Abstract
Wetlands support livelihoods of millions of people in developing countries. However, wetland land cover change, as a result of growing population and subsequent anthropogenic activities, has been evident at a global scale, and ongoing micro-climate alteration has further deteriorating its ecological condition. Nepal is equally vulnerable to wetland changes that can have direct effects on the sustenance of local wetland-dependent people. This study thus attempts to look at how wetland areas of Nepal are undergoing changes, taking a case of Ghodaghodi Lake Complex (GLC). Remote sensing technique, climate, and population data were used in the analysis aided by focus group discussions and key informant interviews. Results showed that total population of the study area has been increased drastically in recent decades. Landsat image analysis for 25 years (1989–2016) depicts changes in the GLC in its land cover, with maximum expansion observed in settlement followed by river and banks, whereas maximum reduction was observed in forests, followed by areas of agricultural land and lake. Similarly, diurnal temperature is increasing while total annual rainfall is slightly decreasing during the same period. Locals have perceived ecological degradation in the GLC due to both anthropogenic pressure and climatic variability. The study outlines linkage of drivers for GLC degradation and finally makes recommendations to achieve longer term sustainability of the lake complex.
Similar content being viewed by others
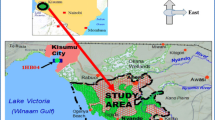
Explore related subjects
Discover the latest articles, news and stories from top researchers in related subjects.Avoid common mistakes on your manuscript.
Introduction
Wetlands are vital to the local people. Often, people are highly dependent on their ecosystem services for their livelihoods. Current global areal extent of wetland is about 14.86 million km2; however, it could have been approximately 29.83 million km2 if no anthropogenic disturbance existed (Hu et al. 2017). Almost 71% of wetlands disappeared during the twentieth century (Gardner et al. 2015). Inland wetlands depleted at higher rate compared with coastal wetlands (Davidson 2014). Disruption of the delicate balance between climatic variables (i.e., precipitation and evaporation) as a result of increased warming made wetlands vulnerable to climate change leading to change in their distribution, areal extent, and overall functioning (Clair 1998). Wetlands have carbon sequestration potential, currently with net carbon sink capacity of about 830 Tg/year on a global scale (Mitsch et al. 2013); however, if degraded, then they could be a source of greenhouse gas and easily emit noxious carbon dioxide and methane into the atmosphere (Kayranli et al. 2010; Junk et al. 2013). Climate change is also certain to alter ecological impacts of aquatic invasive species through enhanced competitive and predatory effects on native species (Rahel and Olden 2008). Similarly, increased temperature affects chemical reaction kinetics resulting in depleted water quality and freshwater ecology (Whitehead et al. 2009). Woodward et al. (2010) considered freshwater as vulnerable to climatic changes because of low dispersal capacity of aquatic species to respond to environmental changes, climatic dependency of water temperature and availability, and exposure of numerous aquatic systems to different anthropogenic stressors.
The major reasons for depletion of wetland ecosystems are anthropogenic, particularly overharvesting of resources, disposal of pollutants, and land use land cover (LULC) changes. Changes in population density alter the land use dynamics of a given landscape and are a driving force of the LULC change. Human activities have modified extensive amounts of wetlands globally, and degree of their pressure varies according to geographical locations (Reis et al. 2017). Prigent et al. (2012) found an overall decline of 6% of global inundated areas within a span of 15 years, and further noted that such decline exhibited especially in regions with soaring population. For example, an increasing population density in Jilin province of China has increased farm land and built up areas, but decreased wetland, water body, and grass land (Fei et al. 2015). Likewise, Lake Pamvotis catchment in North West Greece has seen the rise in population that led to significant increase in settlements, tourism infrastructure, and intense agricultural activities (Papastergiadou et al. 2010). Ruan et al. (2008) reported land cover changes (using Landsat images in Hongze Lake of the northern Jiangsu province) over the period of two decades, especially of urban settlement, fishing pond, and open water body because of human intervention. Approximately 60,404 km2 of natural inland wetlands in China disappeared between 1990 and 2000 because of the population boom and subsequent human disturbances (Gong et al. 2010). Likewise, anthropogenic disturbances in the form of agricultural expansion within the catchment of Harike Wetland in Punjab of India caused the wetland area to shrink by 13% in just 20 years (Mabwoga and Thukral 2014). Even though Asia has the largest wetland area in the world, it has also been affected by the highest human influences, with only 8% of available wetlands protected (Reis et al. 2017).
The wetlands of Nepal are under threats due to increased anthropogenic activities, land cover change, and ongoing climatic alteration. Accurate account of such threats is largely unknown. A recent inventory of 92 lakes and other water bodies in the far-western Nepal (DoF 2017) found no conservation program in place in the 95% of wetlands, thus warrants immediate restoration activities. Currently, there are ten Ramsar-listed wetlands in Nepal, four in Terai plain, two in Mid-hills, and four in the mountain region (Lamsal et al. 2017). In addition to this, there are other forms of wetland scattered around the country such as irrigated paddy fields, rivers, marginal swamps, ponds, lakes, and reservoirs—covering an area of approximately 8192 km2—altogether equivalent to ~ 5% of the total area of Nepal (DoFD 2012). Dependency of people in wetland for goods and services is high, especially in the semi-rural and rural settings. It is believed that wetlands located in the southern Terai plain are more vulnerable from degradation compared with those situated in the hilly and mountainous region, mainly because of high human pressure. The overall population and its growth rate are high in Terai plain and corresponding state of land cover change and resource degradation. The main objectives of this study are therefore to (i) examine the extent of population pressure, land cover change, and climatic variability in the GLC and (ii) analyze the possible consequences of such changes on the existing wetland resources and dependent communities.
Methodology
Study area
This study has focused on the Ghodaghodi Lake Complex (GLC), a biodiversity rich Ramsar site located in western Nepal (Fig. 1) which has been under high population pressure for ecosystem services and thus may be in danger of degradation in the near future if current state of exploitation continues. The GLC is a forested wetland and has 14 lakes, covering an area of 2563 ha, with Ghodaghodi (138 ha) being the largest, followed by Nakharodi (70 ha), Bhainswa (10 ha), and other smaller lakes. The study was carried out in the Ghodaghodi. GLC is situated at an altitude of 205 m above mean sea level (with latitude of 28°42′06.6″N and longitude of 80°56′44″E) in the lowlands of western Nepal, and is under the jurisdiction of the government.
