Abstract
Clubroot disease poses a severe threat to rapeseed (Brassica napus) production worldwide and has recently been spreading across China at an unprecedented pace. Breeding and cultivation of resistant varieties constitute a promising and environment-friendly approach to mitigating this threat. In this study, the clubroot resistance locus PbBa8.1 was successfully transferred into SC4, a shared paternal line of three elite varieties in five generations by marker-assisted backcross breeding. Kompetitive allele specific PCR (KASP) markers of clubroot resistance gene PbBa8.1 and its linked high erucic acid gene (FAE1) were designed and applied for foreground selection, and 1,000 single-nucleotide polymorphisms (SNPs) were selected and used for the background selection. This breeding strategy produced recombinants with the highest recovery ratio of the recurrent parent genome (> 95%) at BC2F2 while breaking the linkage with FAE1 during the selection. An updated version of the paternal line (SC4R) was generated at BC2F3, showing significantly improved clubroot resistance at the seedling stage via artificial inoculation, and was comparable to that of the donor parent. Field trials of the three elite varieties and their updated versions in five environments indicated similar agronomic appearance and final yield. The introduced breeding strategy precisely pyramids the PbBa8.1 and FAE1 loci with the assistance of technical markers in a shorter period and could be applied to other desirable traits for directional improvement in the future.
Similar content being viewed by others
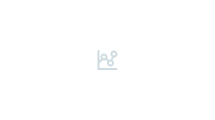
Avoid common mistakes on your manuscript.
Introduction
Rapeseed is a globally cultivated commercial crop, primarily grown for its oil. Clubroot disease (Plasmodiophora brassicae) is an increasing pathogenic threat to rapeseed (Brassica napus) plantations worldwide. Outbreaks of this disease have been frequently reported in Brassica growing areas. Recently, more than 20% total yield loss has been reported in highly infected fields of Canada, China, India, Europe, and Australia (Bhattacharya et al. 2014; Chai et al. 2014; Donald and Porter 2014; Rahman et al. 2014; Wallenhammar et al. 2014). In China, mechanized agricultural production across rapeseed growing regions has accelerated the spread of clubroot. Affected areas have expanded to Sichuan, Hubei, Hunan, Yunnan, and Anhui provinces, increasing by approximately 6.67 Mhm2 in a short period of time and causing heavy yield loss or no harvest at all in growing regions (Chai et al. 2014; Wang et al. 2021).
Seedling transplantation, crop rotation, late seed sowing, biological prevention, and application of resistant varieties are technical approaches to clubroot disease prevention used by the rapeseed industry (Niwa et al. 2007; Pang et al. 2014). However, the resting spores of P. brassicae can survive for over 20 years in the soil, making it difficult to control the infection using cultural or chemical approaches (Donald et al. 2001). Cultivation of resistant cultivars is a more reliable, low-cost, environmentally sustainable, and effective approach to limiting disease spread (Pang et al. 2018). Further, the utilization of clubroot resistance (CR) genes is the premise of disease resistance breeding. More than 26 CR genes have been identified and located on chromosomes A01, A02, A03, A06, and A08 of the A genome in European turnips and Chinese cabbage (Chen et al. 2013; Hirani et al. 2018; Pang et al. 2018; Huang et al. 2019; Karim et al. 2020). The resistance genes CRa and Crr1a have been cloned, are known to encode TIR-NBS-LRR (Toll-interleukin-1 receptor-like domain-nucleotide binding site-leucine-rich repeat) proteins, and share the same locus as CRb (Zhang et al. 2014; Hatakeyama et al. 2017; Shah et al. 2019). In B. rapa, the resistance genes CRk, Crr3, PbBa3.3, and PbBa3.2 are clustered in the same proximal region of chromosome A03 as CRa; however, it remains unclear if they represent a single or multiple genes (Hirai et al. 2004; Piao et al. 2004; Sakamoto et al. 2008; Chen et al. 2013). Additionally, Crr1, Rcr3, and PbBa8.1 have been identified in chromosome A08 from European folder turnips (Matsumoto et al. 1998; Suwabe et al. 2003, 2006; Pang et al. 2014).
Most of these genes were from different genetic resources and were associated with distinct P. brassicae pathotypes. PbBa8.1 was highly resistant to race 4, the most widespread group of P. brassicae, especially in China’s main rapeseed production area (Chen et al. 2013). When crossed with the bridging line ECD04, the clubroot resistant (CR) locus PbBa8.1 was successfully transferred into the elite conventional variety Huashuang5 (HS5), locating the gene within a 2.9 Mb region on chromosome A08, resulting in development of the ZHE226 line (Zhan et al. 2017, 2020). However, the seeds of the improved CR homozygous line contained high levels of erucic acid (approximately 18%) which is associated with potential health risks when ingested by humans and some animals. Therefore, selecting CR materials with low erucic acid content is crucial for rapeseed breeding programs (Tian et al. 2018). The erucic acid content determining gene FAE1 (fatty acid elongase 1) extends fatty acid chain length from C18 to C20 and C22 in B. napus (Han et al. 2001). It has been identified as the main gene that catalyzes the condensation step in the elongation of very long chain fatty acids, resulting in the accumulation of erucic acid (Sun et al. 2013). A close linkage between PbBa8.1 and FAE1 was revealed, and a co-segregating SSR marker CAP-134 has been designed which has the potential to assist in breaking the linkage drag between PbBa8.1 and FAE1 (Zhan et al. 2020).
