Abstract
Carbonate esters are used as additives in biodiesel to improve its thermophysical properties for better applicability. In this work, the experimental densities, dynamic viscosities and refractive indices for binary mixtures of dimethyl carbonate and four fatty acid esters (methyl decanoate, ethyl decanoate, methyl laurate, ethyl laurate) have been measured and presented over the complete concentration range at temperatures range from 293.15 to 323.15 K under normal pressure. The excess molar volumes and the viscosity deviations of the four binary mixtures were calculated with the measured data and correlated with the Redlich-Kister equation. The excess Gibbs energy of activation was also calculated. From the values of excess properties of the binary mixtures and its deviations indicate that the changes of molecular interactions in the mixtures. The experimental results could be useful for the study of biodiesel-carbonate blended fuels.
Similar content being viewed by others
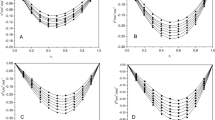
Avoid common mistakes on your manuscript.
1 Introduction
Thermophysical properties of liquid mixtures have important practical application values in many engineering fields, especially in fuel systems [1, 2]. The density of fuel can have a significant effect on the quality of fuel injection in engines, since the injection systems, pumps, and injectors must deliver precisely adjusted amounts of fuel to provide proper combustion [3]. Viscosity is the most important property of biodiesel since it affects the fluidity of the fuel and injection behavior [4, 5]. Viscosity affects the operation of fuel injection equipment, especially at low temperatures. If the viscosity is too high that may lead to less accurate operation of the fuel injectors and poorer atomization of the fuel spray [6]. Therefore, the essential physical properties of fuels such as density and viscosity of the blends are the important physicochemical characteristics of fuel related to fuel storing, transportation and utilization. These properties of blended fuels should be investigated that can provide important information for predictive and diagnostic engine combustion models.
Biodiesels are methyl or ethyl esters of long chain fatty acids that can be produced from renewable and biodegradable resources. It is biologically degradable that is less hazardous for the environment. The advantageous of biodiesel are that it has higher cetane number, higher combustion efficiency, contains no sulfur, safe in handling, non-toxic, etc. [7, 8]. Biodiesels are also easy to mix with normal diesel fuel. However, besides these advantages, biodiesels also have some disadvantages for using in engine such as high viscosity, low volatility and low heating value, which may lead to problems in pumping, atomization of fuel systems [9, 10]. Therefore, it is generally less appropriate for use in lower temperature. It is comparatively less inflammable than the normal diesel. One solution to alleviate this problem is to use add oxygenated compounds to a biodiesel to form blended fuels, examples are ethers, alcohols, esters, glycols, acetates, and carbonates [11,12,13]. Dimethyl carbonate (DMC) has been a green solvent with versatile applications due to its environmental-friendly attributes. It also be can an oxygenated fuel or additive for fuels and has a higher oxygen content than alcohols and excellent compatibility with biodiesel and diesel [14, 15]. DMC is considered as a promising additive for fuel because of its high oxygen content, no carbon–carbon atomic bonds, and excellent solubility. DMC additive can improve the contact of fuel with oxygen during combustion and emission performances. Adding DMC to fuels can solve the problem of hypoxia caused by local fuel-rich zones that can appear in the combustion process [16, 17]. Furthermore, DMC is insoluble in water, which reduces the pollution of groundwater. Razzaq et al. investigated the effect of palm biodiesel blends with DMC on compression ignition engines [14]. Ren et al. investigated the densities, surface tensions, and viscosities of the blends of diesel + DMC [18]. Liu et al. investigated the effect of DMC on density and viscosity of n-butanol as alternative to gasoline [19]. However, thermodynamics properties of fatty acid ethyl esters with DMC are still scarce in the literature.