The GLC is connected to an extensive tropical mixed forest in the lower slopes of the Siwalik Hills to the north, and in between two protected areas: Bardia National Park and Suklaphanta Wildlife Reserve in the south. The GLC functions as an important corridor for wildlife movement between these two protected areas (Sah and Heinen 2001). The major inhabitants of the area are the Tharu, an ethnic group that has been known to reside here for over two centuries. The population has been increasing due to influx of other communities from hill areas. The complex is bounded by three village development committees (VDCs),Footnote 1 namely Darakh, Sandepani, and Ramsikharjhala with east-west national highway passes through the southern section of it.
The lake complex was listed as a Ramsar site of international importance in 2003 due to its rich wetland biological diversity (IUCN 1998; Kafle 2005; Lamsal et al. 2014). It has great potential for tourism development because of its strategic location, existing biodiversity, and visitors’ willingness to contribute in conservation (Lamsal et al. 2016). The area holds religious and cultural values as well, and people from nearby villages and towns travel to worship at a temple in the bank of the lake all year round. Livelihood of many households in the GLC depends on the wetland resources. About 13% of the local household gross income comes from the GLC resources (Lamsal et al. 2015a).
Data collection
Primary data was collected through remote sensing, transect walk, key informant survey, and focus group discussions. The use of remote sensing technique provides both spatial and temporal information on the structure of landscape, aiding in continuous monitoring, management, and conservation of such features. However, lack of a single unifying land cover feature, highly dynamic nature and constantly changing energy signatures, and narrow ecotone due to steep environmental gradient in the edges are a few inherent characteristics of wetlands that make it difficult to monitor remotely (Gallant 2015). Though remote sensing technique for change detection has been applied in the natural resource sectors for a long time globally, its use in Nepal is recent and still being applied scantly. Some of the earlier application includes forest (Khanal 2009; Aryal et al. 2011), riverine floodplain (Chettri et al. 2013; Chaudhary et al. 2016), urban (Thapa and Murayama 2009; Rimal et al. 2015), and glacier (Bajracharya and Mool 2009; Shrestha and Joshi 2009). Hartter and Southworth (2009) suggest that remote sensing approach is useful for initial reconnaissance mapping and continuous monitoring of wetland landscape, and further integration of biophysical and human change data at temporal scale is necessary to unearth drivers and effects of landscape change.
A field observation (transect walk) was made in the study area on December 2015, in order to assess land cover and environmental change. A total of nine key informant interviews (KIIs), three in each VDC, and three focus group discussions (FGDs), one in each VDC that comprised 7–8 local people, were carried out. Comparable informal queries were asked using a checklist that was prepared in consultation with representative of local communities in each VDC and several rounds of discussions among the authors. The main purpose of these activities was to capture local’s observation as well as their perception on the causes of spatiotemporal land cover and climatic changes in the GLC and corresponding possible consequences. Similarly, field observation and pre-examination of satellite images helped us to distinguish a total of five broad land cover classes in the study area (Table 1), and accordingly, 50 ground truth points were taken for each of these categories using Garmin handheld GPS 62S.
The secondary data, i.e., Multispectral Landsats and climatic variables, were obtained from the United States Geological Survey (USGS) website and Department of Hydrology and Meteorology (DHM), Kathmandu, Nepal, respectively. All the satellite images were downloaded from Landsat sensors. This is because satellite data from Landsat sensor provides one of the longest archives and is freely accessible. Multispectral Landsats are the major satellite systems used to study all types of wetlands around the world since their first launch in 1972 (Ozesmi and Bauer 2002), and a number of studies have been using their image data. However, in recent times, some advancement was made and more new data and techniques have been tried in wetland mapping and monitoring with fair success. Satellite images of Landsat Thematic Mapper (TM), Enhanced Thematic Mapper (ETM), and Operational Land Imager (OLI) of different time slices (1989 to 2016) were acquired from the USGS (http://glovis.usgs.gov), and their characteristics are given in Table 2. Similarly, 30 years climatic (temperature and rainfall) data for 1984–2013 of the nearest climatology station in Tikapur (N 28°32″, E 81°07″, and elevation 140 m amsl) were obtained from DHM office in Kathmandu.
Data analysis
Image pre-processing
The 2016 image was considered as a reference map, geometrically corrected, and registered to the UTM map coordinate system zone 45 north, Datum WGS-84. Other images (1989 and 2002) were registered using image to image registration technique. Similarly, dark object subtraction (DOS) and top of atmosphere (TOA) were done as a part of atmospheric correction, as the final outcome is expected to be more accurate on corrected images (Song et al. 2001). The images were subset to limit the processing up to the area of interest (AOI) that made image processing easier and helped in extracting the desired information.
Image processing and classification
All image processing was performed using the environment for visualizing image (ENVI) version 5.1, while maps were prepared using ArcGIS 10.2. For training and validation sampling, we used data obtained from field work, published map, and Google Earth. For 2016 image, field work data were used for training and validation sampling, whereas Google Earth and topographical map were utilized for 2002 and 1989 images, respectively. Supervised classification was done using maximum likelihood classification (MLC) algorithm to extract information on the identified land cover of the GLC catchment, using training samples (in the range of 50–65 points) taken randomly stratified by land cover class. The MLC is one of the most effective methods in supervised classification (Gao 1998; Green et al. 1998), which computes the weighted distance or likelihood of unknown measurement vector belonging to one of the known classes and is based on the Bayesian equation. On the basis of spectral signature–based satellite image classification, identification and distribution of land cover were analyzed.
Image post-processing and change detection analysis
We used post-classification comparison techniques to detect changes in land cover from the three imagery dates. It provides “from-to” change information and is the most common change detection method. Changes in land cover were mapped by subtracting the classified maps between three time periods: 1989–2002, 2002–2016, and 1989–2016. Change matrix was also developed to understand the dimension of the change.
Accuracy assessment
Validation of classification is an integral part of any image classification process (Sinha et al. 2014). We followed accuracy assessment method (Congalton 1991; Congalton and Green 2008) to estimate the accuracy of the image classification.