These CR genes and their closely linked markers have greatly improved CR breeding through marker-assisted selection (MAS) in Brassica crops. Molecular markers have been developed from AFLP, RFLP, RAPD, SSR, InDel, and single-nucleotide polymorphism (SNP) markers (Lander and Botstein 1989; Lynch and Milligan 1994; Vos et al. 1995; Rafalski 2002; Qu and Liu 2013; Liu et al. 2015). Based on advanced SNP genotyping, Kompetitive Allele-Specific Polymerase Chain Reaction (KASP) has emerged as an accurate, cost-effective, and high-throughput SNP genotyping method (Pabinger et al. 2014) which has already been applied to MAS in wheat (Rasheed et al. 2016; Kaur et al. 2020), lentils (Wang et al. 2020a, b, c), maize (Chen et al. 2021), and rice (Feng et al. 2019; Cheon et al. 2020). However, MAS using DNA markers may result in the loss of desirable traits, or a gain in unwanted genes; therefore, clear genetic background selection is required during MAS to preserve original traits. Recently, the Bnapus50K array was developed specifically for B. napus. It provides more accurate and high-density SNPs and can be used for genomic background selection for B. napus breeding (Xiao et al. 2021).
In this study, the CR gene PbBa8.1 from the ZHE226 line was successfully transferred into a polima cytoplasmic male sterility (Pol-CMS) paternal line (SC4) through backcross breeding combining KASP markers and a 1,000 SNP panel. The breeding goal was achieved within five generations and produced an improved paternal line (SC4R), as well as three updated hybrid accessions (Fengyou 306R (FY306R); Fengyou 737R (FY737R); and Fengyou 792R (FY792R)) by crossing corresponding maternal lines. The CR level of SC4R was validated, and the agronomic performance of previous and updated varieties were evaluated and compared to provide resistant varieties for rapeseed production in disease affected areas. Additionally, a modified MAS-based breeding strategy was presented as a reference for selecting other traits in the future.
Materials and methods
Plant materials
The CR gene donor parent ZHE226 (provided by Prof. Chunyu Zhang, Huazhong Agricultural University) contained the resistance locus PbBa8.1, which had been transferred from an ECD04 donor into the elite rapeseed conventional variety HS5 following the method described by Zhan et al. (2020).
F1 plants were generated by crossing ZHE226 and SC4, the recurrent parent, which is a restoring line for Pol-CMS. A series of selfing or segregating populations were produced in the fields of Changsha (28.48 N, 113.36E) and Xining (36.57 N, 101.75E), as well as in a controlled environmental room from 2019 to 2021. Field evaluations of agronomic traits and yield were conducted at five different locations in Hunan Province, China (Fig. 1).
Genotyping and design of specific KASP markers
Seedlings from BC1F1 populations were subjected to foreground and background selection before further backcrossing, while seedlings from BC2F1 were subjected to foreground and recombinant selection (Fig. 1). The SNP panel used for background selection was designed based on the SNP results for SC4 and ZHE226 from the Bnapus50K array (Xiao et al. 2021). First, 5,374 out of 42,090 SNPs were initially selected according to their chromosomal distribution, which were then designed as markers for amplification, and a total of 2,426 SNPs could be designed and validated for markers. Second, 1,000 pairs of SNP markers were manually selected for background screening for even distribution in the chromosomes as much as possible. Finally, a total of 330 SNP markers showing polymorphisms between two parents were chosen for background screening, with an average density of 1 SNP marker per 3 Mb (Fig. S1).
The ratio of the recurrent parent genome was calculated based on the screening results of the 330 SNP loci at BC1F1 population. The recovery ratio (%) = No. of SNPs from recurrent parent (SC4)/ Total screened SNPs × 100%.
Foreground selection included a fertility gene (restorer gene of Pol-CMS, Rfp), CR gene (PbBa8.1 locus), and FAE1. Specific KASP markers for each gene were designed for SNPs around the coding region. KASP markers for Rfp were designed around the flanking sequences of Rfp with 1953 bp coding region about 29.2 K of A9 from B. rapa genome (Liu et al. 2012, 2016). They were designed and validated in Pol-CMS hybrid purity identification and published as technical regulations in Hunan Province Wang et al. 2020a, c).
KASP markers for PbBa8.1 and FAE1 were designed based on the polymorphic SNPs between HS5R and SC4, which were co-segregated with the previously reported closely linked marker CAP-134 (Zhan et al. 2020). In total, 14 SNP polymorphic markers around the position of CAP-134 (physical location: chrA08, 9.5 Mb) were obtained and used to design KASP markers for CR and FAE1 selection (Table S1). Three SNPs were selected and designed for KASP markers based on the sequences and applicable conditions and are highlighted in Table S1.
