Abstract
Coastal ecosystems are the most vulnerable to natural and anthropogenic pressures which should be assessed using various chemical and ecological indicators. Our study aims to provide practical monitoring of anthropogenic pressures related to metal discharges in coastal waters for identifying potential ecological deterioration. The spatial variability of various chemical elements concentrations and their main sources were determined in the surficial sediments of a Mediterranean coastal area submitted to high anthropogenic pressure, the semi-enclosed Boughrara Lagoon located in southeastern Tunisia, by conducting several geochemical and multi-elemental analyses. The grain size and the geochemical analyses both suggested a marine influence of the sediment inputs in the north of the area (near Ajim channel), whereas continental and aeolian features dominated the sedimentary inputs in the southwestern lagoon. This last area was also characterized by the highest concentrations of metals, in particular Pb (4.45–173.33 ppm), Mn (68.45–1469.27 ppm), Cu (7.64–134.26 ppm), Zn (28.74–244.79 ppm), Cd (0.11–2.23 ppm), Fe (0.5–4.9%), and Al (0.7–3.2%). By referring to background crustal values and the contamination factor calculations (CF), the lagoon is considered as highly polluted for Cd, Pb, and Fe (3 < CF < 6). Three possible sources of pollution were identified: phosphogypsum effluents (P, Al, Cu, and Cd), the ex-Pb mine (Pb and Zn), and the cliff weathering and streams input from the red clay quarry (Fe). Furthermore, pyrite precipitation was identified for the first time in the Boughrara lagoon, suggesting the occurrence of anoxic conditions in this lagoon.
Similar content being viewed by others
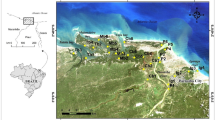
Explore related subjects
Discover the latest articles, news and stories from top researchers in related subjects.Avoid common mistakes on your manuscript.
Introduction
Lagoons are generally considered as the most vulnerable ecosystems in marine coastal environments. With rapid industrialization, anthropogenic activities have indeed increased the amount of toxic chemicals and metals in coastal ecosystems (Hu et al., 2018; Mosbahi et al., 2019; Zaaboub et al., 2015). Without any purification treatment, polluted wastewater is discharged into the coastal environment, possibly leading to the accumulation of pollutants, especially of metals, in the exchangeable fraction of surface coastal sediments. The accumulation of these elements including metals in coastal ecosystems poses a serious threat to human health and the aquatic environment. Metals are characterized by their long residence time allowing to their accumulation and biomagnification in the food chain (Zhao et al., 2019). Metals accumulated in marine coastal environment sediments may result from anthropogenic sources like industrial wastewater, while rock weathering and erosion are considered as natural sources. For instance, data analysis of contaminants distribution in the coastal environment may constitute a crucial tool for predicting and monitoring the status of vulnerable areas and also for the identification of possible sources of contamination (Ferraro et al., 2023; Kükrer et al., 2020).
Recently, various renewed scientific focus in marine pollution indicated that coastal environments were highly contaminated by organic and inorganic pollutants and especially by metals (Pignotti et al., 2018; Çullu et al., 2021; Kükrer et al., 2020; Lin et al., 2021; El Zrelli et al., 2021; Ferraro et al., 2023; Wang et al., 2022). The Mediterranean Sea is considered as the most threatened ecosystem, subject to significant anthropogenic pressure due to the economic and population development. Several recent studies were carried out to evaluate the pollution in various coasts in Tunisia, since they are subject to high anthropogenic pressures (Kharroubi et al., 2012a, b; Zaaboub et al., 2015; Khedhri et al., 2016; El Zrelli et al., 2017; Mna et al., 2017; Gargouri et al., 2018; Naifar et al., 2018; Mosbahi et al., 2019 Keskes et al., 2020; Belkahia et al., 2021; Mna et al., 2021; El Zrelli et al., 2021). However, the extent of pollution by metals is not precisely known in the Boughrara lagoon which is located between the southeastern part of Tunisia and Djerba Island, whereas this semi-enclosed lagoon can represent an accumulation basin of pollution. This lagoon has an important economic function, particularly in fishing and halieutic activities.
Indeed, this lagoon receives various wastes from different sources such as soil mining, aquaculture, fishery, and industry from the Tunisian chemical group (TCG). Industrial wastewater discharged in the Gabes Gulf is advected by the long-shore current oriented towards the south, causing large contamination by phosphogypsum, which can be a source of various chemical elements (Cu, Al, P, Cd…) in the Boughrara lagoon (Amari et al., 1984; Magherbi, 1995; Gargouri et al., 2018).
Phosphorous (P) enrichment of phosphogypsum combined with anoxic conditions due to weak circulation in the lagoon can accelerate the phenomenon of eutrophication, leading to serious environmental problems (El Zrelli et al., 2017; Rabaoui et al., 2017). In this context, citizens of the Boughrara region, especially bathers in summer, have recently observed the appearance of red tides and high fish mortality in the southwestern part of the lagoon, the cause of which remains uncertain.
In addition, metal inputs from the red clay and lead extraction mines upstream of the watersheds could represent other sources of contamination in the lagoon during the flood periods, as well as the erosion of surrounding cliffs. But these potential sources of metal pollutions have not yet been investigated.
Thus, the present study aims to diagnose the state of pollution by various elements like Zn, Pb, Cu, Cd, Fe, Cr, P, Ti, and Ca in the Boughrara lagoon, which will serve as a study model to allow a better ecological risk assessment and help improve resource management in anthropized coastal systems. To this end, we made mineralogical and chemical analyses of 25 surficial sediment samples collected in the western side of the Boughrara lagoon, and we calculated the contamination factors (CF) for each chemical element. Multivariate statistical analyses combined with geostatistical tests assisted with GIS tools were used to further interpret the data. In order to identify the sources of the pollution in the Boughrara lagoon, analyzed surficial sediments were compared with other sites collected in the Boughrara lagoon watershed. These results will contribute to the establishment of a sediment quality baseline and to the management of the local environment.
