Abstract
This study investigated the anti-leukemic effects of Cedrus atlantica extract (CAt extract) on cell cycle distribution and apoptosis in human acute myeloid leukemia (AML) cells. AML often occurs in older adults, accounting for 60% of the cases, and is likely to be resistant to chemotherapy due to multidrug resistance, resulting in early death during cancer treatment. With the increasing focus on prevention medicine, natural plant components are being used as a major source for the development of therapeutic drugs or functional foods to cure or alleviate the disease. Cedrus species are known to have anti-inflammatory, antimicrobial, antiviral, and anticancer effects; however, the anticancer effects of CAt extract have not been elucidated. In this study, CAt extract demonstrated an inhibitory effect on human leukemia cells in a concentration-dependent manner; CAt extract induced G0/G1 phase arrest via restrained protein levels of p-Rb and cell cycle-related proteins. After CAt extract exposure, the extrinsic and intrinsic apoptotic pathways were activated through caspase-8, -9, and -3 cleavage. Additionally, CAt extract suppressed VEGF, MMP-2, and MMP-9 expression. This study demonstrated that CAt extract treatment significantly reduced cell growth, cell cycle arrest in the G0/G1 phase, and induction of apoptosis, leading to leukemia cell death.
Similar content being viewed by others
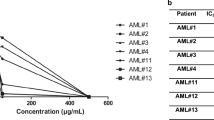
Avoid common mistakes on your manuscript.
Introduction
Acute myeloid leukemia (AML) is multidisciplinary disease characterized by various gene mutation which mediate proliferation and differentiation of cancer cells [1, 2]. Therapeutic options for leukemia are including chemotherapy, target therapy, radiation therapy, and hematopoietic stem cell transplantation. Overall 5-year survival which is less than 8% remains poor in older AML patients who are over 65 years due to comorbid disease and impaired bone marrow stem cell reserve [3,4,5,6]. Additionally, older patients are likely to have multidrug-resistant protein expression that affects the dosage of the chemo-drugs and the performance status of older patients is vulnerable to excess toxicity in chemotherapy, resulting in early mortality [7,8,9]. Despite the advances in understanding the molecular heterogeneity and pathogenesis of AML, there has been little progress in the standard therapy for AML. Hence, there is an urgent need for novel therapeutic agents with less toxicity for the treatment of AML.
Previous studies have revealed several mechanisms involved in the chemopreventive effects of natural plant components, including antioxidative and anti-inflammatory properties, induction of cell cycle arrest, and apoptosis in tumorous cells [10,11,12,13]. Interfering with the cell cycle can lead to cell cycle arrest, cell death, or senescence, which has proven to be highly successful in cancer treatment [14]. Apoptosis is a type of programed cell death that characterizes cell blebbing, cell shrinkage, chromatin condensation, and DNA fragmentation [15]. Therefore, induction of apoptosis and cell cycle arrest is important to regulate the proliferation of tumor cells.
Cedrus atlantica is an evergreen tree known for its phytochemical properties. It is traditionally used for treating skin diseases, cystitis, and bronchitis, for insect-repellent and in perfume [16,17,18]. The pharmacological properties of Cedrus species include anti-inflammatory and analgesic effects [19, 20], as well as immunomodulatory [21], antioxidant [22], antibacterial [23, 24], and insecticidal activities [25]. Moreover, Cedrus species have been shown to have antitumor activity against AML and hepatoma, but the anti-leukemic effect of C. atlantica extract (CAt extract) remains unknown [26,27,28,29]. Therefore, to unravel the antitumor mechanism of CAt extract on leukemia cells.
