The current study evaluates chemical constituents and estimates the antimicrobial, antioxidant, ferrous-ion chelating, tyrosinase inhibition, cytotoxic, and radical scavenging activities of the essential oil of Artemisia turanica Krasch. from northeastern Iran. The antibacterial activity of the oil was evaluated by determination of inhibition zones, minimal inhibitory concentration, minimum bactericidal concentration and decimal reduction time. Phenolic content of the oil was determined using the Folin – Ciocalteu assay. Antioxidant properties of the oil of A. turanica were determined by three methods: the ferric reducing antioxidant power, bleaching of DPPH or radical scavenging activity, and β-carotene-linoleic acid assay. In vitro cytotoxicity was assessed by the MTT assay. Oxygenated monoterpenes, especially 1,8-cineole (35.2%), α-thujone (24.2%), and cis-chrysanthenol (16.8%) were the major components in the oil of A. turanica. Bactericidal kinetics of this oil indicated that E. coli is the most vulnerable (MIC = 2.5 mg/mL, D = 6.43 min). Total phenolic content of the oil was found to be 237.87 ± 6.66 μg GAE/mg oil. The oil exhibited a dose-dependent scavenging of DPPH, nitric oxide, and superoxide anion radicals with IC50 values of 7.00 mg/mL, 9.69 μg, and 14.63 μg, respectively. Ferrous-ion chelating activity of the oil (IC50 = 16.97 μg) was lower than that of EDTA. IC50 values for Hela and lymphocyte cells were calculated to be 17.67 and 3291.49 μg/mL, respectively. The results suggest application of A. turanica oil as a natural antioxidant and anticancer agent.
Similar content being viewed by others
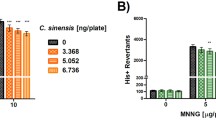
Avoid common mistakes on your manuscript.
Introduction
The genus Artemisia belongs to the important family Compositae (Asteraceae) [1].Within this family, Artemisia is included into tribe Anthemideae that comprises over 500 species. Among these, 34 have been reported in Iran, some of them are endemic [2]. The genus Artemisia has always been of great botanical and pharmaceutical interest and is useful in traditional medicine for the treatment of a variety of diseases and complaints [3]. Among these species, Artemisia turanica Krasch. grows naturally in wide regions of Iran.
In our previous investigation it has been shown that the extract of A. turanica has antimalarial effects in vivo against murine malaria model [4]. Previously, the essential oil of A. turanica has not been characterized with respect to its biological, toxicological and pharmaceutical activities. Therefore, the aims of this work were to study the total phenolic content, ferrous ion chelating, tyrosinase inhibition, antimicrobial, antioxidant, anticancer, cytotoxic, and radical scavenging activities of A. turanica essential oil growing wild in Iran.
Experimental Chemical Part
Plant material. The aerial parts of A. turanica were collected from Baam village, after Gahreman abad in Esfarayen, Province of Khorasan, northeastern Iran in May 2011. Voucher specimens have been deposited at the Herbarium of the Research Institute of Forests and Rangelands (TARI), Tehran, Iran. Plant specimens were identified by Dr. Vali-Aallah Mozaffarian from the same institute.
Isolation of essential oil. The leaves of A. turanica were dried at room temperature for several days. Air-dry leaves of A. turanica (100 g) were separately subjected to hydrodistillation using a clevenger-type apparatus for 3 h. After decanting and drying of the oil over anhydrous sodium sulfate, the oil was recovered. Results showed that essential oil yield was 0.8% (w/w).
Analysis of Essential Oil
The composition of the essential oil obtained by hydrodistillation from the leaves of A. turanica was analyzed by the gas chromatography (GC) and gas chromatography-mass spectrometry (GC/MS) techniques. Identification of the oil constituents was achieved by comparison of their mass spectra and retention indices (RIs) with those reported in the literature and those of authentic samples [5].
Gas chromatography. GC analysis was performed on a Schimadzu 15A gas chromatograph equipped with a split/splitless injector (250°C) and a flame ionization detector (250°C). Nitrogen was used as a carrier gas at a flow rate of 1 mL/min. The capillary column was DB-5 (50 m × 0.2 mm; film thickness, 0.32 μm). The column temperature was kept at 60°C for 3 min, then heated to 220°C at a 5°C/min rate, and then kept constant at 220°C for 5 min. Relative percentages were calculated from peak areas by a Schimadzu C-R4A Chromatopac Data Processor without the use of correction factors.
Gas chromatography/mass spectroscopy. GC/MS analysis was performed using a Hewlett-Packard 5973 instrument with an HP-5MS column (30 m × 0.25 mm; film thickness, 0.25 μm). The column temperature was kept at 60°C for 3 min, programmed to 220°C at a rate of 5°C/min, and kept constant at 220°C for 5 min. The flow rate of helium as carrier gas was 1 mL/min. The mass spectra were taken at electron-impact energy of 70 eV. The RIs for all components were determined according to the van den Dool method, using n-alkanes as standards. The compounds were identified by comparison of their relative retention indices (RRI, DB5) with those reported in the literature and by comparison of their mass spectra with the Wiley library or with the published mass spectra [5].
