Abstract
The present study made an attempt to measure the cortisol content, as an indicator of stress response, in rainbow trout embryos which were exposed to different densities and handling stress (air exposure) during incubation. The three densities of experimental embryos at early development stages were considered as 2.55 embryos/cm2 (low density), 5.10 embryos/cm2 (normal density) and 7.65 embryos/cm2 (high density). The cortisol content of eggs (5.09 ± 0.12 ng/g) decreased to 3.68 ± 0.14 ng/g in newly fertilized eggs. Resting level of cortisol dropped at three densities by day 18 of post fertilization. Then, cortisol increased at hatching stage to 1.16 ± 0.11, 1.20 ± 0.12 and 1.21 ± 0.14 ng/g at low, normal and high densities, respectively. There were no statistically significant differences between cortisol concentrations in three densities. The acute handling stress test (5-min out-of-water), conducted on embryos (48 h post fertilization, organogenesis and eyed stage) in three densities, revealed no differences in whole-body cortisol levels between stressed and unstressed experimental groups. At hatching stage in low-density group, level of cortisol increased but the difference with the pre-stress levels was not statistically significant. Furthermore, significant differences in cortisol levels of stressed and unstressed embryos were detected on hatching in normal and high density groups [1.20 ± 0.12 at time 0–1.49 ± 0.11 ng/g at 1 hps (hours post stress) and from 1.21 ± 0.14 at time 0 to 1.53 ± 0.10 ng/g at 3 hps, respectively]. The results showed no difference in profile of cortisol in different densities, but acute stress conducted on embryos, incubated in different densities, revealed differences in cortisol stress response at hatching between normal and high density, which lead to cortisol increase at hatching time. It indicates that the lag time in the cortisol response to stressors immediately after hatching does not occur when the siblings were stressed during the embryo stage. Results, finally, indicated that hypothalamus–pituitary–interrenal axis was active and responded to an acute stressor under normal and high density, but it is unresponsive to a stressor around hatching under low density.
Similar content being viewed by others
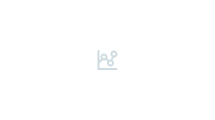
Avoid common mistakes on your manuscript.
Introduction
Changes in oocyte quality can affect the embryos after fertilization (Bromage et al. 1992). Many factors such as nutrition, genetic, stress, health and water temperature in breeders affect egg quality and survival (Schreck et al. 2001). High mortalities of embryos produced under intensive hatchery conditions are often caused by various husbandry acute (e.g., handling and disease) and chronic (e.g., poor water quality, confinement, overcrowding) stressors during incubation period in large-scale hatcheries. These stressors may cause physiological alterations in fishes, known as stress responses. One of the primary responses of fish is release of hormones (cortisol) to blood circulation from interrenal cells (Wendelaar Bonga 1997).
Cortisol is commonly used as an indicator of stress in fish (Barton and Iwama 1991; Wendelaar Bonga 1997; Barton 2002). Elevation of cortisol is one of the most primary responses mediated by the hypothalamus–pituitary–interrenal (HPI) axis (Iwama et al. 2005). This hormone is implicated in reproduction (Milla et al. 2009), metabolic action (Poursaeid et al. 2012), osmoregulation, adaptation to seawater (Mommsen et al. 1999), adaptation to freshwater (McCormick 2001; Kiilerich et al. 2007), stress adaptation (Wendelaar Bonga 1997; Mommsen et al. 1999) and chronic stress with increasing energetic demand that inhibit growth (Pankhurst and Van Der Kraak 1997; Jentoft et al. 2005). Stress caused reduction in female growth (Mileva et al. 2011), food intake (Bernier and Peter 2001; Øverli et al. 2006) and high levels of cortisol in broodstock, decrease body size, gonadosomatic index, egg size and quality (Kime and Nash 1999; Pourhosein Sarameh et al. 2012).
The stress response is variable with regard to species (Falahatkar et al. 2012), life stage (Barton 2002), type of stressor (Wendelaar Bonga 1997) and strain (McGeer et al. 1991; Pottinger et al. 1992). Significant amounts of cortisol are in vitellogenic eggs, freshly ovulated eggs, newly fertilized eggs, embryo and larvae (Tagawa et al. 1990; De Jesus and Hirano 1992; Hwang et al. 1992; Sampath-Kumar et al. 1995; Feist and Schreck 2002; Jentoft et al. 2002; Deane and Woo 2003; Raine and Leatherland 2003; Szisch et al. 2005; Simontacchi et al. 2008; Applebaum et al. 2010; Falahatkar et al. 2013). Also, cortisol levels in embryos and alevins of rainbow trout have been reported (Pottinger and Mosuwe 1994; Barry et al. 1995a, b; Auperin and Geslin 2008).