Materials and methods
Reagents, antibodies, and chemicals
All the other cell culture reagents and BCA Protein Assay Reagent were purchased from Gibco/Thermo Fisher Scientific (Waltham, MA, USA), and all other chemicals were of research grade. Dimethylsulfoxide (DMSO), propidium iodide (PI), and 3-(4,5-dimethylthiazol-2-yl)-5-(3-carboxymethoxyphenyl)-2-(4-sulfophenyl)-2H-tetrazolium (MTS) were purchased from Sigma-Aldrich (St. Louis, MO, USA). T-Pro LumiFast Plus Chemiluminescence Detection Kit was purchased from T-Pro Biotechnology (New Taipei County, Taiwan). The In Situ Cell Death Detection Kit was purchased from Roche (Mannheim, Germany). The primary antibodies against Rb, pRb, proliferating cell nuclear antigen (PCNA), cyclin dependent kinase 2 (cdk2), cdk4, cyclin B1, cyclin D1, FAS, caspase-3, caspase-8, caspase-9, and the horseradish peroxidase (HPR)-conjugated secondary antibody were purchased from Santa Cruz Biotechnology, Inc. (CA, USA). The primary antibodies against Bax, vascular endothelial growth factor (VEGF), matrix metalloproteinases-2 and -9 (MMP-2, -9), and Actin were purchased from iReal Biotechnology Co., Ltd. (Hsinchu, Taiwan).
Preparation of Cedrus atlantica extract
A fresh bark of Cedrus atlantica plant from American was utilized. Extraction of C. atlantica in small-scale was testify in our lab and conditions was showed as below. The generated steam (flow rate: 7.2 ml/min) was passed through the fresh bark of C. atlantica (500 g) at 100–105 °C for 100 min. Cedrus atlantica extract (CAt extract) was extracted by steam distillation and was commissioned by Phoenix (New Jersey, USA) in large-scale. The CAt extract was preserved in aluminum can at 4 °C. CAt extract was dissolved in DMSO and measured in μg/ml to utilize throughout the experiments.
Cell lines and cell culture
HL-60, K562, Jurkat, P338D1, and RAW264.7 were purchased from American Type Culture Collection (Manassas, VA, USA) or the Bioresources Collection and Research Center (BCRC, Hsinchu, Taiwan). HL-60 and K562 cells were performed FemtoPath Primer Set (HongJing Biotech., New Taipei City, Taiwan) to check to gene status of those cells. The cells were grown in Dulbecco's modified Eagle medium (DMEM) or Roswell Park Memorial Institute (RPMI) 1640 medium supplemented with 10% fetal bovine serum (FBS; Gibco, Grand Island, NY, USA; origin: Mexico), 1% penicillin/streptomycin, and 1% sodium pyruvate at 37 °C with 5% CO2.
Cell viability assay
The cells (5 × 103 cells/100 μl) were seeded in 96 well plates and incubated for 12 h. After that, the cells were exposed to CAt extract (0–200 μg/ml) for 12, 24, and 48 h. Each experiment was carried out in triplicate and the percentage cell viability of the treatment and control was calculated according to the formula as follows: Percentage of cell viability (%) = (Absorbance of treated cells/ Absorbance of control) × 100%. The cell inhibition of CAt-induced cells was evaluated using the MTS assay. Absorbance at 490 nm was determined using Spectra Max plus 384 Microplate Reader (Molecular Devices, USA) [30].
Cell cycle analysis
Cells (5 × 106 cells/6 ml) were treated with CAt (0, 5, 15, and 25 μg/ml) for the indicated time intervals. Afterwards, the cells were harvested and washed twice with phosphate-buffered saline (PBS). After washing with PBS, the cells were stained with propidium iodide (PI, 40 μg/ml) and RNase A (10 mg/ml) overnight at 4 °C. Cell cycle distribution was measured using a flow cytometer (BD, NJ, USA), and the percentage of cells in different phases were determined using Cell Quest Pro Software and analyzed by FlowJo 7.6.1 (Ashland, Oregon, USA) [31].
Terminal transferase dUTP nick end labeling (TUNEL) assay
The TUNEL assay was performed to detect the effect of CAt extract on apoptosis. Cells (5 × 106 cells/6 ml) were seeded at 10 cm dish and treated with CAt extract (15 μg/ml) for 24 h, and then the cells were smeared on slides. After that, the slides were prepared to detect for TUNEL staining according to the manufacturer’s protocols and stained with propidium iodide (PI, 10 μg/ml) for 10 min as counter stain (red). For analysis, the images were observed under a microscope (ZEISS AXioskop2, Bremen, Germany) at 400 × magnification to detect apoptotic morphology with TUNEL positive (green) [32].
