Abstract
Neurotensin behaves as a neuromodulator or as a neurotransmitter interacting with NTS1 and NTS2 receptors. Neurotensin in vitro inhibits synaptosomal membrane Na+, K+-ATPase activity. This effect is prevented by administration of SR 48692 (antagonist for NTS1 receptor). The administration of levocabastine (antagonist for NTS2 receptor) does not prevent Na+, K+-ATPase inhibition by neurotensin when the enzyme is assayed with ATP as substrate. Herein levocabastine effect on Na+, K+-ATPase K+ site was explored. For this purpose, levocabastine was administered to rats and K+-p-nitrophenylphosphatase (K+-p-NPPase) activity in synaptosomal membranes and [3H]-ouabain binding to cerebral cortex membranes were assayed in the absence (basal) and in the presence of neurotensin. Male Wistar rats were administered with levocabastine (50 μg/kg, i.p., 30 min) or the vehicle (saline solution). Synaptosomal membranes were obtained from cerebral cortex by differential and gradient centrifugation. The activity of K+-p-NPPase was determined in media laking or containing ATP plus NaCl. In such phosphorylating condition enzyme behaviour resembles that observed when ATP hydrolyses is recorded. In the absence of ATP plus NaCl, K+-p-NPPase activity was similar for levocabastine or vehicle injected (roughly 11 μmole hydrolyzed substrate per mg protein per hour). Such value remained unaltered by the presence of 3.5 × 10−6 M neurotensin. In the phosphorylating medium, neurotensin decreased (32 %) the enzyme activity in membranes obtained from rats injected with the vehicle but failed to alter those obtained from rats injected with levocabastine. Levocabastine administration enhanced (50 %) basal [3H]-ouabain binding to cerebral cortex membranes but failed to modify neurotensin inhibitory effect on this ligand binding. It is concluded that NTS2 receptor blockade modifies the properties of neuronal Na+, K+-ATPase and that neurotensin effect on Na+, K+-ATPase involves NTS1 receptor and -at least partially- NTS2 receptor.
Similar content being viewed by others
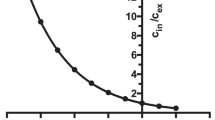
Avoid common mistakes on your manuscript.
Introduction
Neurotensin is a tridecapeptide widely distributed in the nervous system, at both central and peripheral levels. It can behave as a neurotransmitter or as a neuromodulator, exerting diverse biological actions [1]. Neurotensin effects are mediated by its binding to a group of receptors [2, 3]. Two of them, denominated NTS1 and NTS2 receptors, are seven transmembrane domain receptors which are coupled to G proteins. Another two neurotensin receptor types are mainly localized intracellularly and are termed NTS3/sortilin and nts4/SorLA [4].
Na+, K+-ATPase is a transmembrane enzyme with an intracellular site for Mg2+-ATP and Na+ and an extracellular site for K+, which binds ouabain and other cardiotonic glycosides. Ouabain site (K+ site) is regulatory for the enzyme activity. This enzyme catalyzes the hydrolyses of ATP in a two step process: a phosphorylation step and a dephosphorylation step, K+-dependent, sensitive to ouabain [5]. In the last step, it behaves as a non-specific phosphatase, hydrolyzing other phosphates, including p-nitrophenylphosphate (p-NPP), a non-natural substrate [see 6]. Na+, K+-ATPase and K+-p-nitrophenylphosphatase (K+-p-NPPase) activities are enriched in brain synaptosomal membranes [7].
