Abstract
Soils contaminated with heavy metals may pose a threat to environment and human health if metals enter the food chain over and above threshold levels. In general, there is a lack of information on the presence of heavy metals in tea [Camellia sinensis (L). O. Kuntze] plants and the soils in which they are grown. Therefore, an attempt was made to establish a database on the important heavy metals: cadmium (Cd), chromium (Cr), nickel (Ni), and lead (Pb). For an initial survey on heavy metals, soil samples were collected randomly from tea-growing areas of Tamil Nadu, Kerala, and Karnataka, India. Parallel studies were conducted in the greenhouse on uptake of Pb, Cd, and Ni from soils supplemented with these metals at different concentrations. Finally, metal distribution in the tea plants under field conditions was also documented to assess the accumulation potential and critical limit of uptake by plants.
Similar content being viewed by others
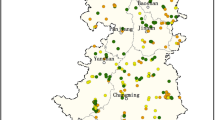
Explore related subjects
Discover the latest articles, news and stories from top researchers in related subjects.Avoid common mistakes on your manuscript.
Introduction
Tea (Camellia sinensis (L). O. Kuntze) plantations, located close to forest ecosystems in the Western Ghats in South India, have a predominant role in the maintenance of terrestrial ecology by providing extensive land cover, preventing soil erosion, and providing a non-polluting atmosphere. It is well known that macronutrients and micronutrients are required at different stages of tea plant growth and development of tea plants. Synthetic fertilizers and mined products are extensively applied to tea fields to increase productivity. Over the years, application of inorganic fertilizers has dominated day-to-day agricultural activities. Concomitant with the increase in tea productivity in south India, refinements have also been made in the fertilizer use policy to achieve higher productivity (Verma and Palani 1995).
Tea is a calcifuge crop and does not grow well in soil with high base saturation. However, a certain amount of calcium is required for growth and productivity. This is met by applying dolomitic lime to the soil. Among the secondary nutrients, magnesium is of vital importance. Application of dolomitic lime to correct soil acidity has become a regular practice in south India, and this also prevents the occurrence of Mg deficiency to some extent. Magnesium sulphate (MgSO4) is also given as foliar spray along with zinc sulphate (ZnSO4) (Verma 1995). Sulphur is another vital nutrient; the soil application of MgSO4 also contributes to the sulphur requirement of plants. Iron and manganese are abundantly available in the acidic soils; their deficiencies are uncommon. Adequate amounts of boron and molybdenum are available in most tea-growing soils, and so they are not additionally required.
Although some trace elements are essential for metabolic activities of living organisms, many of them are toxic at higher concentrations. Traces of toxic elements enter the food chain from the soil. Soil pollution by heavy metals has become a critical environmental problem due to its potential adverse ecological effects (Kabata-Pendias and Pendias 1992). Heavy metals are present in the earth crust and can enter the food chain through plants. Higher levels of these metals in soil affect not only plant growth but also soil biochemical processes. Decomposition of organic matter in soil is inhibited at sites polluted with trace elements. Nitrogen mineralization and nitrification are also inhibited by the presence of heavy metals.
In recent years, various reports have been published on trace elements in soils (Kabata-Pendias 2000). Metal interactions vary considerably with soil type. Availability of metals is determined by the nature of the metal species; its interaction with soil colloids; soil characteristics such as soil pH, clay, organic matter, and moisture content; and duration of contact with the surface. Soil characteristics determine availability of trace elements to plants by controlling speciation, temporary binding by particle surfaces, and precipitation reactions in the soil solution. The dominant role of soil pH in determining the availability of metals in the soil solution and uptake by plants has been well documented (Alloway 1995; Adriano 2001; Jin et al. 2005).
It appears that trace element content in soils is increasing steadily and agricultural, environmental, and governmental agencies are paying close attention to safeguard the environment from these potential pollutants, particularly heavy metals. Naturally, soils accumulate these trace elements through industrial, farming, and anthropogenic activities. Criteria for environmental protection related to the trace elements in soils should consider all the variables that govern their behavior and, in particular, soil-plant interactions (Pendias 2004).
Unlike the other crop plants, tea is harvested at regular intervals where replenishment of nutrients is indispensable. The essentiality of major and minor elements is well known, and they play a paramount role in metabolic activities and crop productivity (Mengel and Kirkby 1987). In order to achieve higher crop productivity, several refinements have been made over the ages. As a result, new policies on fertilizer use have been evolved to meet the nutrient requirements of tea plants (Ranganathan and Natesan 1985; Verma and Palani 1997; Venkatesan 2007).
