Abstract
The genetic effects including genetic main effects and genotype × environment (GE) interaction effects were analyzed for linolenic acid content (LLAC) and erucic acid content (EAC) in rapeseed using unconditional and conditional genetic models for diploid seed quantitative traits. The results from unconditional analysis revealed that GE effects were important for both traits during seed development. Also the higher magnitude of embryo effects at different developmental times indicated that seed selection would be useful for improving these quality traits in rapeseed. The conditional analysis showed that new expression of genes from maternal and embryo genetic systems occurred during different developmental stages and their magnitude could be influenced by the GE effects. The total narrow-sense heritability was high for both LLAC and EAC, with general heritability being more prominent for EAC at 15 days (32.87 %), 29 days (31.44 %), 36 days (60.55 %) and 43 days (76.45 %), and GE interaction heritability dominating for LLAC at 22 days (40.57 %), 29 days (60.91 %), 36 days (63.83 %) and 43 days (47.45 %) after flowering, respectively. Phenotypic and genotypic correlations for both traits were positive among some pairwise developmental times indicating that genes expressed at these times complemented each other to improve LLAC or EAC in rapeseed. Significant negative correlation between LLAC and EAC at most developmental times indicated that it may not be possible to simultaneously reduce the contents of both traits by indirect selection.
Similar content being viewed by others
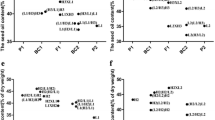
Avoid common mistakes on your manuscript.
Introduction
Rapeseed oil is one of the most important products of rapeseed and is ranked third behind palm and soybean oil (Zhang and Zhou 2006). The utility of oil for industrial or commercial purpose is determined by the oil quality which in turn is guided by the fatty acid profile. The major fatty acid constituents in rapeseed are: Palmitic (C16:0), oleic (C18:1), linoleic (C18:2), linolenic (C18:3), eicosenoic (C20:1) and erucic acid (C22:1). Modifications in the fatty acid composition have been achieved through conventional breeding, chemical mutagenesis and molecular techniques (Miguel and Browse 1995). Although rapeseed oil has several industrial applications, its primary use is in edible products such as salad and cooking oil, margarine, and shortening (Harvey and Downey 1964). As compared to other vegetable oils rapeseed oil contained substantial amounts of erucic acid (22:1) and glucosinolates which are considered anti- nutritional for humans and animals (Lühs and Friedt 1994). Nutritional and end use requirements spawned international efforts to genetically eliminate these undesirable constituents from oilseed Brassica (Pandey et al. 2013). The first notable change in this regard was the decrease in erucic acid content (EAC) in rapeseed oil from around 40 to 0 % of the total fatty acid (FA) composition. Besides erucic acid, rapeseed oil also contained 8–12 % linolenic acid which is easily oxidized to cause an off flavor and odour of the oil, resulting in a shortened shelf life. To reduce the linolenic acid content (LLAC) partial hydrogenation of rapeseed oil is usually followed which results in the production of trans fatty acids that are considered detrimental to human health. The nutritional quality of rapeseed oil can be further improved by increasing the levels of the dietary essential linoleic acid and by decreasing the levels of linolenic acid. Therefore, the development of rapeseed cultivars with lower C18:3 is an important breeding objective.
The genetic studies carried out on LLAC have revealed that it is controlled by embryo or maternal genotype (Kondra and Stefansson 1970; Thomas and Kondra 1973; Bartkowiak-Broda and Krzymanski 1983; Pleines and Friedt 1989), with significant GE interaction effects particularly by temperature during seed development (Tremolieres et al. 1982; Pleines and Friedt 1988). Stefansson (1983) and Rucker and Robbelen (1996) found C18:3 of rapeseed were controlled by minor genes. The results from Chen and Beversdorf (1990) showed that the segregation of C18:3 were fit for a three-locus additive model. This model was further confirmed by the detection of three unlinked QTLs using RAPD markers which individually accounted for 32, 14 and 5 % of the phenotypic variation in LLAC (Somers et al. 1998). Genetic engineering methods have recently been used to modify the FA profile by lowering the LLAC. The low LLAC B. napus accession ‘Stellar’ (3 % C18:3) was previously obtained through chemical mutagenesis of a conventional type rapeseed (around 10 % C18:3) (Auld et al. 1992). Studies carried out at gene level on these mutants have shown that two loci L1 and L2 corresponding to the FAD3 genes control LLAC in rapeseed (Jourdren et al. 1996; Barret et al. 1999). Narrow-sense heritability values as high as 75 % has been found for LLAC in soybean seeds (Fehr et al. 1992; Gesteira et al. 2003). In rapeseed the narrow sense heritability for LLAC at maturity was reported to be 41.8 % (Zhang et al. 2004).
The EAC of B. napus seed oil is under embryo genotype control (Harvey and Downey 1964), with evidences indicating the trait to be controlled by two genes with multiple alleles acting in an additive manner (Kondra and Stefansson 1965; Jonsson 1977; Pandey et al. 2013). But Shi et al. (2003) indicated the EAC trait in B. napus to be controlled mainly by maternal and cytoplasmic genetic and their GE interaction effects, with the embryo effects showing less importance. QTL mapping studies have revealed the erucic acid trait to be controlled by two loci located on two independent linkage groups (Jourdren et al. 1996; Gupta et al. 2004). But Burns et al. (2003) detected three QTLs linked to erucic acid trait using an intervarietal set of B. napus substitution lines. High maternal and cytoplasmic heritabilities were reported for this trait in rapeseed (Shi et al. 2003).
