Abstract
Background
Chlorogenic acid (CGA) is one of the well-known polyphenol compounds possessing several important biological and therapeutic functions. In order to optimize a culture system to achieve complete development of follicles, we focused on the effects of CGA supplementation during in vitro culture (IVC) on follicular development, oxidative stress, antioxidant capacity, developmental gene expression, and functional potential in cultured mouse ovarian tissue.
Methods and results
The collected whole murine ovaries were randomly divided into four groups: (1) non-cultured group (control 1) with 7-day-old mouse ovaries, (2) non-cultured group (control 2) with 14-day-old mouse ovaries, (3) cultured group (experimental 1) with the culture plates containing only the basic culture medium, (4) cultured group (experimental 2) with the culture plates containing basic culture medium + CGA (50, 100 and 200 µmol/L CGA). Afterward, histological evaluation, biochemical analyses, the expression assessment of genes related to follicular development and apoptosis as well as the analysis of 17-β-estradiol were performed. The results showed that supplementation of ovarian tissue with the basic culture media using CGA (100 µmol/l) significantly increased the survival, developmental and functional potential of follicles in whole mouse ovarian tissues after 7 days of culture. Furthermore, CGA (100 µmol/L) attenuated oxidative damage and enhanced the concentration of antioxidant capacity along with developmental gene expression.
Conclusion
It seems that supplementation of ovarian tissue with culture media using CGA could optimize follicular growth and development in the culture system.
Similar content being viewed by others
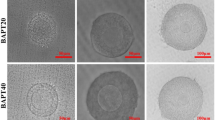
Avoid common mistakes on your manuscript.
Introduction
An in vitro culture (IVC) system for ovarian tissue is an interesting alternative approach to improve reproductive potential and preserve female fertility [1]. Many researchers have also been committed to defining the optimal maturation condition (physical and biochemical conditions) for the IVC system for ovarian tissues [2,3,4,5]. Despite the proposed designation of IVC systems, the improvement of this type of culture system is challenging because the survival rate of follicles, maturation rate of oocytes, and embryo development are still low compared with in vivo [6, 7].
Several studies have clarified that the reasons for these negative effects of in vitro culture on follicular development may be related to multiple exogenous factors, such as culture medium, oxygen tension, visible light, and handling of specimens [8]. It is believed that the IVC system for ovarian follicles contains a higher oxygen concentration than that of in vivo habitats inside the lumen of the female reproductive tract [9]. The high concentration of oxygen is predominantly associated with IVC conditions that result in the production of oxidative stress (OS) which reportedly poses a threat and damage to biological molecules and leads to poor quality, dysfunction, and cell death in in vitro matured oocytes [10].
Many researchers have also been committed to designing alternative therapeutic strategies by supplementing some biological agents, such as growth factors [11], antioxidants [12], nutrients [13], and ROS scavengers [14], with culture media to reduce oxidative stress and improve IVC conditions. Many reports suggest that antioxidant supplementation of IVC media improves oocyte quality by decreasing ROS levels and apoptotic factors [15,16,17,18].
Chlorogenic acid (CGA) (Fig. 1), the most available caffeoylquinic acid, is one of the well-known polyphenol compounds [19]. CGA has several significant biological and therapeutic functions, including antioxidant, antibacterial, hepatoprotective, cardioprotective, anti-inflammatory, antipyretic, neuroprotective, anti-obesity, antiviral, anti-microbial, and anti-hypertension functions [20]. Some studies have also confirmed the antioxidant potential of CGA. Besides, it has been reported that CGA can scavenge free radicals [18, 21]. Moreover, it has been demonstrated that supplementation of human hepatocarcinoma cell line using CGA could limit oxidative stress-related apoptosis by lowering ROS production occurring via increasing the storage of the intracellular glutathione (GSH) [22].
To the best of our knowledge, there is no information on the impact of CGA on cultured ovarian tissue. On the other hand, it is crucial to improve the developmental competence of ovarian follicles because its inefficiency diminishes its applicability in later stages [23, 24]. Therefore, to optimize a culture system and achieve a complete development of follicles and maturity of oocytes, we focused on the effects of CGA on follicular development, oxidative stress, antioxidant capacity, and developmental gene expression in mouse cultured ovarian tissue. Additionally, this study evaluates the effect of CGA on the levels of estradiol hormone (as an indicator of ovarian function) in a culture medium to determine the adequacy or functional potential of the ovarian tissue.
Materials and methods
Animals and ovarian tissue
In this original study, ovarian tissues were obtained from 7 to 14-day-old female mice. The animals were bred in standard cages and kept in laboratory conditions (12:12 light/dark cycle, ambient temperature (22 ± 2 °C), and controlled humidity (40–50%).
Experimental design
The collected mice ovaries were randomly divided into four groups: (1) non-cultured group (control 1) with 7-day-old mice ovaries, (2) non-cultured group (control 2) with 14-day-old mice ovaries, (3) cultured group (experimental 1) with culture plates containing only the basic culture medium + 7-day-old mice ovaries, (4) cultured group (experimental 2) with culture plates containing basic culture medium + CGA (50, 100 and 200 µmol/L) + 7-day-old mice ovaries.
In vitro culture of ovarian tissues
The animals (n = 12/each group) were sacrificed by cervical dislocation, and their ovaries were isolated and dissected (under sterile conditions) mechanically free of fat and mesentery. For tissue culture, whole ovaries in both experimental groups were cultured individually on commercial cell culture inserts (Millicell® CM, 0.4 μM pore size, Millipore Corp, Billerica, MA, USA) and placed in 24-well plates containing the basic culture medium. The culture medium consisted of 400 μl of α-MEM (Gibco, UK) supplemented with 10% FBS, 100 IU/ml penicillin, 100 µg/ml streptomycin, 0.23 mmol sodium pyruvate, and 0.22 gr/ml sodium bicarbonate (Abedpour, Salehnia, et al. 2018). All of the culture systems were incubated at 37 °C in 5% CO2 for seven days.