Western blotting
The cells (5 × 106 cells/6 ml) were seeded in a 10 cm dish and treated with CAt extract (0, 5, 15, and 25 μg/ml) for different time intervals. After treatment, the cells were collected by centrifugation and then lysed using 1 × RIPA buffer (Bio Basic Inc, Toronto, Canada). After that, 20 μg of protein from control and CAt extract treated cells were separated on 8–12% Sodium dodecyl sulfate polyacrylamide gel electrophoresis (SDS-PAGE) and 4% stacking gel and then transferred onto a polyvinylidene fluoride (PVDF) membrane. The membranes were blocked with 10% skim milk for 1 h and then incubated with primary antibodies (1/200 dilution, Santa Cruz Biotechnology, Inc.; 1/500 dilution, iReal Biotechnology Co., Ltd.) overnight at 4 °C. After washing, the membranes were incubated with HRP-conjugated secondary antibodies (1:1000) and signals were detected using a T-Pro LumiFast plus Chemiluminescence Detection Kit and images were captured using the ImageQuant LAS 4000 (GE Healthcare Life Sciences, Little Chalfont, UK) image reader [33].
Statistical analysis
The data are shown as means ± standard deviation (SD) from triplicate experiments and evaluated using Student’ s t-test. Statistical significance was considered at p < 0.05.
Results
CAt extract inhibited the proliferation of leukemia cells
To examine the antiproliferative effect of CAt extract on leukemia and macrophage cells, the cells were exposed to serial concentrations of CAt extract (0–200 μg/ml) for 12, 24, and 48 h. Figure 1 showed that CAt extract inhibited the growth of HL-60 cells in a concentration-dependent manner and reduced about 70% cell viability at the concentration greater than 25 μg/ml. CAt extract showed similar inhibitory effects on Jurkat and K562 cells and the IC50 of these two cells were presented in Table 1. Besides, Jurkat cells were more sensitive to CAt extract than HL-60 and K562 cells. CAt extract inhibited macrophage cell growth (RAW264.7 and P338D1) at high concentrations (≥ 25 μg/ml) at the 12 and 24 h, but induced macrophage proliferation at low concentrations (≤ 25 μg/ml) and then, decreased the cell viability of macrophages at the high concentration of CAt extract (≥ 25 μg/ml). Interestingly, the inhibitory manner of CAt extract (≥ 100 μg/ml) in macrophage cell was similar with leukemia cells at 48 h. Moreover, the IC50 of CAt extract on normal macrophages was higher than that of leukemia cells, ranging from 1.5 to 3.1-fold. Thus, these suggested that CAt extract strongly inhibited leukemia cell proliferation and was less cytotoxic to normal macrophages. Next, to observe the cell morphology of CAt extract-induced, HL-60 cells were exposed CAt extract (0, 5, 15, and 25 μg/ml) for 24 h. The results showed a concentration dependent decrease in cellular density. Besides, the morphology of HL-60 cells was showed that the cell membrane was not smooth and cell bodies was shrinking, result in lots of cell debris and cell death (Fig. 2a). These data revealed that CAt extract did affected HL-60 cells growth and caused cell death.