It is known that a major fraction of cerebral energy production is consumed by the activity of Na+, K+-ATPase (Na,K pump) at synapses for the maintenance of Na/K equilibrium through neuronal membranes [5]. Experimental evidence suggests that neurotensin may modulate ionic transport at neuronal membranes, due to its ability to modify the activity of Na+, K+-ATPase. Assays performed in vitro in cerebral cortex synaptosomal membranes discloses that Na+, K+-ATPase activity is inhibited by neurotensin, an effect blocked by NTS1 antagonist SR 48692 [8]. The activity of K+-p-NPPase is likewise inhibited by neurotensin but only under strict experimental conditions [9]. On assaying ATPase activity with ATP as substrate -which reflects the whole enzyme cycle- neurotensin effect remains unaltered after administration of levocabastine, antagonist for NTS2 receptor [10]. This study was extended herein to explore potential effect of neurotensin on enzyme K+ site. For this purpose, levocabastine was administered to rats and K+-p-NPPase activity in synaptosomal membranes and [3H]-ouabain binding to cerebral cortex membranes were assayed in the absence (basal) and in the presence of neurotensin. Results obtained indicate that NTS2 receptor blockade modifies the properties of neuronal Na+, K+-ATPase and that neurotensin effect on Na+, K+-ATPase involves NTS1 receptor and -at least partially- NTS2 receptor.
Materials and Methods
Animals and Drugs
Adult male Wistar rats weighing 100–150 g were used. All studies described were conducted in accordance with the Guide for Care and Use of Laboratory Animals provided by the National Institutes of Health, USA, and the Committee on Animal Experimentation (CICUAL), Facultad de Medicina, Universidad de Buenos Aires. The reagents were analytical grade. Ouabain, disodium ATP (grade I, prepared by phosphorylation of adenosine), disodium p-NPP and neurotensin acetate were from Sigma Chemical Co., St. Louis, MO, USA. Peptide solutions were freshly prepared in redistilled water for each experiment. BCS Biodegradable counting scintillant was from Amersham Biosciences, UK, and [3H]-ouabain (specific radioactivity 0.932 TBq/mmol) was from New England Nuclear, Du Pont, Boston, MA, USA. Levocabastine chlorhydrate was kindly provided by Janssen-Cilag Farmacéutica, S.A., Argentina.
Administration of Levocabastine
Groups of six rats were employed in each experiment. Half of the animals received the drug i.p. and the other half received the vehicle. Levocabastine chlorhydrate dissolved in saline solution was administered at a dose of 50 μg/kg [11]. Thirty minutes later, animals were killed by decapitation, skulls opened and cerebral cortices harvested.
Preparation of Synaptosomal Membrane Fractions
Synaptosomal membranes from cerebral cortex were isolated by differential and sucrose gradient centrifugation as previously described [7]. In each experiment, tissues were homogenized at 10 % (w/v) in cold 0.32 M sucrose (neutralized to pH 7.0 with 0.2 M Tris base) using a Teflon glass homogenizer of the Potter–Elvehjem type. The homogenate was subjected to differential centrifugation to separate nuclear and mitochondrial fractions. The crude mitochondrial pellet was resuspended in redistilled water (pH 7.0 with 0.2 M Tris base) for the osmotic shock. A pellet containing mitochondria, synaptosomal membranes and myelin was separated by centrifugation at 20,000 g for 30 min, then resuspended in 0.32 M sucrose, layered on top of a gradient containing 0.8; 0.9; 1.0, and 1.2 M sucrose and centrifuged at 50,000 g for two hours in a SW 28 rotor of an L8 Beckman ultracentrifuge. The fraction at the level of 1.0 M sucrose was collected, diluted with 0.16 M sucrose and spun down at 100,000 g for 30 min to obtain the synaptosomal membrane fraction. Pellets were stored at −70 °C and, prior to enzyme assay, resuspended by brief homogenization in redistilled water and used for three weeks without appreciable change in enzyme activities. The whole procedure was replicated at least four times.