This level of cortisol is species specific and related to steroid levels of the brood fish. Because of insufficient blood, whole-body cortisol levels have been used to assess the stress response of developing embryo and larvae (De Jesus et al. 1991; De Jesus and Hirano 1992; Pottinger and Mosuwe 1994; Barry et al. 1995a, b; Feist and Schreck 2002) and also adult of smaller fish such as zebra fish, Danio rerio (Ramsay et al. 2006). The content of cortisol in embryo and larvae should be measured in different culture conditions because its level affects larval quality. Knowledge about the timing of the onset of cortisol stress response is important for developing a successful fish culture, because a more rapid rate of development, affected by variances in species, strain, environmental conditions, and acute and chronic stress, leads to earlier occurrence of stress response capabilities (Barton 2002).
While there have been several reports about cortisol levels following exposure of embryonic and larval stages to an acute stressor (De Jesus et al. 1991; De Jesus and Hirano 1992; Hwang et al. 1992; Pottinger and Mosuwe 1994; Sampath-Kumar et al. 1995; Stratholt et al. 1997, 1998; Jentoft et al. 2002; Falahatkar et al. 2013), almost no information existed on the effect of chronic stress and especially on the interaction between chronic and acute stress on fish embryos and alevins.
Therefore, the main objective of this study was to determine the ontogeny of development of the cortisol-mediated stress response in rainbow trout subjected to various levels of handling and density stressors during early developmental stages in the hatchery.
Materials and methods
Eggs and incubation
Rainbow trout breeders were hatchery produced from the Coldwater Fish Genetic and Breeding Research Center of the Shahid Motahari, Yasouj, Iran, in December 2008. Twenty females and forty males (autumn spawners; 3–5 years of age) were selected according to the ripeness characteristics. Breeders were not fed for 48 h prior to reproduction and anesthetized with clove powder extract (150–200 mg/l). A single mass cross was used, and ova from all females were stripped by application of abdominal pressure. Then, similarly, following dry artificial fertilization technique, sperms from all the male breeders were added to the eggs. Therefore, the sex ratio was 1:2 in fertilization process. After washing the eggs in clean hatchery water, they were maintained in water for 30–45 min until their volume increased. After 5 hours post fertilization (hpf), sufficient numbers of embryos were randomly collected and distributed in laboratory incubator units.
Experimental protocols
Experiment 1: stocking densities (chronic stress)
The embryos were incubated in three densities; 500 (2.55 embryos/cm2; low density), 1,000 (5.10 embryos/cm2; normal density) and 1,500 (7.65 embryos/cm2; high density) embryos/incubator. The usual density in rainbow trout hatcheries (5.10 embryos/cm2) was applied as a normal density in this research. Three small California-type incubators (tray size: 14 × 14 × 14 cm) supplied with spring water at a constant flow rate of 8 l/min were used, each for one treatment. Each incubator contained three trays in each trough, and water passed from one tray to the next one horizontally. At last, embryos were randomly divided into trays, and trays were randomly distributed into troughs. Water parameters were maintained and measured during the incubation period (temperature 10.6 ± 0.1 °C; dissolved oxygen 7.9 ± 0.1 mg/l and pH 8.0 ± 0.1).
Experiment 2: air exposure (acute stress)
In order to assess the impact of air exposure on embryos, five experimental groups were selected, each one containing three densities and each density containing three replicates. One group was not stressed and used as a control (CT) (previous group in experiment 1), and the remaining four groups were exposed to an acute stress of 5 min air exposure. Among these four groups, the first one was exposed to acute stress at 48 hpf, the second one at organogenesis stage (10 dpf), the third one at eyed stage (18 dpf) and finally, the last group at hatching time (31 dpf). The embryos and alevins in experiment 2 were subjected to air exposure stress by reducing water level of the experimental incubators slowly to 80 percent, then embryos and alevins remained in the trays, and the trays were removed from the water for 5 min.