Antioxidant Activity
Ferric-reducing antioxidant power (FRAP) assay of the oil. The FRAP assay was carried out according to the procedure employed by Lim, et al. [6]. Aliquot (1 mL) of the extract dilution was added to 2.5 mL of 0.2 M potassium phosphate buffer (pH 6.6) and 2.5 mL 1% potassium ferricyanide. The mixture was incubated for 20 min at 50°C, after which 2.5 mL of 10% trichloroacetic acid was added. The mixture was then divided into 2.5 mL aliquots, each mixed with 2.5 mL of deionized water. Then, 0.5 mL of 0.1% (w/v) FeCl3 was added to each tube and allowed to stand for 30 min. Absorbance for each tube was measured at 700 nm. The FRAP was expressed in units of gallic acid equivalent (mg GAE per gram sample): y = 16.263x–0.0699; R 2 = 0.9944.
Bleaching of 2,20-diphenylpicrylhydrazyl (DPPH). The hydrogen atom or electron donation abilities of the corresponding extracts and some pure compounds were measured from bleaching of the purple-colored methanol solution of 2,2-diphenylpicrylhydrazyl (DPPH). This spectrophotometric assay uses stable DPPH radical as a reagent. Aliquots (50 μL) of 1:5 dilution of the essential oil in methanol were added to 5 mL of 0.004% methanol solution of DPPH. Trolox (1 mM) (Sigma-Aldrich), a stable antioxidant, was used as a synthetic reference. The essential oil from Thymus x-porlock was used as a natural reference. After 30 min incubation period at room temperature, the absorbance was read against blank at 517 nm. Percentage inhibition of free radical by DPPH (I%) was calculated in the following way [7]:
where Ablank is the absorbance of the control reaction (containing all reagents except the test compound), and Asample is the absorbance of the test compound. Tests were carried out in triplicate (y = 3.8166x + 23.271; R 2 = 0.9704).
β-Carotene–linoleic acid assay. Antioxidant activity of the essential oil was determined using the β-carotene bleaching test [8]. Approximately 10 mg of β-carotene (type I synthetic, Sigma-Aldrich) was dissolved in 10 mL chloroform. The carotene-chloroform solution, 0.2 mL, was pipetted into a boiling flask containing 20 mg linoleic acid (Sigma-Aldrich) and 200 mg Tween 40 (Sigma-Aldrich). Chloroform was removed using a rotary evaporator at 40°C for 5 min. To the residue, 50 mL distilled water was added slowly with vigorous agitation to form an emulsion. The emulsion (5 mL) was added to a tube containing 0.2 mL essential oil solution, prepared, and the absorbance was immediately measured at 470 nm against a blank consisting of the emulsion without β-carotene. The tubes were placed in a water bath at 50°C and oxidation of the emulsion was monitored spectrophotometrically by measuring absorbance at 470 nm over a 60 min period. Control samples contained 10 μL water instead of essential oil. Butylated hydroxy anisole (BHA; Sigma-Aldrich), a stable antioxidant, was used as a synthetic reference. The antioxidant activity (AA) was expressed as inhibition percentage with reference to the control after 60 min of incubation, using the following equation:
where DRC is the degradation rate of the control [ln(a/b)/60], DRS is the degradation rate in the presence of a sample [ln(a/b)/60], a is the absorbance at time 0, and b is the absorbance at 60 min (y = 75.461x + 36.561; R 2 = 0.9743).
Total Phenolic Content Assay
Total phenolic content (TPC) of the oil was determined using the Folin – Ciocalteu assay [9]. Samples (300 μL) were introduced into test tubes, followed by 1.5 mL of the Folin – Ciocalteu reagent (10 x dilutions) and 1.2 mL of sodium carbonate (7.5% w/v). The tubes were allowed to stand for 30 min before measuring absorbance at 765 nm. TPC was expressed as mg GAE per 100 g material:
Radical Scavenging Activity
Nitric oxide radical scavenging. The ability of the extract to scavenge nitric oxide free radicals was determined using a modification of the method described by Marcocci, et al. [10]. In brief, 0.5 mL aliquot of extract (1 mg/mL) or positive control (1 mg/mL) dissolved in KH2PO4–KOH (50 mmol/L, pH 7.4) was mixed with 0.5 mL of (10 mmol/L) sodium nitroprusside solution. The mixture was incubated at 37°C for 2.5 h under normal light condition. After incubation, the sample was placed in dark for 20 min. Then, 1 mL of Griess reagent (1g/lN-(1-naphtyl)ethylenediamine and 10 g/L sulphanilamide dissolved in 20 ml/L aqueous H3PO4) was added and the absorbance at 546 nm was taken after 40 min. The percentage inhibition was calculated using the following formula (y = 2.3467x + 27.259; R 2 = 0.993):
Superoxide anion radical scavenging. The ability of the oil to scavenge superoxide anion radicals was determined by the method described by Lee, et al. [11]. In brief, to 100 μL aliquot of dissolved oil the following was added: 100 μL (30 mmol/L) Na2 EDTA, 100 μL (3 mmol/L) hypoxanthine in 50 mmol/L NaOH and 200 μL (1.42 mmol/L) nitroblue tetrazolium (NBT) in NaH2PO4–NaOH (50 mmol/L, pH 7.4). After 3 min incubation period at room temperature, 100 μL (0.5 U/mL) xanthine oxidase in the NaH2PO4–NaOH buffer was added, followed by 2.4 mL NaH2PO4–NaOH buffer. The resulting solution was incubated at room temperature for 20 min and the absorbance at 560 nm was measured. The absorbance was also measured at 293 nm to detect if the oil inhibited uric acid generation. Once it was confirmed that uric acid formation is not inhibited, the percentage inhibition at 560 nm was calculated using the following equation:
and IC50 values were estimated using a linear regression (y = 1.5587x + 27.194; R 2 = 0.9811).