The accumulation of nutrients in seeds starts immediately after embryo development and continues until maturity. This process involves a complex genetic regulation mechanism where different sets of genes get turned on and off depending on the tissue specific requirement. Therefore the study of genetic effects carried out at maturity cannot predict the genetic changes occurring at specific developmental times. Also unlike the plant agronomic traits, seed quality traits can be simultaneously affected by the genetic and GE interaction effects from diploid embryo nuclear genes, cytoplasmic genes and diploid maternal plant nuclear genes. The cytoplasmic genetic activity results from the expression of genes located in the chloroplast and mitochondria. Chloroplast is the site of nutrient synthesis and the energy required for this process is provided by mitochondria. So both these systems play key role in determining the final nutrient status of developing seed. The maternal and embryo nuclear genes are involved in transferring assimilates to developing seed and seed growth respectively. Until now no literature is available on developmental control of genetic effects for linolenic acid and erucic acid contents in rapeseed. The present study was conducted to evaluate the temporal variation of genetic effects and GE interaction effects for embryo, cytoplasm and maternal plant contributions to LLAC and EAC, to estimate the narrow-sense heritabilities, predict the temporal correlation between the genes expressed from the different genetic systems and to estimate the correlation between the two traits during seed development.
Materials and methods
Plant material, field design and cultivation
A diallel mating design without reciprocal crosses was investigated in 2 years, 2006/2007 and 2007/2008. For the experiment, nine cultivars of Brassica napus L.: Youcai 601, Double 20-4, Huashuang 3, Gaoyou 605, Zhongyou 821, Eyouchangjia, Zhong R-888, Tower and Zheshang 72 were used. The parents are a result of random selection from a reference population and so their cytoplasmic sources were all different. Some of these cultivars are hugely popular and widely cultivated in China. The seeds of nine parents and their F1 progenies were sown on 9 October 2006 and 13 October 2007, respectively, at the experimental farm of Zhejiang University (120°11′27′′E, 30°16′28′′N, semitropical climate, silty loamy soil). The experiment was laid out in a randomized block design with two field replications. Flowers that opened in the same day were counted and recorded. To prevent cross pollination the selected inflorescence were covered with photic bags prior to anthesis. To minimize side effects, seed samples of both parents and of F2 s on F1 plants were harvested from 12 plants in the middle part of each plot. Subsequent seed samples were categorized into five developmental times based on their harvesting date after fertilization (15, 22, 29, 36 and 43 days (d) after flowering (DAF)). The sample size for 15 days comprised of around 50 anthotaxys followed by 45 for 22 days, 30 for 29 days, 20 for 36 days and 15 for 45 days, respectively. The F1 seed samples for each developmental time in both years were obtained by crossing females to males during the same growing season using hand emasculation.
Experimental analysis method for LLAC and EAC
LLAC and EAC (mg/g) at five different developmental times were analyzed by near-infrared reflectance spectroscopy on NIRSystems model 5000 instrument (NIRSystems, Inc., Silver Spring, MD, USA). The NIR equations for LLAC and EAC of rapeseed were developed with coefficients of determination (R 2) of 0.77 and 0.91, and standard errors of calibration as low as 7.08 and 24.4 mg/g respectively. Samples from all five developmental times from each parent, F1 and F2 in both years were measured with two replications. For developing the calibration equation, an additional 210 samples from five developmental stages were included and the contents of LLA and EA were analyzed using gas chromatography (Japan, Model GC-9A). For more details please refer Variath et al. (2010).
Statistical analysis methods
The genetic model used is an extension of the diploid plant seeds method for the analysis of embryo, cytoplasmic and maternal effects by including GE interaction effects for quantitative traits (Zhu and Weir 1994a, b; Zhu 1996), while the conditional genetic model was used for carrying out time dependent measures at different special developmental stages (Zhu 1995). The phenotypic mean (Y hijkl ), for a diallel mating design from a set of inbred lines, of mating type k from maternal line i and paternal line j in block l of environment h can be partitioned as,
where μ is population mean, fixed; E h environmental effect, fixed; G ijk genetic main effect with components of embryo additive (Ao) and dominance (Do) effects, cytoplasmic effect (C), maternal additive (Am) and dominance (Dm) effects; GE hijk genotype × environment interaction effect with components of embryo additive interaction (AoE) and dominance interaction (DoE) effects, cytoplasmic interaction effect (CE), maternal additive interaction (AmE) and dominance interaction (DmE) effects, random; Bl(h) the block effect, random; e hijkl residual effect, random.