Administration of chlorogenic acid
In cultured groups (experimental 2), CGA (Sigma-Aldrich, St. Louis, MO, USA) powder was dissolved in ethanol solution (Sigma-Aldrich, St. Louis, MO, USA) and was directly (after filtering) added into the culture medium (Nguyen, Wittayarat et al. 2018). The optimal dose of CGA was chosen pending our different evaluations.
Histological evaluation
For morphological and histological examinations of all groups, ovaries were fixed in Bouin’s solution and then sent to a pathological laboratory for providing sections and tissue staining (hematoxylin–eosin or H&E). By means of a light microscope, histological evaluation of the follicular stage was determined based on the number of layers and morphology of granulosa cells surrounding the oocytes, using the follicular classification method as described previously [25].
Ovarian area
The ovarian morphology was assessed using an inverted microscope every 2 days during the culture period on days 3, 5, and 7 in all groups (n = 5/subgroup). The area of ovarian tissues was calculated by images of each ovary captured with an inverted microscope secured with a digital 10 MP camera (D-Moticam 10+). The obtained images were analyzed by the ImageJ software (National Institutes of Health, Bethesda).
Evaluation of the ovarian follicular viability using Trypan blue staining
After 1-week culture, the preantral follicles (140–150 μm in diameter) were mechanically isolated by insulin-gauge needles under a stereo microscope. The preantral follicles with 2–3 layers of granulosa cells, healthy and visible oocytes, and a thin layer of theca cells were chosen for three-dimensional culture. The survival rates of pre-antral follicles isolated from cultured ovaries (n = 30/each group) were determined using Trypan blue staining (0.4%) (Sigma, St. Louis, MO). Follicles were labeled as degenerated or viable in a way that degenerated follicles were stained in blue color, damaged follicles were partly stained in blue color, and viable follicles remained colorless [26].
Morphological analysis of apoptosis
In order to quantify apoptosis using morphological criteria, isolated pre-antral follicles (n = 30/each group in 3 repeats) were stained with both Acridine Orange (AO) and Ethidium Bromide (EB) (Sigma-Aldrich, USA). Follicles were observable as viable (green), early apoptotic (bright orange), and late apoptotic (red) under a fluorescent microscope [27].
Hormonal assay
The concentration of 17 β-estradiol (E2) in the collected media obtained from all groups was measured during the culture period on days 3 and 7 using an ELISA Kit according to the manufacturer's instruction. The analytical sensitivity of the assay was expressed to be pg/mL for E2 (MyBioSource, San Diego, California, USA).
Assays for oxidative stress markers
Biochemical assays were carried out to evaluate oxidative stress markers, including malondialdehyde (MDA), glutathione (GSH), and total antioxidant capacity (TAC) in all groups using commercial ELISA Kits according to the manufacturer’s instruction (ZellBio GmbH, Biotechnology company in Lonsee, Germany) [28].
Real-time RT-qPCR
The gene expression analysis of apoptotic genes (Bax and Bcl-2), bone morphogenetic protein (BMP15), and growth differentiation factor (GDF9) was performed by RT-qPCR in ovarian tissues using the Light Cycler 480 with SYBR Green detection and Amplicon Kit (Applied Biosystems). In order to design specific PCR primer sequences, we used an online software package Gen Bank (http://www.ncbi.nlm.nih.gov) website, and the obtained sequences were evaluated using the IDT Primer Quest tool–Oligo analyzer (http://scitools.idtdna.com/analyzer/Applications/OligoAnalyzer). The sequences for forward and reverse primers of Bax, Bcl-2, BMP15, and GDF9 are presented in Supplementary Table1. The expression levels of the genes were normalized against the β-actin (as a housekeeping gene) gene. The relative expression levels were calculated by the 2−ΔΔCT method [28]. All experiments were conducted three times.
Statistical analysis
Data were analyzed using Statistical Package for the Social Sciences (SPSS) program version 16 (SPSS Inc. Chicago, IL, USA) software. The Kolmogorov–Smirnov test was applied to examine whether the data were normally distributed. One-way analysis of variance (ANOVA) followed by Turkey’s post hoc test (for homogeneous variances) was performed for multiple comparisons. The results with normally distributed values were expressed as the means and standard deviation (mean ± SD). The statistical significance was defined when the p-value was less than 0.05 at a 95% confidence level.
Results
Phase-contrast microscopy of whole cultured ovaries
The morphological observations of the ovarian tissues before and after the in vitro culture process in the presence and absence of CGA are presented in Fig. 2A–C. According to phase-contrast microscopy, our morphological results demonstrated that the anterior surfaces of ovaries were swollen, and the follicles exhibited outgrowth in the cortical parts of tissues. These changes were further pronounced in CGA-treated tissues. In addition, the central parts of all cultured ovaries were darker than the marginal parts. This dark area has further visibility in tissues lacking CGA (Fig. 2C).