Material and methods
Study area
The Boughrara Lagoon is located in the southeastern part of Tunisia (Fig. 1), in the Gulf of Gabes. It covers a total surface area of 500 km2 between the coordinates 33°28′–33°45′N and 10°40′–10°57′E. The lagoon has been remained geologically stable since the last 125 ka BP (Rached et al., 2022). The average depth is about 4 m, with a maximum of 16 m in the lagoon center. Water communication between the Mediterranean Sea and the lagoon is mainly assured through the Ajim channel (2.5 km wide) in the northern side, since the passage on the eastern side (6 km wide) is closed by an old Roman road. Both the shape and the bathymetry of the lagoon reduce water circulation causing high rates of salinity (Kharroubi et al., 2012a, b). The Boughrara lagoon is an outlet from the Fjé, Smar, and Bou Hamed streams, whose watershed area is estimated to be of 2394 km2 (Fig. 1). Annual input water into the lagoon is about 11 Mm3 per year, mainly from these main streams (Brahim et al., 2014).
Localization of Boughrara Lagoon and GIS slope map (in degrees) of the southeastern of Tunisia classified into four classes (top right panel), location of surficial sediments stations in the southwestern Boughrara Lagoon, and location of samples collected from Tejra quarry (S1) and from the ex-lead mine (S2) (low right panel)
Sampling procedure
Surface sediments were sampled at 25 sites (Fig. 1) in December 2018 and January 2019 in the middle and the south of the western Boughrara Lagoon, near the three main streams (Fjé, Smar, and Bouhamed; Fig. 1). Sampling sites were chosen because of their geographical locations near the streams, also near the water current flowing from the north and circulating to the south, which carries effluents from industrial and chemical factories. Two additional rock samples were collected in December 2020 in the upper watershed over which the main streams flow: a first sample was collected from the ex-lead mine of Tebaga Mountain and another from the red clay quarry located in the Tejra Esghira Mountain (Figs. 2, 3, and 4) in order to characterize the main sources of sediment input in the southwestern part of Boughrara Lagoon and to assess the origin of the marine pollution by metals.
At each station, the exact location was recorded with a GPS and the top 5 cm of sediments were collected using a gravitational core sampler (Van Veen grab). We carefully rinsed the outside of the sampler with water from the sampling station, in order to remove material that could contaminate the sample during transfer.
Samples were transported directly to the laboratory, and they were oven dried at a temperature < 60 °C to prevent the destruction of the organic matter. Rocks were crushed using an automatic mortar grinder.
Grain size analyses
In order to determine the size distribution of materials, dried samples were passed in dry and wet sieving (using a standard column of AFNOR sieves) to separate the fine (< 63 µm) and coarse (> 63 µm) sediment fractions. We used a decreasing series of sieve meshes as follows: 2000, 1600, 1250, 1000, 800, 630, 500, 400, 315, 250, 200, 160, 125, 100, 80, and 63 µm. Grain size results are given as a percentage of the refusal mass relative to the total mass of the sample, for each diameter. For each sample, the cumulative curve of the fine and coarse fractions of surficial sediments is presented in a semi-logarithmic plot using the particle diameter and the cumulative percentages of the refusal (Folk & Ward, 1957). GRADISAT software version 4.0 was used for grain-size statistical calculations.
Mineralogy
Mineralogical characterization (type and quantification of mineral phases) of the fine fraction was conducted for each sample using X-ray diffractometry. X-ray analyses were performed with a diffractometer (PANALYTICAL X'PERT PRO) using a copper anticathode. The resulting spectra were processed using a high score plus. Many corrections were recommended, followed by smoothing. Peak identification was based on the software database and on the diffraction angle (dƟ) of each mineralogical phase (Lutterotti et al., 1999).
Scanning electron microscopy
Images were taken in the fine fraction using a focused ion beam-scanning electron microscope (SEM) of type FEG-FIB-SEM AURIGA 40 ZEISS. Samples were dehydrated prior to all SEM analyses. Before placing the samples under the microscope, the powder was metallized with gold. This instrument allowed reconstructing the sample topography and provided a sub-nanometer scale resolution fitted with a Zeiss Gemini column.
Total particulate carbon and nitrogen
A CHN elemental analyzer (Flash 2000 Organic Elemental Analyzer, Thermo Scientific) was used to determine the concentrations of total particulate carbon (TPC) and total particulate nitrogen (TPN) in the surficial sediments (Eksperiandova et al., 2011). The samples were dried and well homogenized to ensure the homogeneity of the material analyzed. Samples were weighed (about 4 mg) in tin containers and introduced into the combustion reactor. After the combustion, the analyte gases are carried in a helium flow to a layer containing copper, and finally, carbon and nitrogen are detected by a Thermal Conductivity Detector (TCD) (Eksperiandova et al., 2011). The analyzer was calibrated using a certified standard (BBOT) (about 1–3 mg). The results were calculated as percentages of particulate carbon relative to the total mass of the analyzed sample.
Determination of metal concentrations in the sediments
About 0.2 g of dried sediment was mixed with 1 ml of hydrofluoric acid in Teflon bombs and left enclosed in the ultrasonic bath for 30 min. Then, 1 ml of quartz distilled nitric acid (6 N) was added. The mixture was heated for 12–24 h at a temperature of 100–130 °C (closed Teflon beaker). Next, it was heated at a temperature of 180–200 °C to evaporate the acid (opened Teflon beaker). The residue obtained was mixed with 3 ml of quartz distilled hydrochloric acid (6 N), and the mixture was heated for 12–24 h (closed Teflon beaker). Then, the Teflon plug was opened, and the mixture was heated at a temperature of 180–200 °C until evaporation of the acid. The HCl heating and evaporation step were repeated until a clear solution was obtained. For each digestion program, a blank was prepared with an equal amount of acids. Finally, we added 3 ml of HNO3 (40%) to the dried residue. The solution was consequently diluted in MilliQ water to a final volume of 60 ml. A 0.5 ml aliquot of the diluted sample was collected and diluted to 12.5 ml with HNO3 (2%) (Berthod et al., 2021). Elemental concentrations were assessed using an Agilent 7900 quadruple ICP-MS. Elements with masses between that of sodium (23) and arsenic (75), as well as silver (107), cadmium (111) and gadolinium (157), were all measured by using a collision-reaction cell with helium gas (5 mL/min) to eliminate polyatomic interferences. The remaining elements were measured without collision gas. Scandium, indium, and rhenium internal standards were added after inline mixing with the samples to correct for signal drift and matrix effects. Two sets of multi-element calibration standards (one for major and one for trace elements) were analyzed to confirm and model (through simple linear regression) the linear relationship between signal and concentration. The model was then used to convert measured sample counts to concentrations. Reported uncertainties were calculated using error propagation and considering the combination of standard deviation on replicated consecutive signal acquisitions (n = 3), internal-standard ratio, and blank subtraction. The non-linear term (internal-standard ratio) was linearized using a first-order Taylor series expansion to simplify error propagation (Jochum et al., 2016). Elemental concentrations are expressed in ppm of dry weight. Detection limits for Cu, Zn, Co, Cd, Pb, Mn, Fe, Al, P, Ca, and Ti were, respectively, 0.030, 0.258, 0.020, 0.039, 0.007, 0.018, 0.037, 0.146, 2.040, 0.67, and 0.28 ppb. Cr concentrations were less than the detection limit. To verify the analytical accuracy of the metals, we used the standard reference materials BHVO-1 (Gladney & Roelandts, 1988). Results showed good agreement between the certified and the measured values for all analyzed metals. Recovery percentages for metal contents in sediment were 101, 107, 96, 76, 73, 78, 72, 53, 145, 107, and 56% for, respectively, Fe, Al, Mn, P, Co, Cu, Zn, Cd, Pb, Ca, and Ti.
