Abstract
Fourteen accessions (spring and winter types) and four testers (semi-winter type) of rapeseed (Brassica napus L.) were crossed in line × tester mating design to estimate general and specific combining ability for seed yield, yield components, and oil content. The F1 hybrids and their parents were evaluated in four environments in northern China. Highly significant differences were detected among the parents and hybrids for all the traits across environments. Plant height, setting position of first primary branch and length of terminal raceme were controlled by additive genes, whereas primary branches per plant, siliques on terminal raceme, siliques per plant, seeds per silique, seed yield per plant, 1000-seed weight, oil content, and seed yield were controlled by non-additive gene action. The accessions SP-Armada, 9E49, and CZ25 and the tester Zhong9 were good general combiners for seed yield. Among the 56 F1 hybrids, four hybrids: Zhong9 × CZ25, GZ1R × 9E38, Zhong7 × 9E38, and Zhong7 × CZ49 showed higher yield than the control and were the outstanding combinations for seed yield. These hybrids were recommended to be included in future breeding programs for development of new high yielding varieties with more desirable traits. Both winter and spring germplasm have potential in Chinese semi-winter rapeseed breeding program, for seed oil content improvement, more attention may be paid to spring germplasm.
Similar content being viewed by others
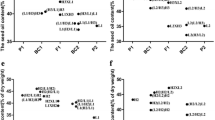
Avoid common mistakes on your manuscript.
Introduction
Rapeseed (Brassica napus L.; AACC, 2n = 38) is the most important edible oilseed crop in the world. B. napus is an amphidiploid originated through spontaneous interspecific hybridization between its two progenitors, B. rapa (AA, 2n = 20) and B. oleracea (CC, 2n = 18) (Allender and King 2010) and is believed to be originated in Southern Europe (Downey 1983). China, Canada, and European countries lead the world in rapeseed production (Li et al. 2012). Recently, the production of rapeseed has expanded remarkably in most of the major rapeseed producing countries (FAOSTAT 2015). In China, B. napus was first introduced in the mid 20th century from Europe and Japan (Sun 1948; Liu 1985). Chinese rapeseed quality improvement breeding program was initiated in late 1970s after the introduction of Oro, Tower and other single-low or double-low B. napus cultivars from Europe, Canada and Australia (Liu 2000). Using these canola varieties as donors, Chinese breeders have successively developed a number of new canola varieties adapted to local conditions. At present, B. napus has become the most important oilseed crop in China (Qian et al. 2006; Wang and Yin 2014) with annual production of 14.5 metric tonnes (FAOSTAT 2015).
Ectopically, rapeseed germplasm was classified into spring, winter, and semi-winter types (Diers and Osborn 1994; Becker et al. 1995). Hybridization between winter, semi-winter, and spring rapeseed is an important approach to broaden the genetic base of these three types of rapeseed (Qian et al. 2009; Kebede et al. 2010). European rapeseed (Hu et al. 2007), US rapeseed (Li et al. 2012) and Australian rapeseed (Chen et al. 2008) are different from Chinese rapeseed and could be used as important germplasm sources to enrich the genetic background of Chinese semi-winter rapeseed.
The phenomenon of heterosis describes that F1 hybrids derived from two genetically dissimilar genotypes exhibit superior phenotypic performance as compared with either parent, usually manifested in rapid growth, high fertility, superior biomass production, resistance to disease and insect pest, and high grain yield (Shull 1948, 1952; Birchler et al. 2010). Heterosis has been progressively applied in crop production for almost a century, with the aim of developing most vigorous, of superior quality and higher yield performing hybrid cultivars (Fu et al. 2014). The commercial use of heterosis has increased production of maize (Crow 1998) and rice (Denning and Mew 1997; Yuan 2014). Significant heterosis was documented for rapeseed within spring type, winter type (McVetty 1995; Brandle and McVetty 1990) and semi-winter type (Tian et al. 2015, 2017) rapeseed. High heterosis has also been reported in hybrids between Chinese semi-winter and winter rapeseed in winter conditions (Lefort-Buson et al. 1987; Qian et al. 2009) and between Chinese semi-winter and spring rapeseed in spring conditions (Udall et al. 2004; Qian et al. 2007). The winter and semi-winter rapeseed germplasm can be used for improvement of seed yield and other traits in spring rapeseed hybrids and open-pollinated cultivars (Butruille et al. 1999; Quijada et al. 2004, 2006; Udall et al. 2004, 2006; Kebede et al. 2010; Rahman 2011, 2013; Rahman and Kebede 2012).
Knowledge of various types of gene action helps in the identification and selection of suitable parents to be included in hybridization program to develop superior F1 hybrids. General combining ability (GCA) is the average performance of a line in hybrid combinations, whereas specific combining ability (SCA) is the deviation of a certain cross from the average performance of the lines (Sprague and Tatum 1942). Line × tester analysis can be used to decide the comparative ability of accessions and testers to produce desirable hybrid combinations (Kempthorne 1957). These crosses also provide information about the genetic components and enable the breeders to choose an appropriate breeding procedure for population improvement and cultivar development. Combining ability analysis is an important breeding method and gives knowledge regarding the desirable parents, magnitude and nature of gene action which control the inheritance of quantitative traits (Ceyhan et al. 2008). Accessions × tester analysis is used by many scientists to examine genetics of agronomic characters and judgment of GCA and SCA effects in different crops such as rapeseed (Rameeh 2011, 2012a; Farshadfar et al. 2013); sunflower (Khan et al. 2009); pea (Ceyhan et al. 2008); wheat (Saeed et al. 2001) and sorghum (Mohammed 2009) etc.
In the present study, 14 accessions (spring and winter types) and four testers (semi-winter type) of rapeseed (B. napus) were crossed. The F1 hybrids and their parents were evaluated for seed yield, yield components, and oil content in four different environments in northern China. The objectives of the present study were; (a) to evaluate the performance of winter and spring type exotic accessions and Chinese semi-winter type testers, (b) to investigate estimates of GCA and SCA effects of parental genotypes and their F1 hybrids respectively, for seed yield, its components and oil traits, (c) to identify potential hybrid combinations to be used in future breeding programs for the development of new desirable varieties and (d) to exploit the breeding value of spring and winter rapeseed in Chinese semi-winter rapeseed genetic improvement.
Materials and methods
Plant materials
A set of 18 genetically diverse and geographically distinct rapeseed (B. napus) genotypes was used in the present study (Table 1). Fourteen spring and winter type exotic rapeseed accessions were used as female parents (lines) and four indigenous Chinese semi-winter genotypes were used as male parents (testers). The parental accessions were selfed for at least six generations before being used in the crossing program according to accession × tester mating design (Kempthorne 1957). At the budding stage, immature buds in the inflorescence of the female and male parents were covered with a butter paper bag before flowering. Before blooming, the flowers of the female plants were manually emasculated and then pollinated in April 2010 and 2014, respectively. F1 seeds generated in 2009/2010 were used for evaluation in the field during cropping seasons 2010/2011 and 2011/2012, whereas those of 2013/2014 were used for evaluation in the field in 2014/2015. Qinyou No. 7, a widely cultivated hybrid in the region of Huang-Huai District and Lower reaches of Yangtze Rivers, China, was used as a control.