Han et al. (2006) investigated the magnitude and causes of Pb contamination in Chinese tea. They found that levels of Pb concentration in tea leaves were positively correlated with soil-exchangeable Pb and negatively correlated with soil pH, indicating the ease of mobilization of Pb from soils. Information on levels of heavy metals in south Indian tea soils is not available. In view of the above, a study was undertaken to determine the heavy metal status of south Indian tea soils. In order to evaluate the impact of heavy metals on tea plants, a parallel study on metal uptake by tea plants was carried out in a greenhouse.
Materials and methods
Reagents
Spectroscopy grade acids for atomic absorption spectroscopy, while other reagents/matrix modifiers such as ammonium dihydrogen phosphate and magnesium nitrate, were obtained from Merck (Darmstadt, Germany) and ACROS Organics, Belgium, respectively. Analytical reagent (AR) grade acids were purchased from S.D. Fine Chemicals, Mumbai, India.
Stock standard solutions
Single element standards of heavy metals (Pb, Cd, Ni, and Cr) at 1000 mg/l were purchased from Merck, Germany. Secondary standards of heavy metals were prepared in 0.1 M HNO3 as and when required.
Soil sampling in different tea-growing districts
In order to develop a database on heavy metal content in tea soils of south India, a survey was conducted. Soil samples were collected from 2005 to 2009 from all the tea-growing areas of south India. Both top (0–9”) and bottom (9–18”) soils (300 samples × 250 g each) were collected as per a standard procedure (Bhargava and Raghupathi 2001). The samples were packed, legibly labeled, and sent to the Tea Research Institute, Valparai, for analysis.
Uptake of Pb, Cd, and Ni by young tea plants
An experiment in randomized block design was conducted in a tea nursery using potted tea plants to generate data on the absorption of heavy metals by young tea plants. There were six treatments, each replicated in four blocks each with tea plants. Treatments included untreated control, external addition of lead at 5, 10, 25, 50, and 100 mg/kg soil as lead (II) nitrate Pb (NO3)2. Required quantity of Pb (NO3)2 was weighed in a micro-electronic balance (Shimadzu, AW-220) and dissolved in 100 ml of water. Dissolved salt was mixed thoroughly with 5 kg of nursery soil and air dried. Plastic pots were filled with soils according to treatment. One-year-old plants (cultivar UPASI-9) were transplanted into the pots and watered adequately. Care was taken to avoid leaching loss from the pots. Plants were maintained in a tea nursery. Control soil, weighing about 200 g, was analyzed for physico-chemical parameters. Similar experiments were conducted for Cd and Ni accumulation, using cadmium chloride (CdCl2) and nickel (II) chloride (NiCl2) as external additives. Plants were sampled on the 30th, 60th, 120th, and 150th day after imposing treatments. At each sampling, leaves, stems, and roots of the tea plants were separated individually and washed with tap water and then with deionized water. Plant samples were dried at 70 °C in an oven until they attained constant weight. Samples were powdered and packed individually until analysis. Soil samples were stored prior to analysis to document the retention of heavy metals. The metal Cr was not included in the nursery experiments; Cr contamination is mainly due to tea processing (Natesan and Ranganathan 1990; Seenivasan et al. 2008).
Vertical distribution of heavy metals in field grown tea plants
Plants of the cultivar UPASI-9, planted in 1964 at the United Planters’ Association of Southern India, Tea Research Institute (UPASI-TRI) Experimental Farm, were selected to study the vertical distribution of heavy metals in plants. A block consisting of 40 bushes was demarcated, and from the bushes crop shoots (three leaves and a bud), mature leaves, small stem (bare stem measuring <1.0 cm diameter), thick wood, and roots (pencil-thick samples with feeder roots) were collected. During the period of study, sampling was carried out three times from 10 randomly selected bushes. After sampling, all plant parts were treated as described earlier for Cd, Ni, and Pb. Soil samples were also collected at the same time and stored until analysis.
Atomic absorption spectroscopy
Soil samples were analysed for Cr, Ni, Cd, and Pb using atomic absorption (AA Analyst 800, PerkinElmer Corporation, USA) equipped with flame and graphite furnace. In flame mode, samples containing very low metal concentrations (not detectable range) could not be quantified while the same lower elemental concentration could be determined using graphite furnace (GF-AAS) mode. Combination of air-acetylene gas flame was used for determination of metals. The instrument was operated under the following conditions in flame mode: acetylene = 2 ml/min, air = 17 ml/min. In graphite furnace mode, inert argon gas flow and the temperature parameters were followed as recommended by manufacturers (Perkin-Elmer 2000).