In order to investigate the effects of the thermodynamics properties of biodiesel blended with DMC, the densities, dynamic viscosities and refractive indices of four binary mixtures of DMC and four fatty acid esters (methyl decanoate, ethyl decanoate, methyl laurate, ethyl laurate) have been measured in the temperature range from 293.15 to 323.15 K with an interval of 5 K and p = 0.1 MPa in the present work. The excess properties and deviations of the tested mixtures are calculated and fitted to the Redlich-Kister equation. These experimental results are expected to supplement the information for the DMC-biodiesel blending fuels.
2 Experimental Section
2.1 Chemicals Used
The samples of dimethyl carbonate, ethyl decanoate, methyl decanoate, ethyl laurate and methyl laurate were supplied by Aladdin Chemistry Co., Ltd., Shanghai, China. The reagents in this work were used without further purification. Table 1 lists the detailed information about the five samples of pure compounds.
2.2 Method
In this work, the samples were prepared by the weighing method with an analytical balance (Mettler Toledo AL204) and the stated precision is 0.0001 g. The combined standard uncertainty of the mole fraction is estimated to be 0.0002.
Densities (ρ) of pure components and their mixtures were determined with a DMA 4500 M densimeter (Anton Paar) with digital vibrating tube at T = (293.15 to 323.15) K and atmospheric pressure. The densitometer can automatically balance the temperature and is stable with a precision of 0.03 K. The densimeter can do self-checks and be calibrated with dried air and double-distilled water before determination. The uncertainty of densities of mixtures is related to the purity of pure compounds and measurement accuracy (± 0.00005 g·cm−3). The relative standard uncertainty of ρ is 0.0005.
The dynamic viscosities (η) of all samples at T = (293.15 to 323.15) K and atmospheric pressure were determined using an Anton-Paar AMVn viscometer in which a stainless steel ball rolls under gravity down a thermostated glass capillary. The temperature of the measurement cell is stable within ± 0.01 K. The calibration of the viscometer was carried out with freshly twice distilled water. The relative uncertainty of η is 0.010 [20].
Refractive indices (nD) of all samples at T = 293.15, 303.15, 313.15 and 323.15 K and atmospheric pressure were determined using a WAY-2 S refractometer (Shenguang). The measurement temperatures were controlled by circulating water bath with an accuracy of ± 0.02 K. The refractometer was calibrated with double-distilled water at each temperature. The presented refractive index values were the average values of three measurements. The uncertainty of the refractive indices is 0.0005.
2.3 Experimental Results
Densities (ρ), viscosities (η) and refractive indices (nD) have been measured for the pure components and four binary mixtures (DMC + methyl decanoate), (DMC + ethyl decanoate), (DMC + methyl laurate) and (DMC + ethyl laurate) in the temperature range from 293.15 to 323.15 K. The experimental values of ρ, η and nD of the binary liquid mixtures were provided in Tables 2, 3 and 4, respectively.
3 Results and Discussion
The experimental and literature [21,22,23,24,25,26,27,28,29,30,31,32,33,34,35,36,37,38,39,40,41,42,43] values of ρ, η and nD for the pure components are listed in Table 5. In order to compare the experimental and literature data, the absolute average relative deviations (AARD) are calculated according to Eq. 1. The results are also listed in Table 5.
In the above equation, Ylit is the value in the literature, and Yexp is the measured data in this work.
The excess molar volumes (\(V_{\text{m}}^{\text{E}}\)) and viscosity deviations (Δη) for four binary systems of DMC + fatty acid esters are calculated from the experimental data according to the following relations.
where x1 stands for the mole fractions of DMC in a binary mixture and x2 is the mole fractions of fatty acid ethyl ester; M1 and M2 stand for the molar masses of DMC and a fatty acid ethyl ester; ρm, ρ1 and ρ2 are the densities of the binary mixture, DMC and a fatty acid ethyl ester, respectively. η, η1 and η2 represent the dynamic viscosity of the measured viscosity of the mixture, DMC and a fatty acid ethyl ester, respectively.