Partitioning of G ijk and GE hijk can be expressed according to different generations:
Parent line P i (k = 0)
For the unconditional analysis, genetic effects were defined as accumulated effects of genes expressed from flowering (0) to a particular time (t) in developmental period of rapeseed (0→t). The unconditional phenotypic variance (V P(t)) was comprised of genetic main variances (V G(t)), GE interaction variances (V GE(t)) and residual variance (V e(t)). The V G(t) included the components of embryo additive main variance (V Ao(t)), embryo dominance main variance (V Do(t)), cytoplasmic main variance (V C(t)), maternal additive main variance (V Am(t)) and maternal dominance main variance (V Dm(t)). The V GE(t) was partitioned into embryo additive interaction variance (V AoE(t)), embryo dominance interaction variance (V DoE(t)), cytoplasmic interaction variance (V CE(t)), maternal additive interaction variance (V AmE(t)) and maternal dominance interaction variance (V DmE(t)). The V P(t) was partitioned as follows:
For the conditional analysis, genetic effects were defined as developmental stage effects of new expression of genes activated from a particular time (t−1) to another particular time (t) (t−1→t). The conditional phenotypic variance (V P(t|t−1)) was comprised of conditional genetic main variances (V G(t|t−1)), conditional GE interaction variances (V GE(t|t−1)) and conditional residual variance (V e(t|t−1)). The V G(t|t−1) included the components of conditional embryo additive main variance (V Ao(t|t−1)), conditional embryo dominance main variance (V Do(t|t−1)), conditional cytoplasmic main variance (V C(t| t−1 )), conditional maternal additive main variance (V Am(t| t−1 )) and conditional maternal dominance main variance (V Dm(t| t−1 )) while the V GE(t| t−1 ) was partitioned into conditional embryo additive interaction variance (V AoE(t| t−1 )), conditional embryo dominance interaction variance (V DoE(t|t−1 )), conditional cytoplasmic interaction variance (V CE(t| t−1 )), conditional maternal additive interaction variance (V AmE(t|t− 1)) and conditional maternal dominance interaction variance (V DmE(t| t−1 )). The V P(t| t−1 ) was partitioned as follows:
The total narrow-sense heritability (h 2 = (V G + V GE )/V P ) was partitioned into general genetic heritability (h 2 G = V G /V P ) from genetic main effects and interaction heritability (h 2 GE = V GE /V P ) from GE interaction effects. The h 2 G was comprised of the components of embryo general heritability (h 2 Go = V Ao /V P ), cytoplasmic general heritability (h 2 C = V C /V P ) and maternal general heritability (h 2 Gm = V Am /V P ) The h 2 GE had components of embryo interaction heritability \( (h_{GoE}^{2} \, = \,V_{AoE} /V_{p} ), \) cytoplasmic interaction heritability (h 2 CE = V CE /V P ) and maternal interaction heritability \( (h_{GmE}^{2} = V_{AmE} /V_{P} ) \). The partitioning for the total narrow-sense heritability is
The genotypic correlation coefficient was comprised of genetic main correlation (r G ) including embryo additive correlation (r Ao ), embryo dominance correlation (r Do ), cytoplasm correlation (r C ), maternal additive correlation (r Am ), maternal dominance correlation (r Dm ); and the GE interaction correlations (r GE ) including embryo additive interaction correlation (r AoE ), embryo dominance interaction correlation (r DoE ), cytoplasm interaction correlation (r CE ), maternal additive interaction correlation (r AmE ), and maternal dominance interaction correlation (r DmE ).
The Jackknife resampling methods (Miller 1974; Zhu and Weir 1996) were applied by sampling generation means of entries to derive the standard errors of estimated unconditional or conditional variance, heritability and correlation coefficient components.
Results
Unconditional variance components analysis at different developmental times
The unconditional variance components for the FAs—linolenic acid and erucic acid of rapeseed at five developmental times (15, 22, 29, 36 and 43 DAF) are presented in Table 1. It revealed that both traits, at different developmental times were simultaneously controlled by the genetic main effects and GE interaction effects.
Comparing the genetic main effects (V G(t)) with GE interaction effects (V GE(t)), the LLAC of rapeseed was mainly affected by GE interaction effects (V GE(t)) at different developmental times, except at 15 DAF where the V G(t) was 52.02 % (Table 1). Similarly EAC at 15, 22 and 29 DAF was mainly affected by GE interaction effects (V GE(t)) with values of 62.72, 63.70, 58.71 % and by genetic main effects (V G(t)) with 67.10 and 83.29 % at 36 and 43 DAF, respectively. Thus, the variation for LLAC or EAC at different seed developmental times was mainly dependent on the environment. No genetic main effect was observed for LLAC at 29 DAF and this period was totally influenced by GE interaction effects in present experiment.
Among the embryo, cytoplasmic, and maternal plant genetic systems for LLAC the analyzed unconditional results showed that the total of maternal and cytoplasmic main effects [(V Am(t) + V Dm(t) + V C(t))/V G(t)] were most important at 15 and 22 DAF as they accounted for 66.89 and 61.93 % of total genetic variance, while the embryo main effects [(V Ao(t) + V Do(t))/V G(t)] with values of 50.29 and 58.47 % were larger at 36 and 43 DAF respectively. Except for 15 DAF, no cytoplasmic effect (V C(t)) was detected for LLAC at the other developmental times. For the GE interaction variance components the cumulative embryo interaction effects (V AoE(t) + V DoE(t)) at 22, 29 and 36 DAF were 54.89, 54.17 and 56.65 %, respectively. Thus the expression of embryo genes during these developmental times was more easily influenced by the environment. The additive variances increased progressively from 35.01 % at 15 DAF to 73.18 % at 43 DAF. The dominance effects on the other hand ranged from 42.78 to 15.18 %. This suggested that the additive effects of the genes expression from different genetic systems were more crucial for controlling the LLAC. For the cumulative embryo or maternal effects, the total embryo effects accounted for most developmental times except for 15 DAF.