Sampling
A pool of 10 eggs or embryos from each replicate was collected at 5 h after fertilization, organogenesis, eyed and hatching stages. To assess the cortisol changes after the acute stress, embryos from each of three replicates (6–7 embryos/replicates) were sampled at 0, 1, 3, 6 and 24 h post stress time. Eggs and embryos were immediately frozen in liquid nitrogen and stored at −20 °C until analysis (Auperin and Geslin 2008).
Cortisol extraction
Before extraction, eggs and embryos were weighed and then were crushed and sonicated (virsonic ultrasonic cell disrupter, UP200S, Hielscher Ultrasonics, Teltow, Germany) in 500 µl PBSG (phosphate-buffered saline containing 0.1 % gelatin, pH 7.2). Samples of alevins were also homogenized in 500 µl PBSG for 1 min using an Ultra Turrax T18 homogenizer (IKA, Wilmington, USA). Recovery of steroids from samples was carried out by addition of 3H–cortisol to homogenized tissue. Eggs and embryos were extracted according to the method of Hwang and Wu (1993). Briefly, the samples were extracted twice with 5 volume of diethyl ether. After diethyl ether evaporation, extracted steroids were suspended in 500 µl PBSG for radioimmunoassay (RIA) of cortisol.
RIA
Cortisol was measured in duplicate, using RIA kit (Immunotech, Prague, Czech Republic). The RIA is a competition assay between cortisol of the samples and 125I–labeled cortisol for binding to an anti-cortisol monoclonal antibody coated onto the tubes. After 1-h incubation, the liquid contents of the tube were aspirated and bound radioactivity was measured by gamma counter (LKB, Mount Waverley, Australia). A calibration curve was established and unknown values were determined by interpolation from the standard curve. The calibrator vials were from 0 to 720 ng/g of cortisol in buffer with bovine serum albumin and sodium azide. Inter-assay and intra-assay were 8.2 and 9.7 %, respectively. One vial of cortisol included <0.1 sodium azide, and 0.1 ng/ml cortisol was used as negative control. The cross reactivity of cortisol antibody with 44 steroids was: 11-deoxycortisol 18 %, corticosterone 8.4 %, 21-deoxycortisol 7.5 %, deoxycorticosterone 7.3 %, prednisolone 6 %, 17α-hydroxyprogesterone 3.5 %, dihydrocortisol 2.4 %, 5α-dihydrocortisone 2.3 %, progesterone 1.8 %; cortisone 1.5 %, pregnenolone 1.1 %, and allotetrahydrocortisone 0.8 % and with most of the other 32 steroids was <0.1 %. Percent recovery average was 89 %.
Statistical analysis
Results were expressed as mean ± standard error of the mean (SE). Before analysis, the data were checked for normality and variances for homogeneity by Kolmogorov–Smirnov and Levene’s tests, respectively. After that, differences between cortisol concentrations at various densities and different developmental stages were analyzed by one-way ANOVA. Then, Tukey’s post hoc test was applied to identify treatments with significant differences (P < 0.05). The effects of density and developmental stage on cortisol content were analyzed by two-way ANOVA. A three-way ANOVA was used to test the effects of density, developmental stage and times post stress on cortisol levels. All procedures were applied by SPSS software (SPSS 14, SPSS, Chicago, IL).
Results
Cortisol concentrations in rainbow trout eggs, embryos at 5 h after fertilization, eyed- and hatching stages are shown in Fig. 1. In eggs, cortisol content was 5.09 ± 0.12 ng/g, which dropped significantly 5 h after fertilization to 3.68 ± 0.14 ng/g. Cortisol level decreased at eyed stage, then increased significantly at the time of hatching (31 dpf). There were no significant differences between cortisol profiles of experimental eggs and embryos between three densities until hatching.
Cortisol concentrations (ng g−1) during developmental stages in rainbow trout embryo at three densities. Each bar represents the mean value of three replicates (±SE). Different letters show the significant difference among different time points. hpf Hours post fertilization, dpf days post fertilization
Exposure of the embryos to an acute stressor (5 min out-of-water) had no effect (1, 3, 6 and 24 h after stressor exposure) on whole-body cortisol concentrations in three stages of 48 hpf, organogenesis and eyed stages (Figs. 2, 3, 4). The interaction between time and stress in all three stages was not significant. At the three mentioned stages, the pattern of changes of cortisol concentration in response to an acute stressor was almost similar in the three experimental densities. Significantly higher whole-body cortisol concentrations between stressed and control groups were detected at hatching time, and the stress effect in this time showed dependence to density (31 dpf; Fig. 5A–C).