Field evaluation
The 18 parental genotypes (14 accessions and 4 testers) and their 56 F1 hybrids were arranged in a randomized complete block design with two replications in four different environments (defined as the combination of year and location) including three cropping seasons, 2010–2011 (108°04′E, 34°15′N; 442 m above sea level), 2011–2012 (108°04′E, 34°17′N; 517 m above sea level) and 2014–2015 (108°03′E, 34°17′N; 523 m above sea level) in Yangling, Shaanxi, China, and one cropping season of 2014–2015 (108°52′E, 34°36′N; 424 m above sea level) in Sanyuan, Shaanxi, China. Each plot contained five rows of two meters length with 0.5 and 0.15 m spacing between and within rows, respectively. Cultural practices including soil preparation, fertilizer and irrigations were applied equally to all the entries/experiments. The following agronomic traits were recorded from 10 randomly selected plants in the three middle rows in each plot just prior to harvest: plant height (PH), setting position of the first primary branch (SPFPB), primary branches per plant (PB), length of the terminal raceme (LTM), siliques on the terminal raceme (STR), siliques per plant (SP), and seeds per silique (SS). After harvest, seed yield per plant (SYP), 1000-seed weight (TSW), oil content (OC), and seed yield per hectare (SYH) were measured in each plot. The oil content (OC) was estimated from seeds taken from each plot using a NIR spectrophotometer (Foss NIR Systems Inc.) as per an established protocol (Velasco and Becker 1998).
Statistical analysis
Data recorded for each trait from four environments were analysed for separate analysis of variance (ANOVA) prior to the combined analysis over environments. ANOVA was performed among hybrid combinations across all locations with the general linear model (GLM) procedure using the Statistical Analysis System (SAS v. 9.3) (SAS Institute Inc. 2005). The accessions and testers used as parents in the experiment were considered fixed effects and the locations of the trials were considered random. The accession × tester analysis was performed based on data combined over locations. Source of variation due to entry and its interaction with locations were subdivided into variation due to hybrids and parents. Likewise, hybrid source of variation was partitioned into variation due to accessions, testers, and accessions × testers. The mean squares for male and female parents are independent estimates of GCA effects. The male × female interaction mean square is an estimate of SCA effects. When hybrids are evaluated in more than one location, the model used was as follows:
where Yijkl= observed value of the ijth hybrid in the klth plot; m = grand mean; El = effect of the lth environment (l = 1, 2, 3, 4); R(l)k = effect of the kth replication in the lth environment; Gi = the average effect of GCA of the ith male parent on its cross; Gj = the average effect of GCA of the jth female parent on its cross; Sij = the deviation of average effect of the ijth cross from expected performance based on the parents average effect SCA; (GE)il, (GE)jl, and (SE)ijl = the interaction with environments for the effects defined previously;and eijkl = the error term associated with the ijklth cross observations; Further, i =1, 2,…, m; m = 4 (m = number of males); j = 1, 2,…, f; f = 14 (f = number of females); k = 1, 2,…, r; r = 2 (number of replications); l = 1, 2,…, e; e = 4 (e = number of environment/location).
The GCA and SCA effects, based on data combined over locations, were estimated following the procedure of Beil and Atkins (1967) which is comparable to the analysis of a two way classification model with interaction components being a measure of the SCA effects. The GCA and SCA effects were estimated for each trait as follows:
where \({\bar{\text{y}}}_{{{\text{i}} .}}\) = the mean of all hybrids involving the ith female parent averaged across all replications and locations; \({\bar{\text{y}}}_{{ . {\text{j}}}}\) = the mean of all hybrids involving the jth male parent averaged across all replications and locations; \({\bar{\text{y}}}_{\text{ij}}\) = the mean of all hybrids of the cross between ith female and jth male parent averaged across all replications and locations; and \({\bar{\text{y}}}_{..}\) = the grand mean of hybrids.
Standard errors (SE) for GCA and SCA estimates were determined from respective mean squares following the method proposed by Groz et al. (1987) as follow:
where Mfl and Mml are the respective mean squares for accession × location and tester × location divided by number of observations (replications, locations, males or females). Mfml is the mean square for (accession × tester) × location divided by number of observations (replications and locations).
The critical difference (CD) was calculated as follows:
CD = SE × t (tabulated). If the absolute effect of GCA and SCA is greater than the CD, it is considered significantly different from zero.
Heterosis was estimated as follows: mid-parent heterosis (MPH) % = [(F1−MP)/MP] × 100, high-parent heterosis (HPH) % = [(F1−HPP)/HPP] × 100, high-check heterosis (HCK) % = [(F1−CK)/CK] × 100, where F1 is the hybrid value, MP = (P1 + P2)/2, in which P1 and P2 are the performance of both parental accessions, HPP is performance of high parent, and CK is the control value.
Results
Mean squares from combined ANOVA for all studied traits are presented in Table 2. ANOVA for each environment (location) showed highly significant (p ≤ 0.01) differences among parental genotypes and their F1 hybrids for all the traits (data not shown). The combined ANOVA revealed highly significant (p ≤ 0.01) differences among genotypes and their sub-sources of variations (parents, hybrids and hybrids vs. parents) for all the traits other than SPFPB, PB, and TSW which were non-significant for hybrids vs. parents. The genotype × location interaction was also highly significant (p ≤ 0.01) for all the traits except PB which was significant (p ≤ 0.05). The interaction of hybrids × locations was significant for all the traits except for SP.
Accession × tester analysis
Accession × tester analysis revealed that mean squares for accessions were highly significant for all the traits except for STR and SYP (Table 2). For testers, mean squares were significant for all the traits except for PH and PB (Table 2). For the interaction of accessions × testers, mean squares were significant for all traits. Results further expressed that interaction of accessions with locations (Loc × A) was significant for all the traits except SP and SYP (Table 2). The interaction of testers with locations (Loc × T) was significant for all the traits except for STR, SP, SYP, and SYH. The interaction of accessions, testers and locations (Loc × A × T) was non-significant for majority of traits except LTM, TSW, OC, SYP, and SYH which were significant.
General combining ability effects
The GCA effects of 14 accessions and four testers are summarized in Table 3. The results revealed that the accessions showed desirable GCA effects for all the traits except PH, whereas three of four testers showed poor GCA values. Among the 18 parental accessions, only the accession Excell exhibited negative and significant (p ≤ 0.05) GCA effects for PH thus, other parental accessions were poor general combiners for this trait. For the trait SPFPB female parents Excell, Elect, Profit, and 9E45 showed highly significant (p ≤ 0.01) negative GCA effects while CZ04, 9E38, 9E54, CZ02, CZ38, and CZ49, expressed highly significant (p ≤ 0.01) positive GCA effects, and the female parent 9E49 revealed significant (p ≤ 0.05) positive GCA effects. Among testers, only SH11 exhibited highly significant (p ≤ 0.01) positive GCA effects (Table 3). The female accessions CZ05, CZ04, 9E38, 9E45, and 9E49 revealed significant (p ≤ 0.01) positive GCA effects for PB, indicating that these parental accessions were good general combiners for this trait, however, all the testers showed non-significant GCA effects, thus considered as poor general combiners for this trait (Table 3). As regards LTM, all the accessions apart from CZ02 exhibited significant (p ≤ 0.01) positive or negative GCA effects for this trait. Among testers, SH11 displayed significant (p ≤ 0.05) negative GCA effects for this trait. The accessions Excell, SP-Armada, Elect, Profit, 9E45, 9E49, and CZ25, expressed positive GCA effects and proved to be good general combiners for this trait.