Plasmodiophora brassicae inoculation and resistance tests
The clubroot pathogen P. brassicae was isolated from a seriously infected B. napus plant collected from Xupu, Hunan Province, and was identified as race 4 using the Williams classification (Williams 1966). For the CR test, four steps were followed:
-
a)
Seedling preparation for inoculation: The seeds of SC4, BC2F3-L49, BC2F3-L59, and ZHE226 (control) were sown in nursery trays (2 cm × 2 cm per hole) using sterile soil, and the resulting seedlings were transferred into 10 cm × 10 cm × 9 cm plastic pots in a controlled environment room, at 20–25 °C room temperature and 70% relative humility, with an 18 h light period between 06:00 and 24:00. The plants were watered regularly to maintain soil moisture.
-
b)
Preparation of conidial suspension: Approximately 50 g of disease-affected clubroots were smashed then filtered with four-layer gauze, centrifuged for 15 min at 2,500 g, precipitated twice using 30 ml sterile water after discarding the upper layer, then precipitated using 50% sucrose solution, and further centrifuged twice using sterile water before counting on a blood cell counting plate (Pang et al. 2014, 2018).
-
c)
Inoculation to roots using conidial suspension: Prepared seedlings from step (a) were inoculated at the 4–5 leaf stage on July 8, 2021, and again on July 15, 2021, with a spore concentration of 1.59 × \({10}^{8}\) and 2.12 ×\({10}^{8}\) per ml, respectively. Seedlings were in separate pots to avoid cross infection, and 20 ml of conidial suspension was dripped onto roots using a pipette.
-
d)
Infection investigation: The seedling infection investigation was conducted 50 days post-inoculation (dpi). The roots were washed carefully and examined for P. brassicae infection; the number of plants at different infection levels was recorded; and the disease rate and index are calculated using the following equations:
$$\mathrm{Disease}\;\mathrm{rate}=\mathrm{No}\;\mathrm{of}\;\mathrm{infected}\;\mathrm{plants}/\mathrm{No}\;\mathrm{of}\;\mathrm{total}\;\mathrm{plants}\times100\%$$$$\mathrm{Disease}\;\mathrm{index}\;(\mathrm{DI})=\frac{\sum(\mathrm{No}\;\mathrm{of}\;\mathrm{infected}\;\mathrm{plants}\times\mathrm{Identified}\;\mathrm{level})}{\mathrm{No}\;\mathrm{of}\;\mathrm{the}\;\mathrm{highest}\;\mathrm{level}\times\mathrm{No}\;\mathrm{of}\;\mathrm{total}\;\mathrm{plants}}\times100\%$$
The classification of different levels of P. brassicae disease at seedling stage followed the criteria established by the Ministry of Agriculture for Brassicaceae crops as follows:
-
Level 0: no tumor at roots
-
Level 1: small tumor at lateral roots
-
Level 3: enlarged tumor at the main root with diameter less than 2 times that of the basal part of stem
-
Level 5: enlarged tumor at the main root with diameter 2–3 times that of the basal part of stem
-
Level 7: enlarged tumor at the main root with diameter 3–4 times that of the basal part of stem
-
Level 9: enlarged tumor at the main root with diameter more than 4 times that of the basal part of stem or turning black at the enlarged root
Evaluation of agronomic traits and erucic acid in seeds
The improved restorer SC4R was crossed with the corresponding maternal lines of FY306, FY737, and FY792 (H20A, X5A, and 167A) to produce the updated hybrid varieties FY306R, FY737R, and FY792R, respectively (Fig. 1).
Six accessions (FY792, FY792R, FY737, FY737R, FY306, and FY306R) were used to evaluate agronomic traits in fields from five environments in Hunan Province: Changsha (28.48 N, 113.36E), Changde (29.05 N, 111.69E), Hengyang (26.98 N, 112.39E,), Huaihua (27.52 N, 109.95E), and Jishou (28.3 N, 109.71E). The field experiments followed a randomized complete block design with three replicates (10 m long × 2 m wide for each plot). Between 25 and 27 plants were kept in each row with 33 cm between rows. Field management followed the regular methods of the China National Field Trials.
At harvest time, 10 representative plants from the middle of each plot were selected randomly for evaluation of agronomic traits, ensuring that each plant had normal growth vigor. Plant height, branch number, main inflorescence length, plant silique number, seed number per pod, 1000-seed weight, and total seed yield were measured and calculated for comparison. Quality traits such as oil, glucosinolate, and erucic acid content were tested using a near-infrared spectrometer (DS 2500F, Foss NIRSystems Inc., Denmark) with dried clean seeds (water content < 9%).
Microscopic investigation and data analysis
Following the examination of the P. brassicae infection during the resistance test, the roots from SC4, BC2F3-L49, and BC2F3-L59 were kept for microscopic investigation. Epidermal tissues from the healthy or enlarged tumor roots were repeatedly scraped into a drop of water on a clean microscope slide using a sharp blade. The tissue samples were covered with a coverslip and then examined under a microscope (DS-Ril, Nikon, Japan).