Results
Field observations, KIIs, and FGDs
Field observations through transect walk by the first author, with the help of two volunteers, witnessed many environmental issues in the study area. Major problems observed were aquatic floral invasion, over extraction of water from the lake and river channels using heavy water extraction pump throughout the year, river bank erosion, industrial activities such as brick kiln, illegal tree felling for timber, and fuelwood. Both KIIs and FGDs reported land cover and environmental changes over the years. During the FGDs, a majority of the participants expressed their view about an increase in atmospheric temperature over the last few years compared with the last 25 years. Participants also reported a decrease in the rainfall amount and intensity and an increase in abrupt and unpredictable rainfall pattern during the same period. The area has had rain-fed agriculture; however, nowadays, underground water is the main source for irrigation. Increased temperature and decreased rainfall in the area have affected water recharge capacity of Sita Kuwa, natural water well situated at the foothill of Siwalik range that supplies water to the lake. Invasion by some species such as Gandhe Jhar (Plectranthus barbatus) and Snails (Helix sp.) has increased drastically in the terrestrial ecosystem, and water hyacinth (Eicchornia crassipes) in the aquatic ecosystem—which locals attributed to the changing climate. Populations of some species of fish, such as Samar and Parni, have been reduced in the lake. A record of 249 bird species was mentioned during a KII (Pers. Comm. Ram Bahadur Chaudhary). However, it was learnt that Comeback Duck, locally called Nakata, were not seen for the last 7–8 years; the population of purple swamphen (Porphyrio porphyrio) has declined drastically these days, and lesser adjunct (Leptoptilos javanicus) has vanished from the area for a long time. Though the area used to be rich in wild animals due to a favorable habitat, the population is declining for marsh crocodile (Crocodylus palustris), bear (Ursus thibetanus), monkey (Macaca mulatta), leopard (Panthera pardus), spotted deer (Axis axis), blue bull (Bos gaurus), wild pig (Sus scrofa), and vulture (Gyps himalayensis). People observed less regeneration capacity of sal (Shorea robusta) and sissoo (Dalbergia sissoo), the major vegetation types of the GLC. Availability of wild food such as wild rice, wild mushroom, and wild vegetables was reduced upon which many indigenous people depend on for their livelihoods. Many people (in the KII, FGDs) viewed that settlement expansion in recent years has directly exerted pressure on the forests and their ecosystem services available in the GLC, especially on fuel wood, leaf litter, sal and lotus leaf, and fish.
Changing demographic pattern of the study area
The populations of the three VDCs that bound the GLC were 25,286 with 3442 households in 1991 (CBS 1991); 43,687 with 6110 households in 2001 (CBS 2001); and 60,531 with 10,587 households in 2011 (CBS 2011) (Fig. 2). The population has more than doubled, and the number of households has increased threefolds.
Satellite image analysis and interpretation
Fig. 3 shows the classified land cover map of GLC for the years 1989, 2002, and 2016, and the overall classification results are summarized in Table 3. The results indicate that agricultural land has the highest share of the total area of GLC in all years, followed by forest, river and banks, lakes, and settlements. Overall, the areas of forest, agricultural land, and lakes have been decreased by 8.73%, 4.40%, and 0.65%, respectively. However, settlement and river and bank areas have been increased by 361.11% and 87.70%, respectively.
The overall classification accuracies were found at 84.57, 89.35, and 90.54 and Kappa coefficient of 0.70, 0.85, and 0.86, respectively, for the years 1989, 2002, and 2016. The rates of change in the land use over the study period were found different (Table 3). In between 1989 and 2002, forest and lake areas decreased by 7.25% and 2.61%, respectively. However, during the same period, expansion of the river and bank (64.75%), settlement (44.44%), and agricultural land (0.75%) was observed. Similarly, during 2002–2016, agricultural land and forest decreased, and river and bank, settlement, and lake areas increased. Overall, settlement had the maximum increment and forest had the maximum reduction in area between 1989 and 2016 (Table 3). Fig. 4 shows the spatiotemporal dynamics of different land cover categories for the above three time periods.
Climatic (diurnal temperature and rainfall) variability in the GLC region
The historical trends of climatic variables, namely diurnal temperature range (DTR) and total annual rainfall, of the study area are shown in Fig. 5 a and b, respectively. Diurnal temperature range has been increasing at an annual rate of 0.0318 °C. On the other hand, total annual rainfall shows a slight decreasing trend (− 0.3381 mm/year) with drastic annual variation.
Discussion
This study shows a decrease in the forest land area (8.7%) and agricultural land area (4.4%), and a tremendous increase in the settlement area (361%) and river bank area (87.7%) during 1989–2016. The population of the study area during the past 20 years (1991–2011) has increased by almost 140% (CBS 2011). Increasing population demands more area to build houses, and it occurred through encroachment of forests and agricultural land. Terai plain of Nepal, which mostly lies below 610 m elevation, comprises only 23% of the total land area, however accommodated 50.3% of total population of the country (MoPE 2017). This shows an increased anthropogenic pressure on available natural resources in the Terai. Khanal (2009) also reported decreased forest land cover in the GLC during the 1990s. Similar result was observed in Koshi Tappu wetland areas where forests were reduced up to 94% within three-decade interval and mostly converted to grasslands with the significant impact on its ecosystem (Chettri et al. 2013). Decreased forest cover and water bodies have also been reported in wetlands of India as a result of increased settlement, for example, Hokersar wetland (Romshoo and Rashid 2014) and Dal wetland (Badar et al. 2013) and also in Inle Lake, Myanmar (Karki et al. 2018). Similarly, Zacharia et al. (2013) reported a significant shrinkage of lake area (almost 68%) over the period of 20 years with the rise in human population, built up structure, and consequent deforestation as well as commercial and subsistence farming in the vicinity of Lake Olbolosat in the central Kenya.