For EAC, the unconditional analysis results showed that the embryo main effects [(V Ao(t) + V Do(t))/V G(t)] were most important at 29, 36 and 43 DAF accounting for 69.33, 69.07 and 77.31 % of V G(t), while the maternal main effects [(V Am(t) + V Dm(t))/V G(t)] with 57.52 and 72.65 % were larger at 15 and 22 DAF, respectively. Cytoplasmic effects (V C(t)) were observed at 15, 36 and 43 DAF and could not be ignored for the improvement of EAC performance in rapeseed. Among the GE interaction effect components for unconditional analysis, the cumulative maternal interaction effects (V AmE(t) + V DmE(t)) were larger at 29 and 36 DAF followed by embryo interaction effects (V AoE(t) + V DoE(t)) at 15 and 22 DAF and then by cytoplasmic interaction effect (V CE(t)) at 43 DAF. The higher additive variances 55.31 ~ 91.65 % of (V G(t) +V GE(t)) detected in the unconditional analysis revealed that dominance effects were not prominent for EAC variation. For the cumulative embryo or maternal effects, the total embryo effects accounted for 22, 29, 36 and 43 days with 50.21, 57.59, 60.39 and 66.87 % of total genetic effects, while the total maternal effects with 45.52 % was more prominent at 15 days, respectively.
Small but significant residual variances (V e(t)) were detected at all developmental times for both LLAC and EAC, which could result from sampling errors or micro-environment effects.
Conditional variance components analysis at different developmental stages
Unconditional variance analysis can predict the changes of accumulated genetic effects expressed from flowering to developmental time t (0 → t), but in order to understand stage specific variation (t−1 → t) for genetically controlled traits, a conditional approach is required. Table 2 shows the variance components for LLAC and EAC estimated using the conditional method.
The analysis results indicated the existence of diversity for the magnitude or type of conditional genetic effects among the developmental stages. The new expression of genes for LLAC was highest for 1–15 days (20.76) after flowering, while for EAC the maximum expression was detected during the period from 23 to 29 days (139.47) after flowering.
For LLAC except for the initial period (1–15 days), the new expression of genes was mainly influenced by the GE interaction effects as they accounted for 80.76, 90.20, 81.88 and 59.22 % of total genetic variance at 16–22, 23–29, 30–36 and 37–43 days, respectively. Hence, the new expression of genes at most of the developmental stages of rapeseed was more sensitive to the environment. The conditional genetic effects revealed that at different developmental stages expression of both embryo and maternal nuclear genes were more prominent especially for the stages 1–15, 16–22, 30–36 and 37–43 days. Conditional cytoplasmic variance was detected during 1–15 and 23–29 days and therefore could be important in LLAC improvement. For the conditional analysis, the net embryo effects including embryo additive and dominance effects and their GE interaction effects accounted for 52.48, 46.00, 54.32 and 43.83 % of total genetic effects at 16–22, 23–29, 30–36 and 37–43 days, respectively. Also, the larger net additive and cytoplasmic variances (57.22–80.53 %) observed in the conditional analysis indicated that the genes mostly acted additively to improve the performance of LLAC in rapeseed.
Conditional analysis for EAC revealed that the net genetic effects from new activation of genes was mainly controlled by the GE interaction effects especially for the developmental stages 1–15, 16–22, 23–29, and 30–36 days respectively, while the genetic effects were important at 37–43 days. Among the different conditional genetic effect components, the new expression of maternal nuclear genes assumed significance, except for the stages 23–29 and 37–43 days where the embryo effects were larger with values of 69.48 and 55.17 %, respectively. The net cytoplasmic effects were mostly absent except for the stages 1–15 and 37–43 days, respectively. Also the net maternal effects including maternal additive and dominance effects and their GE interaction effects accounted for 45.52, 52.13 and 58.55 % of total genetic effects at 1–15, 16–22 and 30–36 days, respectively. Higher net additive and cytoplasmic variances 55.31–77.14 % of (V G(t|t−1) + V GE(t|t−1))) were observed and revealed that the net dominance effects were not prominent for EAC variation.
According to the developmental genetic theory, numerous quantitative polygenes might be selectively and sequentially expressed during seed development. In the current study new gene expressions was detected for V C(29 days|22 days), V AmE(22 days|15 days) and V DmE(43 days|36 days) for LLAC, when compared with the unconditional analysis. For EAC, no genetic activity was found for V Ao(22 days|15 days), V Ao(36 days|29 days), V C(36 days|29 days). So the corresponding significant unconditional genetic effects detected in Table 1 for these developmental times might be attributed to the durative expression of activated genes at the previous developmental stages.