The excess properties of the binary mixed solution will show the difference between the properties of the solution itself and that of the hypothetical solution under ideal conditions [25]. Figure 1 shows the trends of \(V_{\text{m}}^{\text{E}}\) for the four binary systems of DMC with methyl decanoate, ethyl decanoate, methyl laurate and ethyl laurate, respectively. The solid lines in Fig. 1 are the calculated results from Eq. 4. It can be observed that the \(V_{\text{m}}^{\text{E}}\) of the four binary mixed solutions are positive over the entire molar fraction range and the \(V_{\text{m}}^{\text{E}}\) increases with temperature. At the same temperature, the excess volume of the binary mixed solution first increased with the increase of the mole fraction of DMC, and then decreased after reaching the maximum. The maximum value appears at about x1(the mole fraction of DMC) ≈ 0.6. The positive deviation for the \(V_{\text{m}}^{\text{E}}\) of the binary system is thought to be caused by the expansion of the volume of the solution, as a result of the expansive effects associated with the breaking of the homo-molecular interactions [29, 44]. DMC is a polar molecule. Due to the presence of polar C = O bonds and C–O bonds in DMC molecules, DMC molecules exhibit dipole–dipole interactions. Fatty acid ethyl or methyl esters are generally considered to be nonpolar molecules due to the presence of predominantly nonpolar hydrocarbon chains in their structures. The polar ester functional group contributes some degree of polarity but the overall molecule is still relatively nonpolar. This means that during mixing the fatty acid ester molecules with DMC can weaken the dipole–dipole interactions in the case of DMC. The positive values of \(V_{\text{m}}^{\text{E}}\) are the result of a combination of the disruption of the dipole–dipole interactions between molecules and structural differences. An increase in system temperature leads to an increase in the volumes of the component molecules and the excess volume value increases with the increasing temperature. Figure 2 shows a comparison of \(V_{\text{m}}^{\text{E}}\) for (DMC + methyl caprate, DMC + ethyl caprate, DMC + methyl laurate, DMC + ethyl laurate) at 293.15 K. Comparing the mixture of two fatty acid methyl esters and fatty acid ethyl esters with DMC, it is found that the excess volume of the binary system composed of DMC and fatty acid methyl esters is smaller than that of the binary system composed of DMC and fatty acid ethyl esters at the same temperature and composition. At the same temperature and composition, the \(V_{\text{m}}^{\text{E}}\) of fatty acid ester mixtures increases with the increase of carbon chain. This indicates that the values of \(V_{\text{m}}^{\text{E}}\) for the binary systems are affected by the carbon chain length of fatty acid ethyl esters, and the increase of chain length of esters leads to the increase of the values of \(V_{\text{m}}^{\text{E}}\). In addition, the \(V_{\text{m}}^{\text{E}}\) of the binary mixture of (DMC + ethyl ester) is slightly larger than that of (DMC + methyl ester).
Excess molar volumes for binary systems as a function of mole fraction of DMC (a, DMC + methyl decanoate; b, DMC + ethyl decanoate; c, DMC + methyl laurate; d, DMC + ethyl laurate) at T = (293.15 − 323.15) K and pressure p = 0.1 MPa: Filled Square, 293.15 K; Filled Circle, 298.15 K; Filled Triangle, 303.15 K; Filled Down-Pointing Triangle , 308.15 K; Filled Lozenge, 313.15 K; Filled Left-Pointing Triangle, 318.15 K; Filled Right-Pointing Triangle, 323.15 K; Solid Line, Redlich-Kister equation correlations
Comparison of excess molar volumes of DMC + fatty acid ethyl esters at T = 293.15 K: Filled Square, DMC + methyl decanoate; Filled Circle, DMC + ethyl decanoate; Filled Triangle, DMC + methyl laurat; Filled Down-Pointing Triangle, DMC + ethyl laurate; Solid Line, Redlich-Kister equation correlations
Figure 3 displays the dependence of viscosity deviations as the molar fraction of DMC. A negative excess viscosity is observed for all binary systems from the curves of Δη − x1 for entire composition range. The minima of the (Δη − x1) curve for all the four binary mixtures occur at x1 ≈ 0.5–0.7. Moreover, the values of Δη become less negative with increasing temperatures, which means the absolute values of viscosity deviation decrease. The negative values of the viscosity deviations indicate that attractive forces among molecules are stronger than the repulsion forces [45]. A comparison of the viscosity deviation of the studied mixtures as a function of the mole fraction of DMC at 293.15 K is exhibited in Fig. 4. It indicates that the values of Δη become more negative with increasing alkyl ester chain length. The chain length of ester affects the viscosity deviations of the binary mixtures. The viscosity deviations for two systems of DMC + methyl or ethyl dodecanoate are higher than those of DMC + methyl or ethyl decanoate. There is not enough difference of viscosity deviation between the mixture of DMC + methyl and ethyl esters of fatty acids with the same carbon atom number.