Alteration of biophysical processes such as land cover change could have strong negative effect on regional climate, which in turn is detrimental to local climate, water cycle, ecosystems and their productivity, and biodiversity (Perugini et al. 2017). Rivers and their sand banks in the GLC have increased by almost 88% during the past 30 years. This could be due to erosion and accretion process in the GLC catchment (Fig. 6e). The rivers, viz. Tenga in the west and Donga in the east flowing through GLC, are alluvial in nature, and lateral channel erosion leads to widening of river banks in alluvial rivers (Debnath et al. 2017). Furthermore, anthropogenic activities, such as near river bank agricultural production, water abstraction, and rampant sand mining for settlement and other developmental works undergoing in the GLC (Fig. 6b–d), could have led to the expansion of such sand banks. The expanding river channel bank has decreased the areal extent of GLC, thereby creating pressures through trade off among land uses. This has ultimately forced the inhabitants to encroach forest land for expanding settlements (Fig. 6f), thereby impacting overall ecosystem functions of the GLC in the long run including loss of wildlife habitats. Many respondents during the KIIs and FGDs agreed with the increasing pressure of the anthropogenic activities to ecosystem in the GLC. A global meta-analysis (van Asselen et al. 2013) observed agricultural development as a main proximate cause of wetland conversion, and economic growth and increasing population density are the most concerns. Hazarika et al. (2015) also reported erosion-accretion and river channel shift due to near river bank agricultural activities in the upper Brahmaputra plains in India. Ecological degradation of the wetlands due to land cover change and human activities is also indicated elsewhere, for example, in China (Gong et al. 2010; Xie et al. 2012; Wu et al. 2015; Zhao et al. 2015), Zambia (Munyati 2000), Iran (Ghobadi et al. 2015), Bangladesh (Sun et al. 2017), and Vietnam (Funkenberg et al. 2014).
Anthropogenic activities and ecological degradation at GLC. a Water hyacinth and other emergent plants covering lake water body. b Settlement and agricultural activities. c Brick kiln. d Water extraction near a lake for irrigation purpose. e River bank erosion and channel expansion at Donda river. f Fuel wood extraction and transportation at GLC (photos were taken by the first author during field visit in 2015)
The study also found that the area of GLC water body decreased very slightly (< 1%) over the 30-year period. This could be mainly due to its forested inland terrain nature and some lake restoration work carried out locally. However, local people observed degraded water level and its quality in the last few years (Fig. 6a), citing increasing degraded water turbidity arises from the wastes of nearby temple and visitor’s recreational activities, high density of aquatic plant invasion, and loss of many indigenous fishes.
In recent times, the frequency and intensity of weather extremes have been increasing across Nepal (Shrestha et al. 2016; Karki et al. 2017). Both temperature and rainfall records of the study area show variable trends over the last 30 years. The DTR (Fig. 5a) has been increasing, while total annual rainfall pattern (Fig. 5b) shows a slight decreasing trend over the same period. A recent study (Thapa et al. 2015) also reported increased temperature and decreased rainfall in the same area. A similar trend was observed in the adjoining North Indian regions (Sinha 2011; Behera et al. 2012). Climate change is expected to cause significant changes to temperature regimes and rainfall patterns that could pose serious risks for inland freshwater wetland ecosystem such as GLC, where people are benefitting from its several ecosystem services. Climate change could have direct impact on the hydrological dynamics of wetlands by reducing water discharge from watersheds and increasing evapotranspiration which could have severe implication in the overall wetland ecosystem (Zhu et al. 2017). A big natural water well/pit, viz. Sita Kuwa, and two seasonal rivers, viz. Tenga and Donda close to the GLC boundary, help to maintain water table and water source of the study area. A likely change in the water discharge of the rivers with future warming could have serious impacts on the hydrological dynamics of the GLC. For instance, Dahal et al. (2019) reported a possible climate-induced water security concerns in Nepalese watershed because of the altered hydrological processes in some river systems. They reported a case of Roshi watershed of central Nepal where recent changes in temperature and precipitation influenced dry and wet spell, leading to decline in annual and seasonal streamflow of the river.
Based on the analysis of people’s observation, existing anthropogenic pressure, land cover changes, and climatic variability trends, it is clear that ecological condition of the GLC is currently in a degraded state and will continue so if prompt action is not put in place. High settlement demand for the booming local population has increased encroachment of both forests and fertile agricultural land. The forests of GLC used to be a safe habitat for diverse flora and fauna; many of them are endangered species (for details, please refer to IUCN 1998; Kafle and Savillo 2009; Lamsal et al. 2014). The contraction of forests as revealed by our analysis may have detrimental impacts on such species. Degradation of biological diversity could have direct impact on the socio-ecological system of the wetlands. Increasing population with decreasing agricultural land means intensification of agriculture practices using high rates of chemical fertilizers and pesticides. Improper application of chemical fertilizers and pesticides could degrade the soil fertility of the area and increase the chemical pollution into the lake water through surface flow and sub-surface leaching of such chemicals. The chemical fertilizers and pesticides would harm structural and functional diversities of microbial populations that in turn affect numerous soil functions and integrity (Prashar and Shah 2016). Invasion by water hyacinth, for example, has expanded all over the water surface of the lake in recent years. High chemical concentration also hampered the aquatic habitat of fish and other animals. A household of Nepal applies 100 kg of chemical fertilizer in the agriculture land and such application is almost four times higher in Terai region compared with the hills (Shrestha 2010). Increased agricultural productivity obtained through extensive use of fertilizers, for example, has triggered decline in aquatic vegetation, shrinkage of wetland catchment, and heavy water hyacinth infestation in Samaspur wetland ecosystem of India over the period of 30 years (Behera et al. 2012).
The study clearly shows an increase in temperature in the GLC region for the last three decades. The change in rainfall is not clearly observed in the nearby station of Tikapur. Changes in climatic patterns have caused wetland ecological degradation in many regions of the world. For example, Werner et al. (2013) reported a shorter hydroperiod and less vegetation dynamic cycles affecting the productivity of numerous aquatic species in the wetlands of Prairie Pothole Region (PPR) in Canada. Likewise, Rashford et al. (2014) reported decreased productivity at PPR of Dakota, USA, with + 4 °C warming. Similarly, diurnal temperature range (DTR) is an important indicator for climate change (Karl et al. 2004). Englehart and Douglas (2005) mentioned that regional land cover change, to some extent, is a potential forcing mechanism for DTR, including forest cover losses (Alkama and Cescatti 2016). Such temperature variability could impact the population viability of many endothermic animals (Briga and Verhulst 2015) and disrupt plant development and productivity including pollination (Hatfield and Prueger 2015). It should be noted that in future, the impact of climatic change on wetland habitats will act differentially on a regional and mega-watershed level (Erwin 2009). This study considers only two variables, DTR and annual rainfall, in analyzing climatic variability trend of the GLC. Therefore, it is hard for the present study to remark such degradation simply based on the two historic climatic trends; however, overlooking such climate-based ecological damage in the GLC is also not justified.