Although significant residual variances were found for all developmental stages for both LLAC and EAC, it was suggested that both traits were mainly affected by genetic effects from different genetic systems because of the small values of estimated V e(t|t−1).
Analysis of heritability at different developmental times
The narrow-sense heritability h 2(t) estimates for the FAs—LLAC and EAC are depicted in Tables 3 and 4.
For linolenic acid, the total heritability for the developmental times 15, 22, 29, 36 and 43 DAF were 52.02, 54.94, 60.91, 63.83 and 79.35 %, which indicated that the inheritance for LLAC was considerably stronger throughout generations. The general heritability values at different developmental times were 52.02, 14.37, 0.00, 0.00 and 31.90 % of total heritability, while those of interaction heritability components were 0.00, 40.57, 60.91, 63.83 and 47.45 %, respectively. These results, therefore clearly indicated that selection efficiency for LLAC was more likely to be influenced by the environment as the interaction heritabilities were larger for most developmental times, except for 15 DAF. The sum of embryo general and interaction heritability contributed 28.28, 38.53, 30.97 and 35.51 % of total heritability for the developmental times 22, 29, 36 and 43 DAF and were found to be larger than either cytoplasm or maternal heritability. Thus, it was suggested that decreasing LLAC of rapeseed would be more efficient when selection is based on seeds rather than on maternal plants in early generations. From the interaction heritabilities, it was found that the embryo interaction heritabilities were more easily influenced by environmental conditions at 22, 29 and 36 DAF, while the maternal interaction heritabilities were larger at 43 DAF, respectively. No interaction heritability was found at 15 DAF while no general heritabilities were observed at 29 and 36 DAF, respectively. This suggested that heritability at 15 DAF was relatively stable while at 29 and 36 DAF the heritabilities were unstable and more likely to be influenced by the environmental factors.
For erucic acid, the total heritability for the developmental times 15, 22, 29, 36 and 43 DAF were 48.78, 68.02, 60.48, 82.65 and 86.58 %, respectively (Table 4). Out of these, the general heritabilities contributed 32.87, 27.76, 31.44, 60.55 and 76.45 %, while those of interaction heritability components were 15.90, 40.26, 29.04, 22.10 and 10.13 %, respectively for the different developmental times. Thus, the heritability for EAC was mostly affected by the genotype and the selection efficiency was least likely to be influenced by the environment, except for 22DAF. Also, selection advance could be expected in early generations at different environments. The sum of embryo general and interaction heritabilities was larger than either cytoplasm or maternal heritability at all developmental times, except 15 DAF. Results from interaction heritabilities, revealed that embryo heritability was easily influenced by the environment at most developmental times except at 36 and 43 DAF where the maternal or cytoplasmic interaction heritability was larger. Also, as compared to embryo or maternal heritability cytoplasmic heritability was more stable at different environments because it was not found at most developmental times.
Genetic correlation components analysis at different developmental times
The analysis of genotypic correlation components could reveal the genetic relationship for seed traits between different seed developmental times. Since the expression of genes from different genetic systems consisted of genetic main effects and GE interaction effects, the genotypic correlations could also be further partitioned into genetic main correlation and GE interaction correlation. The value of correlation components for the FAs – LLA and EA among developmental times is summarized in Tables 5 and 6.
For linolenic acid, significant phenotypic or genotypic correlations were observed among the pairwise development times of 15 and 43 days (0.113* and 0.113 *), 22 and 29 days (−0.145** and −0.163*), 29 and 43 days (0.215** and 0.231**) or 36 and 43 days (0.133** and 0.127**) after flowering, respectively (Table 5). For the different genetic correlation components, the results indicated that the genetic main correlations were not significant among most pairwise developmental times. Among the GE interaction correlation components, embryo dominant interaction correlation (r DoE ) followed by embryo additive interaction correlation (r AoE ) was more important as most of the correlations between different developmental times were significant. For r DoE the significant values were mostly positive among the different pairwise developmental times, while that for r AoE was mostly negative except between 29 and 43 days (0.679**) or 36 and 43 days (0.326**) after flowering respectively. Cytoplasmic interaction correlation (r CE ), maternal additive interaction (r AmE ) and maternal dominant interaction (r DmE ) correlations were mostly not detected. The presence of non-significant genetic main correlations and significant GE interaction correlations among the pairwise developmental times indicated that LLAC in rapeseed was easily influenced by the prevailing environment. Significant r e was observed between 15 and 22 days (0.189**), 15 and 36 days (−0.138**), 15 and 43 days (0.123+) or 36 and 43 days (0.201**) respectively which could be due to sampling errors or microenvironment influence.
The analysis of genetic correlation between different development times for EAC indicated significant positive or negative relationships for genes expressed during seed development (Table 6). Except between 15 and 22, 15 and 29, 15 and 36 or 15 and 43 days after flowering, significantly positive phenotypic and genotypic correlations were noted for all other developmental times. Among the genetic correlation components from the different genetic systems, maternal additive interaction correlation (r AmE ) was significant for most pairwise seed development times. Also, most of the observed r AmE correlations were significantly negative which indicated that environmental changes can affect EAC during seed development. Besides embryo additive interaction correlations (r AoE ), maternal additive (r Am ), embryo additive (r Ao ) and embryo dominant interaction (r DoE ) correlations were also relevant among different pairwise times. No r CE or r Dm was found between the different developmental times. Significant r e especially between 15 and 29 days (0.113), 15 and 36 days (−0.258**) or 15 and 43 days (0.107) after flowering, indicated that sampling errors or microenvironments could influence the relationship.