For STR only the Excell showed negative and 9E54 showed positive (p ≤ 0.01) GCA effects, whereas all the four testers exhibited non-significant GCA effects (Table 3). For SP, GCA effects were positive and significant (p ≤ 0.01) for seven accessions namely; CZ05, Elect, CZ04, 9E38, 9E45, CZ38, and CZ49 while only one tester GZ1R showed significant positive (p ≤ 0.05) GCA effect, therefore above seven female and one male parents were good general combiners for this trait. In the case of SS, the accessions Excell, 9E49, and 9E54 showed positive and significant (p ≤ 0.01) GCA effects, whereas the accessions CZ05, CZ25, and CZ38 displayed negative and significant (p ≤ 0.05) GCA effects. For SYP, the GCA effects were positive and significant for the accessions SP-Armada, Profit, 9E38, 9E49, and 9E54, which revealed that these accessions were good general combiners for this trait. However, the accessions 9E45, CZ02, CZ25, and CZ49 displayed negative (p ≤ 0.01) GCA effects for this trait. None of the testers showed significant effects for this trait.
For TSW, the accessions Excell, Profit, and 9E54 expressed highly significant positive (p ≤ 0.01) and accessions SP-Armada, Elect, and 9E49 showed positive and significant (p ≤ 0.05) GCA effects and can be regarded as good general combiners for TSW, whereas CZ04, 9E45, CZ02, CZ25, CZ38, and CZ49 revealed negative and significant GCA effects which proved them as poor general combiners for this trait. For OC, GCA effects were positive and highly significant (p ≤ 0.01) for accessions Excell, Elect, Profit, CZ04, 9E38, 9E45, and 9E38, and accessions SP-Armada and 9E49 showed significant (p ≤ 0.05) GCA effects which indicated that these accessions were good general combiners for OC. For SYH, the accessions SP-Armada and 9E49 indicated highly significant (p ≤ 0.01) and CZ25 showed significant (p ≤ 0.05) positive GCA effects and proved to be good general combiners for this trait, whereas Excell, CZ05, and CZ49 showed negative GCA effects. Among the testers the GCA effects were positive and significant (p ≤ 0.01) only for Zhong9 which revealed that this male parent was a good general combiner for SYH.
Specific combining ability effects
Significant SCA effects were observed for all traits other than PH (Supplementary Table S1). Out of 56 hybrids, 46 F1 hybrids revealed significant SCA effects for SPFPB, among them 25 hybrids produced positive and 21 hybrids produced negative SCA effects. Similarly, 23 hybrids displayed significant positive and negative SCA effects for PB. The significant SCA effects for LTM were found in 25 out of 56 hybrids, among them 14 hybrids revealed positive while 11 hybrids revealed negative SCA effects. As far as STR are concerned, only four hybrids showed desirable positive and significant SCA effects (Supplementary Table S1).
For SP, significant positive SCA effects were observed in 11 hybrids, whereas 13 hybrids showed significant negative SCA effects. Highest SCA effects were revealed by hybrids Zhong7 × 9E45, Zhong7 × CZ25, Zhong7 × CZ38, Zhong9 × Elect, Zhong9 × 9E38, Zhong9 × CZ38, GZ1R × 9E54, GZ1R × CZ38, GZ1R × CZ49, SH11 × Profit, and SH11 × 9E49. This indicated that these hybrids were promising specific combinations for SP. For SS, the highest positive and significant (p ≤ 0.01) SCA effects were found in hybrids Zhong7 × 9E49, Zhong7 × 9E54, Zhong9 × Excell, Zhong9 × SP-Armada, Zhong9 × CZ05, Zhong9 × 9E45, Zhong9 × 9E49, GZ1R × CZ05, GZ1R × CZ38, GZ1R × CZ49, and SH11 × CZ02. Significant SCA effects were observed in 21 out of 56 hybrids for SYP, among them 12 hybrids showed positive SCA effects while nine hybrids showed negative SCA effects. Highest significant (p ≤ 0.01) positive SCA effects were found in hybrids Zhong7 × Excell, Zhong9 × SP-Armada, Zhong9 × Profit, Zhong9 × 9E54, GZ1R × CZ38, GZ1R × 9E49, GZ1R × 9E38, GZ1R × CZ49, and SH11 × 9E49 (Supplementary Table S1). SCA effects for TSW indicated that 14 hybrids showed significant SCA effects, the best hybrids included Zhong7 × CZ05, Zhong7 × CZ04, Zhong9 × Excell, Zhong9 × 9E54, Zhong9 × CZ49, GZ1R × SP-Armada, GZ1R × 9E49, GZ1R × CZ38, GZ1R × CZ49, SH11 × CZ05, SH11 × 9E49, and SH11 × CZ02. For OC, most of the hybrids depicted significant SCA effects while highest positive SCA effects were displayed by hybrids Zhong7 × SP-Armada, Zhong7 × Profit, Zhong7 × CZ04, Zhong7 × CZ02, Zhong7 × CZ25, Zhong9 × Excell, Zhong9 × Elect, Zhong9 × 9E38, Zhong9 × 9E54, Zhong9 × CZ02, Zhong9 × CZ38, GZ1R × Elect, GZ1R × Profit, GZ1R × CZ04, GZ1R × CZ49, SH11 × 9E45, SH11 × CZ38, and SH11 × CZ49. Significant SCA effects for SYH were found in 33 hybrids, among them 17 hybrids displayed positive and 16 hybrids showed negative SCA effects. The highest positive SCA effects were found in the hybrids Zhong7 × 9E38, Zhong7 × CZ49, Zhong9 × 9E45, Zhong9 × CZ25, GZ1R × Excell, GZ1R × 9E38, GZ1R × 9E49, GZ1R × CZ38, GZ1R × CZ25, SH11 × SP-Armada, SH11 × 9E49, and SH11 × CZ02. The SCA effects suggested that these hybrids were good specific combinations for seed yield.
GCA and SCA variance components
Estimates of variance components and ratio for GCA and SCA effects are presented in Table 4. The variances of main effects were highly significant (p ≤ 0.01) for most of traits. The variances due to GCA revealed by accessions (σ2GCAAccessions(A)) were higher in magnitude than those for testers (σ2GCATesters(T)) for the traits PH, SPFPB, PB, LTM, SP, and SYH. Similarly, GCA variances of accessions for PH, SPFPB, and LTM were higher than effects shown by accessions × tester interactions (σ2SCAA × T). Overall, the extent of genetic effects displayed by accession × tester interaction (σ2SCAA×T) for traits PB, STR, SP, SS, SYP, TSW, and SYH were higher than those of the accessions (σ2GCAAccessions(A)) as well as testers (σ2GCATesters(T)). The interaction effects of σ2GCAAccessions(A) with locations were higher than those of σ2GCATesters(T) for PH, SPFPB, LTM, STR, SS, and SYH. The interaction of σ2SCAA × T × Loc was higher than σ2GCAAccessions(A) for STR, SP, SYP, TSW, OC, and SYH. The variance ratio of general to specific effects (σ2GCA/σ2SCA) was more than unity for PH, SPFPB, LTM, and OC. On average, the contribution of accession × tester was greater than that of accessions and testers for PB, STR, SP, SYP, TSW, OC, and SYH (Table 5). In contrast, the contribution of accessions was greater than that of testers for all traits except OC which was a little higher in extent than accessions.