There are few community conservation activities carried out at GLC by local community-based organizations (CBOs) through a support of some international initiatives such as International Union for Nature Conservation (IUCN), World Wildlife Fund (WWF), and United Nation Development Program (UNDP); however, looking at the current state of degradation, those efforts seem insufficient. A community-based conservation program has been advocated for wetland conservation (Bamford et al. 2017; Lopez-Maldonado and Berkes 2017; Wali et al. 2017) and GLC management (Lamsal et al. 2015a). Local communities of GLC are willing to participate in the conservation activities (Lamsal et al. 2015b). The recreational potential of the GLC for visitors is immense (Lamsal et al. 2016). Nepal government recently adopted a National Ramsar Strategy and Action Plan for the years 2018–2024 (MoFE 2018), which plans to conserve, wise use, restore, and ensure benefits to local communities, primarily engaging local-, federal-, and state-evel stakeholders. The current increasing population trend (Fig. 2) will expedite unsustainable resource extraction from the complex, which coupled with climatic variability threatens ecosystem supply potential and endangering biodiversity that will have longer term impact on local livelihood (Fig. 7). Livelihood of the Tharu ethnic community in the study area is mostly based on the GLC resources. Lamsal et al. (2015a) reported a significant contribution of GLC resources to the livelihood of local people, supplying fuelwood, timber, fish, leaf litter, and lotus leaf among others, contributing 13% of their total household annual income.
Finally, it is worth to note two limitations of this study, viz. the use of low-resolution Landsat satellite images of longer interval for land cover change and consideration of only two climatic variables, i.e., DTR and annual rainfall. Future studies should try to incorporate high-resolution images of short interval to assess land cover change and more climatic variables to gain an in-depth insight on micro-climatic change and wetland resource degradation.
Conclusion and recommendations
This study has outlined the importance of wetland ecosystems in Nepalese context along with current degradation trend, citing a case of GLC. Multiple resources are derived from the GLC to sustain livelihoods in the study area, signifying their high dependence. Ecological condition of the area, on the other hand, is being degraded in recent times in the form of disappearance of floral and faunal species and emergence of invasion since the last few decades through anthropogenic activities. Alteration of important land cover such as contraction of forest and agricultural land and expansion of settlement in the recent past is a serious concern. Meteorological data further revealed the variability in micro-scale temperature and rainfall patterns that could possibly have serious consequences on the overall biodiversity of the GLC.
In general, this study could help the local-, regional-, and national-level natural resources policy makers in order to prepare a future management plan of the GLC. Having said that, wise use of GLC resources should be continued along with community-based conservation activities through the adoption of socio-ecological system and adaptive management concept by considering increasing population and degraded resource circumstance. All the programs to be implemented both by the government and development partners should strive to make locals aware of the importance of protecting biodiversity in the GLC in order to maintain healthy local ecosystem and ensure sustainable flow of lake-based ecosystem services for human welfare. As the GLC bears high significance in terms of tourism development, the evidence of changes in climatic variability as shown in this study should be seriously considered and start initiating more researches with a focus to the impact of climatic change on its biodiversity. We also suggest concerned authorities utilizing remote sensing technology for continuous monitoring of the GLC resources that could provide a sound basis of resource management in the long run. More specifically, following five recommendations are offered for immediate consideration.
-
1.
In the context of booming population and increasing wetland resource demands in the GLC, monitoring of current and likely future condition is a must, where application of remote sensing can play a role. The land cover change map could be a useful resource for natural resource managers and policy makers of the region for utilizing in current as well as future integrated wetland complex resource planning and management.
-
2.
Ecological restoration of the GLC should be a prime objective to be incorporated in any future lake management plans and programs. Conservation and wise use of GLC through efficient management systems are needed at present. This necessitates good conservation partnership effort between government, private organizations, and local community agencies.
-
3.
Environmental awareness is still lacking. Therefore, more awareness targeting locals—both males and females as well as school children—is necessary on climate change and its likely impact on the GLC resources in addition to the pressure from escalating anthropogenic activities. The attitude of people on conservation needs to be changed, and local conservation organizations need to be motivated to play a role on it.
-
4.
Supporting local people for expanding sustainable tourism activities targeting domestic visitors could be a good viable livelihood alternative for local dependent people. The GLC is a Ramsar-listed wetland that may not only attract domestic visitors but also could fascinate international visitors because of its unique biodiversity and rich ethnic Tharu culture. It is easily accessible by road transport and geographically situated in between two western lowland protected areas (PAs), viz. Bardia National Park and Suklaphanta Wildlife Reserve. Thus, implementing an integrated tourism package involving both the PAs and the GLC would be a useful initiative for the region.
-
5.
Setting a buffer zone between the GLC and human settlement and realignment of east-west highway away from the lake are necessary for the longer term sustainable ecological restoration that supports biodiversity and maintains continuous flow of ecosystem services.
Notes
Following the new constitution, in 2015, all old administrative divisions (eg.municipalities and VDCs) were restructured into new 7 provinces, and 753 municipalities and rural municipalities. At present, the GLC lies in Ghodaghodi Municipality.
References
Alkama, R., & Cescatti, A. (2016). Biophysical climate impacts of recent changes in global forest cover. Science, 351(6273), 600–604.
Aryal, R. R., Shrestha, H. L., & Khanal, S. (2011). Using Landsat data for assessing forest cover change and fragmentation in Laljhadi corridor of Kanchanpur district, Nepal. Banko Jankari, 21(2), 40–44.
Badar, B., Romshoo, S. A., & Khan, M. A. (2013). Modelling catchment hydrological responses in a Himalayan lake as a function of changing land use and land cover. Journal of Earth System Science, 122(2), 433–449.
Bajracharya, S. R., & Mool, P. (2009). Glaciers, glacial lakes and glacial lake outburst floods in the Mount Everest region, Nepal. Annals of Glaciology, 50(53), 81–86.