Effects of CAt extract on cell cycle distribution. a Alterations in cell morphology observed by microscopy. HL-60 cells were treated with CAt extract (0, 5, 15, and 25 μg/ml) for 24 h and observed the cell morphology under × 400 magnification. After treatment with CAt extract, HL-60 cells were stained with propidium iodide (PI, 40 μg/ml) and analyzed for DNA content by flow cytometry (b–d). *It was significantly increased compared to the control with CAt extract treatment, #p < 0.05; it was significantly decreased compared to control with CAt extract treatment, p < 0.05. The values were obtained from three independent experiments
CAt extract induced cell cycle arrest at the G0/G1 phase in HL-60 cells
To explore the antiproliferative mechanism of CAt extract-induced, we analyzed the cell cycle progression after CAt extract treatment. As Fig. 2b, CAt extract altered cell cycle progression and induced cell population of HL-60 cells markedly accumulated at G0/G1 phase. HL-60 cells treating with serial concentration of CAt extract revealed the cell population of G0/G1 phase was prominently increased (48.32 ± 0.62%, 69.24 ± 1.14%, 72.39 ± 1.48% and 75.1 ± 1.32%) and significantly decreased S and G2/M phases (Fig. 2c). Moreover, after exposing the CAt extract on HL-60 cells with indicated time intervals, the similar results were presented at Fig. 2d, suggesting CAt extract induced cell cycle arrest at G0/G1 phase followed by a decrease at S and G2/M phases. These results indicated that CAt extract inhibited the proliferation of HL-60 cells by inducing cell cycle arrest at the G0/G1 phase with time and dose pattern.
CAt extract contributed to apoptosis in HL-60 cells
After CAt extract treatment, an increasing of cell debris and cell death was observed. Besides, Fig. 3a showed that sub-G1 phase level for HL-60 cells clearly increased in the presence of CAt extract (0, 5, 15, and 25 μg/ml) as compared with control, especially, at 25 μg/ml of CAt extract treatment causing 89.7% cell population accumulating at the sub-G1 phase. Thus, we performed TUNEL assay to detect whether CAt extract induced apoptosis in HL-60 cells. The results showed that CAt extract caused characteristic morphological changes of the cells, such as DNA fragments and apoptotic bodies and stimulated about 82.7% of cell apoptosis (Fig. 3b). Furthermore, to testify whether CAt extract induced cell apoptosis in P338D1 cells, the results showed that CAt extract slightly increased TINEL positive cells in P338D1 cells and was no statistic significant on induction of apoptosis in P338D1 cells (Fig. 3c). These results demonstrated that CAt extract induced apoptosis in HL-60 cells and exerted a less cytotoxic to P338D1 cells.
Effects of CAt extract on apoptosis in HL-60 cells. a The sub-G1 phase was determined by flow cytometric analysis. DNA fragmentation was investigated by TUNEL staining in HL-60 cells (b) and P338D1 cells (c). PI, propidium iodide; blue arrow, DNA fragments; white arrow, apoptotic body. *It was significantly increased compared to the control with CAt treatments, p < 0.05. The experiments were obtained from three independent experiments. (Color figure online)
CAt extract modulated cell cycle-related and apoptotic-associated protein expressions
To elucidate the molecular mechanisms of CAt extract-induced on HL-60 cells, the cell cycle and apoptotic-related key proteins were examined. Figure 4 showed that, after CAt extract treatment, the level of total Rb was markedly reduced at 48hrs but remained its protein expression in different dosage of CAt extract treatment; however, the level of p-Rb was sharply decreased in dose- and time-dependent manner. Moreover, we observed decreased protein levels of cell cycle-related modulators. In addition, the level of PCNA noticeably was reduced at 25 μg/ml of CAt extract and exposing to CAt extract for 48 h. The level of cdk2 and cdk4 were showed no clearly decreased after CAt extract, by contrast, the level of cyclinB1 and cyclinD1 was rapidly and apparently reduced at 5 μg/ml of CAt extract and treated with CAt extract for 6 h. These data indicated that CAt extract modulated cell cycle related protein to trigger the cell population accumulating at G0/G1 phase to achieve inhibition of cell proliferation.