Heterosis
The estimates of heterosis were calculated as percentage increase or decrease over mid-parent, high-parent and high-check values for all traits studied. The data regarding mid-parent heterosis (MPH) presented in Table 6 and Supplementary Table S2 revealed positive and negative MPH for all traits. The MPH for PH ranged from − 8.43% (Zhong9 × SP-Armada) to 55.22% (Zhong7 × 9E45) with mean of 24.47%, MPH for SPFPB ranged from − 57.96% (Zhong9 × CZ04) to 100.66% (GZ1R × ZC49) with mean of 10.54%, MPH for PB ranged from − 18.39% (GZ1R × CZ25) to 26.60% (Zhong9 × CZ38) with mean of 2.19%, MPH for LTM ranged from − 84.95% (SH11 × CZ02) to 34.92% (GZ1R × Profit) with mean of − 40.33%, MPH for STR ranged − 5.28% (Zhong7 × SP-Armada) to 71.08% (GZ1R × 9E49) with mean of 19.47%, MPH for SP ranged from − 18.64% (SH11 × SP-Armada) to 67.28% (Zhong7 × CZ38) with mean of 19.87%, MPH for SS ranged from − 8.89% (Zhong9 × CZ38) to 48.04% (Zhong7 × 9E45) with mean of 15.60%, MPH for SYP ranged from − 21.62% (SH11 × SP-Armada) to 86.38% (Zhong7 × Excell) with mean of 20.83%, MPH for TSW ranged from − 18.06% (GZ1R × Elect) to 24.83% (Zhong9 × Profit) with mean of 1.89%, MPH for OC ranged from − 15.68% (SH11 × 9E38) to 21.06% (Zhong7 × Profit) with mean of 1.92%, and MPH for SYH ranged from − 5.61% (SH11 × Excell) to 154.46% (Zhong7 × 9E38) with mean of 39.92%.
The data in Table 6 and Supplementary Table S3 depicted high-parent heterosis (HPH) in positive and negative directions for all traits. The HPH for PH ranged from − 8.89% (Zhong9 × SP-Armada) to 37.83% (Zhong7 × 9E45), HPH for SPFPB ranged from − 64.36% (Zhong7 × CZ04) to 65.56% (GZ1R × 9E45), HPH for PB ranged from − 18.39% (GZ1R × CZ25) to 29.93% (Zhong9 × CZ38), HPH for LTM ranged from − 85.78% (SH11 × 9E38) to 27.67% (GZ1R × Profit), HPH for STR ranged from -10.25% (Zhong9 × SP-Armada) to 69.43% (GZ1R × 9E49), HPH for SP ranged from − 31.75% (Zhong7 × SP-Armada) to 57.16% (Zhong7 × CZ49), HPH for SS ranged from − 23.94% (Zhong7 × CZ38) to 37.96% (GZ1R × 9E38), HPH for SYP ranged from − 35.72% (SH11 × Excell) to 62.08% (Zhong7 × Excell), HPH for TSW ranged from − 28.03% (GZ1R × Elect) to 20.94% (Zhong9 × Profit), HPH for OC ranged from − 18.60% (SH11 × 9E38) to 14.05% (Zhong7 × Profit), and HPH for SYH ranged from − 20.65% (SH11 × Excell) to 125.71% (Zhong7 × 9E38).
The data in Table 6 and Supplementary Table S4 also manifested high-check heterosis (HCK) in both positive and negative directions for all traits. The HCK for PH ranged from − 17.83% (Zhong9 × SP-Armada) to 9.28% (Zhong9 × CZ25), HCK for SPFPB ranged from − 57.89% (Zhong9 × CZ04) to 7.85% (SH11 × CZ25), HCK for PB ranged from − 2.93% (Zhong9 × CZ25) to 28.66% (Zhong9 × CZ38), HCK for LTM ranged from − 86.48% (GZ1R × CZ25) to 13.84% (Zhong7 × CZ04), HCK for STR ranged from − 8.74% (Zhong9 × SP-Armada) to 50.97% (GZ1R × 9E49), HCK for SP ranged from − 19.25% (Zhong7 × CZ05) to 45.04% (Zhong7 × CZ38), HCK for SS ranged from − 24.54% (SH11 × Excell) to 10.93% (GZ1R × 9E38), HCK for SYP ranged from − 28.85% (SH11 × Excell) to 46.0% (GZ1R × 9E49), HCK for TSW ranged from − 18.08% (SH11 × Excell) to 17.40% (Zhong7 × Profit), HCK for OC ranged from − 11.28% (SH11 × 9E38) to 5.66% (Zhong9 × CZ02), and HCK for SYH ranged from − 35.36% (Zhong9 × CZ38) to 20.52% (Zhong9 × CZ25).
MPH values for SYH were positive for 53 out of 56 hybrids and the remaining 3 hybrids showed negative heterosis, it ranged from − 5.61% (SH11 × Excell) to 154.46% (Zhong7 × 9E38) (Tables 6, 7). HPH for SYH showed that 40 out of 56 hybrids displayed positive heterosis and the remaining 16 hybrids revealed negative heterosis, and it ranged from − 20.65% (SH11 × Excell) to 125.71% (Zhong7 × 9E38) (Tables 6, 7). HCK for SYH revealed that five out of 56 F1 hybrids showed positive heterosis and the rest of the 51 F1 hybrids expressed negative heterosis and it ranged from − 35.36% (Zhong9 × CZ38) to 20.52% (Zhong9 × CZ25) (Table 7, Supplementary Table S4). The five hybrids showing heterosis over the check included Zhong9 × CZ25 (HCK = 20.52%), GZ1R × 9E38 (9.05%), Zhong7 × 9E38 (8.60%), Zhong7 × CZ49 (3.54%), and GZ1R × 9E45 (0.28%).
Difference between spring, semi-winter and winter hybrids for some agronomic traits
The 18 parental accessions were classified into three groups; semi-winter, spring, and winter types (Table 1). The results indicated significant differences among the three parental groups for SPFPB and SS, but not for the remaining nine traits (Supplementary Table S5). SPFPB in the spring group was significantly higher than semi-winter and winter group parents while other two groups had no difference in SPFBP. For SS, significant difference existed between Chinese semi-winter and spring groups, whereas no significant difference was observed between spring and winter group parents.
All 56 hybrids in the present study were divided into two hybrid types, the hybrids between spring and semi-winter (spring and semi-winter type), and the hybrids between winter and semi-winter (winter and semi-winter type). The spring and semi-winter type group contained 24 hybrids and the winter and semi-winter type group contained 32 hybrids. The differences for the main agronomic traits between the above both types are presented in Table 8. The results revealed highly significant variation between the both type of hybrids for traits PH, SPFPB, PB, LTM, STR, SP, and OC, whereas, it was not significant for the SYH and its three components (SS, SYP, and TSW).
Correlation between seed yield and other traits
Correlation analysis for the 11 tested traits (Supplementary Table S6) indicated that, SYH expressed highly significant positive correlation with PH (0.594), STR (0.539), SP (0.396), SS (0.505), and SYP (0.560), however it expressed significant negative correlation with OC (− 0.246). OC also showed significant negative correlation with SPFPB (− 0.484). TSW showed significant positive correlation with SS (0.380) and SYP (0.439), and negative correlation with PB(-0.379) and SP (-0.352). SYP showed significant positive correlation with PH (0.409), STR (0.521), SP (0.292) and SS (0.511), and negative correlation with LTM (− 0.261). SS showed significant positive correlation with PH (0.344) and STR (0.307). SP expressed significant positive correlation with PH (0.721), PB (0.674), and STR (0.495), and negative correlation with LTM (− 0.473). STR exhibited significant positive correlation with PH (0.556) and SPFPB (0.245), and negative correlation with LTM (− 0.382). The LTM showed significant negative correlation for PH (− 0.683), SPFPB (− 0.500), and PB (− 0.358). PB and SPFPB expressed significant positive correlation with PH (0.452 and 0.437, respectively).