Cortisol concentrations (ng g−1) in stressed and non-stressed (control) rainbow trout embryos in hatching stage under low density (A), normal density (B), high density (C). Each bar represents the mean value of three replicates (±SE). An asterisk shows the significant difference between stress and control group at each time point (P < 0.05). The cross show the significant difference between 0 and 24 hps in both groups, the control and the stressed (P < 0.05)
In response to an acute stressor, hatched fish in low density had an increase in cortisol 1 h after exposure to an acute stressor which showed no significant difference with control (P > 0.05; Fig. 5A).
In response to an acute stressor, fish at hatching stage in normal density had a significant (P < 0.05) increase in cortisol levels from 1.20 ± 0.12 at resting time to 1.49 ± 0.11 ng/g at 1 hps. Cortisol level was significantly higher at 24 hps than 0, 3 and 6 hps (Fig. 5B).
An insignificant elevation (P > 0.05) in cortisol concentration in newly hatched rainbow trout alevins under high density that were exposed to an acute stressor, within 1 hps was observed. But in 3 hps, the cortisol levels were significantly (P < 0.05) higher than the level measured at time 0 and 1 hps. In 3 hps, the cortisol level in high density group at the hatching stage was still significantly higher (P < 0.05) than the cortisol level at time 0 hps. At 6 hps, it returned to resting level. Cortisol level had significant difference with 0, 1 and 6 hps under high density (Fig. 5C).
The interaction effects of density and developmental stages on cortisol levels were significant (P < 0.05), but interaction effects of density, developmental stages and times post stress on cortisol content were not significant (P > 0.05).
Discussion
In the present study, the effect of acute stress on cortisol concentrations in rainbow trout embryos at four important stages and different densities was examined. Cortisol content in eggs quickly decreased after fertilization. Similar decrease in cortisol concentration was recorded in white sturgeon Acipenser transmontanus by Simontacchi et al. (2008). This decrease could be due to free diffusion of cortisol through the egg chorion, before it has hardened (Auperin and Geslin 2008). The cortisol level in eggs of white sturgeon is almost 4 times higher than eggs in our study, which could be related to the stress condition of the mother and species differences.
Cortisol concentration decreased from fertilization until eyed stage, which is reported to be due to metabolization of the maternal cortisol in the embryos (De Jesus et al. 1991; Hwang et al. 1992; Barry et al. 1995b; Sampath-Kumar et al. 1995, 1997; Stratholt et al. 1997; Jentoft et al. 2002; Auperin and Geslin 2008). Cortisol concentrations increased between eyed and hatching stages (31 days post fertilization) which suggest endogenous production before hatching, as reported by other researchers (De Jesus and Hirano 1992; Stouthart et al. 1998; Feist and Schreck 2002; Jentoft et al. 2002). Auperin and Geslin (2008) also reported that cortisol was produced at the eyed stage. Usage of maternal cortisol by embryos occurred from fertilization until the interrenal cells were functional (Stouthart et al. 1998).
Experimental rainbow trout embryos incubated at three densities did not show a rise in cortisol in response to an acute stressor until hatching. It could be possible that the adrenocorticotrophs and interrenal cells did not fully mature at 48 h after fertilization, organogenesis stage and eyed stage in the three densities. Auperin and Geslin (2008) suggested that the interrenal cells matured at the eyed stage, but they could not determine whether the lack of response to stressor before hatching was due to brain immaturity or pituitary immaturity.
Whereas all the other published studies on Salmonids showed first cortisol response at least 5 days after hatching and in rainbow trout at least 9 days later (Barry et al. 1995a, b; Feist and Schreck 2002; Auperin and Geslin 2008), our results showed higher cortisol immediately after hatching. Lack of cortisol stress response may be related to undeveloped HPI axis in those previous experiments. Also these differences could be due to species specificity, rearing conditions and types of stressors (Feist and Schreck 2002). Cortisol biosynthesis and HPI axis development were affected by different stressors. Barry et al. (1995a, b) and Auperin and Geslin (2008) employed 0 °C exposure. This cold shock may have decline the rate of enzyme systems for cortisol biosynthesis, may have changed cortisol content (Feist and Schreck 2002) or may suppressed development of HPI axis at cold shock time.