Agronomic trait data from 10 sample plants from five environments and quality trait data from five environments were analyzed using Microsoft Excel.
Results
Validation of specific KASP markers for PbBa8.1 and FAE1
Based on the genomic information of the previously reported SSR marker, CAP-134, which was co-segregated with PbBa8.1 and FAE1, three KASP markers were designed for PbBa8.1 and FAE1. They were SNP1 (seq-new-rs36374), SNP2 (seq-new-rs42497), and SNP3 (BN-A08-p11380260), which were approximately 130, 50, and 280 Kb away from CAP-134 and named BN900051, BN900052, and BN900053, respectively. BN900051 and BN900053 were used for selection of the CR gene PbBa8.1, and BN900052 was used for the selection of the FAE1 gene. The sequences of all the applied KASP markers in this study are presented in Table S2.
A total of 188 plants from the F2 population from crosses between ZHE226 and SC4 were used to validate the three KASP markers and showed consistent results as the previously reported SSR marker CAP-134 and well-distinguished in the genotyping diagram by the KASP platform (Fig. 2). Based on the results from BN900051 and BN900053, 132 plants were identified as clubroot-resistant (PbBa8.1/-), of which 44 were homologous at the locus (PbBa8.1/PbBa8.1). Two recombinants of the locus between PbBa8.1 and FAE1 were identified (PbBa8.1/FAE1) using BN900052. The erucic acid content of selfing seeds from the two recombinants varied between 0.34 and 0.56% (undetectable), while the erucic acid value varied between 16.25 and 18.95% for the non-recombinant lines (Table S3).
Genotyping diagram of BN900051 (a), BN900053 (b), and BN900052 (c). In BN900051, G:G, A:A, and G:A represent clubroot resistant, sensitive, and hybrid genotypes, respectively, while C:C, A:A, and C:A represent the corresponding genotypes in BN900053, respectively. In BN900052, T:T, A:A, and T:A represent high erucic acid, low erucic acid, and hybrid genotypes, respectively
Breeding strategies for obtaining CR and minimizing erucic acid content
In the BC1F1 population, 731 plants were subjected to foreground selection, 179 containing heterozygous PbBa8.1 (PbBa8.1/pbBa8.1) and homozygous Rfp (Rfp/Rfp) loci, and were used for genetic background analysis. The recovery ratio of the recurrent parent genome ranged from 64.7 to 88.5%, with an average of 75.0% (Fig. 3a). The two plants with the highest recovery ratio of the recurrent parent genome (> 87%) were used for further backcrossing with SC4 to produce BC2F1 (Fig. 1; Fig. 3b). For the BC2F1 generation, foreground selection and recombinant selection were applied to 198 plants, identifying 2 recombinants with a heterozygous PbBa8.1 locus (PbBa8.1/pbBa8.1) and homozygous recessive BnA.FAE1 locus (fae1/fae1), which were used for selfing to produce BC2F2. In the two populations of BC2F2, 50 of 224 plants contained the homozygous PbBa8.1 locus (PbBa8.1/PbBa8.1); these were subjected to further selfing to BC2F3, before testing for low erucic acid and CR.
a Distribution of the recovery ratio of recurrent parent genome at BC1F1 generation and b the SNP sources of the 330 polymorphic loci from different parents at the individual with the highest recovery ratio. The red, blue, and green bars represent the genotypic resources from SC4, ZHE226, and heterozygous in the BC1F1, respectively
CR test of improved lines at BC2F3 generation, and SC4 at seedling stage
At 50 dpi, seedlings from two improved BC2F3 lines (BC2F3-L49; BC2F3-L59), SC4, and ZHE226 were examined for disease infection. Infections occurred in all four lines, with a 100% infection rate for SC4 and 5.88%, 7.70%, and 2.17% for BC2F3-L49, BC2F3-L59, and ZHE226, respectively. The disease indices were calculated as 61.90 for susceptible SC4, 1.96 for BC2F3-L49, 2.56 for BC2F3-L59, and 1.40 for ZHE226. There were no significant differences between the two BC2F3 lines and the ZHE226 control (Table 1).
Microscopic and phenotypic examination of all the tested lines (Fig. 4) found disease introgression into the roots of SC4 (Fig. 4a) and clearer root cells in the BC2F3 improved lines (Fig. 4b,c), as well as the corresponding enlarged and healthy roots (Fig. 4d). As a result, the improved lines of BC2F3-L49 and BC2F3-L59 had significantly improved CR compared to the original SC4, with comparable resistance levels to the donor parent ZHE226.
Evaluation of agronomic performance between FY306, FY737, FY792 and their corresponding updated version (FY306R, FY737R, FY792R)
The updated versions of the three hybrids were produced by crossing SC4R with their corresponding maternal lines (H20A, X5A, and 167A). Field trials were conducted in five locations in Hunan Province to determine whether the updated version retained the original characters. Plant height, branch number, main inflorescence length, total pod number, seed number per pod, 1000-seed weight, seed yield, oil content, glucosinolate, and erucic acid content were measured and evaluated across five environments. The average value of each trait is presented in Table 2. Student’s t-test between the three varieties and their updated version showed no differences for any of the agronomic traits nor the quality traits, except for the most variable plant pod number, indicating that the updated version was similar to the original version.