Curves of viscosity deviations (Δη): A, DMC + methyl decanoate; B, DMC + ethyl decanoate; C, DMC + methyl laurate; D, DMC + ethyl laurate at T = (293.15 − 323.15) K and pressure p = 0.1 MPa: Filled Square, 293.15 K; Filled Circle, 298.15 K; Filled Triangle, 303.15 K; Filled Down-Pointing Triangle, 308.15 K; Filled Lozenge, 313.15 K; Filled Left-Pointing Triangle, 318.15 K; Filled Right-Pointing Triangle, 323.15 K; Redlich-Kister equation correlations
Viscosity deviations as a function of mole fraction of DMC for four binary systems at 293.15 K: Filled Square, DMC + methyl decanoate; Filled Circle, DMC + ethyl decanoate; Filled Triangle, DMC + methyl laurate; Filled Down-Pointing Triangle, DMC + ethyl laurate; Solid Lines, Redlich-Kister equation correlations
Excess values and deviations (\(V_{\text{m}}^{\text{E}}\), Δη) of binary mixtures are all fitted to the Redlich-Kister polynomial equation.
where xi and Ai are molar fraction and the polynomial coefficients of the Redlich-Kister equation; k is the number of coefficients. Ai are obtained by using the linear least-squares fitting method. The values correlated from Eq. 5 are used to identify the standard deviation of the experimental and theoretical values. The standard deviation (σ) is calculated by the following equation:
where Yexp and Ycal are the experimental and calculated value of excess properties or deviations. n is the number of datum points. The values of the fitting parameters (Ai) along with the standard deviations (σ) for \(V_{\text{m}}^{\text{E}}\) and Δη are all summarized in Tables S1 and S2 in the Supporting Information.
On the basis of the theory of absolute reaction rates, the excess Gibbs energy (ΔGE*) of activation of viscous flow can be calculated by using Eq. 6:
In Eq. 6, V, V1 and V2 are the molar volumes of the binary mixture, DMC and a fatty acid ethyl ester, respectively. The sign of the values of ΔGE* can be considered as a reliable criterion for detecting or excluding the presence of interaction between unlike molecules. The variations of ΔGE* with xDMC for four binary mixtures at 293.15 K are presented in Fig. 5. The ΔGE* has been found to be positive for four tested systems under investigation over the entire range of mole fractions of DMC. The order of positive (ΔGE*) values is as follows: (DMC + ethyl laurate) > (DMC + methyl laurate) > (DMC + ethyl decanoate) > (DMC + methyl decanoate). ΔGE* indicates the degree of intermolecular interaction and the transport behavior of the mixture [2]. Volumetric and viscometric effects both affect the values of ΔGE*. Positive deviations of ΔGE* are the result of specific interactions between unlike molecules [46, 24].