Cortisol level in the present study on rainbow trout at the first stress response, under normal density (1.49 ± 0.11 ng/g, Fig. 5B), was 7.4 times smaller than rainbow trout (Barry et al. 1995b), 2.4 smaller than Chinook salmon Oncorhynchus tshawytscha (Feist and Schreck 2002), and 8.3 smaller than yellow perch Perca flavescens (Jentoft et al. 2002). These differences may be related to different breeders since cortisol in larvae from breeders with high stress response is secreted more than in larvae from breeders with low stress response (Pottinger et al. 1994).
Cortisol did not increase in low-density treatment after an acute stress at hatching, indicated that the HPI axis is unresponsive to a stressor (Fig. 5A), while it increased in normal and high densities after exposure to an acute stressor (Fig. 5B, C). Cortisol stress response in higher densities may be related to either more active HPI axis or higher number of interrenal cells.
A significant result is that the cortisol content at 24 hps in the control and stressed fish in normal and high density were much higher than resting levels (Fig. 5B, C). This may suggest endogenous production after hatching, as previously demonstrated for cortisol in rainbow trout at 1–3 hps (Hwang et al. 1992), which may be due to an increase in the number of interrenal cells in the head kidney. The prolonged elevation for at least 3 h in high density group may be due to the effect of chronic stress in embryonic development on HPI axis.
Cortisol was measured at all stages of development at different densities tested in the present study indicating that cortisol level in rainbow trout is similar to the range of many other teleost species (De Jesus et al. 1991; Hwang et al. 1992; Barry et al. 1995a, b; Sampath-Kumar et al. 1995; Stratholt et al. 1997; Jentoft et al. 2002; Auperin and Geslin 2008; Simontacchi et al. 2008; Applebaum et al. 2010). Cortisol production in response to an acute stressor was around the time of hatching, and the absence of stress effect during the embryonic stage in low density causes a lag time in the stress response. Higher densities responded to acute stress earlier than that of the other treatments which it could be related to earlier development of HPI axis. It is not clear which part of HPI axis is affected by chronic stress: hypothalamus, pituitary or interrenal cells.
In conclusion, these differences in cortisol stress response in three densities indicate that the chronic stress along with acute stress has an effect on the HPI axis of rainbow trout. The HPI axis is unresponsive to a stressor around hatching at low density. However, the HPI axis is responsive to a stressor at hatching in normal and high densities for rainbow trout. The effect of early HPI axis activity on fish is not clear. More research and studies are required to investigate the role of maternal cortisol on the oocyte, embryo and larvae of fish and to determine the time of first cortisol stress response. The demonstration of gene expression, cortisol receptor binding and immunohistochemical localization of corticotrophs for the full developmental series would be made for future works.
References
Applebaum SL, Wilson CA, Holt GJ, Nunez BS (2010) The onset of cortisol synthesis and the stress response is independent of changes in CYP11B or CYP21 mRNA levels in larval red drum (Sciaenops ocellatus). Gen Comp Endocrinol 165:269–276
Auperin B, Geslin M (2008) Plasma cortisol response to stress in juvenile rainbow trout is influenced by their life history during early development and by egg cortisol content. Gen Comp Endocrinol 158:234–239
Barry TP, Ochiai M, Malison JA (1995a) In vitro effects of ACTH on interrenal corticosteroidogenesis during early larval development in rainbow trout. Gen Comp Endocrinol 99:382–387
Barry TP, Malison JA, Held JA, Parrish JJ (1995b) Ontogeny of the cortisol stress response in larval rainbow trout. Gen Comp Endocrinol 97:57–65
Barton BA (2002) Stress in fishes: a diversity of responses with particular reference to changes in circulating corticosteroids. Integr Comp Biol 42:517–525