Phase-contrast microscopy and H&E staining. The first row shows the phase-contrast microscopy of mouse ovaries that were viewed under an inverted microscope on days 0, 3 (A, B), and 7 (C1–C4) of the culture. The second and third rows (D–L) show H&E staining of ovaries with two different magnifications. Indeed, Control group 1 (D, G), Control group 2 (E, H), Experimental group 1 (0 µmol CGA, F1 and I), Experimental group 2 (50 µmol CGA, F2 and J), Experimental group 2 (100 µmol CGA, F3 and K), Experimental group 2 (200 µmol CGA, F4 and L). M: The mean area of mouse cultured ovaries on days 0, 3, 5, and 7 of the culture period. *Indicates a significant difference with days 0 and 3 (p < 0.05). **Indicates a significant difference with day 5 (p < 0.05). aIndicates a significant difference with other groups on the same day (p < 0.05)
Light microscopy
Figure 2 also shows the histological morphology of ovarian tissues in all groups using H&E staining in low (D-F4) and high (G-L) magnifications. Before starting in vitro analysis, the primordial follicles were mainly seen and located in the cortical parts of the ovaries of the control group 1. After 7 days of culture, in the cortical parts of the cultured ovaries, primordial follicles represented a smaller percentage of the total follicle pool, similar to the follicle distribution observed in ovaries isolated from 14-day-old mice. In addition, we detected (in the cortical parts of the cultured ovaries) primary and pre-antral follicles with normal morphology containing oocytes with germinal vesicle and granulosa cells. Close adhesion was visible between the oocyte and granulosa cells in follicles at different developmental stages. Healthy follicles with the normal structure were evident in all examined groups, especially in tissues treated with 100 µmol/l CGA. Moreover, degenerated follicles with pyknotic nuclei of oocytes and disarranged granulosa cells were detected in the central parts of the cultured ovaries. Damaged follicles had further visibility in tissues lacking CGA.
The surface area of ovaries
The results of the analysis of ovarian surface area (using ImageJ software) during the culture period on days 3, 5, and 7 are displayed in Fig. 2 (M). Our findings demonstrated a significant increase in the surface area of ovaries in all groups during the culture period compared to the first day of culture (p < 0.05). The mean surface area of ovaries was significantly increased in the CGA-treated groups in comparison with groups without receiving CGA on days 5 and 7 of culture. The surface area was significantly (p < 0.05) higher in tissues treated with 100 µmol/ CGA than in tissues in other groups.
Follicular count
The overall quantitative results of the percentages of normal primordial, primary, pre-antral, and atretic follicles in all groups of the study are compared and summarized in Table 1.
Before in vitro culture, the proportion of primordial follicles (located mainly in the peripheral parts of ovaries) was higher than follicles with other stages; however, after 7 days of culture, the ovarian tissue and follicles were grown; so the proportion of primordial was decreased, whereas and the proportion of primary, pre-antral, and degenerated follicles was increased (p < 0.05).
At the end of the culture period, the percentage of primordial follicles was significantly decreased in all treated groups compared to the control group 1 (p < 0.05). A normal percentage of primordial follicles was observed in the control group 2 and the experimental group 2 that received 100 µmol CGA. Moreover, the number of primary follicles did not show any significant difference between all experimental groups (0–200 µmol CGA) and the control group 2 (p > 0.05). Based on our results, the percentage of pre-antral follicles was gradually increased in a concentration-dependent manner until the concentration of CGA was increased up to 100 µmol; however, such percentage was reduced at a concentration of 200 µmol (Table 1). In addition, there was a significant decrease in the percentage of pre-antral follicles in cultured groups (i.e., 0, 50, and 200 µmol CGA) in comparison with in vivo sample (control 2).
Moreover, the lowest proportion of atretic follicles was observed in the control group 1 compared to all groups of the study (p < 0.05), while among experimental groups (0–200 µmol CGA), only the experimental group 2 treated with 100 µmol CGA showed the lowest rate of atretic follicles compared to the other experimental groups (p < 0.05).
Totally, no significant difference was detected between experimental group 2 received 100 µmol CGA, and in vivo sample (control 2) in terms of the number of primordial, pre-antral, and atretic follicles (p > 0.05).
The viability of isolated follicles
The morphology of pre-antral follicles after Trypan blue staining is shown in Fig. 3A–C. In the presence of different concentrations of CGA (Experimental group 2), especially in tissues treated with 100 µmol/l of CGA, the survival rate of pre-antral follicles was significantly increased in comparison with other groups.
Using TB staining: A white (unstained) follicles were classified as live (TB−), B pale blue stained follicles were considered damaged, and C dead follicles appear dark blue (TB+). Figure D showed the percentage of survival, damaged, and dead follicles in culture groups at different concentrations of CGA. *Significant difference with other groups (p < 0.05)
Morphological analysis of apoptosis
The representative micrographs of intact (green), late apoptotic (red), and early apoptotic (orange) follicles are depicted in Fig. 4A–C. The percentage of late apoptotic follicles was significantly increased in the experimental 1 group; however, the percentage of late apoptotic follicles was decreased in experimental group 2, especially in tissues treated with 100 µmol of CGA.
Acridine orange and ethidium bromide (AO/EB)-stained ovarian follicles: A intact cells (green), B early apoptotic (bright orange), and C late apoptotic (red) under a fluorescent microscope. Data in figure D are expressed as the total number of intact and apoptotic cells (early + late) as a percentage of the total. *Significant difference with other groups (p < 0.05)
Hormonal assay
The concentrations of E2 hormone obtained from collected media on days 3 and 7 of the culture period in cultured groups were compared (Table 2). The results demonstrated a significant difference in the concentration of E2 hormone on day 7 of culture compared to day 3 (p < 0.05). In addition, the concentration of E2 hormone on day 7 of culture was significantly different in some experimental groups (100 and 200 µmol CGA) in comparison with the experimental group 1 and the experimental group 2 that received 50 µmol CGA. This rate was gradually increased in a concentration-dependent manner until the concentration of CGA was increased up to 100 µmol/L; however, this rate was reduced in response to a concentration of 200 µmol/L (Table 2).