Enzyme Assays
K+-p-nitrophenylphosphatase (K+-p-NPPase) activity was measured as described by Albers et al. [12]. Mg2+, K+-p-NPPase activity was assayed in a medium containing 10 mM MgCl2, 5 mM KCl, 0.20 M Tris-HC1 buffer (pH 7.4) and 10 mM disodium p-NPP. Mg2+-p-NPPase activity was determined in a similar medium without K+ addition and containing 1 mM ouabain. The difference between activities was taken to correspond to K+-p-NPPase. Before performing p-NPPase assay, synaptosomal membrane samples were preincubated with 0.16 M Tris-HC1 buffer (pH 7.4) or with 3.5 × 10−6M neurotensin solution at 37 °C for 10 min. Aliquots of preincubated fractions (10 μl) were distributed in two series of microtubes containing the respective medium (40 μl) for the assay of total- and Mg2+-p-NPPase activities, and incubated at 37 °C for 30 min. The reaction was stopped with 0.1 N NaOH and released p-nitrophenol (p-NP) determined spectrophotometrically at 420 nm. In all enzyme assays, tubes containing enzyme preparations and assay media maintained at 0º C throughout the incubation period were processed to serve as blanks. When indicated, the incubation media contained 0.6 mM ATP plus 45 mM NaC1. Results were expressed as p-NP released per mg protein per hour.
ATPase activity was measured as already described [12]. Total ATPase activity was assayed in a medium containing 100 mM NaCl, 20 mM KCl, 3 mM MgCl2, 0.20 M Tris–HCl buffer (pH 7.4) and 4 mM ATP. Mg2+-ATPase activity was determined in a similar medium with no added Na+ and K+, and containing 1 mM ouabain. The difference between the activities was taken to correspond to Na+, K+-ATPase. Before performing ATPase assay, samples of synaptosomal membrane fractions were preincubated with 0.20 M Tris–HCl buffer (pH 7.4) or 3.5 × 10−6 M neurotensin solution at 37 °C for 10 min; incubation volume (μl) was 35:5 for buffer and membranes. Aliquots of preincubated fractions (3 μl) were distributed in two series of microtubes containing the respective medium (40 μl) for the assay of total- and Mg2+-ATPase activities, and incubated at 37 °C for 30 min. The reaction was stopped with 30 % (w/v) trichloroacetic acid solution. ATPase activity was monitored by colorimetric determination of orthophosphate released [13]. In all enzyme assays, tubes containing enzyme preparations and assay media maintained at 0 °C throughout the incubation period were used as blanks. Data for ATPase activities were expressed as μmol released inorganic phosphate per mg protein per hour.
Isolation of Crude Cerebral Cortex Membranes for [3H]-Ouabain Binding
Membrane fractions from cerebral cortex were isolated by differential centrifugation. In each experiment, tissues were homogenized at 10 % (w/v) in cold 0.32 M sucrose (neutralized to pH 7.0 with 0.2 M Tris base) using a Teflon glass homogenizer of the Potter–Elvehjem type. The homogenate was centrifuged at 900 g for 10 min; supernatants were spun down at 100,000 g for 30 min in a L8-Beckman ultracentrifuge. Resultant pellets were resuspended in 0.16 M sucrose, and distributed in 12 tubes for further spinning as above. After discarding the supernatants, pellets were stored at −70 °C. Prior to use, pellets were resuspended in redistilled water to achieve a 5 mg protein per ml concentration and processed for [3H]-ouabain binding assay. The whole procedure was replicated at least 3 times.
[3H]-Ouabain Binding Assay
[3H]-ouabain binding was carried out in crude membrane preparations isolated from rat cerebral cortex by a filtration assay using [3H]-ouabain. Binding was performed in a medium (0.5 ml final volume) consisting of 3 mM MgCl2, 2 mM H3PO4, 0.25 mM sucrose, 0.25 mM EDTA, 30 mM imidazol-HCl buffer, pH 7.4 [14], 250 μg cerebral cortex membrane protein, 45 nM [3H]-ouabain and neurotensin solution to achieve 1.0 × 10−5M concentration. After incubation at 37 °C for 60 min, samples were filtered under vacuum on GF/B filters positioned in a Millipore multifilter and rinsed twice with 2 ml of ice-cold 30 mM imidazol-HCl buffer, pH 7.4. Filters were transferred to vials, 10 ml of counting scintillant were added and radioactivity was quantified in a Beckman Coulter-LS 6500 scintillation counter with 64 % efficiency. Specific binding was calculated by substracting binding found in the presence of 100 μM unlabelled ouabain. Non-specific binding accounted for less than 10 % of total membrane-bound radioactivity.