Assessment of sediment quality
The contamination factor (CF) was calculated to assess the degree of pollution in the Boughrara lagoon. CF is used to describe the contamination of a toxic substance in the environment (Hakanson, 1980), and it was calculated as follows:
where Cmetal is the metal concentration measured in the sediment sample, and CBackground is the background value of this metal. Since no background values in the soils of the Boughrara lagoon were available, the concentrations in the upper continental crust (UCC) reported by Wedepohl (1995) were used as the background crustal values.
The following criteria were used to describe the level of the contamination: if CFmetal < 1, then the contamination is considered low; it is moderate if 1 < CFmetal < 3; considerable if 3 < CFmetal < 6; and very high if CFmetal > 6 (Hakanson, 1980).
Statistical analysis
In order to explore the spatial distribution of metals in the sediments of the Boughrara lagoon and to visualize potential metal sources, all contents were mapped in the GIS-based software ArcGIS 10.2 from Esri (the Environmental Systems Research Institute Inc). A multivariate statistical method was also used to identify samples with similar elemental composition and to interpret the contamination pattern and distribution of all parameters. For this purpose, the compositional data analysis (CoDA) was applied with the chemical element concentrations analyzed in the surficial sediments (Cu, Zn, Co, Cd, Pb, Mn, Fe, Al, and P) by using RStudio software. Indeed, CoDA is a statistical method useful for the simultaneous analysis of several elemental ratios by reducing a large number of variables to a few unrelated components (Aitchison, 1982; Xu-Yang et al., 2021).
Results
Mineralogy and grain size distribution
Near the 3 streams, the sediment coarse fraction (> 63 µm) mainly constituted of sand represented more than 90% of the total mass of the sample with, respectively, 94.4%, 89.9%, 92.2%, 95.7%, 93.1%, and 95.3% at stations P7, B6, B7, B8, B9, and B10 (Table 1). For these samples, the curves of the sediment size distribution were skewed (S-shaped), indicating homogeneity of the very coarse sand (Fig. 4). By contrast, the sediment fine fraction (< 63 µm) was much more significant in the water inflows from the Ajim channel, as it represented 39 to 69% of the total sample mass (Table 1) at stations C3, C4, C5, C6, C8, C9, C10, and C11 with, respectively, 39.3%, 65.9%, 69.2%, 50.6%, 45.7%, 52.3%, 40.97%, and 51.9%. The semi-logarithmic curves of the sediment size distribution presented a parabolic shape in this area (Fig. 4), indicating heterogeneity of the fine fraction, in which the remains of shells were found to be dominant.
The X-ray diffraction analyses of the surficial sediments highlighted the presence of many minerals like quartz, calcite, aragonite, and some types of clay (Fig. 5). Samples located near the Fjé and Smar streams (stations P1, P3, P7, and B6-B10) presented a simple mineralogical composition, with the dominance of quartz XRD-peaks (SiO2; ICSD: PAN 98–015-4289). Near the Bou Hamed stream (stations B2-B3), the most important peak was for evaporates (gypsum and halite), whereas illite–smectite was less abundant. Near the Ajim channel (stations C6-C11) the main peaks were for calcium carbonate (CaCO3; ICSD: PAN 98–004-0107), with significant peaks in particular for calcite, magnesium-calcite and dolomite. Mica and illite–smectite were also present in this area.
In order to confirm the mineralogical analyses, sediment powders have been also observed using scanning electron microscopy. Three types of clay have been observed, which were illite–smectite, chlorite and mica (stations P1, C1, and C3) (Fig. 6a–b). Furthermore, a rhombohedral shape for quartz was identified in the samples near the three streams. Coccoliths and diatoms and their silica-frustules were identified in the southwestern part of the lagoon (Fig. 6c–d). Pyrite mineral (FeS2) in euhedral form was also identified (stations P1, P3, C1, and C3).
Spatial distribution of metals and phosphorus in surficial sediments
The concentrations of Cu, Zn, Co, Cd, Pb, Mn, Fe, Al, Ca, Ti, and P in surficial sediments of the Boughrara Lagoon are reported in Table 3. Ti and Ca were used to identify the main origin of sediment in the studied lagoon. The spatial distribution of measured elements showed the highest concentrations in the southwestern part of the lagoon (Fig. 7). The ranges of concentrations varied significantly between the different elements, decreasing in the order of Fe > Al > Mn > P > Zn > Cu > Pb > Co > Cd, with mean concentrations of, respectively, 1.63%, 1.2%, 414 ppm, 377 ppm, 86.6 ppm, 40.9 ppm, 34.7 ppm, 4.7 ppm, and 0.5 ppm. The highest concentrations were observed near the three main streams (Fjé, Smar, and Bou Hamed) with 4.9% for Fe, 2.4% for Al, 1469 ppm for Mn, 826 ppm for P, 244.8 ppm for Zn, 173.3 ppm for Pb, 134.3 ppm for Cu, 12.9 ppm for Co, and 2.2 ppm for Cd.