Biochemical assessment
Table 3 represents the biochemical results (MDA, GSH and TAC) obtained from ovarian tissue. Accordingly, the MDA level was significantly higher in all groups of the study compared to the control group1 (p ˂ 0.05). However, in the group treated with 100 µmol CGA compared to the control group 2, no significant difference was observed (p > 0.05). For the MDA level, the highest dose (200 µmol CGA) did not show any remarkable difference when compared to the dose of 100 µmol CGA, while there was a significant difference when compared to the groups treated with 0 and 50 µmol CGA (p ˂ 0.05).
For two other parameters, GSH and TAC, no significant difference was observed between both control groups (p > 0.05). All experimental groups showed a significant difference compared to the control group 1 (p ˂ 0.05). However, in comparison with the control group 2, the group treated with 100 µmol CGA showed an acceptable level of antioxidants (p > 0.05).
RT-qPCR analysis
The expression ratio of apoptotic-related genes (i.e., Bax and Bcl-2) and developmental genes (GDF-9 and BMP1-5) in comparison to the β-actin gene in all studied groups are demonstrated and compared in Table 4. As displayed in Table 4, the expression ratio of the Bax to the β-actin gene was significantly increased in all experimental groups and the control group 2 compared with the control group 1 (p < 0.05). However, in some experimental groups, 0, 50, and 200 µmol CGA, there was a significant difference when the mRNA expression of Bax compared with the control group 2 (p < 0.05). For the Bax parameter, in the group treated with 100 µmol CGA, no significant difference compared with the control group 2 was observed, also there was no significant difference between the two experimental groups that received 100 and 200 µmol CGA, respectively (p > 0.05).
The expression ratio of the Bcl-2 to the β-actin gene was significantly decreased in all experimental groups (0–200 µmol CGA) compared with the control group 1 (p < 0.05). In some experimental groups, 0, 50, and 200 µmol CGA, there was a significant difference when the mRNA expression of Bcl-2 compared with the control group 2 (p < 0.05). However, for the Bcl-2 parameter, in the group treated with 100 µmol CGA, no significant difference compared with the control group 2 was observed (p < 0.05).
The Bax/Bcl-2 ratio showed that there was a significant difference between the control group 1 and all other groups (p < 0.05). Compared to the control 2, this ratio remarkably decreased when culture media was supplemented with 100 µmol CGA (p < 0.05).
Interestingly, the expression of both developmental genes, GDF-9 and BMP-15, was remarkably lower in the groups that received 0 and 50 µmol CGA than in both control groups (p < 0.05). In the experimental group 2 treated with 100 µmol CGA, the expression levels of these two genes were markedly increased compared to groups treated with 0 and 50 µmol CGA (p < 0.05). On the other hand, in the group treated with 200 µmol CGA, the expression levels of GDF-9 and BMP-15 were increased compared with the group that received 0 µmol CGA, and also they were decreased compared with the control group 2 and the group treated with 100 µmol CGA (p < 0.05). Totally, no significant difference was detected between the experimental group 2 that received 100 µmol CGA and the control groups in terms of all parameters mentioned earlier (GDF-9 and BMP-15).
Discussion
The present study was the first report that revealed the supplementation of the culture media for ovarian tissue with CGA could improve in vitro growth and development of mouse ovarian follicles by reducing oxidative stress and enhancing antioxidant capacity.
In the current study, we first attempted to determine morphological changes in all experimental groups. The morphology of tissue and the number of ovarian follicles were evaluated under light microscope field by field. The normal ovarian follicles were determined and classified as follows: (i) the primordial follicles were contained the immature oocyte with a single layer of flattened granulosa cells; (ii) the primary follicles with a single layer of cuboidal granulosa cells; (iii) the preantral follicles were surrounded by two or more layers of cuboidal granulosa cells. The morphological findings (using H&E, AO/EB, and TB staining techniques) demonstrated that the administration of CGA could significantly improve the survival rate of follicles, the proliferation of granulosa cells (GCs), and the growth of primordial and primary follicles to the pre-antral follicle stage in parallel with an increase in the mean surface area of ovarian tissue. The phase-contrast microscopy exhibited that follicles had a higher tendency to grow on the periphery of ovarian tissues, and they had a large and clear antral cavity. The growth of follicles was parallel to an increase in ovarian size during the culture period. However, in the central parts of the ovaries, the density of the tissue was higher, and this part of the tissue was dark. It showed that the central part of cultured tissue suffered from hypoxia and there were signs of follicular degeneration and cell fragmentation in this part. These changes were prominent especially in the absence of CGA. The light microscopy showed the granulosa cells of follicles were well-arranged around the oocyte. The damaged follicles (pyknotic nuclei oocytes and disarranged granulosa cells) were prominent, especially in the central areas of ovaries cultured in the absence of CGA (experimental 1: 0 µmol/l of CGA). It seems that the effects of CGA are dose-dependent since all mentioned parameters were gradually improved during in vitro culture up to 100 µmol/L concentration of CGA. However, although the concentration of 200 µmol/l CGA could significantly change all mentioned parameters, a concentration of 100 µmol/L CGA showed a better function than 200 µmol/l CGA.
Nguyen et al. indicated the supplementation of the culture media for porcine oocytes with 50 µmol/l CGA could significantly enhance the maturation, fertilization, and developmental competence of oocytes by preventing apoptosis. They concluded that the application of CGA during in vitro maturation (IVM) of oocytes has the potential to improve in vitro production (IVP) of porcine oocytes [21].