Correlation between LLAC and EAC at different developmental times
The correlation analysis presented in Table 7 revealed the existence of significant negative r P (−0.142**) and r G (−0.148**) at 22 DAF and significant negative r G at 36 DAF (−0.063+). The r AoE and r DoE was significantly negative at 22 and 29 DAF while positive correlation was observed at 36 DAF. Cytoplasmic correlation (r C and r CE ) except for 15 DAF, was not found to be of any significance between the two traits for other developmental times. Significant negative r Ao and r Do was found at 43 DAF after flowering. Most of the significant r Am and r DmE between the two traits was negative except for a positive r Am at 43 DAF and positive r DmE at 15 DAF. The correlation values between the two traits were mostly weak.
Discussion
Seed quality traits unlike the agronomic traits have a very complex expression pattern as it requires the coordinated and simultaneous expression of genes from multiple genetic systems. A few major genes and numerous polygenes are involved during this process. Also being quantitative in nature the expression of these genes is controlled by the environment. Quantitative trait loci (QTL) mapping studies have identified numerous loci involved in the control of LLAC (Jourdren et al. 1996; Barret et al. 1999; Burns et al. 2003) and EAC in rapeseed (Jourdren et al. 1996; Burns et al. 2003; Gupta et al. 2004). Inheritance and genetic basis of EAC and LLAC in mature seeds has been widely researched in rapeseed. During seed development, many genes, with or without environmental influence, involved in different biochemical pathways are expressed. While some genes are expressed continually, some are expressed only at specific stages and some others are brokenly expressed at different developmental stages. In the present study it was clarified that both LLAC and EAC are quantitative traits and their contents in the seed are determined by the environmental conditions prevailing during seed development. Canvin (1965) reported that the amount of the more highly unsaturated fatty acids decreased as the temperature was increased in rape, sunflower and flax. For the different developmental times the embryo effects were more prominent and so seed selection could be useful for improving these quality traits. The embryo control of FA traits in rapeseed has also been suggested by other researchers (Harvey and Downey 1964). However, the results are in contrast with Shi et al. (2003) who found the EAC in mature rapeseed to be maternally controlled. The detection of different conditional genetic variances during seed development suggests that genes controlling rapeseed LLAC and EAC were expressed differently during individual stages of the development period. Dissimilar category and activities of isozyme were observed during development of embryo and endosperm in maize, and had significant correlation with seed weight and volume (Yang and Zeng 1984). Expression data of 8095 genes that are activated during various periods of B. napus seed development have been obtained through cDNA microarray hybridization and are available in the RAPESEED database (Wu et al. 2008).
Heritability analysis is an important parameter for carrying out selection and for predicting trait transferability to the next generation. The narrow-sense heritability analysis for LLAC and EAC performed at different developmental times revealed that heritability was high for both traits at all times, and for both traits single seed selections could be employed in breeding programs due to their higher embryo heritability values. In the case of LLAC, no GE interaction heritability was found at 15 DAF, but at later stages especially 29 and 36 DAF a strong GE component was noted. Since genetic activities during seed development depend on the genes that are getting expressed at that particular stage/time, understanding the heritability of the trait under different time intervals allows us to make a more accurate prediction of behavior of the trait in subsequent generations and take appropriate decisions for trait improvement. Also, in our study it was found that selection could be carried out in early generations because of their high net additive effects. High broad sense heritabilities have been reported for LLAC (Mollers and Schierholt 2002) and for EAC in B. napus (Paisan and Thitiporn 2012).
Correlation analysis between the genes expressed during different seed developmental times could be useful in predicting the final outcome of a trait. If a gene expressed during a particular stage of seed development shows a positive correlation with the genes expressed at other stages then it can be assumed that there will be an overall improvement in that particular trait. In present study significant positive phenotypic and genotypic correlations were noted at some pairwise developmental times for both traits. However, the correlation values were weak especially among the initial developmental times for both traits. Accumulation of seed nutrients occurs after the seed development and most of the fatty acids synthesized in the initial stages are utilized in membrane formation (Neidleman 1987; Heppard et al. 1996). So it is possible that the genes that are expressed during initial development might be different from those that are expressed at later stages. In that case a weak correlation would be expected in the initial developmental times and stronger correlation would be expected towards seed maturity. For linolenic acid as compared to GE interaction correlation most of the genetic main correlation was non-significant among pairwise developmental times, which might be due to the higher prevalence of environmental factors in determining linolenic acid content in rapeseed (Tremolieres et al. 1982; Pleines and Friedt 1988).