Bamford, A. J., Razafindrajao, F., Young, R. P., & Hilton, G. M. (2017). Profound and pervasive degradation of Madagascar’s freshwater wetlands and links with biodiversity. PLoS ONE, 12(8), e0182673.
Behera, M. D., Chitale, V. S., Shaw, A., Roy, P. S., & Murthy, M. S. R. (2012). Wetland monitoring, serving as an index of land use change: a study in Samaspur Wetlands, Uttar Pradesh, India. Journal of the Indian Society of Remote Sensing, 40(2), 287–297.
Briga, M., & Verhulst, S. (2015). Large diurnal temperature range increases bird sensitivity to climate change. Scientific Reports, 5, 16600. https://doi.org/10.1038/srep16600.
CBS. (1991). National population and housing census 1991 report. Kathmandu, Nepal: Central Bureau of Statistics, National Planning Commission Secretariat, Government of Nepal.
CBS. (2001). National population and housing census 2001 report. Kathmandu, Nepal: Central Bureau of Statistics, National Planning Commission Secretariat, Government of Nepal.
CBS. (2011). National population and housing census 2011 report. Kathmandu, Nepal: Central Bureau of Statistics, National Planning Commission Secretariat, Government of Nepal.
Chaudhary, S., Chettri, N., Uddin, K., Khatri, T. B., Dhakal, M., Bajracharya, B., & Ning, W. (2016). Implications of land cover change on ecosystem services and people’s dependency: a case study from the Koshi Tappu wildlife reserve, Nepal. Ecological Complexity, 28, 200–211.
Chettri, N., Uddin, K., Chaudhary, S., & Sharma, E. (2013). Linking spatio-temporal land cover change to biodiversity conservation in the Koshi Tappu Wildlife Reserve, Nepal. Diversity, 5, 335–351.
Clair, T. A. (1998). Canadian freshwater wetlands and climate change. Climatic Change, 40, 163–165.
Congalton, R. G. (1991). A review of assessing the accuracy of classifications of remotely sensed data. Remote Sensing of the Environment, 37(1), 35–46.
Congalton, R. G., & Green, K. (2008). Assessing the accuracy of remotely sensed data: principles and practices. New York, NY, USA: CRC Press.
Dahal, N., Shrestha, U. B., Tuitui, A., & Ojha, H. R. (2019). Temporal changes in precipitation and temperature and their implications on the streamflow of Roshi River, central Nepal. Climate, 7(1), 3. https://doi.org/10.3390/cli7010003.
Davidson, N. C. (2014). How much wetland has the world lost? Long term and recent trends in global wetland area. Marine and Freshwater Research, 65, 934–941.
Debnath, J., Das, N., Ahmed, I., & Bhowmik, M. (2017). Channel migration and its impact on land use/land cover using RS and GIS: a study on Khowai River of Tripura, North East India. The Egyptian Journal of Remote Sensing and Space Sciences, 20, 197–210. https://doi.org/10.1016/j.ejrs.2017.01.009.
DoF. (2017). Wetlands of western Nepal: a brief profile of selected lakes (p. 281). Kathamndu, Nepal: Department of Forest, Babarmahal.
DoFD. (2012). Country profile – Nepal 2011/2012, fisheries sub-sector. Kathmandu, Nepal: Directorate of Fisheries Development, Kathmandu.
Englehart, P. J., & Douglas, A. V. (2005). Changing behavior in the diurnal range of surface air temperatures over Mexico. Geophysical Research Letters, 32, L01701.
Erwin, K. L. (2009). Wetlands and global climate change: the role of wetland restoration in a changing climate. Wetland Ecology and Management, 17, 71–84.
Fei, L., Shuwen, Z., Kun, B., Jiuchun, Y., Qing, W., & Liping, C. (2015). The relationships between land use change and demographic dynamics in western Jilin province. Journal of Geographical Science, 25(5), 617–636.
Funkenberg, T., Binh, T. T., Moder, F., & Dech, S. (2014). The Ha Tien Plain: wetland monitoring using remote sensing. International Journal of Remote Sensing, 35(8), 2893–2909.
Gallant, A. L. (2015). The challenges of remote monitoring of wetlands. Remote Sensing, 7, 10938–10950.
Gao, J. (1998). A hybrid method towards accurate mapping of mangroves in a marginal habitat from SPOT multispectral data. International Journal of Remote Sensing, 19(10), 1887–1899.
Gardner, R. C., Barchiesi, S., Beltrame, C., Finlayson, C. M., Galewski, T., Harrison, I., Paganini, M., Perennou, C., Pritchard, D. E., Rosenqvist, A., & Walpole, M. (2015). State of the world’s wetlands and their services to people: a compilation of recent analyses. Gland, Switzerland: Ramsar Briefing Note no. 7, Ramsar Convention Secretariat.
Ghobadi, Y., Pradhan, B., Shafri, H. Z. M., Ahmad, N. B., & Kabiri, K. (2015). Spatio-temporal remotely sensed data for analysis of the shrinkage and shifting in the Al Hawizeh wetland. Environmental Monitoring and Assessment, 187, 41–56.
Gong, P., Niu, Z., Xiao, C., et al. (2010). China’s wetland change (1990-2000) determined by remote sensing. Science China Earth Sciences, 53(7), 1036–1042.
Green, E. P., Clark, C. D., Mumby, P. J., Edwards, A. J., & Ellis, A. (1998). Remote sensing techniques for mangrove mapping. International Journal of Remote Sensing, 19(5), 935–956.
Hartter, J., & Southworth, J. (2009). Dwindling resources and fragmentation of landscapes around parks: wetlands and forest patches around Kibale National Park, Uganda. Landscape Ecology, 24, 643–656.
Hatfield, J. L., & Prueger, J. H. (2015). Temperature extremes: effect on plant growth and development. Weather and Climate Extreme, 10, 4–10.
Hazarika, N., Das, A. K., & Borah, S. B. (2015). Assessing land-use changes driven by river dynamics in chronically flood affected Upper Brahmaputra plains, India, using GIS-RS techniques. The Egyptian Journal of Remote Sensing and Space Science, 18(1), 107–118.