Discussion
An increasing number of clubroot disease outbreaks in rapeseed growing areas have caused severe yield loss, resulting in urgent demand from growers for updated CR varieties with local adaptations to control the threat (Pang et al. 2018). Pol-CMS derived hybrids are the dominant varieties and transfer CR genes into the parental lines, and the corresponding hybrids are promising approaches to limit the threat of disease. Compared to sterile maternal lines, paternal lines with good combining ability are shared among hybrids; therefore, the common recovery line of three local varieties (FY306, FY737, and FY792) was chosen as the recurrent parent in the breeding program.
The donor parent ZHE226 is an earlier version of Huashuang5R (HS5R) and shares the same CR locus as HS5R, which has been widely applied as a CR variety in China. Unlike HS5R, ZHE226 has a high erucic acid content due to the FAE1 gene (Zhan et al. 2020). A combined KASP-assisted foreground selection and an SNP panel-assisted background selection strategy were applied to the breeding program, achieving the breeding goal within five generations. Ultimately, the homologous CR gene PbBa8.1, which was successfully transferred from ZHE226 to SC4, retained the original agronomic and quality traits of SC4 while improving CR to a level similar to that of ZHE226 (Fig. 1).
Efficient indoor selection by KASP and SNP panel based-genotyping
Backcrossing breeding is a traditional method of disease resistance breeding, transferring targeted R-genes from a donor parent into a recurrent parent by crossing and backcrossing (Swathi et al. 2019). It typically requires 7–8 generations, and field phenotypic selection during the process is often constrained by individual development, leading to low selection efficiency. In this study, in addition to the CR locus (PbBa8.1), the fertility gene of Pol-CMS (Rfp) and the high erucic acid determining gene FAE1 were also target genes during selection, making it even harder to complete the breeding goal in the same period. MAS was required to shorten breeding time and co-dominant SSR markers for those loci that had been previously developed (Liu et al. 2016; Wang et al. 2020a, b, c; Zhan et al. 2020). Nevertheless, standard procedures using SSR and running agarose gel for gene validation are labor-intensive and time consuming, not accessible for the current breeding scale, and require a large population to screen three genes and the recombinant individual between PbBa8.1 and FAE1 loci because they were closely linked (Zhan et al. 2020). Compared to SSR and other molecular markers, KASP can complete genotyping in 96-well or 384-well plates simultaneously and distinguish the allele sources accurately; moreover, it is very flexible and can genotype sequences containing multiple SNPs if mixed bases are added to the primer sequences (Patterson et al. 2017). Therefore, in this study, the SNP-based KASP platform enabled utilization of polymorphic SNPs near the targeted genes and development of five specific KASP markers for high-throughput screening of the BC1F1 and BC2F1 generations (Tables S1 and S2).
High recovery ratio of recurrent parent genome to shorten the backcrossing cycle
Traditional MAS may result in the introduction of undesirable genes or loss of desirable traits with unclear genetic backgrounds. In addition to foreground selection, background selection is essential as it is more accurate and efficient (Feng et al. 2019). Recently, a Bnapus50K array that provides precise genomic information on Chinese local accessions of B. napus was developed (Xiao et al. 2021). The recovery ratio of the recurrent parent genome was the key value required prior to further backcrossing or selfing. In this study, 330 polymorphic SNPs widely distributed across the 19 chromosomes of B. napus were used to identify the recovery ratio compared to the SC4 genome at the BC1F1 generation; the values ranged from 64.7% to 88.5% for 179 individual plants, with the average at 75% (Fig. 3a). Two plants that had the highest recovery ratio of the recurrent parent genome (> 87%) were used for further backcrossing with SC4 to produce BC2F1 (Fig. 3b), making us confident in obtaining a > 95% recovery ratio for the BC2 generation. Moreover, recombinant selection between PbBa8.1 and FAE1 was possible at BC2, which is earlier than at BC4 as reported by Zhan et al. (2020), and is consistent with their discussion.
Similar phenotypic and yield performance and necessity of pyramiding more CR genes
FY306, FY737, and FY792 are the representative local varieties, which are Pol-CMS hybrids and share the same parental line as SC4. Within five generations, an updated version of SC4 was produced (SC4R), as well as the corresponding hybrid varieties (FY306R, FY737R, and FY792R). The field trials of the updated hybrid varieties in the five environments showed that phenotypic and yield-quality performance was consistent with previous versions, and the artificial inoculation during CR testing led to confidence in the next stage of resistance testing in the field.
Large-scale cultivation of CR-improved local varieties tends to be the main approach to control loss caused by soil-borne root diseases in the rapeseed industry. In the long term, and with the development of mechanized production in China and other countries, the spread of P. brassicae spores will continue, resulting in different pathotypes with a wider distribution. Therefore, pyramiding different sources of CR genes into one variety is necessary to provide alternative durable resistance to a broader spectrum of pathotypes. Following a similar strategy, introducing other CR genes into maternal lines and obtaining combined CR genes in hybrid varieties would be accessible and efficient strategies for future CR breeding.