In quality breeding program, breeders are more interested with the overall improvement of multiple traits during selection. Analysis of genetic correlation components could reveal the genetic relationships among the different quality traits of rapeseed. Complex relations exist among some quality traits of rapeseed because of genetic linkage and the pleiotropic function of genes, causing difficulties in selecting better genotypes from subsequent generations. Genotypic correlation could eliminate the interference caused by random error, and is an important statistical parameter that is superior to phenotypic correlation (Chen et al. 2011a). In the present experiment, most components of genetic main and GE interaction correlation between LLA and EA contents were significantly negative at various developmental times, which indicated that the activation and expression of genes for both quality traits at different seed growth periods had close relationship (Chen et al. 2011b). Molecular markers significantly associated with both erucic and linolenic acid levels were detected in rapeseed. This marker was predicted to be linked in repulsive phase with previously mapped erucic acid genes (Rajcan et al. 1999). In contrast Mandal et al. (2002) obtained a significant positive correlation between linolenic acid and erucic acid content of mature rapeseed.
The present study provides new understanding on the dynamic changes of genetic effect components when conditioned on variability at earlier developmental times. Using this technique, it is possible to detect when significant episodes in the generation of new variation might occur. Then using the tools of molecular biology, markers can be developed to detect the genes or the loci that are explicitly expressed at a particular stage of seed development.
References
Auld DL, Heikkinen MK, Ericksson DA, Sernyk JL, Romero JE (1992) Rapeseed mutants with reduced levels of polyunsaturated fatty acids and increased levels of oleic acid. Crop Sci 32:657–662
Barret P, Delourme R, Brunel D, Jourdren C, Horvais R, Renard M (1999) Low linolenic acid level in rapeseed can be easily assessed through the detection of two single base substitution in fad3 genes. In: Proceeding of the 10th international rapeseed congress, Canberra, pp 26–29
Bartkowiak-Broda I, Krzymanski J (1983) Inheritance of C18 fatty acid composition in seed oil of zero erucic winter rape Brassica napus L. In: Proceeding of international rapeseed conference 1, Paris (France), pp 477–482
Burns MJ, Barnes SR, Bowman JG, Clarke MHE, Werner CP, Kearsey MJ (2003) QTL analysis of an intervarietal set of substitution lines in Brassica napus: (i) Seed oil content and fatty acid composition. Heredity 90:39–48
Canvin DT (1965) The effect of temperature on the oil content and fatty acid composition of the oil from several oil seed crops. Can J Bot 43:63–69
Chen JL, Beversdorf WD (1990) Fatty acid inheritance in microspore-derived population of spring rapeseed (Brassica napus L.). Theor Appl Genet 80:465–469
Chen GL, Wu JG, Variath MT, Shi CH (2011a) Timing of gene expression from different genetic systems in shaping leucine and isoleucine contents of rapeseed (Brassica napus L.) meal. J Genetics 90:1–10
Chen GL, Wu JG, Variath MT, Yang ZW, Shi CH (2011b) Analysis of embryo, cytoplasmic and maternal genetic correlations for seven essential amino acids in rapeseed meal (Brassica napus L.). J Genetics 90:67–74
Fehr WR, Welke GA, Hammond EG, Duvick DN, Cianzio SR (1992) Inheritance of reduced linolenic acid content in soybean genotypes A16 and A17. Crop Sci 32:903–906
Gesteira AS, Schuster I, Jose IC, Piovesan ND, Viana JMS, de Barros EG, Moreira MA (2003) Biometrical analyses of linolenic acid content of soybean seeds. Genet Mol Biol 26:65–68
Gupta V, Mukhopadhyay A, Arumugam N, Sodhi YS, Pental D, Pradhan AK (2004) Molecular tagging of erucic acid trait in oilseed mustard (Brassica juncea) by QTL mapping and single nucleotide polymorphisms in FAE1 gene. Theor Appl Genet 108:743–749
Harvey BL, Downey RK (1964) The inheritance of erucic acid content in rapeseed (Brassica napus L.). Can J Plant Sci 44:104–111
Heppard PE, Kinney AJ, Stecca KL, Miao GH (1996) Developmental and growth temperature regulation of two different microsomal ω−6 desaturase genes in soybeans. Plant Physiol 110:311–319
Jonsson R (1977) Erucic acid heredity in rapeseed (Brassica napus L. and Brassica campestris L.). Hereditas 86:159–170
Jourdren C, Barret P, Horvais R, Foisset N, Delourme R, Renard M (1996) Identification of RAPD markers linked to the loci controlling erucic acid level in rapeseed. Mol Breed 2:61–71
Kondra ZP, Stefansson BR (1965) Inheritance of erucic and eicosenoic acid content of rapeseed oil (Brassica napus). Can J Genet Cytol 7:505–510
Kondra ZP, Stefansson BR (1970) A maternal effect on the fatty acid composition of rapeseed oil Brassica napus. Can J Plant Sci 50:345–346