Analysis
Determination of heavy metals in soils
Air-dried soil (0.25 g) was weighed into a pre-cleaned Teflon® vessel. To the soil, 8.0 ml of aqua-regia was added and digested by using a microwave reaction system (Anton Paar MDS 3000). After digestion, the contents were allowed to cool to room temperature, and then filtered through Whatman No. 1 filter paper. The filtrate was transferred to a 50-ml pre-calibrated volumetric flask and diluted to volume with deionized water. Samples were further diluted 10× with deionized water and analyzed by GF-AAS (Bettinelli et al. 2000). Reagents without standards or samples were used as blanks.
Determination of heavy metals in parts of tea plants
Glassware and polyethylene containers used for analysis were pre-washed with tap water, then soaked overnight in HNO3 (6 N) and rinsed with deionized water. The analytical procedure described by the Association of Official Analytical Chemists (AOAC 2005) was followed for sample preparation and quantification of heavy metals. About 0.5 g of powdered sample was transferred into a silica crucible and kept in a muffle furnace at 450 °C for 3 h. To the ash sample, 5 ml of 6 M HCl was added and thoroughly mixed. Crucible containing acid-ash mixture was kept on a hot plate and digested (>150 °C for 30 min). Final residue was dissolved in 0.1 M HNO3 solution and diluted to 50 ml.
Method validation
Prior to heavy metal quantification in various matrices, the analytical method was validated. A series of solutions were prepared to check for linearity of the instrument. The instrument’s response was linear for different concentrations of Pb, Cd, Ni, and Cr with a correlation coefficient (r 2). This indicated that this concentration range could be used in the preparation of standard curves during the analysis of heavy metals in the samples of green leaves and soil. Recoveries of heavy metals at different fortification levels were determined in three replicates from each matrix (green leaves and soil) to evaluate the accuracy of the method. The untreated control samples used for fortification studies were pre-analyzed for respective heavy metals.
Results and discussion
Method validation studies
Green tea leaves and soil
The percent relative standard deviation (%RSD) clearly established the repeatability, reproducibility, precision, and accuracy of the method adopted for the analysis (Tables 1 and 2). Recovery was calculated by subtracting heavy metal concentration in these un-treated samples.
Occurrence of metals in tea soils
The heavy metal content of the soils from the tea-growing areas of south India varied significantly. Lead content of the top soil (0–9”) varied from 1.0 to 75 mg/kg, while it was at trace levels in the bottom (9–18”) profile. About 38 % of soils from Anamallais contained 46–60 mg/kg of Pb, and 32 % of the soils had 1.0 to 15 mg/kg. Only 2 % of soil samples from Anamallais contained more than 61 mg/kg Pb. Lead content of the Nilgiri soils ranged between 1.0 and 60 mg/kg; 69 % of soils had Pb concentrations <mg/kg.
Cd content varied from 1.0 to 30 mg/kg in top soils while it was at trace levels in bottom soils. As in the case of Pb, the range of Cd content varied widely from place to place and did not follow a definite pattern of distribution. Several samples collected from Nilgiri contained the low levels of Ni followed by the soils of Anamallais. However, Nilgiri soils collected between 9 and 18” in depth had a higher content of Ni. Cr content in tea soils was low compared to the other heavy metals tested.
Top soils of all tea-growing regions contained almost twice the amount of heavy metals as bottom soils (Table 3). The mean Pb content in top soils was twice that present in the bottom soil. Same amount of Ni was detected in all tea soils, irrespective of region. Mean Cd content of top soil was double that of bottom soil. Among the heavy metals studied, available Cr content was very low. Nilgiri-Wayanad soils had higher Pb content while the Nilgiri soils had very low Pb content. Cd content was observed to be the same in soils of Chikmagalur and the Nilgiris, followed by soils of Wayanad. Gudalur soils contained very low levels of Ni, but it was high in nearby Meppadi. Both Nilgiri-Wayanad and High Range tea-growing soils possessed higher levels of Cr. As indicated earlier, bottom soils of all tea-growing regions had very little heavy metal content compared to top soils.
Main source of Pb contamination in tea soils appears to be agricultural inputs. Usage of nitrogen fertilizers over the past few decades has probably resulted in a shift in soil health. Effect of pH on metal availability in soil and uptake by plants has been well documented (Alloway 1995; Adriano 2001; Jin et al. 2005). McLaughlin et al. (1996) reported that phosphatic and nitrogenous fertilizers were the sources of Pb which is more strongly bound than most other heavy metals on soil surfaces. Lambert et al. (1997) reported the chemical reactions between soil applied with phosphatic fertilizers and formation of lead pyromorphite which is an insoluble complex form of Pb. This has a beneficial effect as Pb cannot be easily spread by water.