Protein Measurement
Protein content in synaptosomal membrane preparations was determined by the method of Lowry et a1. [15] using bovine serum albumin as standard.
Data Analyses
Data for enzyme assays were expressed as mean values ± SE from at least three independent experiments. The differences between two groups were analyzed by Student’s t test. The differences among more than two groups were analyzed by one-way ANOVA followed by Bonferroni’s multiple comparison test. Results for [3H]-ouabain binding were expressed as per cent binding taken as 100 % values recorded after administration of the vehicle; statistical analyses were performed using one-sample Student’s t-test. In all cases, the probability level indicative of statistical significance was set at P < 0.05.
Results
Synaptosomal membrane fractions were isolated after levocabastine administration to rats and p-NPPase activity was assayed in non-phosphorylating and phosphorylating media (plus ATP + NaCl), with or without neurotensin.
In the non-phosphorylating mediun, basal K+-p-NPPase activity in vehicle injected membranes was 11.3 ± 1.02 μmol p-NP released. mg prot−1 · h−1, a value which was not different in the presence of 3.5 × 10−6M neurotensin. Similar results were recorded after levocabastine administration, either with or without neurotensin (Fig. 1a). In these conditions, basal Mg2+-p-NPPase activity was roughly 1 μmol p-NP released. mg prot−1 · h−1 in vehicle injected membranes, a value which was not modified by the presence of 3.5 × 10−6M neurotensin. Administration of levocabastine enhanced basal Mg2+-p-NPPase activity (+118 %) but neurotensin presence failed to modify this enzyme activity (Fig. 1b).
Neurotensin effect on synaptosomal membrane p-NPPase activities in non-phosphorylating media after levocabastine administration. Rats received i.p. a single dose of levocabastine or vehicle and 30 min later, were decapitated, tissues harvested and subjected to subcellular fractionation to obtain synaptosomal membranes. The procedure for rat treatment and subcellular fractionation was replicated at least 4 times. Membrane samples were preincubated in non-phosphorylating media, with or without 3.5 × 10−6M neurotensin and assayed for p-NPPase activities. Results are expressed as specific enzyme activity. Data are mean values ± SE from 6 assays carried out by triplicate in different membrane fractions. White bars, without neurotensin; dotted bars, with neurotensin. (A) K+-p-NPPase activity; (B) Mg2+-p-NPPase activity. * P < 0.05 between bars, by one-way ANOVA followed by Bonferroni´s multiple comparison test. ns, non-significant difference
Basal K+-p-NPPase activity was lower in a medium supplemented with ATP + NaCl, in agreement with previous results [9]. The enzyme activity in vehicle injected membranes was roughly 2 μmol p-NP released. mg prot−1 · h−1, a value which decreased 32 % by the presence of 3.5 × 10−6M neurotensin. A trend to increase basal K+-p-NPPase activity was observed after levocabastine administration. This treatment prevented neurotensin effect on the enzyme (Fig. 2a). Basal Mg2+-p-NPPase activity was higher (+35 %) after levocabastine administration. In no case, neurotensin modified this enzyme activity (Fig. 2b).
