10.1016/j.cis.2014.04.002
Yu J, Han Y, Jong H, Jong HI, Ra G (2020) Two-step hydrothermal synthetic method of niobium-tungsten complex oxide and its adsorption of methylene blue. Inorganica Chim Acta 507:119562. https://doi.org/10.1016/j.ica.2020.119562
Funding
The authors would like to thank CAPES (Coordination for the Improvement of Higher Education Personnel) and CNPq (National Council for Scientific and Technological Development) for the financial support.
Author information
Authors and Affiliations
Contributions
Conceptualization: [Mery L. G. Vieira, Luiz A. A. Pinto]; Methodology: [Cláudio P. Pinheiro, Keli A. Silva], Formal analysis and investigation: [Mery L. G. Vieira, Tito R. S. Cadaval Jr, Guilherme L. Dotto]; Writing—original draft preparation: [Mery L. G. Vieira, Tito R. S. Cadaval Jr, Luiz A. A. Pinto]; Writing—review and editing: [Tito R. S. Cadaval Jr, Luiz A. A. Pinto]; Funding acquisition: [Tito R. S. Cadaval Jr, Luiz A. A. Pinto]; Supervision: [Luiz A. A. Pinto]. All authors read and approved the final manuscript.
Corresponding author
Ethics declarations
Ethics approval
Not applicable.
Consent to participate
Not applicable.
Consent for publication
Not applicable.
Competing interests
The authors declare no competing interests.
Additional information
Responsible Editor: Philippe Garrigues
Publisher's note
Springer Nature remains neutral with regard to jurisdictional claims in published maps and institutional affiliations.
Rights and permissions
About this article
Cite this article
Vieira, M.L.G., Pinheiro, C.P., Silva, K.A. et al. Development of adsorbent rigid structure based on Spirulina sp./chitosan bioblends coatings for dye adsorption in fixed bed column. Environ Sci Pollut Res 29, 79466–79477 (2022). https://doi.org/10.1007/s11356-022-21372-x
Received:
Accepted:
Published:
Issue Date:
DOI: https://doi.org/10.1007/s11356-022-21372-x