Effect of CAt extract on cell cycle and apoptosis regulatory protein levels. Representative changes in the expression of these proteins after treatment with various concentrations of CAt extract for the indicated time intervals by western blot analysis. GAPDH protein expression served as a loading control. Data represent the mean of three independent experiments
Next, to validate the mechanism of CAt-induced apoptosis pathway, ligand receptor and mitochondrial induced apoptosis related proteins were assessed. The level of FAS was reduced; however, cleaved caspase-8 increased at 25 μg/ml of CAt extract and giving CAt extract for 48 h, suggesting that the extrinsic apoptotic pathway was activated. In the mitochondrial-induced apoptosis pathway, the level of Bax increased, followed by a decrease of procaspase-9, indicating that the intrinsic apoptosis pathway was triggered by CAt extract treatment. Ultimately, the protein level of procaspase-3 was detected to be reduced with dose and time dependent manner, suggesting the caspase cascade was turned on after CAt extract treatment in HL-60 cells. On the other hand, to address whether the CAt extract suppressed the angiogenic and metastatic related proteins and the protein levels of VEGF, MMP-2, and MMP-9 were determined. We found that CAt extract not affected the level of VEGF in HL-60 cells, suggesting CAt extract might show little effect on repression of VEGF protein expression. The level of MMP-2 and MMP-9 was reduced after CAt extract treatment in serial of concentration and time intervals. These results demonstrated that CAt extract reduced HL-60 cells growth by downregulating cell cycle regulators, leading to cell cycle arrest and activation of the caspase cascade, causing cell apoptosis. Besides, CAt extract also reduced angiogenic- and metastatic-related protein expression.
Discussion
We demonstrated the antiproliferative properties of CAt extract in AML, Acute lymphocytic leukemia (ALL), and Chronic myeloid leukemia (CML). Interestingly, HL-60 and Jurkat cells were more sensitive to CAt extract than K562 cells with multiple resistance characteristics [34, 35]. In addition, a previous study also found that C. atlantica extract inhibited the proliferation of the K562 cell line, exhibiting an IC50 value of 59.37 ± 2.6 µg/ml after 5 days treatment and revealed a long-term inhibitory effects of C. atlantica extract [29]. Moreover, our results indicated for the first time, a significant proliferative effect of CAt extract in RAW 264.7 and P338D1 macrophage cells at the low dose of CAt extract treatment. Macrophages play an essential role in immunity and inflammatory processes, and release numerous cell factors that regulate the activity of other immune cells. As a result, CAt extract exhibited an inhibitory ability against leukemia cells and CAt extract might have an immunomodulating effect on macrophages. Such a hypothesis would need to be explored in the future and also to verify the lower cytotoxic effects on normal cells.
We further investigated the antileukemic mechanisms of CAt extract. First, the results indicated that CAt extract triggered cell population accumulating at G0/G1 phase by flowcytometry analysis. After that, to explore CAt extract-mediated cell cycle mechanisms on HL-60 cells, the data revealed that CAt extract repressed the level of total Rb and p-Rb, which is a tumor suppressor playing an essential role in the negative control of the cell cycle, and is responsible for the G1 checkpoint to block S-phase entry and cell growth [36]. Moreover, the level of phosphorylated Rb was rapidly diminished at 6 h and lasted the suppressing effects to 48 h, suggesting that CAt extract represented strongly inhibitory effect on dephosphorylation of Rb. PCNA is a key protein in the regulation of DNA replication and cell cycle progression [37]. Our results indicated that CAt extract reduced the expression level of PCNA and indicated the CAt extract did reduce the HL-60 cell growth. Next, the level of cyclin, and cyclin-dependent kinase was reduced after CAt extract treatment. Among these, cyclinB1 and cyclinD1 protein expressions was repressed stronger than cdk4 and cdk2 protein expressions, suggesting that CAt extract was possible to regulate the cell cycle by inhibiting cyclin expression within 48hrs. Taken together, CAt extract might first reduce p-Rb expression (6 h), and rapidly diminished cyclin D1 and cyclin B1 expressions (6 h), and then affected PCNA, cdk2 and cdk4 (48 h). As a results, CAt extract modulated tumor suppressors (Rb) and affected downstream protein expression, leading to an inhibitory effect on HL-60 cells by blocking cell cycle progression at G0/G1 phase.