Excess Gibbs energies of activation vs. composition of DMC for the binary systems at 293.15 K: Filled Square, DMC + methyl decanoate; Filled Circle, DMC + ethyl decanoate; Filled Triangle, DMC + methyl laurate; Filled Down-Pointing Triangle, DMC + ethyl laurate; Solid Line, Redlich-Kister equation correlat ions
The experimental nD values are analyzed using the theoretical methods. The Dale-Gladstone equation (Eq. 7) Arago-Biot equation (Eq. 8) and Lorentz-Lorenz equation (Eq. 9) were used to predicting the refractive index for binary systems.
where nD the refractive index of the mixture, φi the volume fractions of component i, and nDi is the refractive index of pure component i, respectively. The solid line in Fig. 6 is the results correlated from Lorentz-Lorenz equation. The standard deviations of values of three theoretical methods for binary systems are listed at Table 6.
Variation of nD for the binary systems: A, DMC + methyl decanoate, B, DMC + ethyl decanoate, C, DMC + methyl laurate, D, DMC + ethyl laurate: Filled Square, 293.15 K; Filled Circle, 303.15 K; Filled Triangle, 313.15 K; Filled Down-Pointing Triangle, 323.15 K; − the correlated results of Lorentz-Lorenz equation
4 Conclusions
New experimental data for densities, viscosities and refractive indices of binary systems of (DMC + methyl decanoate), (DMC + ethyl decanoate), (DMC + methyl laurate) and (DMC + ethyl laurate) have been determined over the temperatures ranging from 293.15 to 323.15 K at atmospheric pressure. The densities, viscosities and refractive indices of the binary mixture all decrease with the increase of temperature. The excess molar volume, viscosity deviations of the studied systems have been calculated based on the experimental data of density and dynamic viscosity and compared. The calculated excess properties were also fitted to the Redlich–Kister equation. The excess mole volumes are positive within the measured range, and the viscosity deviations are negative. The excess Gibbs energy of activation was also calculated that presented positive values. Investigating the thermophysical and excess properties of fatty acid methyl and ethyl esters and their mixtures will assist in researching the surrogate mixtures of biodiesels using as a model system to investigate the combustion characteristic of fuels.
Data Availability
All data generated or analysed during this study are included in this published article.
References
Alptekin, E., Canakci, M.: Characterization of the key fuel properties of methyl ester – diesel fuel blends. Fuel. 88, 75–80 (2009)
Sharma, C., Bakshi, A., Syal, U., Sharma, M.: Excess thermodynamic and transport investigations for the binary mixtures of 1,2,3,4-tetrahydronaphthalene with fatty acid ethyl esters as potential biodiesel fuels. J. Chem. Eng. Data 67(12), 3622–3636 (2022)
Liu, H., Ma, J., Dong, F., Yang, Y., Liu, X., Ma, G., Zheng, Z., Yao, M.: Experimental investigation of the effects of diesel fuel properties on combustion and emissions on a multi-cylinder heavy-duty diesel engine. Energ. Convers. Manag. 171, 1787–1800 (2018)
Demirbas, A.: Biodiesel production via non-catalytic SCF method and biodiesel fuel characteristics. Energ. Convers. Manag. 47, 2271–2282 (2006)
Saxena, P., Jawale, S., Joshipura, M.H.A.: Review on prediction of properties of biodiesel and blends of biodiesel. Proc. Eng. 51, 395–402 (2013)