Hu, S., Niu, Z., Chen, Y., Li, L., & Zhang, H. (2017). Global wetlands: potential distribution, wetland loss, and status. Science of the Total Environment, 586, 319–327. https://doi.org/10.1016/j.scitotenv.2017.02.001.
IUCN. (1998). An inventory of Nepal’s Tarai Wetlands. Kathmandu: IUCN Nepal.
Junk, W. J., An, S., Finlayson, C. M., Gopal, B., Kvet, J., Mitchell, S. A., Mitsch, W. J., & Robarts, R. D. (2013). Current state of knowledge regarding the world’s wetlands and their future under global climate change: a synthesis. Aquatic Sciences, 75, 151–167.
Kafle, G. (2005). Avifaunal survey and vegetation analysis focusing on threatened and near-threatened species on Ghodaghodi Lake of Nepal. Bedford: A Report Submitted to Oriental Bird Club (OBC).
Kafle, G., & Savillo, I. T. (2009). Present status of Ramsar sites in Nepal. International Journal of Biodiversity and Conservation, 1(5), 146–150.
Karki, R., Hassan, S. U., Schickhoff, U., Scholten, T., & Bohner, J. (2017). Rising precipitation extremes across Nepal. Climate, 5(1). https://doi.org/10.3390/cli5010004.
Karki, S., Thandar, A. M., Uddin, K., Tun, S., Aye, W. M., Aryal, K., Kandel, P., & Chettri, N. (2018). Impact of land use land cover change on ecosystem services: a comparative analysis on observed data and people perception in Inle Lake, Myanmar. Environmental System Research, 7, 25. https://doi.org/10.1186/s40068-018-0128-7.
Karl, B., Karoly, D. J., & Arblaster, J. M. (2004). Diurnal temperature range as an index of global climate change during the twentieth century. Geophysical Research Letters, 31, L13217.
Kayranli, B., Scholz, M., Mustafa, A., & Hedmark, A. (2010). Carbon storage and fluxes within freshwater wetlands: a critical review. Wetlands, 30, 111–124.
Khanal, S. (2009). Change assessment of forest cover in Ghodaghodi Lake area in Kailali district of Nepal. Banko Jankari, 19(2), 15–19.
Lamsal, P., Pant, K. P., Kumar, L., & Atreya, K. (2014). Diversity, uses, and threats in the Ghodaghodi Lake Complex, a Ramsar site in western lowland Nepal. ISRN Biodiversity, 680102, 12.
Lamsal, P., Atreya, K., Pant, K. P., & Kumar, L. (2015a). An analysis of willingness to pay for community based conservation activities at the Ghodaghodi Lake Complex, Nepal. International Journal of Biodiversity Science, Ecosystem Services & Management, 11, 341–348.
Lamsal, P., Pant, K. P., Kumar, L., & Atreya, K. (2015b). Sustainable livelihood through conservation of wetland resources: a case of economic benefits from Ghodaghodi Lake, western Nepal. Ecology and Society, 20(1), 10.
Lamsal, P., Atreya, K., Pant, K. P., & Kumar, L. (2016). Tourism and wetland conservation: application of travel cost and willingness to pay an entry fee at Ghodaghodi Lake Complex, Nepal. Natural Resources Forum, 40, 51–61.
Lamsal P., Atreya K., Pant K.P., & Kumar L. (2017) People’s Dependency on Wetlands: South Asia Perspective with Emphasis on Nepal. In: Prusty B., Chandra R., Azeez P. (eds) Wetland Science. Springer, New Delhi
Lopez-Maldonado, Y., & Berkes, F. (2017). Restoring the environment, revitalizing the culture: cenote conservation in Yucatan, Mexico. Ecology and Society, 22(4), 7.
Mabwoga, S. O., & Thukral, A. K. (2014). Characterization of change in the Harike wetland, a Ramsar site in India, using Landsat satellite data. SpringerPlus, 3, 576. https://doi.org/10.1186/2193-1801-3-576.
Mitsch, W. J., Bernal, B., Nahlik, A. M., Mander, U., Zhang, L., Anderson, C. J., Jorgensen, S. E., & Brix, H. (2013). Wetlands, carbon, and climate change. Landscape Ecology, 28, 583–597.
MoFE. (2018). National Ramsar Strategy and Action Plan, Nepal (2018-2024). Ministry of Forests and Environment. Kathmandu, Nepal: Singha Durbar.
MoPE. (2017). National Population Repot. Ministry of Population and Environment (p. 274). Kathmandu: Singha Durbar.
Munyati, C. (2000). Wetland change detection on the Kafue Flats, Zambia, by classification of a multitemporal remote sensing image dataset. International Journal of Remote Sensing, 21(9), 1787–1806.
Ozesmi, S. L., & Bauer, M. E. (2002). Satellite remote sensing of wetlands. Wetland Ecology and Management, 10, 381–402.
Papastergiadou, E., Kagalou, I., Stefanidis, K., Retalis, A., & Leonardos, I. (2010). Effects of anthropogenic influence on the trophic state, land uses and aquatic vegetation in a shallow Mediterranean lake: implication for restoration. Water Resources Management, 24, 415–435.
Perugini, L., Caporaso, L., Marconi, S., Cescatti, A., Quesada, B., de Noblet-Ducoudre, N., House, J. I., & Arneth, A. (2017). Biophysical effects on temperature and precipitation due to land cover change. Environmental Research Letters, 12, 053002.
Prashar, P. & Shah, S. (2016). Impact of fertilizers and pesticides on soil microflora in agriculture. In: E. Lichtfouse (ed.), Sustainable agriculture reviews, Vol 19, Springer, Cham. 331-361, https://doi.org/10.1007/978-3-319-26777-7_8
Prigent, C., Papa, F., Aires, F., Jimenez, C., Rossow, W. B., & Matthews, E. (2012). Changes in land surfaces water dynamics since the 1990s and relation to population pressure. Geophysical Research Letters, 39, L08403.
Rahel, F. J., & Olden, J. D. (2008). Assessing the effects of climate change on aquatic invasive species. Conservation Biology, 22(3), 521–533.
Rashford, B. S., Adams, R. M., Wu, J., Voldseth, R. A., Guntenspergen, G. R., Werner, B., & Johnson, W. C. (2014). Impacts of climate change on land use and wetland productivity in the Prairie Pothole Region of North America. Regional Environmental Change, 16, 515–526.