Besides, CAt extract also displayed the ability to induce cell death via apoptosis. Induction of apoptosis is generally a therapeutic approach due to cancer cell characteristics, such as excessive cell proliferation and insufficient cell apoptosis. The two main pathways of cell apoptosis are death receptor-mediated apoptosis pathway (the extrinsic pathway) and mitochondrial apoptotic pathway (the intrinsic pathway). In addition, the caspase family plays important roles in regulating apoptosis, such as caspase-8 cleavage as a critical protein for extrinsic pathway activation, caspase-9 as a vital protein for intrinsic pathway activation, especially caspase-3 as a key molecule to initiate the caspase cascade. Our data revealed that the level of FAS was reduced after CAt extract and the level of cleaved caspase-8 was increased, indicated CAt extract might induced other death receptor-mediated pathway to trigger HL-60 cell apoptosis. These results Our data demonstrated that CAt extract induced HL-60 cell apoptosis through the death receptor-mediated pathway and mitochondrial apoptotic pathway activation.
In conclusion, CAt extract could induce cell cycle arrest and apoptosis in HL-60 cells (Fig. 5). The CAt extract is a natural product that can be a potential source of anticancer therapeutics. Thus, our results provide a promising evidence that CAt extract could be a natural drug to treat AML with less pronounced side effects.
Data availability
The datasets generated during and/or analyzed during the current study are available from the corresponding author on reasonable request.
References
Schlenk RF (2014) Post-remission therapy for acute myeloid leukemia. Haematologica 99:1663–1670. https://doi.org/10.3324/haematol.2014.114611
Chaudhury SS, Morison JK, Gibson BES, Keeshan K (2015) Insights into cell ontogeny, age, and acute myeloid leukemia. Exp Hematol 43:745–755. https://doi.org/10.1016/j.exphem.2015.05.008
Hahn AW, Jamy O, Nunnery S, Yaghmour G, Giri S, Pathak R, Martin MG (2015) How center volumes affect early outcomes in acute myeloid leukemia. Clin Lymphoma Myeloma Leuk 15:646–654. https://doi.org/10.1016/j.clml.2015.07.646
Wang ES (2014) Treating acute myeloid leukemia in older adults. Hematol Am Soc Hematol Educ Program 2014:14–20. https://doi.org/10.1182/asheducation-2014.1.14
Ravandi F (2014) Are adjuncts to induction chemotherapy worthwhile in the treatment of acute myeloid leukemia? Best Pract Res Clin Haematol 27:241–246. https://doi.org/10.1016/j.beha.2014.10.005
Krug U, Buchner T, Berdel WE, Muller-Tidow C (2011) The treatment of elderly patients with acute myeloid leukemia. Dtsch Arztebl Int 108:863–870. https://doi.org/10.3238/arztebl.2011.0863
Ferrara F, Schiffer CA (2013) Acute myeloid leukaemia in adults. Lancet 381:484–495. https://doi.org/10.1016/S0140-6736(12)61727-9
Dombret H, Gardin C (2016) An update of current treatments for adult acute myeloid leukemia. Blood 127:53–61. https://doi.org/10.1182/blood-2015-08-604520
Nakazato T (2017) Treatment of elderly patients with acute myeloid leukemia. Rinsho Ketsueki 58:1905–1912. https://doi.org/10.11406/rinketsu.58.1905
Chandra-Kuntal K, Lee J, Singh SV (2013) Critical role for reactive oxygen species in apoptosis induction and cell migration inhibition by diallyl trisulfide, a cancer chemopreventive component of garlic. Breast Cancer Res Treat 138:69–79. https://doi.org/10.1007/s10549-013-2440-2
Nobili S, Lippi D, Witort E, Donnini M, Bausi L, Mini E, Capaccioli S (2009) Natural compounds for cancer treatment and prevention. Pharmacol Res 59:365–378. https://doi.org/10.1016/j.phrs.2009.01.017