Ferrous-Ion Chelating (FIC) Assay
FeSO4 (2 mM) and ferrozine (5 mM) were prepared and 20 times diluted oil (250, 500 and 1000 μL diluted to 1 mL) was mixed with 1 mL diluted FeSO4, followed by 1 mL of diluted ferrozine. The tubes were mixed well and allowed to stand for 10 min at room temperature. Absorbance of each oil dilution was measured against blank at 562 nm [6]. The ability of the sample to chelate ferrous ions was calculated and expressed as (sample: y = 1.1428x + 30.606; R 2 = 0.9803), (EDTA: y = 16.226x + 34.893; R 2 = 0.9871):
Tyrosinase Inhibition
Tyrosinase inhibitory activity was determined by a spectrophotometric method as described by Chan, et al. [12] using a modified dopachrome method with L-DOPAas the substrate. A 5 mg aliquot of the oil was weighed and dissolved in 2 mL 50% DMSO. Then, 40 μL of sample was added to 80 μL of 0.1 M phosphate buffer (pH 6.8), 40 μL of 0.02 mg/mL tyrosinase and 40 μL of L-DOPA (2.5 mM) in wells of a 96-well test plate. The samples were incubated for 30 min at 37°C. Each sample was accompanied by a blank that contained all components except L-DOPA. Absorbance was measured at 475 nm. Results were compared with the control containing 50% DMSO instead of the sample solution. Quercetin was used as the positive control. The percentage of tyrosinase inhibition was calculated as
Anti-tyrosinase activity of the oil (y = 4.6504x + 17.338; R 2 = 0.9948) was also expressed as quercetin equivalent (QE, mg/g of oil) which was obtained from the following standard curve: y = 11.872x + 16.506; R 2 = 0.993, where, y represents % inhibition and x represents concentration in mg.
Results of Chemical Testing
Chemical Composition of Essential Oil
The composition of the essential oil obtained by hydrodistillation from the leaves of A. turanica growing in Iran, as analyzed by GC and GC/MS, is listed in Table 1, where the percentage contents and RIs of components are given. In the leaf oil of A. turanica, 11 components, representing 94.1% of the total composition, have been identified, including 1,8-cineole (35.2%), α-thujone (24.2%) and cis-chrysanthenol (16.8%) as the main components in this oil. The other major components of this essential oil were terpinen-4-ol (4.2%) and α-terpinyl acetate (2.6%). As can be seen from these data, the leaf oil of A. turanica is rich in oxygenated monoterpenes (90.9%). In contrast, the aerial parts of A. turanica Krasch, which were collected from the Marzdaran region (northern Khorasan province, Iran), in November 2002, revealed the main identified compounds in the volatile oil of A. turanica to be 1,8-cineole (40.9%), cis-verbenyl acetate (19.0%), and camphor (11.0%) [13].
Antioxidant Activity and Total Phenolic Content
Total phenolic content. The TPC of the oil of A. turanica was found to be 237.87 ± 6.66 μgg GAE/mg oil. The phenolic assay involving an electron-transfer reaction evaluated by using the Folin – Ciocalteu reagent. TPC measures both types of antioxidants, hydrophobic and hydrophilic form complexes with Fe2+. Phenolic and flavonoid compounds are known to inhibit lipid peroxidation by quenching lipid peroxy radicals and reduce or chelate iron in lipoxygenase enzyme and thus prevent initiation of lipid peroxidation reaction.
Antioxidant activity. Antioxidant properties of the essential oil of A. turanica was determined by three methods: ferric-reducing antioxidant power (FRAP), radical-scavenging capacity of the oil (or DPPH bleaching), and β-carotene–linoleic acid assay. Data on the antioxidant activity of the essential oil are shown in Table 2. The FRAP, expressed as GAE or known Fe(II) concentration, was found to be 0.343 ± 0.02 mg GAE/g. The percentage inhibition of DPPH activity was 60.30 ± 0.88% (10 mg/mL of oil) with IC50 = 7.00 mg/mL. The radical scavenging activity of the essential oil of A. turanica was compared to BHT, BHA and Trolox as standards. The oil of A. turanica at 10 mg/ml concentration was found to be 1.57 times more potent than BHT, 1.22 times more potent than BHA, and 1.76 times more potent than Trolox. In β-carotene-linoleic acid test system, oxidation of linoleic acid was also effectively inhibited by A. turanica oil (59.27 ± 1.48% at 0.312 mg/mL of essential oil). The β-carotene–linoleic acid assay of the essential oil of A. turanica was performed in the presence of BHT and BHA as standards.