Reis, V., Hermoso, V., Hamilton, S. K., Ward, D., Fluet-Chouinard, E., Lehner, B., & Linke, S. (2017). A global assessment of inland wetland conservation status. BioScience, 67(6), 523–533.
Rimal, B., Baral, H., Stork, N. E., Paudyal, K., & Rijal, S. (2015). Growing city and rapid land use transition: assessing multiple hazards and risks in the Pokhara Valley, Nepal. Land, 4, 957–978.
Romshoo, S. A., & Rashid, I. (2014). Assessing the impacts of changing land cover and climate on Hokersar wetland in Indian Himalayas. Arabian Journal of Geosciences, 7(1), 143–160.
Ruan, R., Zhang, Y., & Zhou, Y. (2008). Change detection of wetland in Hongze Lake using a time series of remotely sensed imagery. The international archives of the photogrammetry, remote sensing and spatial information sciences, Vol. XXXVII, Part B7. Beijing: 1545–1548.
Sah, J. P., & Heinen, J. T. (2001). Wetland resource use and conservation attitudes among indigenous and migrant peoples in Ghodaghodi Lake area, Nepal. Environmental Conservation, 28(4), 345–356.
Shrestha, R. K. (2010). Fertilizer policy development in Nepal. The Journal of Agriculture and Environment, 11, 126–137.
Shrestha, A. B., & Joshi, S. P. (2009). Snow cover and glacier change study in Nepalese Himalaya using remote sensing and geographic information system. Journal of Hydrology and Meteorology, 6(1), 26–36.
Shrestha, A. B., Bajracharya, S. R., Sharma, A. R., Duo, C., & Kulkarni, A. (2016). Observed trends and changes in daily temperature and precipitation extremes over the Koshi river basin 1975-2010. International Journal of Climatology, 37(2), 1066–1083.
Sinha, C. P. (2011). Climate change and its impacts on the wetlands of North Bihar, India. Lakes & Reservoirs: Research and Management, 16, 109–111.
Sinha, P., Kumar, L., Drielsma, M., & Barrett, T. (2014). Time-series effective habitat area (EHA) modeling using cost-benefit raster based technique. Ecological Informatics, 19, 16–25.
Song, C., Woodcock, C. E., Seto, K. C., Lenny, M. P., & Macomber, S. A. (2001). Classification and change detection using Landsat TM data: when and how to correct atmospheric effects? Remote Sensing of Environment, 75(2), 230–244.
Sun, C., Zhen, L., & Miah, G. (2017). Comparison of ecosystem services provided by China’s Poyang Lake wetland and Bangladesh’s Tanguar Haor wetland. Ecosystem Services, 26, 411–421.
Thapa, R. B., & Murayama, Y. (2009). Examining spatiotemporal urbanization patterns in Kathmandu Valley, Nepal: remote sensing and spatial metrics approaches. Remote Sensing, 1(3), 534–556.
Thapa, L. B., Thapa, H., & Magar, B. G. (2015). Perceptions, trends and impacts of climate change in Kailali district, far west Nepal. International Journal of Environment, 4(4), 62–76.
van Asselen, S., Verburg, P. H., Vermaat, J. E., & Janse, J. H. (2013). Drivers of wetland conversion: a global meta-analysis. PLoS ONE, 8(11), e81292.
Wali, A., Alvira, D., Tallman, P. S., Ravikumar, A., & Macedo, M. O. (2017). A new approach to conservation: using community empowerment for sustainable well-being. Ecology and Society, 22(4), 6.
Werner, B. A., Johnson, W. C., & Guntenspergen, G. R. (2013). Evidence for 20th century climate warming and wetland drying in the North American Prairie Pothole Region. Ecology and Evolution, 3(10), 3471–3482.
Whitehead, P. G., Wilby, R. L., Battarbee, R. W., Kernan, M., & Wade, A. J. (2009). A review of the potential impacts of climate change on surface water quality. Hydrological Sciences Journal, 54(1), 101–123.
Woodward, G., Perkin, D. M., & Brown, L. E. (2010). Climate change and freshwater ecosystems: impacts across multiple level of organizations. Philosophical Transactions of the Royal Society B, 365, 2093–2106.
Wu, G., Gao, Y., Wang, Y., Wang, Y., & Xu, D. (2015). Landuse/landcover changes and their driving forces around wetlands in Shangri-La County, Yunnan Province, China. International Journal Sustainable Development and World Ecology, 22(2), 110–116.
Xie, Z., Liu, J., Ma, Z., Duan, X., & Cui, Y. (2012). Effect of surrounding land use change on the wetland landscape pattern of a natural protected area in Tianjin, China. International Journal of Sustainable Development and World Ecology, 19(1), 16–24.
Zacharia, M., Elias, A., Jeremiah, K., Simon, M., & Olang, L. O. (2013). Assessment of land cover changes in Lake Olbolosat Region of the Central Kenyan Highlands using Landsat satellite imagery aided by indigenous knowledge. Journal of Biodiversity Management & Forestry, 2, 2.
Zhao, Z., Zhang, Y., Liu, L., Liu, F., & Zhang, H. (2015). Recent changes in wetlands on the Tibetan Plateau: a review. Journal Geographical Sciences, 25(7), 879–896.
Zhu, J., Sun, G., Li, W., Zhang, Y., Miao, G., Noormets, A., McNulty, S. G., King, J. S., Kumar, M., & Wang, X. (2017). Modeling the potential impacts of climate change on the water table level of selected forested wetlands in the southeastern United States. Hydrology and Earth System Science, 21, 6289–6305.
Author information
Authors and Affiliations
Corresponding author
Additional information
Publisher’s note
Springer Nature remains neutral with regard to jurisdictional claims in published maps and institutional affiliations.
Rights and permissions
About this article
Cite this article
Lamsal, P., Atreya, K., Ghosh, M.K. et al. Effects of population, land cover change, and climatic variability on wetland resource degradation in a Ramsar listed Ghodaghodi Lake Complex, Nepal. Environ Monit Assess 191, 415 (2019). https://doi.org/10.1007/s10661-019-7514-0
Received:
Accepted:
Published:
DOI: https://doi.org/10.1007/s10661-019-7514-0