Discussion
Rapeseed (B. napus) germplasm could be classified into spring, winter, and semi-winter types (Diers and Osborn 1994; Becker et al. 1995). Hybridization between these three types is an important approach to broadening the genetic base of each of the three types. Previous investigations revealed that significant heterosis existed between winter and semi-winter type hybrids (Lefort-Buson et al. 1987; Qian et al. 2009), and between semi-winter and spring type hybrids (Qian et al. 2007) in European and Canadian environments (Rahman 2013). The winter and semi-winter rapeseed germplasm was used for improvement of seed yield and other traits in hybrids and open-pollinated cultivars of spring type rapeseed (Butruille et al. 1999; Quijada et al. 2004, 2006; Udall et al. 2004, 2006; Kebede et al. 2010; Rahman 2011, 2013; Rahman and Kebede 2012). However, the potential of winter and spring rapeseed genetic resource for semi-winter rapeseed breeding was not fully exploited. In the present study, 14 exotic accessions (spring and winter types) and four testers (semi-winter type) of rapeseed were crossed in line × tester mating design to estimate GCA and SCA for seed yield, yield components, and oil content in four different environments in northern China. Highly significant differences were observed among the parental genotypes and their F1 hybrids for all the traits. The extent of GCA/SCA variance ratio showed that PH, SPFPB, LTM, and OC were controlled by additive genes. However, PB, STR, SP, SS, SYP, TSW, and SYH were controlled by non-additive gene action.
Heterosis and combining ability between Chinese semi-winter and exotic spring and winter germplasm
Higher seed yield is the most important objective of all the breeding programs. In plant breeding GCA and SCA effects are important indicators in the identification of potential parental accessions in hybrid combinations. In the present study, we have identified some elite parents with significant positive GCA effects for seed yield and its components. For SYH, the accessions 9E49, SP-Armada, and CZ25, and the tester Zhong9 were identified with significant positive GCA effects, indicating that these accessions would increase grain yield in their hybrids. F1 hybrids Zhong9 × CZ25, GZ1R × 9E38, Zhong7 × 9E38, Zhong7 × CZ49, and GZ1R × 9E45 proved to be the best hybrids. Seed oil content is very important trait which determines the economic value of rapeseed. The parents and hybrids with positive GCA and SCA effects are highly desirable in rapeseed breeding. In this study, significant GCA effects for OC were observed for the accessions 9E45, 9E38, Excell, Profit, CZ04, SP-Armada, and 9E49 across the tested locations suggesting that these accessions could be useful contributors of desirable alleles for improvement in oil content. Among F1 hybrids Zhong7 × CZ25, Zhong7 × Profit, Zhong7 × CZ04, SH11 × 9E45, GZ1R × CZ04, Zhong7 × CZ02, GZ1R × CZ49, Zhong9 × Excell, Zhong9 × CZ02, SH11 × CZ49, GZ1R × Profit, and Zhong7 × SP-Armada revealed significant SCA effects which indicates that selection in upcoming generations would be effective for improvement in oil content.
The GCA and SCA variances are used to assess the contribution of additive and non-additive gene actions involved in the inheritance of characters. In present study, additive and non- additive gene actions were important in the studied traits, with the preponderance of additive gene action for PH, SPFPB, and LTM, and non-additive gene action for PB, STR, SP, SS, SYP, TSW, OC, and SYH (Table 4). For SP, our results are in line with Rameeh (2012b) and Nassimi et al. (2006). For SS, the present findings agree with results of Davik (1997) and Teklewold and Becker (2005) who reported importance of non-additive gene action for this trait. For SYP, non-additive gene action controlled this trait which is in contradiction with findings of Zhai et al. (2002) and Ali et al. (2015) who reported that this trait was governed by additive gene action. For TSW, our results are in accordance with the results of Davik (1997), Teklewold and Becker (2005) and Nassimi et al. (2006). They reported non-additive type of gene action controlling this trait. The present study further revealed that trait OC was governed by non-additive genes, our results are in conformity with those of Downey and Rimer (1993), Teklewold and Becker (2005), Huang et al. (2010) and disagree with the findings of Shen et al. (2002), Rameeh et al. (2003), Qian et al. (2007, 2009), Wang et al. (2010), Shehzad et al. (2015) who reported additive gene action underlying this trait. For SYH, our findings are similar to the results of Huang et al. (2010), Turi et al. (2011) and Rameeh (2012b) and disagree with those of Qian et al. (2007, 2009), who reported additive gene action to be more important than non-additive gene action in rapeseed hybrids between Chinese semi-winter accessions and winter and spring accessions evaluated for seed yield in European and Canadian environments. That our results are in contradiction to the results of previous workers may be due to different germplasm evolved in different environments. The results obtained for contributions of accessions, testers and their interaction to the total variance (Table 5) verify that SCA effects were more important than GCA effects in these traits.
Substantial levels of heterosis for yield and yield components have been observed in F1 hybrids of winter, spring and Chinese semi-winter types of rapeseed (Lefort-Buson et al. 1987; Udall et al. 2004; Qian et al. 2007; Tian et al. 2015). In these situations one of the improvement strategies might be hybrid breeding. For a successful heterosis breeding program there must be an evidence of the presence of significant heterotic effects in the hybrids which could be utilized for commercial hybrid seed production.
In rapeseed, traits like SP, SS, and TSW directly contribute to seed yield. Therefore, positive heterotic effects are important in the improvement of rapeseed hybrids. All F1 hybrids exhibited variable positive and negative MPH, HPH, and HCK heterotic effects for yield and its contributing traits (Supplementary Tables S2, S3, and S4). For seed yield, substantial MPH of 154% and HPH of 125.71% was observed in this study. Several researchers have reported different levels of heterosis for seed yield in rapeseed for instance; Brandle and McVetty (1989) found 120% HPH, Riaz et al. (2001) reported 169% MPH in B. napus, Ahmad et al. (2011) observed 127% MPH in spring type rapeseed hybrids. In contrast, a little lower extent of heterosis than the values reported in our study has also been reported by Grant and Beversdorf (1985), Lefort-buson et al. (1987), Dhillon et al. (1996), Starmer et al. (1998), Rameeh (2011, 2012a). Qian et al. (2007, 2009) reported the average MPH of 50.1 and 57.8% for hybrids derived from hybridization between spring and Chinese semi-winter type and, winter and Chinese semi-winter type parents, respectively in European and Canadian environments. In the present study, results showed that four hybrids (4/56) were high yielding than the check, the HCK of highest hybrid reached 20.52%. Although the number of hybrids with yield over check is far lower than the hybrids within semi-winter type, conducted by Tian et al. (2015, 2017) who reported that 21/36 hybrids over yielded the check with the highest HCK of 23.56%, this indicates that spring and winter type rapeseed germplasm can be utilized for increasing yielding potential of Chinese semi-winter type rapeseed. The heterosis levels for SP found in this study were higher than those previously reported by Rameeh et al. (2003), Sabaghnia et al. (2010a), Nasrin et al. (2011), Dar et al. (2012), Rameeh (2012a), Nasim et al. (2014) and Shehzad et al. (2015). For SS the findings of present study are higher than those discovered by Larik and Hussain (1990), Rameeh et al. (2003) and Sincik et al. (2011) in B. napus. In this study, F1 hybrids revealed high positive heterosis values for TSW. Our results are higher than previously reported by Sabaghnia et al. (2010a), Azizinia (2011), Sincik et al. (2011), Rameeh (2012b) and Shehzad et al. (2015) in B. napus. However, higher heterosis levels than obtained in our results were reported by Ahsan et al. (2013) and Shehzad et al. (2015) in B. napus.
High oil content is one of the most important objectives in rapeseed breeding and positive heterosis effects are considered useful for selecting superior hybrids for oil yield. Heterotic effects for oil content found in this investigation were higher than those reported by Ahmad et al. (2011), Azizinia (2011) and Shehzad et al. (2015) in B. napus; Meena et al. (2014) in B. juncea, Mohammed (2011) in B. carinata. In contrast, Shen et al. (2005), Sabaghnia et al. (2010a) and Abideen et al. (2013) reported higher heterotic effects in B. napus than found in our study. Several researchers have also reported that negative or absence of heterosis for OC is a common phenomenon in oil seed Brassicas (Brandle and McVetty 1990; Falk et al. 1994; Goffman and Becker 2001; Adefris and Becker 2005; Teklewold and Becker 2005; Ofori and Becker 2008).