Moreover, in the present study, we employed several complementary techniques in addition to morphological staining. The result of hormonal analysis confirmed morphological findings and showed that the concentration of E2 was significantly enhanced in the group treated with 100 µmol/l CGA. This finding is in agreement with the results of Zhou et al., who postulated that CGA exerted estrogenic activity and prevented estrogen deficiency-induced osteoporosis in ovariectomized rats [29]. In addition, Wang et al. indicated that CGA exhibited an estrogen-stimulating effect on ovarian granulosa cells. They concluded that CGA might have therapeutic potential in the treatment of postmenopausal syndrome [30]. It appears that CGA might stimulate granulosa cells to express cytochrome P450 aromatase genes that convert androgens to E2 and promote ovarian follicular development.
Moreover, our biochemical results were in accordance with those obtained by Ali et al., who confirmed that CGA could significantly increase the activity of anti-oxidant capacity, such as SOD (scavenger of the superoxide anion to form H2O2), CAT (converting H2O2 into H2O), and the GSH content [31]. The antioxidant effects of CGA have also been addressed in the literature [31,32,33]; however, CGA has been administrated in different tissues and cells. For example, Koriem et al. confirmed that caftaric acid (an ester form of caffeic acid) enhanced GSH concentrations while it reduced MDA and catalase concentrations in the brain, liver, and kidney [34]. In the present study, we evaluated these criteria in the ovarian tissue for the first time. Several lines of evidence showed that an increase in free radicals following oxidative stress could cause lipid peroxidation, which in turn leads to an increase in MDA levels during the culture period. This phenomenon has negative effects on follicular development, percentage of mature oocytes with the first polar body, percentage of large follicles, ovulated oocytes, and fertilization rate [8,9,10]. The antioxidant agents used in this and other studies have extensive properties in biological systems that have already been shown to help improve the IVC system for oocytes by increasing intracellular GSH or decreasing ROS levels.
In most tissues, GSH act as an electron donor for some antioxidant enzymes, maintaining the cells in a reduced state. In the ovary, the strongest GR (Glutathione Reductase) activity (catalyzes the conversion of GSSG (oxidized glutathione) into GSH with NADPH consumption) is found in oocytes [15]. Several studies suggest that GSH plays an important role during the culture period of ovarian follicles, including cell proliferation, differentiation, developmental competence of oocytes, improvement of fertilization, and apoptosis [14, 35,36,37,38]. In-vitro studies have shown that the anti-apoptotic effect of FSH on granulosa cell apoptosis can be markedly inhibited by blocking the synthesis of GSH in cultured follicles [39]. A number of studies indicated that the measurement of the GSH concentration in oocytes after in-vitro maturation might be a valuable indicator for monitoring the viability and quality of oocytes [40,41,42]. In addition, multiple studies have clarified that the level of TAC in granulosa and theca cells is associated with an increase in ovulation and oocyte maturation [43, 44, 45]. These antioxidant effects are also considered to be related to their ability to stimulate the antioxidant defense system through the redox-regulated transcription factors and mitogen-activated protein kinases (MAPK) signaling pathway [46]. Therefore, the supplementation of IVC medium with antioxidants may be a prerequisite for folliculogenesis and oocyte quality.
These observations can be related to the antioxidant effect of CGA and have important physiological and biological effects, namely antioxidant and anti-inflammatory. It has been shown that hydroxyl groups (OH) on phenolic acids act as positive substitutions because of their antioxidant properties, and the number of hydroxyl groups seems to be associated with their contribution to this antioxidant activity [19, 20].
Finally, our molecular findings supported both histological and biochemical results. Otherwise stated, molecular data amplified the result of developmental ability and functional potential of CGA during IVC of ovarian tissue. Our molecular findings corroborated the results of Dkhil et al., who showed the protective effect of CGA against the injuries induced by sodium arsenite (NaAsO2) in the liver of mice. They demonstrated that, in the presence of CGA, the mRNA levels of pro-apoptotic markers (Bax and Caspase-3) were significantly decreased, but the mRNA level of Bcl-2, an anti-apoptotic marker, was significantly increased [32]. On the other hand, our results showed that CGA up-regulated the expression levels of GDF-9 and BMP-15 (p < 0.05).
GDF-9 and BMP-15 direct the follicle symphony and play important roles in folliculogenesis and oocyte quality [47,48,49]. Overall, GDF-9 has been implicated in follicular development (the progression of the primary follicle to the secondary follicle stage) in parallel with an increase in GC differentiation [50]. BMP-15 is also another significant factor involved in the promotion of follicle maturation, gonadotropin-independent phase of primordial follicles, regulation of GC sensitivity to follicle-stimulating hormone (FSH), the prevention of GC apoptosis, and regulation of the ovulation quota [51,52,53].
To date, there is no information on the effect of CGA on the expression levels of developmental genes. This study is the first report that revealed the promoting effects of CGA on the expression level of GDF-9 and BMP-15 genes. There is a limited number of resources that analyze the molecular mechanisms of CGA in culture conditions. It seems that the supplementation of the IVC medium with CGA regulates these molecular changes by the phosphatidylinositol-3-kinase (PI3K/Akt) and mitogen-activated protein kinase (MAPK) signaling pathways. Cheng et al. demonstrated that CGA has a cytoprotective effect against Aluminum, which may cause damage to macrophages, possibly through PI3K/Akt and MAPK signaling pathways in RAW264.7 cells [46].