Mean concentrations of Cd in subsurface soils of Karnataka were lower which suggested that long-term applications of amendments and mineral fertilizers did not significantly affect Cd content in soils. It should be pointed out that tea cultivation in this area is very much limited and scattered, unlike in the major tea areas of Nilgiri, Anamallais, etc. McLaughlin et al. (1996) reported that raw materials used to produce phosphatic fertilizers may be an important source of Cd.
Ni content in soils mainly depends on its concentration in the rock layer, soil clay content, and fertilizer application. Kabata-Pendias and Pendias (1991) recognized that 100 mg Ni/kg is at an acceptable level in farmland soils. Considering the biological responses of soil to liming, it is prudent to bear in mind that liming may lead to changes other than soil pH. In general, tea soils have both direct and indirect impact on health via food cycle and it is therefore of great importance to preserve the resource and ensure its sustainability besides its health. In the modern agricultural scenario, tea plantations are also resorting to high inputs.
Irrespective of the region, the top soil contained higher amount of heavy metals and eventually attained higher concentrations that are greater than those in lower sections by a factor of 2. Various elemental concentrations along the profiles are probably related to variations in the content of clay/sand or organic matter, which appeared to influence the concentrations of these elements (Vaselli et al. 1997). Apart from the impact of agro inputs added to the tea soils on values of heavy metals, the physico-chemical nature of soils also influences the bio-availability of heavy metals.
Degree of heavy metal accumulation in different parts of tea plants
Experiments showed that nursery-grown tea plants accumulated varying amounts of heavy metals when externally added (Table 4). Tea plants grown under controlled conditions had no heavy metal accumulation, though the soils possessed a meager amount of heavy metals. There was a positive correlation between externally added heavy metals and their accumulation in different plant parts. Irrespective of the sampling day, Pb accumulation in leaves linearly increased in accordance with the amount of externally added Pb fitting a linear regression model (Table 5). A similar trend was observed in the case of stems and roots. Residual amounts of Pb in soil increased linearly with increasing concentration of externally added Pb. Similar trend was noticed for Cd and Ni.
Different parts of field-grown tea plants accumulated varying levels of heavy metals during their growth. From the bud to thick wood, accumulation of Pb linearly increased (Table 6). Feeder roots possessed higher amounts of Pb than mature leaves. Residues of Pb in soil were lower than those in thick wood. Cadmium accumulation in plant parts showed a different trend where from bud to stem, the content of Cd linearly increased but declined in thick wood and again increased in feeder roots. Residues of Cd in soils were higher when compared with that in plant parts. The pattern of Ni accumulation in plant parts was entirely different from that of Pb and Cd. Its accumulation from bud to feeder roots gradually increased. Cd contents in thick wood and soils were comparable, but were significantly different from other plant parts. Results indicated that Cd was relatively less mobile while Pb and Ni moved rapidly in soils. It has been documented that in tea plants mobility of Cd was poor; a major portion of absorbed Cd was fixed in feeder roots, and only a small amount was transported to the aboveground parts. On the basis of Cd concentrations, its distribution per unit dry matter in tea plants grown in uncontaminated soils was in the order of feeder roots > stems > main roots > mature leaves > young leaves. When tea plants were grown in soils supplied with salts, feeder roots possibly acted as a buffer and restricted the transportation of Cd to parts aboveground. Cadmium content of soil has been significantly and negatively correlated with chlorophyll content, photosynthetic rate, transpiration, and ultimately to the biomass production of tea plants (Shi et al. 2008).
Conclusion
A database was developed for Pb, Cd, Ni, and Cr in tea soils of south India; this particular soil survey work will throw more light on heavy metal contamination of tea soils. Tea plants grown under controlled conditions had no significant heavy metal accumulation. However, there was a positive correlation between externally added heavy metals and their accumulation in different plant parts. In addition, different parts of field-grown tea plants accumulated varying levels of heavy metals during their growth.
References
Adriano, D. C. (2001). Trace elements in terrestrial environment (2nd ed.). New York: Springer-Verlag, New York, Inc.
Alloway, B. J. (1995). Heavy metals in soil (2nd ed.). Glasgow: Blackie Academic and Professional.
AOAC. (2005). Official methods of analysis of AOAC international (18th ed.). Rockville, MD: AOAC International.