Zinc and Cu presented similar features, with high values at stations B3 and B9 of, respectively, 131 ppm and 134 ppm for Cu, 245 ppm and 244 ppm for Zn (Fig. 7). Cobalt and Cd also showed a similar spatial distribution, with the highest concentrations at stations B8-B9 with 12.90 ppm for Co and 2.24 ppm for Cd (Fig. 7). Concerning Pb concentrations, its highest concentration of 173 ppm was observed near the Bou Hamed stream at station B3 (Fig. 7). The spatial distribution of Mn was similar to that of Fe, with high values near the Fjé and Smar streams (Fig. 7). The maximum concentrations of Mn and Fe were recorded at station B8 with, respectively, 1469 ppm and 4.9%. Aluminum and phosphorus concentrations also showed a similar distribution (Fig. 7) with high values observed near the Bou Hamed stream at station B3, with 3.2% for Al and 826 ppm for P. The concentrations of Cd were much lower than those of the other metals values, ranging from 0.1 to 2.2 ppm, with a maximum at station B8. The enrichment in metals (Pb, Fe, Mn, Zn, Cu, and Al) detected with the measurement of high concentrations was further confirmed by the calculations of their relatively high contamination factor (CF) (Table 4).
Samples collected from the red clay quarry (S1) and the lead–zinc deposits (S2) were highly enriched in metals (Table 3). The red clay quarry (S1) was particularly enriched in Fe, Al, Mn, P, Cd, and Pb with concentrations of, respectively, 6.6%, 7.1%, 351.4 ppm, and 13,063.98 ppm (Table 3). In the lead–zinc deposits (S2), Pb, Zn, Cd, and Fe displayed the highest concentrations of, respectively, 307,587.7 ppm, 77,558.9 ppm, 682.1 ppm, and 2.9% for Fe (Table 3).
Total particulate carbon and nitrogen in surficial sediments
The relative abundance of total particulate carbon (TPC) in the surficial sediments was relatively low, ranging from 1 to 13%, with an average value of 7%. The minimum and maximum TPC relative abundance were observed at stations C1 and B6, respectively (Table 2). The relative abundance of total particulate nitrogen (TPN) was even lower compared to TPC, with maximum and minimum values of, respectively, 0.8% at station C1 and 0.1% at station B7 (Fig. 8).
Compositional data analysis (CoDA)
Based on the compositional data analysis (CoDA) tool, the projection of 9 variables of chemical elements (8 metals and phosphorus) and 17 individuals of surficial sediments was plotted in Fig. 9. Percentages of variability explained by the first two components were 65% for comp.1 and 21% for comp.2, a total of 86%. This biplot highlighted the presence of three different groups to classify the surficial sediments in the lagoon. The first group (A) contains samples near the Bou Hamed stream (B2, B3, P1, and P3), which were enriched in Pb and Cu. The second group (B) contained samples near the Fjé and Smar streams that were enriched in Fe, Mn, and Co. Finally, the third group (C) included the samples located in the water inflows from the Ajim channel (C1, C2, C8, C9, C10, and C11) and were enriched in P and Al.
Compositional data analysis (CoDA) biplot of surficial sediments in the Boughrara Lagoon for 9 chemical variables (8 metals and phosphorus) with comp.1 = 65% and comp.2 = 21%. Area A regroups samples near Bou Hamed stream, area B contains the samples near Fjé stream and Area C the samples located in the water inflows from the Ajim channel
Furthermore, the biplot achieved with a selection of 4 metals as variables (Cd, Zn, Pb, and Cu) that combined our results and those recorded in the Gulf of Gabes (El Zrelli et al., 2015), suggested similar enrichment, mainly in Cu and Pb, in the southwestern of the lagoon and near the Gabes port (Fig. 10). In this compositional biplot, the first two axes explained 91% of the total variance, with 80% for comp.1 and 11% for comp.2.
Compositional data analysis (CoDA) biplot of the surficial sediments analyzed in the present study (in blue) and in the Gulf of Gabes (in red) by El Zrelli et al. (2015) for 4 variables of metals (Cd, Zn, Pb, Cu) with comp.1 = 80% and comp.2 = 11%
Discussion
Main sediment input
Samples located near the water inflows from the Ajim channel were dominated by the fine fraction (< 63 µm) which is enriched with carbon. This enrichment observed at stations C1-C11 can indicate a high biological production (Aleksandrov, 2010; Luczak et al., 1997). Furthermore, the elemental ratios of Fe/Ca and Ti/Ca were relatively low in this area (Fig. 8) due to comparatively high concentrations of calcium (Table 3). Such high levels of calcium are generally associated with carbonate fraction that specifies the biogenic autochthonous source (Affouri et al., 2017; Plewa et al., 2012). The TPC/TPN ratios ranged between 10 and 44, with a mean of 23 (Table 2). The highest values were found at stations C2 and C11 influenced by the water inflows from the Mediterranean Sea. Here, the relative enrichment in TPC correlated with high percentages of mud in the fine fraction (Tables 1 and 2) which can point out a marine origin of the particulate matter. A relatively high abundance of mud rich in gastropods and mollusks was also recently recorded in the center of the lagoon and near the Ajim channel (Keskes et al., 2020).
Near the three streams, the granulometric analyses showed the predominance of the coarse fraction and a relatively high abundance of quartz as detected with SiO2 XRD-peaks, all suggesting continental inputs (aeolian and fluvial) to surficial sediments in these areas. High values of Fe/Ca and Ti/Ca ratios were also observed in the southwestern side of the Boughrara lagoon (Fig. 8) due to relatively low Ca concentrations (Table 3), confirming an allochthonous lithogenic source. For all samples near the three main streams, the TPC/TPN ratios were low (stations P1-B6) which confirms the terrestrial origin of sediment input.
Contamination and ecological risk assessment of chemical elements
Background on chemical contamination
Surficial sediments of the southwestern part of the Boughrara lagoon were characterized by high concentrations of metals, especially by Pb, Fe, Mn, Cu, and Zn. Phosphorus concentrations were also very high, ranging between 77 and 888 ppm, similar to what was observed by Keskes et al. (2020) in the Boughrara lagoon (Table 5). Cupper concentrations recorded in the surficial sediments of the Boughrara lagoon (7.64–134.26 ppm) were also similar to those reported by Keskes et al. (2020) of 3.8–161.2 ppm. Cadmium, Cu, and Zn concentrations recorded in this study were in a similar range than those reported by Kharroubi et al. (2012a, b) in the Boughrara lagoon with values of, respectively, 0.11–2.23 ppm, 8–134 ppm, and 29–245 ppm in this study and 0–3.1 ppm, 14–56 ppm, and 39–119 ppm in Kharroubi et al. (2012a, b) (Table 5). The concentrations of Fe and Mn in the sediments were much higher in the southwestern sediments (this study) compared to those located in the North of the lagoon (Kharroubi et al., 2012a, b) with values of, respectively, 4881–48,802 ppm and 68–1469 ppm in this study and 8320–9580 ppm and 59–99 ppm in Kharroubi et al. (2012a, b) (Table 5). High Fe concentrations were also previously recorded in the western part of the Boughrara lagoon (Keskes et al., 2020), but these concentrations were lower than the maximum values obtained in our study (Table 5).