The order of heterotic effects of traits, SYP, its components and, OC, is in agreement with that of Shen et al. (2002, 2005), who reported that heterosis of SYP was higher than that of OC. For yield components, heterosis of SP was the highest, followed by SS and TSW. The reason may be that vegetative traits can be regarded as ‘source’, and the yield traits include ‘source’ and ‘sink’, and the quality traits of ‘sink’ also involve more complex biochemical processes. The ‘flow’ is the coordinator between ‘source’ and ‘sink’. For the heterosis level of ‘source’ and ‘reservoir’, ‘flow’ is the important factor limiting the heterosis of ‘sink’, thus adjusting the flow can reduce the loss of ‘flow’ and result in improvement of ‘reservoir’(Shen et al. 2002).
Correlation between seed yield, its components, and oil content
The breeding programs aiming at yield improvement primarily depend on the knowledge of the extent and direction of correlation between yield and its components and the relative importance of each component in its contribution to seed yield. Therefore, it is prerequisite of any breeding program to investigate the inter-relationships between yield and its components. In the present study, highly significant correlation was recorded between SYH and PH, STR, SP, SS, and SYP, whereas it showed significant negative correlation with OC. This reveals that SYH strongly depends on its components. However, increased seed yield resulted in lower oil content. Our findings are in agreement with Rameeh (2012c, 2015) who reported positive correlation between SP and SYH suggesting that SP could be used as good selection criterion in the improvement of SYH. Our results also supported those of Sabaghnia et al. (2010b) who reported significant positive correlation between SYH and PH, SP, and SS.
Breeding potential of winter and spring germplasm in semi-winter type breeding program
Most Chinese semi-winter cultivars of rapeseed were developed through the pedigree breeding method and interspecific hybridization between European B. napus and the indigenous B. rapa varieties (Liu 2000; Qian et al. 2006; Hu et al. 2007). For analysis of the differences for the main agronomic traits between spring and semi-winter, and winter and semi-winter type hybrids, we divided all 56 hybrids into two types, the hybrids between spring and semi-winter, and the hybrids between winter and semi-winter type. The results revealed highly significant differences between the spring and the winter type hybrids for some traits, including OC. But for seed yield, we did not detect significant differences between the two hybrid groups. This means that both winter and spring germplasm have potential in Chinese rapeseed breeding program. Winter rapeseed germplasm has more value for seed yield improvement, all the four parents of five hybrids whose seed yield was higher than Check, were winter type (Table 7), however, for seed oil content improvement, we should pay more attention to spring germplasm.
In the present study, the tested 18 parental accessions were divided into three groups; semi-winter, spring, and winter group. The results revealed that all the traits showed non-significant differences except SPFPB and SS (Supplementary Table S5). This is not in consistence with the results of Qian et al. (2007, 2009), who found that semi-winter and spring and winter materials used had significant differences in most of the traits. The reason may be that their materials had been selected without consideration of adaptation to local rapeseed-growing conditions. In our study, we have improved adaptation of the introduced spring and winter germplasm to Chinese local condition before using as parents in the present study.
In conclusion, highly significant differences were observed among parents and their F1 hybrids for all the traits. The accessions showed more variability in performance than testers and interaction of accessions with testers was highly significant for all traits suggesting the presence of specific effects. The GCA and SCA variances revealed that plant height, setting position of the first primary branch, and length of the terminal raceme were controlled by additive genes and primary branches per plant, siliques on the terminal raceme, siliques per plant, seeds per silique, seed yield per plant, 1000-seed weight, oil content, and seed yield per hectare were controlled by non-additive genes. The parents SP-Armada, 9E49 and CZ25, and the tester Zhong9 were good general combiners for seed yield. Among 56 F1 hybrids, four hybrids Zhong9 × CZ25, GZ1R × 9E38, Zhong7 × 9E38, and Zhong7 × CZ49 high yielded than the check and were the outstanding combinations for seed yield. These hybrids are recommended to be included in future breeding programs for development of new high yielding varieties with more desirable traits. Both winter and spring germplasm have potential in Chinese rapeseed breeding program, and winter germplasm has more value for seed yield improvement, however, for seed oil content improvement more attention may be paid to spring germplasm.
References
Abideen NS, Nadeem F, Abideen SA (2013) Genetic variability and correlation studies in Brassica napus genotypes. Int J Innov Appl Stud 2:574–581
Adefris T, Becker HC (2005) Heterosis and combining ability in a diallel cross of Ethiopian mustard inbred lines. Crop Sci 45:2629–2635
Ahmad R, Farhatullah, Carlos FQ (2011) Inter and intra-cluster heterosis in spring type oilseed rape (Brassica napus L.) hybrids and prediction of heterosis using SRAP molecular markers. SABRAO J Breed Genet 43:27–43
Ahsan MZ, Khan FA, Kang SA, Rasheed K (2013) Combining ability and heterosis analysis for seed yield and yield components in Brassica napus L. J Biol Agric Healthc 3:31–36
Ali N, Khan NU, Ali S, Farhatullah, Gul S, Saeed M, Naveed K (2015) Combining ability studies for quantitative traits in Brassica juncea. J Anim Plant Sci 25:494–501
Allender CJ, King GJ (2010) Origins of the amphidiploid species Brassica napus L. investigated by chloroplast and nuclear molecular markers. BMC Plant Biol 10:54–66
Azizinia S (2011) Combining ability analysis of yield component parameters in winter rapeseed genotypes (Brassica napus L.). J Agric Sci 4:87–94
Becker HC, Engqvist GM, Karlsson B (1995) Comparison of rapeseed cultivars and resynthesized lines based on allozyme and RFLP markers. Theor Appl Genet 91:62–67. https://doi.org/10.1007/BF00220859
Beil GM, Atkins RE (1967) Estimates of general and specific combining ability in F1 hybrids for grain yield and its components in grain sorghum (Sorghum vulgare Pers). Crop Sci 7:225–228
Birchler JA, Yao H, Chudalayandi S, Vaiman D, Veitia RA (2010) Heterosis. Plant Cell 22:2105–2112. https://doi.org/10.1105/tpc.110.076133
Brandle JE, McVetty PBE (1989) Heterosis and combining ability in hybrids derived from oilseed rape cultivars and inbred lines. Crop Sci 29:1191–1195
Brandle JE, McVetty PBE (1990) Geographical diversity, parental selection and heterosis in oilseed rape. Can J Plant Sci 70:935–940