Barton BA, Iwama GK (1991) Physiological changes in fish from stress in aquaculture with emphasis on the response and effects of corticosteroids. Annu Rev Fish Dis 1:3–26
Bernier NJ, Peter RE (2001) The hypothalamic–pituitary–interrenal axis and the control of food intake in teleost fish. Comp Biochem Physiol 129B:639–644
Bromage NR, Jones J, Randall C, Thrush M, Davies B, Springate J, Duston J, Barker G (1992) Broodstock management, fecundity, egg quality and the timing of egg production in the rainbow trout (Oncorhynchus mykiss). Aquaculture 100:141–166
De Jesus EG, Hirano T (1992) Changes in whole body concentrations of cortisol, thyroid hormones, and sex steroids during early development of the chum salmon, Oncorhynchus keta. Gen Comp Endocrinol 85:55–61
De Jesus EG, Hirano T, Inui Y (1991) Changes in cortisol and thyroid hormone concentrations during early development and metamorphosis in the Japanese flounder, Paralichthys olivaceus. Gen Comp Endocrinol 82:369–376
Deane EE, Woo NYS (2003) Ontogeny of thyroid hormones, cortisol, hsp70 and hsp90 during silver sea bream larval development. Life Sci 72:805–818
Falahatkar B, Akhavan SR, Efatpanah I, Meknatkhah B (2012) Primary and secondary responses of a teleostean, pikeperch Sander lucioperca, and a chondrostean, Persian sturgeon Acipenser persicus juveniles, to handling during transport. N Am J Aquac 74:241–250
Falahatkar B, Akhavan SR, Ghaedi GR (2013) Egg cortisol response to stress at early stages of development in Persian sturgeon Acipencer persicus. Aquac Int 21:215–223
Feist G, Schreck CB (2002) Ontogeny of the stress response in chinook salmon, Oncorhynchus tshawytscha. Fish Physiol Biochem 25:31–40
Hwang PP, Wu SM (1993) Role of cortisol in hypo-osmoregulation in larvae of tilapia (Oreochromis mossambicus). Gen Comp Endocrinol 92:318–324
Hwang PP, Wu SM, Lin JH, Wu LS (1992) Cortisol content of eggs and larvae of teleosts. Gen Comp Endocrinol 86:189–196
Iwama GK, Afonso LOB, Vijayan MM (2005) Stress in fish. In: Evans DH, Claiborne JB (eds) The physiology of fishes, 3rd edn. CRC Press, Boca Raton, FL, pp 319–342
Jentoft S, Held JA, Malison JA, Barry TP (2002) Ontogeny of the cortisol stress response in yellow perch (Perca flavescens). Fish Physiol Biochem 26:371–378
Jentoft S, Aastveit AH, Torjesen PA, Andersen Ø (2005) Effects of stress on growth, cortisol and glucose levels in non-domesticated Eurasian perch (Perca fluviatilis) and domesticated rainbow trout (Oncorhynchus mykiss). Comp Biochem Physiol 141A:353–358
Kiilerich P, Kristiansen K, Madsen SS (2007) Cortisol regulation of ion transporter mRNA in Atlantic salmon gill and the effect of salinity on the signaling pathway. J Endocrinol 194:417–427
Kime DE, Nash JP (1999) Gamete viability as an indicator of reproductive endocrine disruption in fish. Sci Total Environ 233:123–129
McCormick SD (2001) Endocrine control of osmoregulation in teleost fish. Am Zool 41:781–794
McGeer JC, Baranyi L, Iwama GK (1991) Physiological responses to challenge tests in six stocks of coho salmon (Oncorhynchus kisutch). Can J Fish Aquat Sci 48:1761–1771
Mileva VR, Gilmour KM, Balshine S (2011) Effects of maternal stress on egg characteristics in a cooperatively breeding fish. Comp Biochem Physiol 158A:22–29
Milla S, Wang N, Mandiki SNM, Kestemont P (2009) Corticosteroids: friends or foes of teleost fish reproduction? Comp Biochem Physiol 153A:242–251
Mommsen TP, Vijayan MM, Moon TW (1999) Cortisol in teleosts: dynamics, mechanisms of action, and metabolic regulation. Rev Fish Biol Fish 9:211–268
Øverli Ø, Sørensen C, Kiessling A, Pottinger TG, Gjøen HM (2006) Selection for improved stress tolerance in rainbow trout (Oncorhynchus mykiss) leads to reduced feed waste. Aquaculture 261:776–781
Pankhurst NW, Van Der Kraak G (1997) Effects of stress on reproduction and growth of fish. In: Iwama GK, Pickering AD, Sumpter JP, Schreck CB (eds) Fish stress and health in aquaculture. Cambridge University Press, Cambridge, pp 73–93