Compared to other coastal lagoons threatened by anthropogenic pressure such as the Bizerte Lagoon in Tunisia (El Zrelli et al., 2021; Mna et al., 2017), the Ghar El Melh Lagoon in Tunisia (Oueslati, 2011) and the Manzala Lagoon in Egypt (Khatita et al., 2016), the concentrations of Fe in the sediments were higher in this study, except for values obtained in the Ghar El Melh Lagoon (Oueslati, 2011) (Table 5).
Distribution, origin, and degree of contamination by chemical elements
The contamination factor (CF) values for Pb ranged between 3 and 6 near Bou Hamed (stations P3 and B9; Table 4), indicating a considerable level of contamination. The CF value of Pb was 10.2 at station B3 near the Bou Hamed stream (Table 4), indicating a very high contamination by Pb. For Cu, the CF values were higher than 6 at stations P3, B3, B8, and B9, indicating a very high level of contamination. Copper and Zn had a similar spatial distribution showing the highest values in the sediments near the Bou Hamed and Smar streams (Fig. 7). Like Cu, the enrichment by Zn was confirmed with CF values ranging between 3 and 6 at stations P3, B3, B8, and B9 indicating a considerable degree of contamination.
The CF values calculated for Fe ranged between 1 and 3 at stations B3, B6, and B7 with values of, respectively, 1.1, 1.3, and 1.6, indicating a moderate degree of contamination. Here, Fe concentrations were slightly higher than concentrations in the upper continental crust (UCC) (Wedepohl, 1995), and they were much higher than those reported in the same lagoon by Keskes et al. (2020) and Kharroubi et al. (2012a, b). Results similar to those of Fe were obtained for Mn, with CF values ranging between 1 and 3 with values of 1.9, 1.8, 2.8, 2, and 1.5 at stations P7, B6, B8, B9, and B10, respectively. Near the Fjè and Smar streams, the CF values of Cd were higher than 6 at stations P1, P3, B3, B8, B9, C1, C6, and C10 indicating a very high level of contamination by Cd in these areas.
The CF values for P were generally lower than 1 (Table 4) indicating that this area is not contaminated by P, but phosphorous concentrations were higher than those obtained in Kharroubi et al. (2012a, b).
Compared to other coastal regions in Tunisia, the Boughrara lagoon has already been reported to have a high level of contamination by metals like Pb, Fe, Mn, Al, Cu, Cd, and Cr (Gargouri et al., 2011; Kharroubi et al., 2012a, b; El Zrelli et al., 2017; Keskes et al., 2020; El Zrelli et al., 2021), and our study confirmed these contaminations. In view of these results and by comparing with the previous studies, three possible sources of pollutants can be identified. The first potential source is phosphogypsum discharges, pumped from the chemical industry of Gabes, which are very enriched in P, Cu, Cd, and Co. This source of pollution was also proposed by Kharroubi et al. (2012a, b) by comparing metal concentrations analyzed in surficial sediments with those obtained in phosphogypsum effluents. The second anthropogenic source concerned Pb and Zn, and it was probably due to the transportation of Pb from the Pb ex-mine by Fjé, Smar, and Bouhamed streams to the Boughrara Lagoon. The third source which caused Fe enrichment can be related to both natural (cliff weathering) and anthropogenic (stream inputs from Tejra red clay quarry) precursors.
Environmental consequences of metal contamination
As a part of the Gulf of Gabes, the Boughrara lagoon has become one of the most polluted ecosystems in this region. Previous studies have already suggested that the Gulf of Gabes is highly contaminated by organic and inorganic pollutants (Darmoul, 1988; El Zrelli et al., 2015, 2017; Rabaoui et al., 2014), leading to a significant degradation of the environment.
The accumulation of metals by various marine species and its biomagnification in the food chains cause many serious human threats (Annabi-Trabelsi et al., 2021). Many authors attributed these threats to either the high metallic marine pollution or radio-chemical wastes like phosphogypsum (Dethlefsen et al., 1996; Ayadi et al., 2015; El Zrelli et al., 2017).
Since metals can be bioaccumulated in the tissues of marine biota, some marine species can even serve as bio-indicators of metal pollution in coastal environments (Rainbow et al., 2006; Zhou et al., 2008). For instance, various species of mollusks (like Pinna nobilis and P. radiate) and gastropods (like G. ardens and P. cearulea) of the Gulf of Gabes, especially of the Boughrara lagoon, have shown to be highly contaminated by metals (Cd, Zn, Cu, Al, Pb,…) (El Zrelli et al., 2017; Rabaoui et al., 2017).
Eutrophication phenomenon in the Boughrara Lagoon
We detected the presence of pyrite (FeS2) for the first time in the sediments of the Boughrara Lagoon. Pyrite precipitation (pyritization) is a result of sulfate and Fe (oxy-)hydroxides reduction in anoxic conditions (Sabadini-Santos et al., 2014). For instance, the hydrogen sulfide (H2S) generated during early diagenesis in anoxic sediments can precipitate as authigenic sulfide minerals, like pyrite (Morse & Cornwell, 1987). The high inputs of sulfate-rich phosphogypsum and Fe-rich red clays into the lagoon likely favored the formation of pyrite. Anyway, the presence of pyrite that we detected in these sediments when it had not been reported in previous studies indicated a preservation of pyrite which can only be explained by an increase of anoxic conditions in the lagoon. The occurrence of red tides and algae bloom reported in the southwestern part of the Boughrara lagoon indeed indicated eutrophication of the lagoon that can lead to the development of hypoxic-anoxic conditions (Fig. 11). Increasing anthropogenic pressures combined with the extremely low renewing of the waters in the lagoon (0.4% of renewing daily) that leads to the inevitable stagnation of the waters and the stronger stratification of the water-column (Ben Aoun et al., 2007; Kharroubi et al., 2012a, b; Brahim et al., 2014; Atoui et al., 2020; Ciglenečki et al., 2020; Pagano & Sammari, 2021) were likely the causes of the eutrophication of the lagoon and the development of anoxic conditions. The eutrophication phenomenon can lead to many environmental threats in the lagoon like the proliferation of macro-algae and the development of tolerant species. This would have a drastic impact on the social-economic development of the village of Boughrara.