Lühs W, Friedt W (1994) Major oil crops. In: Murphy DJ (ed) Designer oil crops. VCH, New York, pp 5–71
Mandal S, Yadav S, Singh R, Begum G, Suneja P, Singh M (2002) Correlation studies on oil content and fatty acid profile of some cruciferous species. Genet Resour Crop Evol 49:551–556
Miguel FM, Browse JA (1995) Molecular biology of oilseed modification. INFORM 6:108–111
Miller RG (1974) The Jackknife: a review. Biometrika 61:1–15
Mollers C, Schierholt A (2002) Genetic variation of palmitate and oil content in a winter oilseed rape doubled haploid population segregating for oleate content. Crop Sci 42:379–384
Neidleman SL (1987) Effects of temperature on lipid unsaturation. Biotechnol Genet Eng Rev 5:245–268
Paisan L, Thitiporn M (2012) Inheritance of erucic acid, glucosinolates and oleic acid contents in rapeseed (Brassica napus L.). J Northeast Agric Univ 19:3–10
Pandey S, Kabdal M, Tripathi MK (2013) Study of inheritance of erucic acid in Indian mustard (Brassica juncea L. Czern & Coss). Octa J Biosci 1:77–84
Pleines S, Friedt W (1988) Breeding for improved C18-fatty acid composition in rapeseed (Brassica napus L.). Fat Sci Technol 90:167–171
Pleines S, Friedt W (1989) Genetic control of linolenic acid concentration in seed oil of rapeseed (Brassica napus L.). Theor Appl Genet 78:793–797
Rajcan I, Kasha KJ, Kott LS, Beversdorf WD (1999) Detection of molecular markers associated with linolenic and erucic acid levels in spring rapeseed (Brassica napus L.). Euphytica 105:173–181
Rucker B, Robbelen G (1996) Impact of low linolenic acid content on seed yield of winter oilseed rape (Brassica napus L.). Plant Breed 115:226–230
Shi CH, Zhang HZ, Wu JG, Li CT, Ren YL (2003) Genetic and genotype × environment interaction effects analysis for erucic acid content in rapeseed (Brassica napus L.). Euphytica 130:249–254
Somers DJ, Friesen KRD, Rakow G (1998) Identification of molecular markers associated with linoleic acid desaturation in Brassica napus. Theor Appl Genet 96:897–903
Stefansson B (1983) High and low erucic acid rapeseed oil. In: Kramer JKG, Sauer FD, Pigden WJ (eds) The development of improved rapeseed cultivars. Academic Press, New York, pp 143–159
Thomas PM, Kondra ZP (1973) Maternal effects on the oleic, linoleic, and linolenic acid content of rapeseed oil. Can J Plant Sci 53:221–225
Tremolieres A, Dubacq JP, Drapier D (1982) Unsaturated fatty acids in maturing seeds of sunflower and rape: regulation by temperature and light. Phytochemistry 21:41–45
Variath MT, Wu J, Zhang L, Shi C (2010) Analysis of developmental genetic effects from embryo, cytoplasm and maternal plant for oleic and linoleic acid contents of rapeseed. J Agric Sci 148:375–391
Wu GZ, Shi QM, Niu Y, Xing MQ, Xue HW (2008) Shanghai RAPESEED database: a resource for functional genomics studies of seed development and fatty acid metabolism of Brassica. Nucleic Acids Res 36:1044–1047
Yang TX, Zeng MQ (1984) The changes of isozyme, soluble protein and the dry matter accumulation during endosperm and embryo development in Maize. Acta Phytophysiol Sin 10(1):77–86
Zhang GQ, Zhou WJ (2006) Genetic analyses of agronomic and seed quality traits of synthetic oilseed Brassica napus produced from interspecific hybridization of B. campestris and B. oleracea. J Genetics 85:45–51
Zhang HZ, Shi CH, Wu JG, Ren YL, Li CG, Zhang DQ, Zhang YF (2004) Analysis of genetic effects and heritabilities for linoleic and α-linolenic acid content of Brassica napus L. across Chinese environments. Eur J Lipid Sci Technol 106:518–523
Zhu J (1995) Analysis of conditional genetic effects and variance components in development genetics. Genetics 141:1633–1639
Zhu J (1996) Analysis methods for seed models with genotype × environment interaction. Acta Genet Sin 23(1):56–68
Zhu J, Weir BS (1994a) Analysis of cytoplasmic and maternal effects. I. A genetic model for diploid plant seeds and animals. Theor Appl Genet 89:153–159
Zhu J, Weir BS (1994b) Analysis of cytoplasmic and maternal effects. II. Genetic models for triploid endosperms. Theor Appl Genet 89:160–166
Zhu J, Weir BS (1996) Diallel analysis for sex-linked and maternal effects. Theor Appl Genet 92(1):1–9
Acknowledgments
The project was financially supported from Zhejiang Provincial Key Laboratory of Crop Germplasm Resources, Technology Office of Zhejiang Province (No. 2008C22084), Foundation for University Key Teacher by the Ministry of Education of China and by the 151 Program for the Talents of Zhejiang Province.
Author information
Authors and Affiliations
Corresponding author
Rights and permissions
About this article
Cite this article
Variath, M.T., Wu, J.G. & Shi, C.H. Dynamic gene expression analysis of maternal, cytoplasmic and embryo genetic systems for linolenic and erucic acid contents in rapeseed (Brassica napus L.). Euphytica 205, 585–598 (2015). https://doi.org/10.1007/s10681-015-1436-x
Received:
Accepted:
Published:
Issue Date:
DOI: https://doi.org/10.1007/s10681-015-1436-x