Many different methods have been established for evaluating the antioxidant capacity of certain biological samples. These methods are classified, roughly, into two categories based upon the nature of the reaction that the method involved [14]. The methods involving an electron-transfer reaction include the DPPH radical-scavenging, ferric-reducing antioxidant power, and β-carotene–linoleic acid assay. The essential oil of A. turanica exhibited dose-dependent scavenging of DPPH radicals, and 7.00 mg of the oil was sufficient to scavenge 50% of DPPH radicals/mL. The DPPH radical scavenging is a sensitive antioxidant assay and is independent of substrate polarity [15]. DPPH is a stable free radical that can accept an electron or hydrogen radical to become a stable diamagnetic molecule [16]. A significant correlation was shown to exist between the TPC and DPPH scavenging capacity for each sample.
In lipid peroxidation inhibition (LPI) test, the oxidation of linoleic acid was effectively inhibited by A. turanica oil (59.27%, at 0.312 mg/mL). Results such as the relative abundance of phenolic compounds, and the significant correlations that existed between phenolic content and antioxidant capacity, as measured by β-carotene or DPPH scavenging methods, would appear to be highly consistent with the corresponding results presented by other researches [17]. In general, the antioxidant and radical scavenging properties of plant oils and extracts are associated with the presence of phenolic compounds possessing the ability to donate hydrogen to the radical. Numerous reports indicated good correlation between the antioxidant activity and the concentration of phenolic compounds measured by Folin – Ciocalteu method. A great number of simple phenolic compounds as well as flavonoids can act as antioxidants, however, their antioxidant power depends on some important structural prerequisites, particularly on the number and arrangement of hydroxyl groups, the extent of structural conjugation, and the presence of electron-donating and electron-accepting substituents on the ring structure [18].
Radical Scavenging Properties, Chelating Ability and Anti-Tyrosinase Activity
Nitric oxide radical scavenging activity. The nitric oxide radical-scavenging activities of the oil of A. turanica are presented in Table 3. The oil showed 63.86 ± 2.66% NO• scavenging activity at 16 μg and produced 50% nitric oxide radical scavenging (IC50) at 9.69 μg. According to the results, the percentage of nitric oxide radical scavenging activity was also increased with increasing concentration of the oil. Increased levels of nitric oxide can be found in certain spasmodic conditions, for example, allergic rhinitis, adult respiratory distress syndrome, and asthma immediate and late phase [19]. In addition to reactive oxygen species, nitric oxide is also implicated in inflammation, cancer, and other pathological conditions [20]. Radical scavenging properties of plant oils are associated with the presence of phenolic compounds possessing the ability to donate hydrogen to the radical.
Superoxide anion radical scavenging activity. Data on the superoxide anion radical scavenging activity of the essential oil of A. turanica are presented in Table 3. The oil exhibited a dose-dependent scavenging of superoxide anion radicals and 14.63 μg of the A. turanica oil was sufficient to scavenge 50% of superoxide anions. Superoxide radicals are produced in human body by various oxidative enzymes in the form of one-electron reduction of molecular oxygen. Xanthine oxidase is one of the major oxidative enzymes to produce superoxide radical as a result in tissue injury [21]. In vitro superoxide radical was generated by xanthine oxidase during the test reaction; NBT undergoes oxidation and leads to water-soluble blue formazan [22]. The decrease in blue color formation after adding the solvent fractions in the reaction mixture was measured as superoxide radical scavenging. Percentage of superoxide radical scavenging activity and inhibition of uric acid formation increased with increasing concentration of the oil. The maximum percentage superoxide radical scavenging activity of the oil was 60.85 ± 3.13% at 22.24 μg.