Conclusion
Coastal marine systems are subject to increasing anthropogenic pressures and various chemical pollutions that can threaten aquatic ecosystems and human health. It is therefore becoming urgent to develop pollution monitoring tools in order to preserve resources and biodiversity in these environments. The lagoon of Boughrara constitutes a privileged study site for developing these pollution indicators since it constitutes a semi-closed basin in which the phosphogypsum discharges from a chemical industry and the metal inputs from an ex-Pb mine and a red clay quarry can accumulate.
Our study showed that the central-western part of the lagoon was influenced by the advection of the Mediterranean waters through the Ajim channel and, there, the sediments were enriched in P, Cd, Cu and Co, due to the accumulation of phosphogypsum released by the chemical industry in the Gulf of Gabes. However, the contamination by Cd and Cu was estimated to be low in this area, on the contrary to the radiochemical phosphogypsum that can have a profound deleterious impact on the environment and health.
In the south-western part of the lagoon, continental inputs carried by three main streams were prevalent and the surficial sediments were highly enriched in Pb, Zn, and Cu in the southern part of the lagoon and in Fe, Mn, and Co in the southwestern part, due to the inputs from the ex-Pb mine and the red clay quarry, and to the cliff weathering. In these areas, the degree of contamination by Pb, Cd, Zn, and Cu was found to be very high to considerable, which can have serious toxic effects.
Furthermore, pyrite deposits were detected in the surficial sediments of the lagoon, likely resulting from the high inputs of sulfate-rich phosphogypsum and Fe-rich red clays, combined with the eutrophication and increasing anoxia of the lagoon.
Systematic monitoring of metal and mineral levels in the sediments of marine coastal systems will therefore make it possible to better assess the level of metal contamination and the degree of anoxia of these systems sensitive to anthropogenic pressures. It is a prerequisite for preserving the water-resource quality and the marine biodiversity.
Data availability
The datasets generated during and/or analyzed during this study are available from the corresponding author upon reasonable request.
References
Affouri, A., Dezileau, L., & Kallel, N. (2017). Extreme flood event reconstruction spanning the last century in the El Bibane Lagoon (southeastern Tunisia): A multi-proxy approach. Climate of the past, 13(6), 711–727.
Aitchison, J. (1982). The statistical analysis of compositional data. Journal of the Royal Statistical Society: Series B (Methodological), 44(2), 139–160.
Aleksandrov, S. V. (2010). Biological production and eutrophication of Baltic Sea estuarine ecosystems: The Curonian and Vistula Lagoons. Marine Pollution Bulletin, 61(4–6), 205–210.
Amari, A., Soussi, N., & Bobier, C. (1984). Courantologie, hydrologie et environnements sédimentaires sur le platier de Kerkennah et le littoral de Sfax (Tunisie). Réunion annuelle des sciences de la terre, (10).
Annabi-Trabelsi, N., Guermazi, W., Karam, Q., Ali, M., Uddin, S., Leignel, V., & Ayadi, H. (2021). Concentrations of trace metals in phytoplankton and zooplankton in the Gulf of Gabès, Tunisia. Marine Pollution Bulletin, 168, 112–392.
Atoui, A., Smeti, H., Sammari, C., & Ismail, S. B. (2020). Water renewal in the Boughrara lagoon (Tunisia, central Mediterranean Sea) under tidal forcing. Estuarine, Coastal and Shelf Science, 238, 106–680.
Ayadi, N., Aloulou, F., & Bouzid, J. (2015). Assessment of contaminated sediment by phosphate fertilizer industrial waste using pollution indices and statistical techniques in the Gulf of Gabes (Tunisia). Arabian Journal of Geosciences, 8(3), 1755–1767.
Belkahia, N. M., Pagano, M., Chevalier, C., Devenon, J. L., & Yahia, M. N. D. (2021). Zooplankton abundance and community structure driven by tidal currents in a Mediterranean coastal lagoon (Boughrara, Tunisia, SW Mediterranean Sea). Estuarine, Coastal and Shelf Science, 250, 107101.
Ben Aoun, Z., Farhat, F., Chouba, L., & Hadj Ali, M. S. (2007). Investigation on possible chemical pollution of the Boughrara lagoon, south of Tunisia, by chemical wastes. Bull. Inst. Nat. Scien. Tech. Mer de Salammbô, 34, Tunisie, 119–127.
Berthod, C., Médard, E., Di Muro, A., Hassen Ali, T., Gurioli, L., Chauvel, C., & Fouquet, Y. (2021). Mantle xenolith-bearing phonolites and basanites feed the active volcanic ridge of Mayotte (Comoros archipelago, SW Indian Ocean). Contributions to Mineralogy and Petrology, 176(10), 1–24.
Brahim, M., Hosni, S., & Atoui, A. (2014). La dynamique sédimentaire au voisinage du port de pêche de Zarzis.
Ciglenečki, I., Čanković, M., Kuzmić, M., & Pagano, M. (2020). Accumulation of organic matter in a mesotidal Mediterranean lagoon (Boughrara, Tunisia). Estuarine, Coastal and Shelf Science, 240, 106–780.
Çullu, A. F., Sönmez, V. Z., & Sivri, N. (2021). Microplastic contamination in surface waters of the Küçükçekmece Lagoon, Marmara Sea (Turkey): Sources and areal distribution. Environmental Pollution, 268, 115–801.
Darmoul, B. (1988). Pollution dans le golfe de Gabès (Tunisie): bilan de six années de surveillance (1976–1981).
Dethlefsen, V., Söffker, K., Büther, H., & Damm, U. (1996). Organochlorine compounds in marine organisms from the international North Sea incineration area. Archive of Fishery and Marine Research, 44, 215–242.
Eksperiandova, L. P., Fedorov, O. I., & Stepanenko, N. A. (2011). Estimation of metrological characteristics of the element analyzer EuroVector EA-3000 and its potential in the single-reactor CHNS mode. Microchemical Journal, 99(2), 235–238.
El Zrelli, R., Courjault-Radé, P., Rabaoui, L., Castet, S., Michel, S., & Bejaoui, N. (2015). Heavy metal contamination and ecological risk assessment in the surface sediments of the coastal area surrounding the industrial complex of Gabes city, Gulf of Gabes, SE Tunisia. Marine Pollution Bulletin, 101(2), 922–929.