Neurotensin effect on synaptosomal membrane p-NPPase activities in phosphorylating media after levocabastine administration. Rats received i.p. a single dose of levocabastine or vehicle and 30 min later, were decapitated, tissues harvested and subjected to subcellular fractionation to obtain synaptosomal membranes. The procedure for rat treatment and subcellular fractionation was replicated at least 4 times. Membrane samples were preincubated in phosphorylating media, with or without 3.5 × 10−6M neurotensin and assayed for p-NPPase activities. Results are expressed as specific enzyme activity. Data are mean values ± SE from 9 assays carried out by triplicate in different membrane fractions. White bars, without neurotensin; dotted bars, with neurotensin. (A) K+-p-NPPase activity; (B) Mg2+-p-NPPase activity. * P < 0.05 between bars, by one-way ANOVA followed by Bonferroni´s multiple comparison test. ns, non-significant difference
With confirmatory and comparative purposes of our previous study [10], in three of the assays carried out to determine phoshatase activity with p-NPP as substrate, additional new determinations for ATPase activity with ATP as substrate were performed at the same time in the same synaptosomal membrane samples (Table 1). Data recorded in samples from levocabastine or vehicle injected rats showed that either in the presence or absence of neurotensin, in no case differences for total Mg2+, K+-p-NPPase were observed in a non-phosphorylating medium. However, in a phosphorylating medium, neurotensin decreased Mg2+, K+-p-NPPase activity, an effect blocked by levocabastine administration. The assay of total Mg2+, Na+, K+-ATPase activity showed that levocabastine administration enhanced this enzyme activity and that neurotensin inhibited the enzyme in both levocabastine and vehicle treated samples (Table 1).
Basal values for high affinity [3H]-ouabain binding to cerebral cortex membranes ranged from 730 to 1250 fmol per mg protein (n = 6). After i.p. administration of levocabastine high affinity [3H]-ouabain binding was 53 % higher than in controls injected with the vehicle. The presence of 1.0 × 10−5M neurotensin decreased roughly 40 % and 60 % [3H]-ouabain binding to membranes obtained from control and levocabastine injected rats, respectively (Fig. 3).
High affinity [3H]-ouabain binding after administration of levocabastine. Rats received i.p. a single dose of levocabastine or vehicle and 30 min later, were decapitated, tissues harvested and subjected to subcellular fractionation to obtain synaptosomal membranes. Cerebral cortex membranes were obtained 30 min after i. p. administration of levocabastine and processed for high affinity [3H]-ouabain binding. The procedure for rat treatment and subcellular fractionation was replicated at least 3 times. Results are expressed as per cent binding taken as 100 % values recorded after administration of the vehicle. Data are mean values ± SE from 6 binding assay replications carried out by triplicate in different membrane fractions. * P < 0.05; ** P < 0.001, by one way ANOVA followed by Bonferroni´s multiple comparison test
Discussion
The administration of NTS2 receptor antagonist levocabastine allowed disclosing that different responses to neurotensin occur depending on the experimental approach carried out to evaluate Na+, K+-ATPase, that is, employing: (a) ATP as substrate; (b) p-NPP as substrate in a non-phosphorylating medium; (c) p-NPP as substrate in a phosphorylating medium or (d) on assaying high affinity [3H]-ouabain binding to cerebral cortex membranes.
The treatment with levocabastine enhanced total ATPase values [10 and present results] but produced no effect on total p-NPPase activity. Differences between Na+, K+-ATPase and K+-p-NPPase properties have been shown [16]. Basal K+-p-NPPase activity and neurotensin effect on the enzyme in synaptosomal membranes differed according to the assay conditions. In the presence of ATP + NaCl, basal K+-p-NPPase activity was remarkably lower and neurotensin inhibited this enzyme activity [10 and present results].
Levocabastine administration enhanced basal Mg2+-p-NPPase activity either in the absence or presence of ATP + NaCl. Such enzyme stimulation is hardly attributable to a single response because: (a) Mg2+ is necessary for normal enzyme reactivity; (b) Mg2+ required for ATPase phosphorylation normally remains tightly bound to the phosphoenzyme and (c) it is difficult to separate the effects of free Mg2+ from those of the complexes Mg-ATP and Mg-ADP [17, 18].