Gullett NP, Ruhul Amin AR, Bayraktar S, Pezzuto JM, Shin DM, Khuri FR, Aggarwal BB, Surh YJ, Kucuk O (2010) Cancer prevention with natural compounds. Semin Oncol 37:258–281. https://doi.org/10.1053/j.seminoncol.2010.06.014
Shanmugam MK, Lee JH, Chai EZ, Kanchi MM, Kar S, Arfuso F, Dharmarajan A, Kumar AP, Ramar PS, Looi CY, Mustafa MR, Tergaonkar V, Bishayee A, Ahn KS, Sethi G (2016) Cancer prevention and therapy through the modulation of transcription factors by bioactive natural compounds. Semin Cancer Biol 40–41:35–47. https://doi.org/10.1016/j.semcancer.2016.03.005
Haschka M, Karbon G, Fava LL, Villunger A (2018) Perturbing mitosis for anti-cancer therapy: is cell death the only answer? EMBO Rep. https://doi.org/10.15252/embr.201745440
Elmore S (2007) Apoptosis: a review of programmed cell death. Toxicol Pathol 35:495–516. https://doi.org/10.1080/01926230701320337
Mojay G (2002) Healing the jade pool—the phyto-aromatic and acupressure treatment of dysmenorrhoea and menopausal syndrome: an East-West approach. Int J Aromather 12:131–141. https://doi.org/10.1016/S0962-4562(02)00057-7
Mojay G (2004) The aromatic and acupressure treatment of common musculoskeletal disorders: an Oriental medicine approach. Int J Aromather 14:81–88. https://doi.org/10.1016/j.ijat.2004.04.010
Lovell CR (1998) Some biblical plants of dermatological importance. Clin Dermatol 16:33–40. https://doi.org/10.1016/S0738-081X(97)00190-9
Thabrew MI, Dharmasiri MG, Senaratne L (2003) Anti-inflammatory and analgesic activity in the polyherbal formulation Maharasnadhi Quathar. J Ethnopharmacol 85:261–267. https://doi.org/10.1016/s0378-8741(03)00016-3
Emer AA, Donatello NN, Batisti AP, Oliveira Belmonte LA, Santos ARS, Martins DF (2018) The role of the endocannabinoid system in the antihyperalgesic effect of Cedrus atlantica essential oil inhalation in a mouse model of postoperative pain. J Ethnopharmacol 210:477–484. https://doi.org/10.1016/j.jep.2017.09.011
Shinde UA, Phadke AS, Nair AM, Mungantiwar AA, Dikshit VJ, Saraf MN (1999) Preliminary studies on the immunomodulatory activity of Cedrus deodara wood oil. Fitoterapia 70:333–339. https://doi.org/10.1016/S0367-326X(99)00031-3
Wu YP, Liang X, Liu XY, Zhong K, Gao B, Huang YN, Gao H (2015) Cedrus deodara pine needle as a potential source of natural antioxidants: bioactive constituents and antioxidant activities. J Funct Foods 14:605–612. https://doi.org/10.1016/j.jff.2015.02.023
Dakir M, El Hanbali F, Mellouki F, Akssira M, Benharref A, Quilez Del Moral JF, Barrero AF (2005) Antibacterial diterpenoids from Cedrus atlantica. Nat Prod Res 19:719–722. https://doi.org/10.1080/14786410512331330675
Zeng WC, He Q, Sun Q, Zhong K, Gao H (2012) Antibacterial activity of water-soluble extract from pine needles of Cedrus deodara. Int J Food Microbiol 153:78–84. https://doi.org/10.1016/j.ijfoodmicro.2011.10.019
Lamiri A, Lhaloui S, Benjilali B, Berrada M (2001) Insecticidal effects of essential oils against Hessian fly, Mayetiola destructor (Say). Field Crops Res 71:9–15. https://doi.org/10.1016/S0378-4290(01)00139-3
Shashi B, Jaswant S, Madhusudana RJ, Kumar SA, Nabi QG (2006) A novel lignan composition from Cedrus deodara induces apoptosis and early nitric oxide generation in human leukemia Molt-4 and HL-60 cells. Nitric Oxide 14:72–88. https://doi.org/10.1016/j.niox.2005.09.009
Saxena A, Saxena AK, Singh J, Bhushan S (2010) Natural antioxidants synergistically enhance the anticancer potential of AP9-cd, a novel lignan composition from Cedrus deodara in human leukemia HL-60 cells. Chem Biol Interact 188:580–590. https://doi.org/10.1016/j.cbi.2010.09.029