El Zrelli, R., Courjault-Radé, P., Rabaoui, L., Daghbouj, N., Mansour, L., Balti, R., & Bejaoui, N. (2017). Biomonitoring of coastal pollution in the Gulf of Gabes (SE, Tunisia): Use of Posidonia oceanic seagrass as a bio-indicator and its mat as an archive of coastal metallic contamination. Environmental Science and Pollution Research, 24(28), 22214–22225.
El Zrelli, R., Yacoubi, L., Wakkaf, T., Castet, S., Grégoire, M., Mansour, L., & Rabaoui, L. (2021). Surface sediment enrichment with trace metals in a heavily human-impacted lagoon (Bizerte Lagoon, Southern Mediterranean Sea): Spatial distribution, ecological risk assessment, and implications for environmental protection. Marine Pollution Bulletin, 169, 112–512.
Ferraro, A., Parisi, A., Barbone, E., Race, M., Mali, M., Spasiano, D., & Fratino, U. (2023). Characterising contaminants distribution in marine-coastal sediments through multivariate and nonparametric statistical analyses: A complementary strategy supporting environmental monitoring and control. Environmental Monitoring and Assessment, 195(1), 1–19.
Folk, R. L., & Ward, W. C. (1957). Brazos River bar (Texas): A study in the significance of grain size parameters. Journal of Sedimentary Research, 27(1), 3–26.
Gargouri, D., Azri, C., Serbaji, M. M., Jedoui, Y., & Montacer, M. (2011). Heavy metal concentrations in the surface marine sediments of Sfax Coast, Tunisia. Environmental Monitoring and Assessment, 175(1), 519–530.
Gargouri, D., Gzam, M., Kharroubi, A., & Jedoui, Y. (2018). Use of sediment quality indicators for metals contamination and ecological risk assessment in urbanized coastal zones. Environmental Earth Sciences, 77(10), 38.
Gladney, E. S., & Roelandts, I. (1988). 1987 compilation of elemental concentration data for USGS BHVO-1, MAG-1, QLO-1, RGM-1, SCo-1, SDC-1, SGR-1 and STM-1. Geostandards Newsletter, 12(2), 253–362.
Hakanson, L. (1980). An ecological risk index for aquatic pollution control. A sedimentological approach. Water Research, 14(8), 975–1001.
Hu, C., Yang, X., Dong, J., & Zhang, X. (2018). Heavy metal concentrations and chemical fractions in sediment from Swan Lagoon, China: Their relation to the physiochemical properties of sediment. Chemosphere, 209, 848–856.
Jochum, K. P., Weis, U., Schwager, B., Stoll, B., Wilson, S. A., Haug, G. H., & Enzweiler, J. (2016). Reference values following ISO guidelines for frequently requested rock reference materials. Geostandards and Geoanalytical Research, 40(3), 333–350.
Keskes, F. A., Ayadi, N., Atoui, A., Mahfoudi, M., Abdennadher, M., Walha, L. D., & Hassen, M. B. (2020). Dinoflagella tesencystment with emphasis on blooms in Boughrara lagoon (South-Western Mediterranean): Combined effects of trace metal concentration and environmental context. Estuarine, Coastal and Shelf Science, 237, 106–648.
Kharroubi, A., Gzam, M., & Jedoui, Y. (2012a). Anthropogenic and natural effects on the water and sediments qualities of costal lagoons: Case of the Boughrara lagoon (Southeast Tunisia). Environmental Earth Sciences, 67(4), 1061–1067.
Kharroubi, A., Gargouri, D., Baati, H., & Azri, C. (2012b). Assessment of sediment quality in the Mediterranean Sea-Boughrara lagoon exchange areas (southeastern Tunisia): GIS approach-based chemometric methods. Environmental Monitoring and Assessment, 184(6), 4001–4014.
Khatita, A. M. A., Shaker, I. M., El Zawahry, M. K., & Shetaia, S. A. (2016). Distribution and ecological risk assessment of heavy metals in the sediment cores from Manzala lagoon, Egypt. International Journal of Innovative Science, Engineering and Technology, 3, 377–387.
Khedhri, I., Atoui, A., Ibrahim, M., Afli, A., & Aleya, L. (2016). Assessment of surface sediment dynamics and response of benthic macrofauna assemblages in Boughrara Lagoon (SW Mediterranean Sea). Ecological Indicators, 70, 77–88.
Kükrer, S., Erginal, A. E., Kılıç, Ş, Bay, Ö., Akarsu, T., & Öztura, E. (2020). Ecological risk assessment of surface sediments of Çardak Lagoon along a human disturbance gradient. Environmental Monitoring and Assessment, 192(6), 1–15.
Lin, H., Lan, W., Feng, Q., Zhu, X., Li, T., Zhang, R., & Zhao, B. (2021). Pollution and ecological risk assessment, and source identification of metals in sediment from the Beibu Gulf, South China Sea. Marine Pollution Bulletin, 168, 112–403.
Luczak, C., Janquin, M. A., & Kupka, A. (1997). Simple standard procedure for the routine determination of organic matter in marine sediment. Hydrobiologia, 345(1), 87–94.
Lutterotti, L., Matthies, S., & Wenk, H. R. (1999). Quantitative phase analysis. Newsletter of the CPD, 21, 14–15.
Maghrebi, S. (1995). Dynamique sédimentaire dans le golfe de Gabes (Tunisie) : Impact des aménagements côtiers (Doctoral dissertation, University of Paris 11, France).
Mna, H. B., Helali, M. A., Oueslati, W., Amri, S., & Aleya, L. (2021). Spatial distribution, contamination assessment and potential ecological risk of some trace metals in the surface sediments of the Gulf of Tunis, North Tunisia. Marine Pollution Bulletin, 170, 112–608.
Mna, H. B., Oueslati, W., Helali, M. A., Zaaboub, N., Added, A., & Aleya, L. (2017). Distribution and assessment of heavy metal toxicity in sediment cores from Bizerte Lagoon, Tunisia. Environmental Monitoring and Assessment, 189(7), 1–18.
Morse, J. W., & Cornwell, J. C. (1987). Analysis and distribution of iron sulfide minerals in recent anoxic marine sediments. Marine Chemistry, 22(1), 55–69.
Mosbahi, N., Serbaji, M. M., Pezy, J. P., Neifar, L., & Dauvin, J. C. (2019). Response of benthic macrofauna to multiple anthropogenic pressures in the shallow coastal zone south of Sfax (Tunisia, central Mediterranean Sea). Environmental Pollution, 253, 474–487.