Ramirez-Verduzco, L.F., Garcia-Flores, B.E., Rodriguez-Rodriguez, J.E., Jaramillo-Jacob, A.R.: Prediction of the density and viscosity in biodiesel blends at various temperatures. Fuel. 90, 1751–1761 (2011)
Ramachandran, E., Krishnaiah, R., Venkatesan, E.P., Murugan, M., Medapati, S.R., Seka, P.: Experimental investigation for determining an ideal algal biodiesel–diesel blend to improve the performance and mitigate emissions using a response surface methodology. ACS Omega 8, 9187–9197 (2023)
Datta, A., Hossain, A., Roy, S.: An overview on biofuels and their advantages and disadvantages. Asian J. Chem. 31, 1851–1858 (2019)
Wakil, M.A., Kalam, M.A., Masjuki, H.H., Atabani, A.E., Rizwanul Fattah, I.M.: Influence of biodiesel blending on physicochemical properties and importance of mathematical model for predicting the properties of biodiesel blend. Energ. Convers. Manage. 94, 51–67 (2015)
Silitonga, A.S., Masjuki, H.H., Mahlia, T.M.I., Ong, H.C., Chong, W.T., Boosroh, M.H.: Overview properties of biodiesel diesel blends from edible and non-edible feedstock. Renew. Sustain. Energy Rev. 22, 346–360 (2013)
Chen, H., Su, X., He, J.: Investigation on combustion and emission characteristics of a common rail Diesel engine fueled with diesel/n-pentanol/methanol blends. Energy. 167, 297–311 (2019)
Guo, W., Yin, Y., Pi, N., Liu, F., Tu, S., Ye, L.: Investigation on a novel mixed dialkyl oxalate system as an oxygenated fuel additive. Energy Fuels 34, 4213–4220 (2020)
Rounce, P., Tsolakis, A., Leung, P., York, A.P.E.: A comparison of diesel and biodiesel emissions using dimethyl carbonate as an oxygenated additive. Energy Fuels 24, 4812–4819 (2010)
Razzaq, L., Mujtaba, M.A., Shahbaz, M.A., Nawaz, S., Khan, H.M., Hussain, A., Ishtiaq, U., Kalam, M.A., Soudagar, M.E.M., Ismail, K.A., Elfasakhany, A., Rizwan, H.M.: Effect of biodiesel-dimethyl carbonate blends on engine performance, combustion and emission characteristics. Alex Eng. J. 61, 5111–5121 (2022)
SenthilKumar, S., Rajan, K.: Performance and emission characteristics of diesel engine using biodiesel with the effect of dimethyl carbonate (DMC) fumigation. Energ. Source Part. A. 44, 2, 2986–2998 (2022)
Pan, M., Qian, W., Zheng, Z., Huang, R., Zhou, X., Huang, H., Li, M.: The potential of dimethyl carbonate (DMC) as an alternative fuel for compression ignition engines with different EGR rates. Fuel. 257, 115920 (2019)
Devarajan, Y.: Experimental evaluation of combustion, emission and performance of research Diesel engine fuelled di-methyl- carbonate and biodiesel blends. Atmos. Pollut. Res. 10, 795–801 (2019)
Ren, Y., Wang, H.: Densities, surface tensions, and viscosities of diesel–oxygenate mixtures at the temperature 301.15 K. Energy Fuels 21, 1628–1630 (2007)
Liu, X., Xue, S., Ikram, R., Zhu, C., Shi, Y., He, M.: Improving the viscosity and density of n-butanol as alternative to gasoline by blending with dimethyl carbonate. Fuel. 286, 119360 (2021)
Huber, M.L., Perkins, R.A., Laesecke, A., Friend, D.G., Sengers, J.V., Assael, M.J., Metaxa, I.N., Vogel, E., Mareš, R., Miyagawa, K.: New international formulation for the viscosity of H2O. J. Phys. Chem. Ref. Data. 38, 101–125 (2009)
Romano, E., Trenzado, J.L., González, E., Matos, J.S., Segade, L., Jiménez, E.: Thermophysical properties of four binary dimethyl carbonate + 1-alcohol systems at 288.15–313.15 K. Fluid. Phase. Equilib. 211, 219–240 (2003)