Ferrous-ion chelating assay. As shown in Table 4, the strongest iron chelating activity was noticed at a concentration of 31.5 μg (65.43 ± 8.17%), while the concentration of 3.93 μg exhibited the lowest activity (33.30 ± 8.21%). The ferrous ion chelating activity increased with the increasing concentration. EDTA which serves as the positive control shows the highest percentage of the chelating effect (60.17 ± 2.04%) at the concentration of 1.6 μg. The FIC assay is a common test used to determine the secondary antioxidant activity by observing the reducing purple color of the reaction solution. The assay mechanism is based on the decrease in the absorbance of iron(II)–ferrozine complex. Meanwhile, secondary antioxidants are also known as the peroxide decomposers, which inhibit polypropylene oxidation by decomposing hydroperoxide. Secondary antioxidants are responsible for suppressing the formation of radicals and protecting against oxidative damage [23]. Iron-ferrozine complex exhibits maximum absorbance at 562 nm and large decrease in absorbance indicates strong chelating power. By forming a stable iron(II) chelate, an extract with a high chelating power reduces free ferrous ion concentration, which leads to decrease in the extent of Fenton reaction that is involved in many diseases [23]. Iron is known to generate free radicals through the Fenton and Haber – Weiss reaction. Fenton – Weiss reaction is a reaction between ferrous ion and hydrogen peroxide which produces highly reactive hydroxyl radicals implicated in many diseases [24]. Metal ion-chelating capacity plays a significant role in antioxidant mechanism since it reduces the concentration of catalytic transition metal in lipid oxidation [25]. In this research, the maximum percentage of iron chelating activity of the oil obtained 65.43% at 31.5 μg of the oil. The activity of EDTA was observed to be the highest (60.17%) at an amount of 1.6 μg and the lowest (37.10%) at 0.2 μg. the IC50 of the oil of A. turanica was 16.97 μg, while the IC50 of EDTA was 0.931 μg. As shown in Table 4, the ferrous-ion chelating activity of the oil was lower than that of EDTA. EDTA showed excellent chelating ability. There was a correlation between ferrous-ion chelating activity and total phenolic content, implying that the oil contained chelating ligands.
Tyrosinase inhibition. Anti-tyrosinase activity of A. turanica oil was expressed in quercetin equivalent (QE) units. The results are presented in Table 4. The maximum percentage of anti-tyrosinase activity of A. turanica oil was 33.84 ± 0.84% at 1.46 mg QE. The anti-tyrosinase activity of A. turanica oil at 50% inhibition (IC50) was 7.02 mg. Determination of the tyrosinase inhibitory activity due to treatment with essential oils serves a useful target in the treatment of hyper-pigmentation skin disorder [26]. The obtained results showed that the essential oil possesses increased reducing power which forms the basis for exploring the tyrosinase inhibitory potential of plant. Tyrosinase is a copper containing enzyme, hence any substance which reduces this metal ion was considered as an effective tyrosinase inhibitor [27]. Essential oils are found to be rich in compounds containing a hydrophobic part, which would act as competitive inhibitors on the enzyme tyrosinase and thereby on melanin synthesis. Hence, the determination of tyrosinase inhibitory potential of the present essential oil may lead to develop skin whitening agents [28]. In this study, the maximum percentage of anti-tyrosinase activity of the essential oil of A. turanica was 33.84 ± 0.84% at 3.58 mg oil. Quercetin was used as positive control. The maximum percentage of tyrosinase inhibition by quercetin was 40.05 ± 4.30% at an amount of 2 mg. However, the activity of quercetin was much more pronounced than that of the essential oil of A. turanica. The reducing power reported might be due to phytoconstituents such as phenolics, carbonyl compounds, and also some other constituents present in crude essential oils. The possible mechanism underlying the tyrosinase inhibitory ability might be chelation of copper ion present in tyrosinase enzyme by phytoconstituents and thereby suppression of tautomerization to dopochrome by the oils, thereby the oils act as reducing agents on melanin intermediates by blocking oxidation chain reaction at various points from tyrosinase/DOPA to melanin and hence causing reduction of skin pigmentation [29]. The effect of antioxidant phytochemicals in biological systems is defense of their ability to scavenge radicals, chelate metals, activate antioxidant enzymes, and inhibit oxidases.
Experimental Biological Part
Antimicrobial Activity
Oil dilution solvent. Bacterial strains were streaked on Mueller Hinton agar plates using sterile cotton swabs. Aliquots (5 μL) of dimethylsulfoxide (DMSO) loaded on sterile blank disks were placed on the agar plates and were incubated at 37°C for 24 h. There was no antibacterial activity on the plates and hence DMSO was selected as a safe diluting agent for the oil. Five microliters of each oil dilution, followed by sterilization using 0.45 μm membrane filter, were added to sterile blank discs. The same solvent also served as control.
Microbial strains and growth media. Escherichia coli (ATCC25922), Staphylococcus aureus (ATCC25923), Pseudomonas aeruginosa (ATCC8830), Candida albicans (ATCC 5027) and Acinetobacter baumannii (ATCC 17978) were employed in the study. Nutrient agar was used. Bacterial suspensions were made in brain heart infusion (BHI) broth to a concentration of approximately 108 cfu/ml. Subsequent dilutions were made from these suspensions and used in the tests.
Oil sterility test. In order to ensure sterility of the oils, geometric dilutions ranging from 0.036 to 72.0 mg/mL of the essential oil were prepared in a 96-well microtitre plate, including one growth control (BHI + Tween 80) and one sterility control (BHI + Tween 80 + test oil). Plates were incubated under normal atmospheric conditions at 37°C for 24 h. The contaminating bacterial growth, if at all, was indicated by the presence of a white ‘’pellet” on the well bottom.