Na+, K+-ATPase K+ site is the binding site for specific and selective inhibitor ouabain [5] and the evaluation of [3H]-ouabain binding to crude brain membrane preparations is an additional approach for the study of this enzyme. Administration of levocabastine modified Na+, K+-ATPase K+ site because it enhanced basal high affinity [3H]-ouabain binding to cerebral cortex membranes. This ex vivo result differed from an in vitro finding because 1 × 10−5 M levocabastine added during the assay decreases 28 % [3H]-ouabain binding [19]. This apparent discrepancy may receive an explanation because in vitro potential signaling mechanisms are not operative. In fact, neurotensin, SR 48692 and levocabastine behave as agonists or antagonists at NTS2 receptor, depending on the specific signaling events and cell types under examination [20].
Evidence supports the notion that the ouabain and K+ binding sites are not the same. To illustrate, the presence of K+ is eight-fold more effective to diminish phospho-enzyme levels than [3H]-ouabain binding [21]. There is no close correlation between the binding of cardiac glycosides to K+ site of Na+, K+-ATPase and its inhibitory effect on the enzyme [22]. Na+, K+-ATPase mutants show remarkable differences between binding constants for ouabain versus their affinities for K+ [23]. Spectroscopic studies show that Na+, K+-ATPase with bound ouabain is deeply inserted into the transmembrane domain with the lactone ring very close to the bound K+ [24] and suggest that ouabain binds at two sites along the ion permeation pathway of the enzyme [25]. Besides, differences in ouabain binding, ATPase activity, and enzyme phosphorylation are observed in six ATP1A3 mutations associated with alternating hemiplegia [26].
Findings may be interpreted as an effect of levocabastine administration. The increase in enzyme activities was recorded ex vivo, indicating a permanent effect because it resisted the procedure for the isolation and purification of synaptosomal membranes (tissue homogenization, differential centrifugation, osmotic shock, etc.). Since the short time elapsing between drug injection and rat decapitation (30 min) after levocabastine administration, the observed effects may occur through an allosteric change of the protein. In support, it has been reported that Mg2+ is important in the conformational transitions of Na+, K+-ATPase [27].
Literature related to the employ of levocabastine nasal spray and eye drops for the treatment of allergic rhinoconjuntivitis is abundant [28–30]. At high doses levocabastine alters locomotor activity, induces sleep and inhibition of active avoidance response in rodents [31] whereas by topical administration it fails to alter psychomotor and cognitive function in humans [32]. So far there was no information regarding levocabastine effects at CNS level.
To sum up, present findings described novel effects of levocabastine at CNS and disclosed different responses to neurotensin according to the experimental approach carried out to evaluate Na+, K+-ATPase. The sodium pump may be a target for neurotensin effects at synaptic level involving the neurotensin NTS1 receptor and, at least partially, the neurotensin NTS2 receptor, indicating that a functional relationship between the neurotensinergic system and synaptic Na+, K+-ATPase activity may take place. It is suggested an action on Na+, K+-ATPase, and therefore, on energetic metabolism and central neurotransmission. It may be postulated that under levocabastine effect, by blocking NTS2 receptor, neurotensin will not be able to inhibit Na+, K+-ATPase. As a result, an improvement of synaptic energetic function may be expected. Lastly, these findings may be of interest for new pharmacological developments oriented to psychiatric diseases.