Butruille DV, Guries RP, Osborn TC (1999) Increasing yield of spring oilseed rape hybrids through introgression of winter germplasm. Crop Sci 39:1491–1496
Ceyhan E, Avci MA, Karadas S (2008) Line × tester analysis in pea (Pisum sativum L.): Identification of superior parents for seed yield and its components. Afr J Biotechnol 7:2810–2817
Chen S, Nelson MN, Ghamkhar K, Fu T, Cowling WA (2008) Divergent patterns of allelic diversity from similar origins: the case of oilseed rape (Brassica napus L.) in China; and Australia. Genome 51:1–10. https://doi.org/10.1139/G07-095
Crow JF (1998) 90 years ago: the beginning of hybrid maize. Genetics 148:923–928
Dar ZA, Shafiq AW, Gulzaffar, Habib M, Sofi NR et al (2012) Heterosis studies in brown sarson (Brassica rapa L.). Electron J Plant Breed 3:676–681
Davik J (1997) Parameter estimates from generation means in Swedes (B. napus ssp. rapifera L.). Euphytica 98:53–58
Denning GL, Mew TW (1997) China and IRRI: improving China’s rice productivity in the 21st century. In: Proceedings of the China-IRRI dialogue, discussion Series No. 31. Beijing, China. 7–8 Nov. 1997. International Rice Research Institute, Beijing
Dhillon BS, Banga SS, Mangat BK, Allah-Rang, Randhawa LS et al (1996) Hybrid breeding in crop plants. J Res Punjab Agric Univ 33:1–4
Diers BW, Osborn TC (1994) Genetic diversity of oilseed Brassica napus germplasm based on restriction fragment length polymorphisms. Theor Appl Genet 88:662–668. https://doi.org/10.1007/Bf01253968
Downey RK (1983) The origin and description of the Brassica oilseed crops. In: Kramer JKG et al (eds) High and low erucic acid rapeseed oils: production, usage, chemistry, and toxicological evaluation. Academic Press Canada, Don Mills
Downey RK, Rimer SR (1993) Agronomic improvement in oilseed Brassicas. Adv Agron 50:1–66
Falk KC, Rakow GFW, Downey RK, Spurr DT (1994) Performance of inter-cultivar summer turnip rape hybrids in Saskatchewan. Can J Plant Sci 74:441–445
FAOSTAT (2015) FAOSTAT. www.faostat.fao.org. March 2017
Farshadfar E, Kazemi Z, Yaghotipoor A (2013) Estimation of combining ability and gene action for agro-morphological characters of rapeseed (Brassica napus L.) using line × tester mating design. Int J Adv Biol Biomed Res 1:711–717
Fu DH, Li XM, Alice H, Fu Y, Liu G, Jiang G, Zhang H (2014) Utilization of crop heterosis: a review. Euphytica 197:161–173. https://doi.org/10.1007/s10681-014-1103-7
Goffman FD, Becker HC (2001) Diallel analysis for tocopherol content in seeds of rapeseed. Crop Sci 41:1072–1079
Grant I, Beversdorf WD (1985) Heterosis and combining ability estimates in spring oilseed rape (Brassica napus L.). Can J Genet Cytol 27:472–478
Groz HJ, Haskins FA, Pedersen JF, Ross WM (1987) Combining ability effects for mineral elements in forage sorghum hybrids. Crop Sci 27:216–219
Hu SW, Yu CY, Zhao HX, Sun GL, Zhao SL et al (2007) Genetic diversity of Brassica napus L. germplasm from China and Europe assessed by some agronomically important characters. Euphytica 154:9–16. https://doi.org/10.1007/s10681-006-9263-8
Huang Z, Laosuwan P, Machikowa T, Chen Z (2010) Combining ability for seed yield and other characters in rapeseed. Suranaree J Sci Technol 17:39–47
Kebede B, Thiagarajah M, Zimmerli C, Rahman MH (2010) Improvement of open-pollinated spring rapeseed (Brassica napus L.) through introgression of genetic diversity from winter rapeseed. Crop Sci 50:1236–1243. https://doi.org/10.2135/cropsci2009.06.0352
Kempthorne O (1957) An introduction to genetic statistics. Wiley, New York, p 458
Khan SA, Ahmad H, Khan A, Saeed M, Khan SM, Ahmad B (2009) Using line × tester analysis for earliness and plant height traits in sunflower (Helianthus annus L). Recent Res Sci Technol 1:202–206
Larik AS, Hussain M (1990) Heterosis in Indian mustard (Brassica juncea L.). Pak J Bot 22:168–171
Lefort-Buson M, Guillot-Lemoine B, Dattee Y (1987) Heterosis and genetic distance in rapeseed (Brassica napus L.): crosses between European and Asian selfed lines. Genome 29:413–418
Li W, Jiang W, Zhao HX, Vyvadilova M, Stamm M, Hu SW (2012) Genetic diversity of rapeseed accessions from different geographic locations revealed by expressed sequence tag-simple sequence repeat and random amplified polymorphic DNA markers. Crop Sci 52:201–210. https://doi.org/10.2135/cropsci2011.06.0301
Liu HL (1985) Rapeseed genetics and breeding. Shanghai Science and Technology Press, Shanghai, pp 38–42
Liu HL (2000) Genetics and breeding in rapeseed. Chinese Agricultural University Press, Beijing, pp 26–45
McVetty PBE (1995) Review of performance and seed production of hybrid Brassicas. In: Proceedings of the 9th international rapeseed congress, Cambridge, UK, pp 98–103
Meena HS, Ram B, Kumar A, Singh BK, Meena PD et al (2014) Heterobeltiosis and standard heterosis for seed yield and important traits in Brassica juncea. J Oilseed Brassica 5:134–140
Mohammed IM (2009) Line × tester analysis across locations and years in Sudanese × exotic lines of sorghum. J Plant Breed Crop Sci 1:311–319
Mohammed W (2011) Combining ability and potential heterosis in Ethiopian Mustard (Brassica carinata A. Braun). East Afr J Sci 5:99–107
Nasim A, Farhatullah, Khan N, Afzal M, Azam SM et al (2014) Combining ability and heterosis for yield and yield contributing traits in Brassica rapa (L.) ssp. Dichotoma (roxb.) Hanelt. Pak J Bot 46:2135–2142
Nasrin S, Nur F, Nasreen MK, Bhuiyan MSR et al (2011) Heterosis and combining ability analysis in Indian mustard (Brassica juncea L.). Bang Res Pub J 6:65–71
Nassimi AW, Raziuddin R, Ali N, Ali S, Bakht J (2006) Combining ability analysis in Brassica napus L. lines for yield associated traits. Asian J Biol Sci 9:2333–2337
Ofori A, Becker HC (2008) Breeding of Brassica rapa for biogas production: heterosis and combining ability of biomass yield. Bioenergy Res 1:98–104
Qian W, Meng J, Li M, Frauen M, Sass O et al (2006) Introgression of genomic components from Chinese Brassica rapa contributes to widening the genetic diversity in rapeseed (B. napus L.) with emphasis on the evolution of Chinese rapeseed. Theor Appl Genet 113:49–54. https://doi.org/10.1007/s00122-006-0269-3
Qian W, Sass O, Meng J, Li M, Frauen M et al (2007) Heterotic patterns in rapeseed (Brassica napus L.): I. Crosses between spring and Chinese semi-winter lines. Theor Appl Genet 115:27–34. https://doi.org/10.1007/s00122-007-0537-x
Qian W, Li Q, Noack J, Sass O, Meng J et al (2009) Heterotic patterns in rapeseed (Brassica napus L.): II. Crosses between European winter and Chinese semi-winter lines. Plant Breed 128:466–470. https://doi.org/10.1111/j.1439-0523.2008.01597.x
Quijada PA, Udall JA, Polewicz H, Vogelzang RD, Osborn TC (2004) Phenotypic effects of introgressing French winter germplasm into hybrid spring canola. Crop Sci 44:1982–1989
Quijada PA, Udall JA, Lambert B, Osborn TC (2006) Quantitative trait analysis of seed yield and other complex traits in hybrid spring rapeseed (Brassica napus L.): 1. Identification of genomic regions from winter germplasm. Theor Appl Genet 113:549–561