Disc diffusion method. The agar disc diffusion method was employed for the determination of antimicrobial activities of the essential oils in question. Briefly, 0.1 mL from 108 cfu/mL bacterial suspension was spread on the Mueller Hinton Agar (MHA) plates. Filter paper discs (6 mm in diameter) were impregnated with 5 μL of undiluted oil and placed on the inoculated plates. These plates, after keeping at 4°C for 2 h, were incubated at 37°C for 24 h. The diameters of the inhibition zones were measured in millimeters. All tests were performed in triplicate.
Determination of minimum inhibitory (MIC) and bactericidal (MBC) concentrations. All tests were performed in BHI broth supplemented with Tween 80 detergent (final concentration of 0.5% v/v). Test strains were suspended in BHI broth to give a final density of 107 cfu/mL as confirmed by viable counts. The MIC and MBC were assessed according to our modified procedure [30]. The MIC was determined by the broth dilution method in test tubes as follows: 40 μL aliquot from each oil dilution was added to 5 mL of BHI broth in tubes containing 107 cfu/mL live bacterial cells. The tubes were then incubated on an incubator shaker to evenly disperse the oil throughout the broth volume in tubes. The highest dilution (lowest concentration) showing no visible bacterial growth was regarded as the MIC. Cell suspensions (0.1 mL) from the tubes showing no growth were subcultured on BHI agar plates in triplicate to determine if the inhibition was reversible or permanent. The MBC was determined as the highest dilution (lowest concentration) at which no growth occurred on the plates.
Bactericidal kinetics of the oil. Aliquots (40 μL) of each oil at the dilution determined by MBC was added to each 5 mL of BHI broth in tubes containing bacterial suspension of 107 cfu/ml and were then incubated at 37°C in an incubator shaker. Samples (0.1 mL) were taken after 5, 10, 15, 20, 25, 30, 45, 90, 120, 150, 180, 210 and 240 min. The samples were immediately washed with sterile phosphate buffer, pH 7.0, centrifuged at 10000 rpm, resuspended in the buffer, and spread-cultured on BHI agar for 24 h at 37°C. Phosphate buffer was used as diluent when needed. Bactericidal experiments were repeated three times. Microbial colonies were counted from triplicates after the incubation period and the mean total number of viable cells per mL was calculated. The mean total number of viable bacteria from bactericidal kinetics experiments at each time interval was converted to log10 viable cells using routine mathematical formulas. The trend of bacterial death was plotted graphically.
Cytotoxicity Assay
The human cervical carcinoma Hela cell lines NCBI code No. 115 (ATCC number CCL-2) were obtained from Pasteur Institute, Tehran, Iran. The cells were grown in RPMI 1640 supplemented with 10% fetal calf serum, 1% (w/v) glutamine, 100 U/mL penicillin, and 100 μg/mL streptomycin. Cells were cultured in a humidified 5% CO2 atmosphere at 37°C. Cytotoxicity was measured using a modified MTT assay [31]. This assay detects the reduction of MTT [3-(4,5-dimethylthiazolyl)-2,5-diphenyltetrazolium bromide] by mitochondrial dehydrogenase to blue formazan product, which reflects the normal functioning of mitochondrial and cell viability [32]. Briefly, the cells (5 × 104) were seeded in each well containing 100 μL of RPMI medium supplemented with 10% FBS in a 96-well plate. After 24 h adhesion, serial double dilutions of the essential oil were added to triplicate wells over the range of 1.0 – 0.005 μL/mL. The final concentration of ethanol in the culture medium was maintained at 0.5% (v/v) to avoid toxicity of the solvent [33]. After 2 days, 10 μL of MTT (5 mg/mL stock solution) was added and the plates were additionally incubated for 4 h. The medium was discarded and the formazan blue, which formed in the cells, was dissolved in 100 μL DMSO. The optical density was measured at 490 nm using a microplate ELISA reader. The cell survival curves were calculated from cells incubated in the presence of 0.5% ethanol. Cytotoxicity was expressed as the concentration of drug inhibiting cell growth by 50% (IC50). All tests and analyses were run in triplicate and mean values were recorded (Hela cells: y = 1.2827 x + 27.327, R 2 = 0.9815; lymphocyte cells: y = 0.006 x + 27.976; R 2 = 0.9787).
Statistical Analysis
All analyses and tests were run in triplicate and mean values recorded. All the experimental data are presented as mean ± SEM of three individual samples. Data are presented as percentage of inhibition or radical scavenging on different concentration of A. turanica oil. IC50 (the concentration required to scavenge/inhibit 50% of free radicals/tyrosinase or lipid peroxidation) value was calculated from the dose-response curves. Antibacterial effect was measured in terms of zone of inhibition to an accuracy of 0.1 mm and the effect was calculated as a mean of triplicate tests. All of the statistical analyses were performed by means of Microsoft Office Excel 2007 software.