In conclusion, the breeding strategy introduced here combined specifically designed KASP markers and a 1000 + SNP panel for foreground selection, recombinant selection, and background selection to precisely introduced a CR gene (PbBa8.1) into a Pol-CMS recovery line within five generations while retaining the original phenotypic traits of the recurrent parent. The demonstrated process not only provides accessible CR-improved varieties for disease-infected rapeseed growing regions, but also sets a model reference for efficient breeding for more traits in the future.
Data availability
Additional datasets generated by this study are included in the Supplementary Materials.
References
Bhattacharya I, Dutta S, Mondal S, Mondal B (2014) Clubroot disease on Brassica crops in India. Can J Plant Pathol 36(1):154–160
Chai AL, Xie XW, Shi YX, Li BJ (2014) Research status of clubroot (Plasmodiophora brassicae) on cruciferous crops in China. Can J Plant Pathol 36:142–153
Chen J, Jing J, Zhan Z, Zhang T, Zhang C, Piao Z et al (2013) Identification of novel QTLs for isolate-specific partial resistance to Plasmodiophora brassicae in Brassica rapa. PLoS One 8(12):e85307
Chen ZJ, Tang DG, Ni JX, Li P, Wang L, Zhou JH et al (2021) Development of genic KASP SNP markers from RNA-Seq data for map-based cloning and marker-assisted selection in maize. BMC Plant Biol 21:157–167
Cheon KS, Jeong YM, Oh H, Oh J, Kang DY, Kim N et al (2020) Development of 454 new kompetitive allele-specific PCR (KASP) markers for temperate japonica rice varieties. Plants 9:1531–1544
Donald EC, Porter IJ (2014) Clubroot in Australia: the history and impact of Plasmodiophora brassicae in Brassica crops and research efforts directed towards its control. Can J Plant Pathol 36(1):66–84
Donald EC, Porter IJ, Lancaster RA (2001) Band incorporation of fluazinam (Shirlan) into soil to control clubroot of vegetable brassica crops. Aust J Exp Agric 41:1223–1226
Feng XM, Lin KX, Zhang WQ, Nan JZ, Zhang XH, Wang C et al (2019) Improving the blast resistance of the elite rice variety Kongyu-131 by updating the pi21 locus. BMC Plant Biol 19:249–260
Han J, Lühs W, Sonntag K, Zähringer U, Borchardt DS, Wolter FP et al (2001) Functional characterization of β-ketoacyl-CoA synthase genes from Brassica napus L. Plant Mol Biol 46:229–239
Hatakeyama K, Niwa T, Kato T, Ohara T, Kakizaki T, Matsumoto S (2017) The tandem repeated organization of NB-LRR genes in the clubroot resistant CRb, locus in Brassica rapa L. Mol Genet Genomics 292:397–405
Hirai M, Harada T, Kubo N, Tsukada M, Suwabe K, Matsumoto S (2004) A novel locus for clubroot resistance in Brassica rapa and its linkage markers. Theor Appl Genet 108(4):639–643
Hirani AH, Gao F, Liu J, Fu GH, Wu CR, McVetty PBE et al (2018) Combinations of independent dominant loci conferring clubroot resistance in all four turnip accessions (Brassica rapa) from the European clubroot differential set. Front Plant Sci 9:1628
Huang Z, Peng G, Gossen BD, Yu F (2019) Fine mapping of a clubroot resistance gene from turnip using SNP markers identified from bulked segregant RNA-Seq. Mol Breed 39:131–140
Karim MM, Dakouri A, Zhang Y, Chen Q, Yu F (2020) Two clubroot resistance genes, Rcr3 and Rcr9wa, mapped in Brassica rapa using bulk segregant RNA sequencing. Inter J Mol Sci 21(14):5033
Katsunori H, Keita S, Norio TR, Takeyuki K, Tsukasa N, Hiroyuki F et al (2013) Identification and characterization of Crr1a, a gene for resistance to clubroot disease Plasmodiophora brassicae Woronin) in Brassica rapa L. PLoS One 8:e54745
Kaur B, Mavi GS, Gill MS, Saini DK (2020) Utilization of KASP technology for wheat improvement. Cereal Res Commu 8057
Lander ES, Botstein D (1989) Mapping Mendelian factors underlying quantitative traits using RFLP linkage maps. Genetics 121(1):185–199
Liu Z, Liu PW, Hong DF, He QB, Yang GS (2012) Fine mapping and candidate gene analysis of the nuclear restorer gene Rfp for pol CMS in rapeseed (Brassica napus). Theor Appl Genet 125(4):773–779
Liu J, Qu J, Yang C, Tang D, Li J, Lan H et al (2015) Development of genome-wide insertion and deletion markers for maize, based on next-generation sequencing data. BMC Genomics 16(1):601
Liu Z, Yang ZH, Wang X, Li KD, An H, Liu J et al (2016) A mitochondria-targeted PPR protein restores pol cytoplasmic male sterility by reducing orf224 transcript levels in oilseed rape. Mol Plant 9(7):1082–1084
Lynch M, Milligan BG (1994) Analysis of population genetic structure with RAPD markers. Mol Ecol 3(2):91–99
Malosetti M, Zwep LB, Forrest K, Eeuwijk FAV, Dieters M (2021) Lessons from a GWAS study of a wheat pre-breeding program: pyramiding resistance alleles to Fusarium crown rot. Theor Appl Genet 134(6):1–12