Zhou, J., Zhu, R., Xu, H., Tian, Y.: Densities, excess molar volumes, isothermal compressibilities, and isobaric expansivities of dimethyl carbonate + cyclohexane systems at temperatures from (293.15 to 313.15) K and pressures from (0.1 to 40) MPa. J. Chem. Eng. Data 55, 5569–5575 (2010)
Mou, L., Chai, Y., Yang, G., Xia, Q., Liu, Q., Zheng, Q., Zhang, Q.: Density and viscosity of four binary mixtures of [C2mmim][NTf2]/[C4mmim][NTf2] + dimethyl carbonate/diethyl carbonate. J. Chem. Thermodyn. 130, 183–191 (2019)
Chen, F., Yang, Z., Chen, Z., Hu, J., Chen, C., Cai, J.: Density, viscosity, speed of sound, excess property and bulk modulus of binary mixtures of γ-butyrolactone with acetonitrile, dimethyl carbonate, and tetrahydrofuran at temperatures (293.15 to 333.15) K. J. Mol. Liq. 209, 683–692 (2015)
Comelli, F., Bigi, A., Vitalini, D., Rubin, K.: Densities, viscosities, refractive indices, and heat capacities of poly(ethylene glycol-ran-propylene glycol) + esters of carbonic acid at (293.15 and 313.15) K and at atmospheric pressure. J. Chem. Eng. Data 55, 205–210 (2010)
Rodríguez, A., Canosa, J., Tojo, J.: Density, refractive index, and speed of sound of binary mixtures (diethyl carbonate + alcohols) at several temperatures. J. Chem. Eng. Data. 46, 1476–1486 (2001)
Gayol, A., Casás, L.M., Martini, R.E., Andreatta, A.E., Legido, J.L.: Volumetric properties of (dialkyl carbonate + n-alkane) mixtures at high pressures: Experimental measurement and Nitta–Chao model prediction. J. Chem. Thermodyn. 58, 245–253 (2013)
Yang, C., Xu, W., Ma, P.: Excess molar volumes and viscosities of binary mixtures of dimethyl carbonate with chlorobenzene, hexane, and heptane from (293.15 to 353.15) K and at atmospheric pressure. J. Chem. Eng. Data 49, 1802–1808 (2004)
You, S., Jeong, I., Hwang, I., Park, S.J.: The solid–liquid equilibrium, excess molar volume and refractive deviation properties of binary systems containing dimethyl carbonate, anisole and phenol. Fluid. Phase. Equilib. 383, 21–26 (2014)
Pratas, M.J., Freitas, S., Oliveira, M.B., Monteiro, S.C., Lima, A.S., Coutinho, J.A.P.: Densities and viscosities of fatty acid methyl and ethyl esters. J. Chem. Eng. Data 55, 3983–3990 (2010)
Ndiaye, E.H.I., Nasri, D., Daridon, J.L.: Speed of sound, density, and derivative properties of fatty acid methyl and ethyl esters under high pressure: Methyl caprate and ethyl caprate. J. Chem. Eng. Data. 57, 2667–2676 (2012)
Wang, X., Wang, X., Chen, J.: Experimental investigations of density and dynamic viscosity of n-hexadecane with three fatty acid methyl esters. Fuel. 166, 553–559 (2016)
Althouse, P.M., Hunter, G.W., Triebold, H.O.: Refractive indices of the methyl, propyl, and isopropyl esters of the C6–C18 saturated fatty acids for various temperatures between 20 and 45 °C. J. Am. Oil Chem. Soc. 24, 257–259 (1947)
Dzida, M., Jezak, S., Sumara, J., Zarska, M., Goralski, P.: High-pressure physicochemical properties of ethyl caprylate and ethyl caprate. J. Chem. Eng. Data 58, 1955–1962 (2013)
Habrioux, M., Bazile, J.P., Galliero, G., Daridon, J.L.: Viscosities of fatty acid methyl and ethyl esters under high pressure: methyl caprate and ethyl caprate. J. Chem. Eng. Data 60, 902–908 (2015)
Shigley, J.W., Bonhorst, C.W., Liang, C.C., Althouse, P.M., Triebold, H.O.: Physical characterization of a) a series of ethyl esters and b) a series of ethanoate esters. J. Am. Oil Chem. Soc. 32, 213–215 (1955)