Pottinger TG, Mosuwe E (1994) The corticosteroidogenic response of brown and rainbow trout alevins and fry to environmental stress during a ‘critical period’. Gen Comp Endocrinol 95:350–362
Pottinger AD, Pickering TG, Hurley MA (1992) Consistency in the stress response of individuals of two strains of rainbow trout, Oncorhynchus mykiss. Aquaculture 103:275–289
Pottinger TG, Moran TA, Morgan JAW (1994) Primary and secondary indexes of stress in the progeny of rainbow trout (Oncorhynchus mykiss) selected for high and low responsiveness to stress. J Fish Biol 44:149–163
Pourhosein Sarameh S, Falahatkar B, Azari Takami G, Efatpanah I (2012) Effects of different photoperiods and handling stress on spawning and reproductive performance of pikeperch Sander lucioperca. Anim Reprod Sci 132:213–222
Poursaeid S, Falahatkar B, Mojazi Amiri B, Van Der Kraak G (2012) Effects of long-term cortisol treatments on gonadal development, sex steroids levels and ovarian cortisol content in cultured great sturgeon Huso huso. Comp Biochem Physiol 163A:111–119
Raine JC, Leatherland JF (2003) Trafficking of L-triiodothyronine between ovarian fluid and oocytes of rainbow trout (Oncorhynchus mykiss). Comp Biochem Physiol 136B:267–274
Ramsay JM, Feist GW, Varga ZM, Westerfield MM, Kent L, Schreck CB (2006) Whole-body cortisol is an indicator of crowding stress in adult zebrafish, Danio rerio. Aquaculture 258:565–574
Sampath-Kumar R, Byers RE, Munro AD, Lam TJ (1995) Profile of cortisol during ontogeny of the Asian seabass, Lates calcarifer. Aquaculture 132:349–359
Sampath-Kumar R, Lee ST, Tan CH, Munro AD, Lam TJ (1997) Biosynthesis in vivo and excretion of cortisol by fish larvae. J Exp Zool 277:337–344
Schreck CB, Contreras-Sanchez W, Fitzpatrick MS (2001) Effects of stress on fish reproduction, gamete quality, and progeny. Aquaculture 197:3–24
Simontacchi C, Negrato E, Pazzaglia M, Bertotto D, Poltronieri C, Radaelli G (2008) Whole-body concentrations of cortisol and sex steroids in white sturgeon (Acipenser transmontanus, Richardson 1836) during early development and stress response. Aquacult Int 17:7–14
Stouthart AJHX, Lucassen ECHET, van Strien FJC, Balm PHM, Lock RAC, Wendelaar Bonga SE (1998) Stress responsiveness of the pituitary-interrenal axis during early life stages of common carp (Cyprinus carpio). J Endocrinol 157:127–137
Stratholt ML, Donaldson EM, Liley NR (1997) Stress induced elevation of plasma cortisol in adult female coho salmon (Oncorhynchus kisutch), is reflected in egg cortisol content, but does not appear to affect early development. Aquaculture 158:141–153
Szisch V, Papandroulakis N, Fanouraki E, Pavlidis M (2005) Ontogeny of the thyroid hormones and cortisol in the gilthead sea bream, Sparus aurata. Gen Comp Endocrinol 142:186–192
Tagawa M, Tanaka M, Matsumoto S, Hirano T (1990) Thyroid hormones in eggs of various freshwater, marine and diadromous teleosts and their changes during egg development. Fish Physiol Biochem 8:515–520
Wendelaar Bonga SE (1997) The stress response in fish. Physiol Rev 77:591–625
Acknowledgments
This work was financially supported by a research grant of Khorramshahr University of Marine Science and Technology. We thank all the members of the Shahid Motahari Coldwater Fish Genetic and Breeding Research Center, Yasouj, Iran for supplying the experimental eggs and rearing facilities and also the microbiology laboratory, Faculty of Veterinary Medicine, University of Tehran for kind assistance in the cortisol measurement. Also special thank to G. Van Der Kraak for some comments on the manuscript.
Author information
Authors and Affiliations
Corresponding author
Rights and permissions
About this article
Cite this article
Ghaedi, G., Falahatkar, B., Yavari, V. et al. The onset of stress response in rainbow trout Oncorhynchus mykiss embryos subjected to density and handling. Fish Physiol Biochem 41, 485–493 (2015). https://doi.org/10.1007/s10695-014-9999-3
Received:
Accepted:
Published:
Issue Date:
DOI: https://doi.org/10.1007/s10695-014-9999-3