Abbreviations
- NTS1 receptor:
-
High affinity neurotensin receptor
- NTS2 receptor:
-
Low affinity neurotensin receptor
- p-NP:
-
p-Nitrophenol
- p-NPP:
-
p-Nitrophenylphosphate
- p-NPPase:
-
p-Nitrophenylphosphatase
References
Kitabgi P, Nemeroff CB (1992) The neurobiology of neurotensin. Ann N Y Acad Sci 668:1–374
Carraway R, Leeman SE (1973) The isolation of a new hypotensive peptide neurotensin from bovine hypothalami. J Biol Chem 248:6854–6861
Vincent JP, Mazella J, Kitabgi P (1999) Neurotensin and neurotensin receptors. Trends Pharmacol 2:302–309
Dobner PR (2005) Multitasking with neurotensin in the central nervous system. Cell Mol Life Sci 62:1946–1963
Albers RW, Siegel GJ (2012) Membrane transport. In: Brady ST, Siegel GJ, Albers RW, Price D (eds) Basic neurochemistry: principles of molecular, cellular and medical neurobiology, 8th edn. Elsevier Academic Press, Massachusetts, USA, pp 41–62
Rodríguez de Lores Arnaiz G (2007) Na+, K+-ATPase in the brain: structure and function. In: Reith MEA (ed) Neural Membranes and Transport. Lajtha A (ed) Handbook of Neurochemistry and Molecular Neurobiology Vol. 11, Springer-Verlag, Berlin-Heidelberg, pp 209–224
Rodríguez de Lores Arnaiz G, Alberici M, De Robertis E (1967) Ultrastructural and enzymic studies of cholinergic and non–cholinergic synaptic membranes isolated from brain cortex. J Neurochem 14:215–225
López Ordieres MG, Rodríguez de Lores Arnaiz G (2000) Neurotensin inhibits neuronal Na+, K+-ATPase activity through high affinity peptide receptor. Peptides 21:571–576
López Ordieres MG, Rodríguez de Lores Arnaiz G (2001) K+-p-nitrophenylphosphatase inhibition by neurotensin involves high affinity neurotensin receptor: influence of potassium concentration and enzyme phosphorylation. Regul Pept 101:183–187
Álvarez Juliá A, Gutnisky A, López Ordieres MG, Rodríguez de Lores Arnaiz G (2013) High affinity receptors but not low affinity receptors for neurotensin are involved in neuronal Na+, K+-ATPase inhibition by the peptide. Curr Top Pept Protein Res 14:27–32
Tyler BM, Groshan K, Cusack B, Richelson E (1998) In vivo studies with low doses of levocabastine and diphenhydramine, but not pyrilamine, antagonize neurotensin-mediated antinociception. Brain Res 787:78–84
Albers RW, Rodríguez de Lores Arnaiz G, De Robertis E (1965) Sodium-potassium-activated ATPase and potassium-activated p-nitrophenylphosphatase: a comparison of their subcellular localizations in rat brain. Proc Natl Acad Sci USA 53:557–564
Lowry OH, López JH (1946) Determination of inorganic phosphate in presence of labile P esters. J Biol Chem 162:421–428
Antonelli M, Casillas T, Rodríguez de Lores Arnaiz G (1991) Effect of Na+, K+-ATPase modifiers on high-affinity ouabain binding determined by quantitative autoradiography. J Neurosci Res 28:324–331
Lowry OH, Rosebrough NJ, Farr AL, Randall RJ (1951) Protein measurement with the Folin phenol reagent. J Biol Chem 193:265–275
Yoshida H, Nagai K, Ohashi T, Nakagawa Y (1969) K+-dependent phosphatase activity observed in the presence of both adenosine triphosphate and Na+. Biochim Biophys Acta 171:178–185
Fukushima Y, Post RL (1978) Binding of divalent cation to phosphoenzyme of sodium- and potassium-transport adenosine triposphatase. J Biol Chem 253:6853–6862
Swann AC, Albers RW (1978) Sodium and potassium ion-dependent adenosine triphosphatase of mammalian brain: interactions of magnesium ions with the phosphatase site. Biochim Biophys Acta 523:215–227