Shi X, Liu D, Zhang J, Hu P, Shen W, Fan B, Ma Q, Wang X (2016) Extraction and purification of total flavonoids from pine needles of Cedrus deodara contribute to anti-tumor in vitro. BMC Complement Altern Med 16:245. https://doi.org/10.1186/s12906-016-1249-z
Saab AM, Lampronti I, Borgatti M, Finotti A, Harb F, Safi S, Gambari R (2012) In vitro evaluation of the anti-proliferative activities of the wood essential oils of three Cedrus species against K562 human chronic myelogenous leukemia cells. Nat Prod Res 26:2227–2231. https://doi.org/10.1080/14786419.2011.643885
Gao HW, Huang XF, Yang TP, Chang KF, Yeh LW, Hsieh MC, Weng JC, Tsai NM (2019) Juniperus communis Suppresses melanoma tumorigenesis by inhibiting tumor growth and inducing apoptosis. Am J Chin Med 47:1171–1191. https://doi.org/10.1142/S0192415X19500605
Chien JH, Lee SC, Chang KF, Huang XF, Chen YT, Tsai NM (2020) Extract of Pogostemon cablin possesses potent anticancer activity against colorectal cancer cells in vitro and in vivo. Evid Based Complement Alternat Med 2020:9758156. https://doi.org/10.1155/2020/9758156
Lee CC, Hsiao CY, Lee SC, Huang XF, Chang KF, Lee MS, Hsieh MC, Tsai NM (2020) Suppression of oral cancer by induction of cell cycle arrest and apoptosis using Juniperus communis extract. Biosci Rep 40:BSR20202083. https://doi.org/10.1042/BSR20202083
Huang XF, Chang KF, Lee SC, Li CY, Liao HH, Hsieh MC, Tsai NM (2020) Extract of Juniperus indica Bertol synergizes with cisplatin to inhibit oral cancer cell growth via repression of cell cycle progression and activation of the caspase cascade. Molecules (Basel, Switzerland) 25:2746. https://doi.org/10.3390/molecules25122746
Yanovich S, Hall RE, Gewirtz DA (1989) Characterization of a K562 multidrug-resistant cell line. Cancer Res 49:4499–4503
Assef Y, Rubio F, Colo G, del Monaco S, Costas MA, Kotsias BA (2009) Imatinib resistance in multidrug-resistant K562 human leukemic cells. Leuk Res 33:710–716. https://doi.org/10.1016/j.leukres.2008.09.024
Giacinti C, Giordano A (2006) RB and cell cycle progression. Oncogene 25:5220–5227. https://doi.org/10.1038/sj.onc.1209615
Strzalka W, Ziemienowicz A (2011) Proliferating cell nuclear antigen (PCNA): a key factor in DNA replication and cell cycle regulation. Ann Bot 107:1127–1140. https://doi.org/10.1093/aob/mcq243
Acknowledgements
The authors would like to acknowledge Instrument Center of Chung Shan Medical University for ZEISS Axio Imager A2 microscopy and BD FASCS Calibur facility.
Funding
The research Grant CSH-2014-C-031 and CSH-2016-C-025 from Chung Shan Medical University Hospital, Taiwan and the Grant Number CSMU-CYC-101-01 was funded by Ditmanson Medical Foundation Chia-Yi Christian Hospital, Taiwan.
Author information
Authors and Affiliations
Corresponding authors
Ethics declarations
Conflict of interest
All the authors declared that they have no conflict of interest to declare.
Additional information
Publisher's Note
Springer Nature remains neutral with regard to jurisdictional claims in published maps and institutional affiliations.
Rights and permissions
About this article
Cite this article
Hung, PH., Hsieh, MC., Lee, SC. et al. Effects of Cedrus atlantica extract on acute myeloid leukemia cell cycle distribution and apoptosis. Mol Biol Rep 47, 8935–8947 (2020). https://doi.org/10.1007/s11033-020-05947-w
Received:
Accepted:
Published:
Issue Date:
DOI: https://doi.org/10.1007/s11033-020-05947-w