Results of Biological Activity Evaluation
Antimicrobial Assays
Antibacterial and antifungal activities of the oil of A. turanica were tested by three methods: (i) agar diffusion method; (ii) determination of minimum inhibitory concentration (MIC) and minimum bactericidal concentrations (MBC), and (iii) bactericidal kinetics of the oil, using serial dilutions, viz., 2.5, 5, and 10 mg/mL. The data on antibacterial activity of the essential oil of A. turanica are presented in Table 5. Maximum inhibition was observed against Staphylococcus aureus (20.50 mm) followed by Escherichia coli (20.17 mm), Candida albicans (19.67 mm), Pseudomonas aeruginosa (14.33 mm) and Acinetobacter baumannii (13.17 mm) at a concentration of 10 mg/mL of the oil. The oil of A. turanica exhibited moderate inhibitory activity against all tested microorganisms. In the present study, determination of MBC and MIC from the oil of A. turanica indicated that all the test organisms were approximately sensitive to the oil, but E. coli was the most vulnerable. Determination of the inhibition zone showed the oil of A. turanica was bactericidal in the following order: S. aureus > E. coli > C. albicans > P. aeruginosa > A. baumannii (Fig. 1). The results showed that E. coli and C. albicans have the minimum MIC and MBC values of A. turanica oil (2.5 and 5 mg/mL, respectively). Complete death time on exposure to A. turanica oil was 6.43 min for E. coli. Bactericidal kinetics of the oil of A. turanica indicated that E. coli is the most vulnerable strain.
Cytotoxicity Assay
Cytotoxicity was measured using a modified MTT assay [31]. The cytotoxic effects of the essential oil of A. turanica were tested on lymphocyte and Hela cells. As shown in Table 6, at a concentration of 28 μg/ml, oil destructed Hela cells by 62.53%. At lower doses, the oil was tolerated by the cells and its 50% cytotoxic concentration was 17.67 μg/ml. The oil displayed an excellent cytotoxic action towards the human tumor cell line. On the other hand at a concentration of 5600 μg/ml, oil destructed lymphocyte cells by 60.62%. At a concentration of 28 μg/mL, oil destructed Hela cells by 62.53%. This makes the tested oil certainly deserve some further investigation. Although all in vitro experiments hold limitations with regards to possible in vivo efficacy, the results of this study are very promising with regards to possible antineoplastic chemotherapy and form a very sound basis for future research.
The IC50 values for Hela and lymphocyte cells were calculated to be 17.67 μg/mL and 3291.49 μg/mL, respectively. The IC50 shows that cytotoxicity of the oil toward human tumor cell line is much higher than that required for healthy human cells. These results indicate low adverse side effects of the oil. Cancer chemoprevention is defined as the use of chemicals or dietary components to block, inhibit, or reverse the development of cancer in normal or pre-neoplastic tissue.
A large number of potential chemopreventive agents have been identified, and they function by mechanisms directed at all major stages of carcinogenesis [34]. Essential oil constituents have very different modes of action in bacterial and eukaryotic cells. For bacterial cells, they exhibit having strong bactericidal properties, while in eukaryotes they modify apoptosis and differentiation, interfere with the post-translational modification of cellular proteins, and induce or inhibit some hepatic detoxifying enzymes. So, essential oils may induce very different effects in prokaryotes and eukaryotes [35]. In another investigation, the cytotoxic activity of A. turanica essential oil was investigated using a potato disc tumor inhibition method. The essential oil of A. turanica exhibited tumor growth inhibition (100%) at concentrations equal to 0.02% (w/v) and higher [36].
Even though essential oil might be not ideal for the treatment of human cancers, the oil tested certainly deserves some further investigation. The results of this study deserve attention with regard to possible anti-neoplastic chemotherapy that provides a basis for future research. From the obtained results, it can be concluded that the oil of A. turanica exhibited antimicrobial activity against tested microorganisms and it could be a natural radical scavenger and antioxidant agent. The cytotoxicity of the oil of A. turanica toward human tumor cell line is much higher than that required for human healthy cells. These results indicate low adverse side effects of the oil. So, the essential oil of A. turanica may be exploited as a natural source of bioactive phytochemicals bearing antimicrobial, antioxidant and anticancer potentials that could be supplemented for medicinal applications.
References
K. H. Rechinger, Artemisia, in: Flora Iranica, No. 158, K. H. Rechinger and I. C. Hedge (eds.), Akademische Druck und Verlagsanstalt, Gras, Austria (1986).
V. A. Mozaffarian, Dictionary of Iranian Plant Names, Farhang Moaser, Tehran, Iran (1996).
A. Rustaiyan and S. Masoudi, Phytochem. Lett., 4(4), 440 – 447 (2011).
M. Taherkhani, A. Rustaiyan, H. Nahrevanian, et al., J. Vector Borne Dis., 50(1), 51 – 56 (2013).
R. P. Adams, Identification of Essential Oil Components by Gas Chromatography / Quadrupole Mass Spectroscopy, Allured Publ. Corp., Carol Stream, IL (2001).