In conclusion, the present study confirmed that the supplementation of ovarian tissue culture media with CGA, especially at a concentration of 100 µmol/l, could optimize the culture system and improve the survival, developmental, and functional potential of follicles in whole mouse ovarian tissues after 7 days of culture. Furthermore, the result of this study (for the first time) showed that CGA could suppress oxidative damage and improve the culture system by enhancing the concentration of antioxidants and developmental gene expression. However, before the clinical application of CGA, further experiments are warranted to elucidate the precise mechanism at the molecular levels.
Change history
29 April 2023
A Correction to this paper has been published: https://doi.org/10.1007/s11033-023-08348-x
References
Filatov MA, Khramova YV, Kiseleva MV, Malinova IV, Komarova EV, Semenova ML (2016) Female fertility preservation strategies: cryopreservation and ovarian tissue in vitro culture, current state of the art and future perspectives. Zygote 24(5):635–653. https://doi.org/10.1017/S096719941600006
Higuchi CM, Maeda Y, Horiuchi T, Yamazaki T (2015) A simplified method for three-dimensional (3-D) ovarian tissue culture yielding oocytes competent to produce full-term offspring in mice. PLoS ONE. https://doi.org/10.1371/0143114
Abedpour N, Salehnia M, Ghorbanmehr N (2018) The effects of lysophosphatidic acid on the incidence of cell death in cultured vitrified and non-vitrified mouse ovarian tissue: separation of necrosis and apoptosis border. Cell J 20(3):403–411
Abedpour N, Salehnia M, Ghorbanmehr N (2018) Effect of lysophosphatidic acid on the follicular development and the expression of lysophosphatidic acid receptor genes during in vitro culture of mouse ovary. Vet Res Forum 9(1):59–66
Abdi S, Salehnia M, Hosseinkhani S (2015) Kit ligand decreases the incidence of apoptosis in cultured vitrified whole mouse ovaries. Reprod Biomed Online 30(5):493–503. https://doi.org/10.1016/j.rbmo.2015.01.009
Anderson RA, Telfer EE (2018) Being a good egg in the 21st century. Br Med Bull 127(1):83–89. https://doi.org/10.1093/bmb/ldy023
Santana-Gálvez J, Cisneros-Zevallos L, Jacobo-Velázquez DA (2017) Chlorogenic acid: recent advances on its dual role as a food additive and a nutraceutical against metabolic syndrome. Molecules (Basel, Switzerland). https://doi.org/10.3390/molecules22030358
Lu J, Wang Z, Cao J, Chen Y, Dong Y (2018) A novel and compact review on the role of oxidative stress in female reproduction. Reprod Biol Endocrinol 16(1):80. https://doi.org/10.1186/s12958-018-0391-5
Mastroianni LJ, Jones R (1965) Oxygen tension within the rabbit fallopian tube. J Reprod Fertil 9:99–102. https://doi.org/10.1530/jrf.0.0090099
Agarwal A, Aponte-Mellado A, Premkumar BJ, Shaman A, Gupta S (2012) The effects of oxidative stress on female reproduction: a review. Reprod Biol Endocrinol 10:49. https://doi.org/10.1186/1477-7827-10-49
Hirao Y (2011) Oocyte growth in vitro: potential model for studies of oocyte-granulosa cell interactions. Reprod Med Biol 11(1):1–9. https://doi.org/10.1007/s12522-011-0096-3
Liang LF, Qi ST, Xian YX, Huang L, Sun XF, Wang WH (2017) Protective effect of antioxidants on the pre-maturation aging of mouse oocytes. Sci Rep. https://doi.org/10.1038/s41598-017-01609-3
Herta AC, Lolicato F, Smitz JEJ (2018) In vitro follicle culture in the context of IVF. Reprod 156(1):59–73. https://doi.org/10.1530/REP-18-0173
Khazaei M, Aghaz F (2017) Reactive oxygen species generation and use of antioxidants during In Vitro maturation of oocytes. Int J Fertil Steril 11(2):63–70
Wang S, He G, Chen M, Zuo T, Xu W, Liu X (2017) The role of antioxidant enzymes in the ovaries. Oxid Med Cell Longev 2017:4371714
Showell MG, Mackenzie-Proctor R, Jordan V, Hart RJ (2017) Antioxidants for female subfertility. Cochrane Database Syst Rev 7(7):CD007807. https://doi.org/10.1002/14651858.CD007807
Roychoudhury S, Agarwal A, Virk G, Cho CL (2017) Potential role of green tea catechins in the management of oxidative stress-associated infertility. Reprod Biomed Online 34(5):487–498. https://doi.org/10.1016/j.rbmo.2017.02.006
Nguyen TV, Wittayarat M, LtK Do et al (2018) Effects of chlorogenic acid (CGA) supplementation during in vitro maturation culture on the development and quality of porcine embryos with electroporation treatment after in vitro fertilization. Anim Sci J 89(8):1207–1213. https://doi.org/10.1111/asj.13049
Naveed M, Hejazi V, Abbas M, Kamboh AA et al (2018) Chlorogenic acid (CGA): a pharmacological review and call for further research. Biomed Pharmacother 97:67–74. https://doi.org/10.1016/j.biopha.2017.10.064
Tajik N, Tajik M, Mack I, Enck P (2017) The potential effects of chlorogenic acid, the main phenolic components in coffee, on health: a comprehensive review of the literature. Eur J Nutr 56(7):2215–2244. https://doi.org/10.1007/s00394-017-1379-1