Rosin C, López Ordieres MG, Rodríguez de Lores Arnaiz G (2011) Neurotensin decreases high affinity [3H]-ouabain binding to cerebral cortex membranes. Regul Pept 172:35–40
Vita N, Oury-Donat F, Chalon P, Guillemot M, Kaghad M, Bachy A, Thurneyssen O, García S, Poinot-Chazel C, Casellas P, Keane P, Le Fur G, Maffrand JP, Soubrie P, Caput D, Ferrara P (1998) Neurotensin is an antagonist of the human neurotensin NT2 receptor expressed in Chinese hamster ovary cells. Eur J Pharmacol 360:265–272
Han CS, Tobin T, Akera T, Brody TM (1976) Effects of alkali metal cations on phospho-enzyme levels and [3H]ouabain binding to (Na+ + K+)-ATPase. Biochim Biophys Acta 429:993–1005
Songu-Mize E, Gunter JL, Caldwell RW (1989) Comparative ability of digoxin and an aminosugar cardiac glycoside to bind to and inhibit Na+, K+ -adenosine triphosphatase: effect of potassium. Biochem Pharmacol 38:3689–3695
Johnson CL, Schultheis PJ, Lingrel JB, Johnson CG, Wallick ET (1995) Comparison of the effects of potassium on ouabain binding to native and site-directed mutants of Na, K-ATPase. Arch Biochem Biophys 317:133–141
Ogawa H, Shinoda T, Cornelius F, Toyoshima C (2009) Crystal structure of the sodium-potassium pump (Na+, K+-ATPase) with bound potassium and ouabain. Proc Natl Acad Sci USA 106:13742–13747
Sandtner W, Egwolf B, Khalili-Araghi F, Sánchez-Rodríguez JE, Roux B, Bezanilla F, Holmgren M (2011) Ouabain binding site in a functioning Na+, K+-ATPase. J Biol Chem 286:38177–38183
Weigand KM, Messchaert M, Swarts HG, Russel FG, Koenderink JB (2014) Alternating Hemiplegia of childhood mutations have a differential effect on Na+, K+-ATPase activity and ouabain binding. Biochim Biophys Acta 1842:1010–1016
Hegyvary C, Jorgensen PL (1981) Conformational changes of renal sodium plus potassium iontransport adenosine triphosphatase labeled with fluorescein. J Biol Chem 256:6296–6303
Heykants J, Van Peer A, Van de Velde V, Snoeck E, Meuldermans W, Woestenborghs R (1995) The pharmacokinetic properties of topical levocabastine: a review. Clin Pharmacokinet 29:221–230
Wijk RG (1995) Treatment of allergic rhinoconjunctivitis: a review of the role of topical levocabastine. Mediators Inflamm 4:S31–S38
Sheikh A, Singh Panesar S, Dhami S, Salvilla S (2007) Seasonal allergic rhinitis in adolescents and adults. Clin Evid (Online) pii: 0509
Tasaka K, Kamei C, Tsujimoto S, Yoshida T, Aoki I (1990) Central effect of the potent long-acting H1-antihistamine levocabastine. Arzneimittelforschung 40:1295–1299
Rombaut N, Bhatti JZ, Curran S, Hindmarch I (1991) Effects of topical administration of levocabastine on psychomotor and cognitive function. Ann Allergy 67:75–79
Acknowledgments
G. R. de L. A. is chief investigator from the Consejo Nacional de Investigaciones Científicas y Técnicas (CONICET). The authors are indebted to CONICET and Universidad de Buenos Aires, Argentina, for financial support.
Author information
Authors and Affiliations
Corresponding author
Ethics declarations
Conflict of interest
The authors declare that they have no conflict of interest.
Rights and permissions
About this article
Cite this article
Gutnisky, A., López Ordieres, M.G. & Rodríguez de Lores Arnaiz, G. The Administration of Levocabastine, a NTS2 Receptor Antagonist, Modifies Na+, K+-ATPase Properties. Neurochem Res 41, 1274–1280 (2016). https://doi.org/10.1007/s11064-015-1823-7
Received:
Revised:
Accepted:
Published:
Issue Date:
DOI: https://doi.org/10.1007/s11064-015-1823-7