T. Y. Lim, Y. Y. Lim and C. M. Yule, Food Chem., 114(2), 594 – 599 (2009).
D. Yadegarinia, L. Gachkar, M. B. Rezaei, et al., Phytochemistry, 67(12), 1249 – 1255 (2006).
M. S. Taga, E. E. Miller and D. E. Pratt, J. Am. Oil Chem. Soc., 61(5), 928 – 931 (1984).
M. P. Kahkonen, A. I. Hopia, H. J. Vuorela, et al., J. Agric. Food Chem., 47(10), 3954 – 3962 (1999).
L. Marcocci, J. J. Maguire, M. T. Droy-Lefaix and L. Packer, Biochem. Biophys. Res. Commun., 201(2), 748 – 755 (1994).
J. C. Lee, H. R. Kim, J. Kim and Y. S. Jang, J. Agric. Food Chem., 50(22), 6490 – 6496 (2002).
E. W. C. Chan, Y. Y. Lim, L. F. Wong, et al., Food Chem., 109(3), 477 – 483 (2008).
M. Hassanzadeh Khayyata and H. Karimi, J. Pharm. Sci., 1, 33 – 37 (2004).
D. Huang, O. U. Boxin and R. L. Prior, J. Agric. Food Chem., 53(6), 1841 – 1856 (2005).
T. Yamaguchi, H. Takamura, T. Matoba and J. Terato, Biosci. Biotechnol. Biochem., 62(6), 1201 – 1204 (1998).
S. C. Ho, T. H. Tsai, P. J. Tsai and C. C. Lin, Food Chem. Toxicol., 46(3), 920 – 928 (2008).
B. Shan, Y. Z. Cai, M. Sun and H. Corke, J. Agric. Food Chem., 53(20), 7749 – 7759 (2005).
G. Miliauskas, T. A. Van Beek, P. D. Waard, et al., J. Nat. Prod., 68(2), 168 – 172 (2005).
K. Ashutosh, Curr. Opin. Pulm. Med., 6, 21 – 25 (2000).
S. M. Nabavi, M. A. Ebrahimzadeh, S. F. Nabavi, et al., Pharmacologyonline, 2, 560 – 567 (2008).
H. Haraguchi, H. Ishikawa, K. Mizutani, et al., Bioorg. Med. Chem., 6 (3), 339 – 347 (1998).
I. Gulcin, M. Uguz and M. Oktay, Turk J. Agric. For., 28, 25 – 33 (2004).
Y. Y. Lim, T. T. Lim and J. H. Tee, Sunway Acad. J., 3, 9 – 20 (2006).
R. V. Lloyd, P. M. Hanna and R. P. Mason, Free Radical Biol. Med., 22(5), 885 – 888 (1997).
S. F. Che Othman, S. Z. Idid, M. Suleiman Koya, et al.,, Pertanika J. Trop. Agric. Sci., 34(2), 253 – 261 (2011).
A. Perez-Bernal, M. A. Munoz-Perez and F. Camacho, Am. J. Clin. Dermatol., 1(5), 261 – 268 (2000).
E. Amin, A. A. Saboury, H. Mansuri-Torshizi and A. A. Moosavi-Movahedi, J. Enzyme Inhib. Med. Chem., 25(2), 272 – 281 (2010).
S. Momtaz, B. M. Mapunya, P. J. Houghton, et al., J. Ethnopharmacol., 119(3), 507 – 512 (2008).
A. Slominski, D. J. Tobin, S. Shibahara, and J. Wortsman, Physiol. Rev., 84 (4), 1155 – 1228 (2004).
I. Rasooli and S. A. Mirmostafa, J. Agric. Food Chem., 51(8), 2200 – 2205 (2003).
S. M. Sharafi, I. Rasooli, P. Owlia, et al., Pharmacogn. Mag., 6(23), 147 – 153 (2010).
C. B. Lau, C. Y. Ho, C. F. Kim, et al., Life Sci., 75(7), 797 – 808 (2004).
N. Sylvestre, J. Legault, D. Dufour and A. Pichette, Phytomedicine, 12(4), 299 – 304 (2005).
R. M. Samarth, M. Panwar, M. Kumar, and A. Kumar, Mutagenesis, 21(1), 61 – 66 (2006).
J. R. Lazutka, J. Mierauskiene, G. Slapsyte and V. Dedonyte, Food Chem. Toxicol., 39(5), 485 – 492 (2001).
J. Behravan, M. Ramezani, M. K. Hassanzadeh, et al., J. Essent. Oil-Bear. Plants, 9(2), 196 – 202 (2006).
Acknowledgments
The author is grateful to the Islamic Azad University’s (Takestan Branch) research deputy office for the sanction of research grant to conduct the current research (Grant No. TIAU: 50155). I would like to thank sincerely Prof. Rustaiyan for his assistance in the botanical information, Prof. Iraj Rasooli in the medicinal plant research center of Shahed University for his scientific assistance, and Dr. Mozaffarian for help in identifying plant material.
Author information
Authors and Affiliations
Corresponding author
Rights and permissions
About this article
Cite this article
Taherkhani, M. In Vitro Cytobiochemical Potentials and Protective Effects of Bioactive Phytochemicals from Artemisia Turanica . Pharm Chem J 50, 668–677 (2017). https://doi.org/10.1007/s11094-017-1510-x
Received:
Published:
Issue Date:
DOI: https://doi.org/10.1007/s11094-017-1510-x