Zhao, G., Yuan, Z., Yin, J., Ma, S.: Thermophysical properties of fatty acid methyl and ethyl esters. J. Chem. Thermodyn. 134, 195–212 (2019)
Pratas, M.J., Oliveira, M.B., Pastoriza-Gallego, M.J., Queimada, A.J., Pineiro, M.M., Coutinho, J.A.P.: High-pressure Biodiesel Density: Experimental measurements, correlation, and cubic-plus-association equation of state (CPA EoS) modeling. Energy Fuels. 25, 3806–3814 (2011)
Aissa, M.A., Ivanis, G.R., Radovic, I.R., Kijevcanin, M.L.: Experimental investigation and modeling of thermophysical properties of pure methyl and ethyl esters at high pressures. Energy Fuels 31, 7110–7122 (2017)
Liew, K.Y., Seng, C.E., Oh, L.L.: Viscosities and densities of the methyl esters of some n-Alkanoic acids. J. Am. Oil Chem. Soc. 69, 155–158 (1992)
Gouw, T.H., Vlugter, J.C.: Physical properties of fatty acid methyl esters. II. Refractive index and molar refraction. J. Am. Oil Chem. Soc. 41, 426–429 (1964)
Liau, W., Tang, M., Chen, Y.P.: Densities and viscosities of butyl acrylate + 1-butanol and ethyl laurate + 1-butanol at 293.15, 303.15, and 313.15 K. J. Chem. Eng. Data. 43, 826–829 (1998)
Wang, X., Kang, K., Lang, H.: High-pressure liquid densities and derived thermodynamic properties for methyl laurate and ethyl laurate. J. Chem. Thermodyn. 103, 310–315 (2016)
Lopez, E.R., Lugo, L., Comunas, M.J.P., García, J., Fernández, J.: Temperature dependence of the excess molar volume of (dimethyl carbonate, or diethyl carbonate + toluene) from T = 278.15 K to 323.15 K. J. Chem. Thermodyn. 32, 743–754 (2000)
Yue, L., Qin, X., Wu, X., Xu, L., Guo, Y., Fang, W.: Excess molar volume along with viscosity, refractive index and relative permittivity for binary mixtures of exo-tetrahydrodicyclopentadiene with four octane isomers. J. Chem. Thermodyn. 81, 26–33 (2015)
Kumar, S., Jeevanandham, P.: Densities, viscosities, refractive indices and excess properties of aniline and o-anisidine with 2-alkoxyethanols at 303.15 K. J. Mol. Liq. 174, 34–41 (2012)
Author information
Authors and Affiliations
Contributions
XG and DL wrote and revised the main manuscript text. DL prepared Figs. 1, 2, 3, 4, 5 and 6. MZ and BH investigated and collected the data. All authors reviewed the manuscript.
Corresponding author
Ethics declarations
Competing Interests
The authors declare no competing interests.
Additional information
Publisher’s Note
Springer Nature remains neutral with regard to jurisdictional claims in published maps and institutional affiliations.
Thermophysical properties of blended biodiesel are important to design transfer heat and mass equipment and to interpret the kind of interactions that occur in the studied blends.
Supplementary Information
Below is the link to the electronic supplementary material.
Rights and permissions
Springer Nature or its licensor (e.g. a society or other partner) holds exclusive rights to this article under a publishing agreement with the author(s) or other rightsholder(s); author self-archiving of the accepted manuscript version of this article is solely governed by the terms of such publishing agreement and applicable law.
About this article
Cite this article
Gao, X., Hong, B., Zhang, M. et al. Investigations on the Thermophysical Properties of Binary Systems of Fatty Acid Esters + Dimethyl Carbonate. J Solution Chem 53, 257–277 (2024). https://doi.org/10.1007/s10953-023-01327-7
Received:
Accepted:
Published:
Issue Date:
DOI: https://doi.org/10.1007/s10953-023-01327-7