Matsumoto E, Yasui C, Ohi M, Tsukada M (1998) Linkage analysis of RFLP markers for clubroot resistance and pigmentation in Chinese cabbage (Brassica rapa ssp. Pekinesis). Euphytica 104(2):79–86
Niwa R, Kumei T, Nomura Y, Yoshida S, Osaki M, Ezawa T (2007) Increase in soil PH due to Ca-rich organic matter application causes suppression of the clubroot disease of crucifers. Soil Biol Biochem 39:778–785
Pabinger S, Dander A, Fischer M, Snajder R, Sperk M, Efremova M et al (2014) A survey of tools for variant analysis of next-generation genome sequencing data. Brief Bioinform 15(2):256–278
Pang WX, Liang S, Li XN, Li PP, Yu S, Lim YP et al (2014) Genetic detection of clubroot resistance loci in a new population of Brassica rapa. Hort Environ Biotechnol 55(6):540–547
Pang W, Fu P, Li X, Zhan Z, Yu S, Piao Z (2018) Identification and mapping of the clubroot resistance gene CRd in Chinese cabbage (Brassica rapa ssp. pekinensis). Front Plant Sci 9:653.
Patterson EI, Fleming MB, Kessler KC, Nissen SJ, Gaines TA (2017) A KASP genotyping method to identify northern watermilfoil, Eurasian watermilfoil, and their interspecific hybrids. Front Plant Sci 8:752
Piao ZY, Deng YQ, Choi SR, Park YJ, Lim YP (2004) SCAR and CAPS mapping of CRb, a gene conferring resistance to Plasmodiophora brassicae in Chinese cabbage (Brassica rapa ssp. Pekinesis). Theor Appl Genet 108(8):1458–1465
Qu J, Liu J (2013) A genome-wide analysis of simple sequence repeats in maize and the development of polymorphism markers from next-generation sequence data. BMC Res Notes 6(1):403
Rafalski A (2002) Applications of single nucleotide polymorphisms in crop genetics. Curr Opin Plant Biol 5(2):94–100
Rahman H, Peng G, Yu F, Falk KC, Kulkarni M, Selvaraj G (2014) Genetics and breeding for clubroot resistance in Canadian spring canola (Brassica napus L.). Can J Plant Pathol 36(1):122–134
Rasheed A, Wen W, Gao FM, Zhai SN, Jin H, Liu JD et al (2016) Development and validation of KASP assays for genes underpinning key economic traits in bread wheat. Theor Appl Genet 129(10):1843–1860
Sakamoto K, Saito A, Hayashida N, Taguchi G, Matsumoto E (2008) Mapping of isolate-specific QTL for clubroot resistance in Chinese cabbage Brassica rapa L. ssp. pekinensis. Theo Appl Genet 117:759–767
Shah N, Sun J, Yu S, Yang Z, Wang Z, Huang F et al (2019) Genetic variation analysis of field isolates of clubroot and their responses to Brassica napus lines containing resistant gene CRb, PbBa8.1 and their combination inhomozygous and heterozygous state. Mol Breed 39:153.
Song YX, Duan XY, Wang PF, Li X, Yuan X, Wang ZY et al (2021) Comprehensive speed breeding: a high-throughput and rapid generation system for long-day crops. Plant Biotech J 10:1111
Steele KA, Quinton-Tulloch MJ, Amgai RB, Dhakal R, Khatiwada SP, Vyas D et al (2018) Accelerating public sector rice breeding with high-density KASP markers derived from whole genome sequencing of Indica rice. Mol Breed 38:38–51
Sun X, Pang H, Li M, Peng B, Guo H, Yan Q et al (2013) Evolutionary pattern of the FAE1 gene in Brassicaceae and its correlation with the erucic acid trait. PLoS One 8:e83535
Suwabe K (2006) Simple sequence repeat-based comparative genomics between Brassica rapa and Arabidopsis thaliana: the genetic origin of clubroot resistance. Genetics 173(1):309–319
Suwabe K, Tsukazaki H, Iketani H, Hatakeyama K, Fujimura M, Nunome T et al (2003) Identification of two loci for resistance to clubroot (Plasmodiophora brassicae Woronin) in Brassica rapa L. Theor Appl Genet 107(6):997–1002
Swathi G, Rani CVD, Md J, Madhav MS, Vanisree S, Anuradha Ch et al (2019) Marker-assisted introgression of the major bacterial blight resistance genes, Xa21 and xa13, and blast resistance gene, Pi54, into the popular rice variety, JGL1798. Mol Breed 39:58
Tian E, Liang H, Wang J, Guo J, Cao H, Lin S (2018) Variation and correlation of erucic acid, oleic acid and glucosinolate contents in Brassica rapa seeds. Asian Agric Res 10:57–60
Vos P, Hogers R, Bleeker M, Reijans M, Tvd L, Hornes M et al (1995) AFLP: a new technique for DNA fingerprinting. Nucleic Acids Res 23(21):4407–4414
Wallenhammar AC, Almquist C, Schwelm A, Roos J, Marzec-Schmidt K, Jonsson A, et al (2014) Clubroot, a persistent threat to Swedish oilseed rape production. Can J Plant Pathol 135–141
Wang TH, Li B, Guo YM, Liu XH, Li B, Peng P et al (2020a) Technical regulation for Pol CMS hybrids purity identification of Brassica napus L. by KASP method. HNZ267–2020a, Department of agriculture and rural areas, Hunan Provinces.