Naifar, I., Pereira, F., Zmemla, R., Bouaziz, M., Elleuch, B., & Garcia, D. (2018). Spatial distribution and contamination assessment of metals in marine sediments of the southern coast of Sfax, Gabes Gulf, Tunisia. Marine Pollution Bulletin, 131, 53–62.
Oueslati, W. (2011). Cycles biogéochimiques des métaux lourds dans les sédiments marins de la lagune de Ghar El Melh. Université Tunis-El Manar (Tunis).
Pagano, M., & Sammari, C. (2021). Planktonic responses to hydrodynamic forcing in coastal ecosystems in the context of climatic and anthropogenic change. Estuarine Coastal and Shelf Science, 256, 107390.
Pignotti, E., Guerra, R., Covelli, S., Fabbri, E., & Dinelli, E. (2018). Sediment quality assessment in a coastal lagoon (Ravenna, NE Italy) based on SEM-AVS and sequential extraction procedure. Science of the Total Environment, 635, 216–227.
Plewa, K., Meggers, H., Kuhlmann, H., Freudenthal, T., & Zabel, & M., Kasten, S. (2012). Geochemical distribution patterns as indicators for productivity and terrigenous input off NW Africa. Deep Sea Research Part I: Oceanographic Research Papers, 66, 51–66.
Rabaoui, L., Balti, R., Zrelli, R., & Tlig-Zouari, S. (2014). Assessment of metals pollution in the gulf of Gabes (Tunisia) using four mollusk species. Mediterranean Marine Science, 15(1), 45–58.
Rabaoui, L., El Zrelli, R., Balti, R., Mansour, L., Courjault-Radé, P., Daghbouj, N., & Tlig-Zouari, S. (2017). Metal bioaccumulation in two edible cephalopods in the Gulf of Gabes, South-Eastern Tunisia: Environmental and human health risk assessment. Environmental Science and Pollution Research, 24(2), 1686–1699.
Rached, L., Rachida, T., Mohamed, S., Younès, J., Sahbi, J., & Marco, A. (2022). Sedimentary record from Holocene to present-day Southeastern Tunisia: Facies, paleoenvironments and climate changes. Arabian Journal of Geosciences, 15(10), 1–20.
Rainbow, P. S., Poirier, L., Smith, B. D., Brix, K. V., & Luoma, S. N. (2006). Trophic transfer of trace metals from the polychaete worm Nereisdiversicolor to the polychaete N. virens and the decapod crustacean Palaemonetesvarians. Marine Ecology Progress Series, 321, 167–181.
Sabadini-Santos, E., Senez, T. M., Silva, T. S., Moreira, M. R., Mendonça-Filho, J. G., Santelli, R. E., & Crapez, M. A. (2014). Organic matter and pyritization relationship in recent sediments from a tropical and eutrophic bay. Marine Pollution Bulletin, 89(1–2), 220–228.
Wang, Z., Lin, K., & Liu, X. (2022). Distribution and pollution risk assessment of metals in the surface sediment of the intertidal zones of the Yellow River Estuary, China. Marine Pollution Bulletin, 174, 113–286.
Wedepohl, K. H. (1995). The composition of the continental crust. Geochimica Et Cosmochimica Acta, 59(7), 1217–1232.
Xu-Yang, Y., Losno, R., Monna, F., Rajot, J. L., Labiadh, M., Bergametti, G., & Marticorena, B. (2021). Compositional data analysis (CoDA) as a tool to evaluate a new low-cost settling-based PM 10 sampling head in a desert dust source region. Atmospheric Measurement Techniques, 14(12), 7657–7680.
Zaaboub, N., Martins, M. V. A., Dhib, A., Béjaoui, B., Galgani, F., El Bour, E. M., & Aleya, L. (2015). Accumulation of trace metals in sediments in a Mediterranean Lagoon: Usefulness of metal sediment fractionation and elutriate toxicity assessment. Environmental Pollution, 207, 226–237.
Zhao, M., Wang, E., Xia, P., Feng, A., Chi, Y., & Sun, Y. (2019). Distribution and pollution assessment of metals in the intertidal zone environments of typical sea areas in China. Marine Pollution Bulletin, 138, 397–406.
Zhou, W., Wu, H., & Yildirim, T. (2008). Enhanced H2 adsorption in isostructural metal-organic frameworks with open metal sites: Strong dependence of the binding strength on metal ions. Journal of the American Chemical Society, 130(46), 15268–15269.
Acknowledgements
We are deeply grateful to Dr. Pascale BESSON, Stephan BORENSZTAJN, and Clément DEMASY at IPGP (France) for their help and assistance with the geochemical and SEM analyses, and to Dr. Yahya MOUMNI at University of Gabes for his contribution to the sampling missions.
Funding
This work was supported by a LEFE-CYBER project of the French INSU-CNRS coordinated by Dr. Marie BOYE and by an exchange international grant from the University of Gabes (Tunisia).
Author information
Authors and Affiliations
Contributions
Nejia TLIG contributed to data interpretation and wrote the major manuscript. Marie BOYE contributed to writing and revising the manuscript. Tahani HALLEK contributed to sampling and data collection. Pierre BURCKEL contributed to analyses. Maher GZAM contributed to sampling. Mohamed Ali TAGORTI revised the manuscript. All authors reviewed and approved the final version to be published.
Corresponding author
Ethics declarations
Conflict of interest
The authors declare no competing interests.
Additional information
Publisher's Note
Springer Nature remains neutral with regard to jurisdictional claims in published maps and institutional affiliations.
Highlights
• The origin of the main sediment input was identified through ratios calculations.
• Enrichment by metals was determined in surficial sediment of the lagoon.
• Anoxic conditions due to low water circulation allowed to eutrophication phenomena.
• Pyrite mineral is identified for the first time in the Boughrara Lagoon.
• Three possible sources of pollution are identified based on the studied lagoon.
Rights and permissions
Springer Nature or its licensor (e.g. a society or other partner) holds exclusive rights to this article under a publishing agreement with the author(s) or other rightsholder(s); author self-archiving of the accepted manuscript version of this article is solely governed by the terms of such publishing agreement and applicable law.
About this article
Cite this article
Tlig, N., Boye, M., Hallek, T. et al. Sediment quality and environmental risk assessment in a Mediterranean coastal system using geochemical and multivariate statistical analyses: the case of Boughrara Lagoon (southeastern Tunisia). Environ Monit Assess 195, 422 (2023). https://doi.org/10.1007/s10661-023-11029-8
Received:
Accepted:
Published:
DOI: https://doi.org/10.1007/s10661-023-11029-8