Rahman H (2011) Use of European winter canola Brassica napus L. for the improvement of Canadian spring canola: a practical breeding example. In: Proceedings of the 13th international rapeseed congress, Prague. 5–9 June 2011. Int Consultative Group for Res on Rapeseed, Paris. pp 875–878
Rahman H (2013) Review: breeding spring canola (Brassica napus L.) by the use of exotic germplasm. Can J Plant Sci 93:363–373
Rahman H, Kebede B (2012) Improvement of spring canola Brassica napus (L.) by use of winter canola. J Oilseed Brassica 3:1–17
Rameeh V (2011) Line × tester analysis for seed yield and yield components in spring and winter type varieties of oil seed rape. J Cereals Oilseeds 2:66–70
Rameeh V (2012a) Combining ability analysis of plant height and yield components in spring type of rapeseed varieties (Brassica napus L.) using line × tester analysis. Int J Agric For 2:58–62. https://doi.org/10.5923/j.ijaf.20120201.10
Rameeh V (2012b) Combining ability and heritability estimates of main agronomic characters in rapeseed breeding lines using Line × tester analysis. J Agric Sci 57:111–120. https://doi.org/10.2298/JAS1203111R
Rameeh V (2012c) Heterosis and heterobeltiosis of yield associated traits in rapeseed cultivars under limited nitrogen application. Agriculture 58:77–84. https://doi.org/10.2478/v10207-012-0009-4
Rameeh V (2015) Genetic variability and interrelationships among quantitative traits in rapeseed (Brassica napus) advanced lines. J Agric Sci 3:158–167. https://doi.org/10.4038/jas.v10i3.8069
Rameeh V, Rezai A, Saeidi G (2003) Estimation of genetic parameters for yield, yield components and glucosinolate in rapeseed (Brassica napus L). J Agric Sci Technol 5:143–151
Riaz A, Li G, Quresh Z, Swati MS, Quiros CF (2001) Genetic diversity of oilseed Brassica napus inbred lines based on sequence-related amplified polymorphism and its relation to hybrid performance. Plant Breed 120:411–415. https://doi.org/10.1046/j.1439-0523.2001.00636.x
Sabaghnia N, Dehghan H, Alizadeh B, Mohghaddam M (2010a) Heterosis and combining ability analysis for oil yield and its components in rapeseed. Aus J Crop Sci 4:390–397
Sabaghnia N, Dehghan H, Alizadeh B, Mohghaddam M (2010b) Interrelationships between seed yield and 20 related traits of 49 canola (Brassica napus L.) genotypes in non-stressed and water-stressed environments. Span J Agric Res 8:356–370
Saeed A, Chaudhry MA, Saeed N, Khaliq I, Johar MZ (2001) Line × tester analysis for some morpho-physiological traits in bread wheat. Int J Agric Biol 3:444–447
SAS Institute (2005) Statistics analysis software (SAS) user’s guide. SAS Institute Inc, Cary
Shehzad A, Ashraf MF, Sultan S, Ali M, Sadaqat HA (2015) Heterosis studies for some morphological, seed yield and quality traits in rapeseed (Brassica napus L.). J Biol Agric Healthc 23:39–47
Shen JX, Fu TD, Yang GS (2002) Heterosis of double low self-incompatibility in oilseed rape (Brassica napus L.). Agric Sci China 1:732–737
Shen JX, Fu TD, Yang GS, Ma CZ, Tu X (2005) Genetic analysis of rapeseed self-incompatibility lines reveals significant heterosis of different patterns for yield and oil content traits. Plant Breed 124:111–116
Shull GH (1948) What is heterosis? Genetics 33:439–446
Shull GH (1952) Beginnings of the heterosis concept. In: Gowen JW (ed) heterosis. Iowa State College Press, Lowa, pp 14–48
Sincik M, Goksoy AT, Turan ZM (2011) The heterosis and combining ability of diallel crosses of rapeseed inbred lines. Not Bot Horti Agrobot 39:242–248
Sprague GF, Tatum LA (1942) General vs. specific combining ability in single crosses of corn. J Am Soc Agron 34:923–932
Starmer KP, Brown J, Davis JB (1998) Heterosis in spring Canola hybrids grown in Northern Idaho. Crop Sci 38:376–380
Sun VG (1948) Breeding plants of brassica. Proc Chin Agron Assoc 71:42–52
Teklewold A, Becker HC (2005) Heterosis and combining ability in a diallel cross of Ethiopian mustard inbred lines. Crop Sci 45:2629–2635
Tian HY, Channa SA, Hu SW (2015) Heterotic grouping and the heterotic pattern among Chinese rapeseed (Brassica napus L.) accessions. Agron J 107:1321–1330. https://doi.org/10.2134/agronj14.0557
Tian HY, Channa SA, Hu SW (2017) Relationship between genetic distance, combining ability and heterosis in rapeseed (Brassica napus L.). Euphytica 213:1. https://doi.org/10.1007/s10681-016-1788-x
Turi NA, Raziuddin Farhatullah, Khan NU, Hassan G et al (2011) Combining ability for yield related traits in Brassica juncea. Pak J Bot 43:1241–1248
Udall JA, Quijada PA, Polewicz H, Vogelzang R, Osborn TC (2004) Phenotypic effects of introgressing Chinese winter and resynthesized Brassica napus L. germplasm into hybrid spring canola. Crop Sci 44:1990–1996
Udall JA, Quijada PA, Lambert B, Osborn TC (2006) Quantitative trait analysis of seed yield and other complex traits in hybrid spring rapeseed (Brassica napus L.): 2. Identification of alleles from unadapted germplasm. Theor Appl Genet 113:597–609
Velasco L, Becker HC (1998) Estimating the fatty acid composition of the oil in intact-seed rapeseed (Brassica napus L.) by near-infrared reflectance spectroscopy. Euphytica 101:221–230
Wang HZ, Yin Y (2014) Analysis and strategy for oil crop industry in China. Chin J Oil Crop Sci 36:414–442. https://doi.org/10.7505/j.issn.1007-9084
Wang X, Hua W, Liu G, Liu J, Yang Q et al (2010) Genetic analysis on oil content in rapeseed (Brassica napus L.). Euphytica 173:17–24
Yuan LP (2014) Development of hybrid rice to ensure food security. Rice Sci 21:1–2. https://doi.org/10.1016/S1672-6308(13)60167-5
Zhai HQ, Cao SQ, Tang YL, Zhang RX, Sheng SL et al (2002) Analysis of combining ability and heritability of photosynthetic characters in indica hybrid rice. Acta Agron Sin 13:135–142
Acknowledgements
This research work was supported by the earmarked fund for China Agriculture Research System (CARS-13), Key Research and Development Project in Shaanxi Province of China (2018ZDXM-NY-008), Modern Crop Seed Industry Project of Shaanxi Province (20171010000004), and Tang Zhongyin breeding foundation of Northwest A&F University.
Author information
Authors and Affiliations
Contributions
S.A.C and H.T are equal first contributing authors to this paper. The experiments were designed and conducted by S.A.C, H.T, and S. H. The basic germplasm resources were developed by S.A.C, H. T, R. Z, S.H, M.K, and M.S. The data was collected by S.A.C, H. T, R.Z, S.F, and Y.G. The analysis was conducted by S.A.C, S.H and M.I.M. The manuscript was written and revised by S.A.C, H.T, S.H, M.K, and M.S.
Corresponding author
Ethics declarations
Conflict of interest
The authors declare that they have no conflict of interest.
Electronic supplementary material
Below is the link to the electronic supplementary material.
Rights and permissions
About this article
Cite this article
Channa, S.A., Tian, H., Mohammed, M.I. et al. Heterosis and combining ability analysis in Chinese semi-winter × exotic accessions of rapeseed (Brassica napus L.). Euphytica 214, 134 (2018). https://doi.org/10.1007/s10681-018-2216-1
Received:
Accepted:
Published:
DOI: https://doi.org/10.1007/s10681-018-2216-1