Nguyen TV, Tanihara F, Ltk Do, Sato Y, Taniguchi M, Takagi M, Nguyen TV, Otoi T (2017) Chlorogenic acid supplementation during in vitro maturation improves maturation, fertilization and developmental competence of porcine oocytes. Reprod Domest Anim 52(6):969–975. https://doi.org/10.1111/rda.13005
Granado-Serrano AB, Martín MA, Izquierdo-Pulido M, Goya L, Bravo L, Ramos S (2007) Molecular mechanisms of (-)-epicatechin and chlorogenic acid on the regulation of the apoptotic and survival/proliferation pathways in a human hepatoma cell line. J Agric Food Chem 55(5):2020–2027. https://doi.org/10.1021/jf062556x
Samiec M, Skrzyszowska M (2014) Biological transcomplementary activation as a novel and effective strategy applied to the generation of porcine somatic cell cloned embryos. Reprod Biol 14(2):128–139. https://doi.org/10.1016/j.repbio.2013.12.006
Pang YW, An L, Wang P et al (2013) Treatment of porcine donor cells and reconstructed embryos with the antioxidant melatonin enhances cloning efficiency. J Pineal Res 54(4):389–397. https://doi.org/10.1111/jpi.12024
Lee J, Lee JR, Youm HW, Suh CS, Kim SH (2015) Effect of preoperative simvastatin treatment on transplantation of cryopreserved-warmed mouse ovarian tissue quality. Theriogenology 83(2):285–293. https://doi.org/10.1016/j.theriogenology
Ghavami M, Mohammadnejad D, Beheshti R, Solmani-Rad J, Abedelahi A (2015) Ultrastructural and morphalogical changes of mouse ovarian tissues following direct cover vitrification with different cryoprotectants. J Reprod Infertil 16(3):138–147
Monsefi M, Nadi A, Alinejad Z (2017) The effects of Salvia officinalis L. on granulosa cells and in vitro maturation of oocytes in mice. Int J Reprod Biomed 15(10):649–660
Somi MH, Hajipour B, Asl NA, Estakhri R, Azar AN, Zade MN, Haghjou AG, Vatankhah AM (2009) Pioglitazone attenuates ischemia/reperfusion-induced liver injury in rats. Transplant Proc 41(10):4105–4109. https://doi.org/10.1016/j.transproceed
Zhou RP, Lin SJ, Wan WB, Zuo HL, Yao FF, Ruan HB et al (2016) Chlorogenic acid prevents osteoporosis by Shp2/PI3K/Akt pathway in ovariectomized rats. PLoS ONE 11(12):e0166751. https://doi.org/10.1371/journal.pone.0166751
Wang X, Wang GC, Rong J et al (2018) Identification of steroidogenic components derived from Gardenia jasminoides Ellis potentially useful for treating postmenopausal syndrome. Front Pharmacol 9:390. https://doi.org/10.3389/fphar
Ali N, Rashid S, Nafees S, Hasan SK, Shahid A, Majed F, Sultana S (2017) Protective effect of Chlorogenic acid against methotrexate induced oxidative stress, inflammation and apoptosis in rat liver: an experimental approach. Chem Biol Interact 272:80–91. https://doi.org/10.1016/j.cbi
Dkhil MA, Moneim AEA, Bauomy AA, Khalil M, Al-Shaebi EM, Al-Quraishy S (2020) Chlorogenic acid prevents hepatotoxicity in arsenic-treated mice: role of oxidative stress and apoptosis. Mol Biol Rep 47(2):1161–1171. https://doi.org/10.1007/s11033-019-05217-4
Telfer EE, Andersen CY (2021) In vitro growth and maturation of primordial follicles and immature oocytes. Fertil Steril 115(5):1116–1125
Koriem KMM, Arbid MS (2017) Role of caftaric acid in lead-associated nephrotoxicity in rats via antidiuretic, antioxidant and anti-apoptotic activities. J Complement Integr Med. https://doi.org/10.1515/jcim-2017-0024
Appeltant R, Somfai T, Santos ECS, Dang-Nguyen TQ, Nagai T, Kikuchi K (2017) Effects of vitrification of cumulus-enclosed porcine oocytes at the germinal vesicle stage on cumulus expansion, nuclear progression and cytoplasmic maturation. Reprod Fertil Dev 29(12):2419–2429. https://doi.org/10.1071/RD16386
You J, Kim J, Lim J, Lee E (2010) Anthocyanin stimulates in vitro development of cloned pig embryos by increasing the intracellular glutathione level and inhibiting reactive oxygen species. Theriogenology 74(5):777–785
Choe C, Shin YW, Kim EJ, Cho SR, Kim HJ, Choi SH et al (2010) Synergistic effects of glutathione and β-mercaptoethanol treatment during in vitro maturation of porcine oocytes on early embryonic development in a culture system supplemented with L-cysteine. J Reprod Dev 56(6):575–582. https://doi.org/10.1262/jrd.09-214h
Nakamura BN, Fielder TJ, Hoang YD, Lim J, McConnachie LA, Kavanagh TJ et al (2011) Lack of maternal glutamate cysteine ligase modifier subunit (Gclm) decreases oocyte glutathione concentrations and disrupts preimplantation development in mice. Endocrinology 152(7):28062815–28062815
Tsai-Turton M, Luderer U (2006) Opposing effects of glutathione depletion and follicle-stimulating hormone on reactive oxygen species and apoptosis in cultured preovulatory rat follicles. Endocrinology 147(3):1224–1236. https://doi.org/10.1210/en.2005-1281
de Matos DG, Furnus CC (2000) The importance of having high glutathione (GSH) level after bovine in vitro maturation on embryo development effect of beta-mercaptoethanol, cysteine and cystine. Theriogenology 53(3):761–771. https://doi.org/10.1016/S0093-691X(99)00278-2
de Matos DG, Gasparrini B, Pasqualini SR, Thompson JG (2002) Effect of glutathione synthesis stimulation during in vitro maturation of ovine oocytes on embryo development and intracellular peroxide content. Theriogenology 57(5):1443–1451. https://doi.org/10.1016/s0093-691x(02)00643-x