Bhargava, B. S., & Raghupathi, H. B. (2001). Analysis of plant materials for macro and micronutrients. In H. L. S. Tandon (Ed.), Methods of analysis of soils, plants, waters and fertilizers (pp. 49–82). New Delhi: Fertiliser Association and Consultation Organization.
Bettinelli, M., Baffi, C., Beone, G. M., & Spezia, S. (2000). Soil and sediment analysis by spectroscopic techniques part I: determination of Cd, Co, Cr, Cu, Mn, Ni, Pb and Zn. Atomic Spectroscopy, 21(2), 50–59.
Han, W. Y., Zhao, F. J., Shi, Y. Z., Ma, L. F., & Ruan, J. Y. (2006). Scale and causes of lead contamination in Chinese tea. Environmental Pollution, 139, 125–132.
Jin, C. W., Zheng, S. J., He, Y. F., Zhou, G. D., & Zhou, Z. X. (2005). Lead contamination in tea garden soils and factors affecting its bioavailability. Chemosphere, 59, 1151–1159.
Kabata-Pendias, A., & Pendias, H. (1991). Trace elements in plants. In Trace elements in soils and plants (2nd ed., pp. 67–85). Boca Raton: CRC Press.
Kabata-Pendias, A., & Pendias, H. (1992). Trace elements in soils and plants (2nd ed., p. 365). Boca Raton: Lewis Publishers.
Kabata-Pendias, A. (2000). Trace elements in soils and plants (3rd ed.). Boca Raton: CRC Press.
Lambert, M., Pierzynski, G., Erickson, L., & Schnoor, J. (1997). Remediation of lead, zinc, and cadmium contaminated soils. In R. Hester & R. Harrison (Eds.), Contaminated land and its reclamation (pp. 91–102). Cambridge: the Royal Society of Chemistry.
McLaughlin, M. J., Tiller, K. G., Naidu, R., & Stevens, D. P. (1996). Review: the behaviour and environmental impact of contaminants in fertilizers. Australian Journal of Soil Resarch, 34, 1–54.
Mengel, K., & Kirkby, E. A. (1987). Principles of plant nutrition (4th ed., pp. 520–563). Bern: IPI Pub.
Natesan, S., & Ranganathan, V. (1990). Content of various elements in different parts of the tea plant and in infusions of black tea from southern India. Journal of the Science of Food and Agriculture, 51, 125–139.
Pendias, A. K. (2004). Soil-plant transfer of trace elements—an environmental issue. Geoderma, 122, 143–149.
Perkin-Elmer. (2000). Analytical methods for atomic absorption spectrometry. 0303-0152, E.
Ranganathan, V., & Natesan, S. (1985). Potassium nutrition in tea. In Potassium in Agriculture (pp. 981–1022). Madison: ASA-CSSA-SSSA, Wisconsin.
Seenivasan, S., Manikandan, N., & Muraleedharan, N. (2008). Chromium contamination in black tea and its transfer into tea brew. Food Chemistry, 106, 1066–1069.
Shi, Y. Z., Ruan, J. Y., Ma, L. F., Han, W. Y., & Wang, F. (2008). Accumulation and distribution of arsenic and cadmium by tea plants. Journal of Zhejiang University. Science. B, 9(3), 265–270.
Vaselli, O., Bucccianti, A., & De-Siena, C. (1997). Geochemical characterization of ophiolithic soils in a temperate climate: a multivariate statistical approach. Geoderma, 75, 117–133.
Venkatesan, S. (2007). Notes and amendments to the recommendations on manuring of tea in south India (Section 11. In: Handbook of tea culture). Valparai: UPASI Tea Research Institute.
Verma, D. P., & Palani, N. (1995). Phosphorous nutrition for sustained tea productivity in south India. Bulletin of UPASI Tea Scientific Department, 48, 47–58.
Verma, D. P. (1995). Magnesium nutrition of tea. Newsletter of UPASI Tea Research Institute, 5, 3.
Verma, D. P., & Palani, N. (1997). Manuring of tea in south India (revised recommendations). Valparai: UPASI Tea Research Institute.
Acknowledgements
The authors are grateful to the Tea Board, Government of India, for the financial assistance for this work under the X 5-year plan.
Author information
Authors and Affiliations
Corresponding author
Rights and permissions
About this article
Cite this article
Seenivasan, S., Anderson, T.A. & Muraleedharan, N. Heavy metal content in tea soils and their distribution in different parts of tea plants, Camellia sinensis (L). O. Kuntze. Environ Monit Assess 188, 428 (2016). https://doi.org/10.1007/s10661-016-5440-y
Received:
Accepted:
Published:
DOI: https://doi.org/10.1007/s10661-016-5440-y