Wang D, Yang T, Liu R, Li NN, Wang XW, Ashutosh S et al (2020b) RNA-Seq analysis and development of SSR and KASP markers in lentil (Lens culinaris Medikus subsp. culinaris). Crop J 8:953–965
Wang TH, Li B, Guo YM, Zhang YT, Liu XH, Fan LY et al (2020c) Development of SSR markers associated with Polima CMS and its application. Mol Plant Breed 11:10
Wang YY, Yang ZQ, Yang QY, Zhang CY (2021) Genetic improvement and application of clubroot resistance in Brassica napus. J Huazhong Agric Uni 40(2):1–5
Williams PH (1966) A system for the determination of races of Plasmodiophora brassicae that infect cabbage and rutabaga. Phytopathol 56:624–626
Xiao Q, Wang HD, Song N, Yu Z, Khan I, Xie WB et al (2021) The Bnapus50K array: a quick and versatile genotyping tool for Brassica napus genomic breeding and research. G3 11(10):jkab241
Yang GL, Chen SP, Chen LK, Sun K, Huang CH, Zhou DH et al (2019) Development of a core SNP arrays based on the KASP method for molecular breeding of rice. Rice 12(1):21
Zhan ZX, Nwafor CC, Hou ZK, Gong JF, Zhu BB, Jiang YF et al (2017) Cytological and morphological analysis of hybrids between Brassicoraphanus and Brassica napus for introgression of clubroot resistant trait into Brassica napus L. PLoS One 12:e0177470
Zhan ZX, Jiang YF, Shah N, Hou ZK, Zhou YW, Dun BC et al (2020) Association of clubroot resistance locus PbBa8.1 with a linkage drag of high erucic acid content in the seed of the European turnip. Front Plant Sci 11:810
Zhang T, Zhao Z, Zhang CY, Pang WX, Choi SR, Lim YP, Piao ZY (2014) Fine genetic and physical mapping of the CRb gene conferring resistance to clubroot disease in Brassica rapa. Mol Breed 34:1173–1183
Acknowledgements
The clubroot-resistant donor parent was generously shared by Prof. Chunyu Zhang. We appreciate Prof. Zhongyun Piao for earlier examination of pathotypes from the Hunan clubroot-infected region. We are deeply thankful for the assistance with agronomic evaluation and field sampling provided by the five local agricultural research institutes of Changsha, Changde, Hengyang, Jishou, and Huaihua.
Funding
This project was supported by grants from the National Key Research and Development Program of China (Grant No. 2021YFD1600500), Natural Science Foundation for Young Scientists of Hunan Province (Grant No. 2021JJ40330), Changsha Municipal Natural Science Foundation (Grant No. kq2014179), and the China Agricultural Research System (CARS-12).
Author information
Authors and Affiliations
Contributions
Yiming Guo and Bao Li conducted the experiments and drafted the manuscript. Mei Li, Liang Qu, and Lianyi Fan were involved in the agronomic evaluation of the previous and updated varieties. Qian Yang took part in data analysis and plotting. Hongjian Zhu and Xinhong Liu participated in the artificial inoculation of seedlings. Tonghua Wang designed the experiment and had oversight of the entire process. All authors read and approved the final manuscript.
Corresponding author
Ethics declarations
Ethics approval and consent to participate
Not applicable.
Consent for publication
The authors consented for the publication.
Competing interests
The authors declare no competing interests.
Additional information
Publisher's note
Springer Nature remains neutral with regard to jurisdictional claims in published maps and institutional affiliations.
Supplementary Information
Below is the link to the electronic supplementary material.
Rights and permissions
About this article
Cite this article
Guo, Y., Li, B., Li, M. et al. Efficient marker-assisted breeding for clubroot resistance in elite Pol-CMS rapeseed varieties by updating the PbBa8.1 locus. Mol Breeding 42, 41 (2022). https://doi.org/10.1007/s11032-022-01305-9
Received:
Accepted:
Published:
DOI: https://doi.org/10.1007/s11032-022-01305-9