Cogni´e Y, Baril G, Poulin N, Mermillod P (2003) Current status of embryo technologies in sheep and goat. Theriogenology 59(1):171–188. https://doi.org/10.1016/s0093-691x(02)01270-0
Rajaei S, Alihemmati Ph and Abedelahi Ph (2019) Antioxidant effect of genistein on ovarian tissue morphology, oxidant and antioxidant activity in rats with induced polycystic ovary syndrome. Int J Reprod Biomed 17(1):11–22. https://doi.org/10.18502/ijrm.v17i1.3816
Li Y, Dong Z, Liu S, Gao F, Zhang J, Peng Z et al (2022) Astaxanthin improves the development of the follicles and oocytes through alleviating oxidative stress induced by BPA in cultured follicles. Sci Rep 12(1):7853. https://doi.org/10.1038/s41598-022-11566-1
Lee CT, Wang JY, Chou KY, Hsu MI (2019) 1,25-Dihydroxyvitamin D3 modulates the efects of sublethal BPA on mitochondrial function via activating PI3K-Akt pathway and 17beta-estradiol secretion in rat granulosa cells. J Steroid Biochem Mol Biol 185:200–211. https://doi.org/10.1016/j.jsbmb
Wang X, Fan X, Yuan S, Jiao W, Liu B, Cao J, Jiang W (2017) Chlorogenic acid protects against aluminium-induced cytotoxicity through chelation and antioxidant actions in primary hippocampal neuronal cells. Food Funct 8(8):2924–2934. https://doi.org/10.1039/c7fo00659d
Otsuka F, Yao Z, Lee T, Yamamoto S, Erickson GF, Shimasaki S (2000) Bone morphogenetic protein-15. Identification of target cells and biological functions. J Biol Chem 275(50):39523–39528. https://doi.org/10.1074/jbc.M007428200
Glister C, Richards SL, Knight PG (2005) Bone morphogenetic proteins (BMP) -4, -6, and -7 potently suppress basal and luteinizing hormone-induced androgen production by bovine theca interna cells in primary culture: could ovarian hyperandrogenic dysfunction be caused by a defect in thecal BMP signaling? Endocrinol 146(4):1883–1892. https://doi.org/10.1210/en.2004-1303
Gui LM, Joyce IM (2005) RNA interference evidence that growth differentiation factor-9 mediates oocyte regulation of cumulus expansion in mice. Biol Reprod 72(1):195–199. https://doi.org/10.1095/biolreprod.104.033357
Diaz FJ, Wigglesworth K, Eppig JJ (2007) Oocytes are required for the preantral granulosa cell to cumulus cell transition in mice. Dev Biol 305(1):300–311. https://doi.org/10.1016/j.ydbio.2007.02.019
Chang HM, Qiao J, Leung PC (2016) Oocyte-somatic cell interactions in the human ovary-novel role of bone morphogenetic proteins and growth differentiation factors. Hum Reprod Update 23(1):1–18. https://doi.org/10.1093/humupd/dmw039
Persani L, Persani L, Rossetti R, Pasquale ED, Cacciatore C, Fabre S (2014) The fundamental role of bone morphogenetic protein 15 in ovarian function and its involvement in female fertility disorders. Hum Reprod Update 20(6):869–883. https://doi.org/10.1093/humupd/dmu036
Hashimoto O, Moore RK, Shimasaki S (2005) Posttranslational processing of mouse and human BMP-15: potential implication in the determination of ovulation quota. Proc Natl Acad Sci USA 102(15):5426–5431. https://doi.org/10.1073/pnas.0409533102
Acknowledgements
This work was supported by the Urmia University of Medical Sciences. Neda Abedpour designed and directed the project. All authors performed editing and approved the final version of the manuscript before being submitted, also participated in the finalization of the manuscript, and approved the final draft.
Funding
The current research was funded by the Urmia University of Medical Sciences (No. 2560).
Author information
Authors and Affiliations
Corresponding author
Ethics declarations
Conflict of interest
The authors declare no conflict of interest.
Ethical approval
Animal procedures and protocols were conducted in accordance with the Principles of Laboratory Animal Care—ethically approved by the Animal Care and Use Committee in Urmia University of Medical Sciences (UMSU). Approval was granted by the Ethics Committee of Urmia University of Medical Sciences (IR.UMSU.REC.1398.078).
Additional information
Publisher's Note
Springer Nature remains neutral with regard to jurisdictional claims in published maps and institutional affiliations.
Supplementary Information
Below is the link to the electronic supplementary material.
Rights and permissions
Springer Nature or its licensor (e.g. a society or other partner) holds exclusive rights to this article under a publishing agreement with the author(s) or other rightsholder(s); author self-archiving of the accepted manuscript version of this article is solely governed by the terms of such publishing agreement and applicable law.
About this article
Cite this article
Abedpour, N., Javanmard, M.Z., Karimipour, M. et al. Chlorogenic acid improves functional potential of follicles in mouse whole ovarian tissues in vitro. Mol Biol Rep 49, 10327–10338 (2022). https://doi.org/10.1007/s11033-022-07793-4
Received:
Accepted:
Published:
Issue Date:
DOI: https://doi.org/10.1007/s11033-022-07793-4