Abstract
In agricultural systems, responsive management can mitigate the effects of risk and uncertainty by facilitating adaptation to changing conditions. A tool for evaluating management systems while accounting for risk and uncertainty is Probabilistic Cost Benefit Analysis (PCBA). This study used PCBA to contrast a new responsive disease management strategy against an existing prescriptive strategy. Fungicide application to prevent myrtle rust (MR) in NZ plant nurseries was used as a case study to test if the expected benefits of the responsive strategy justified the investment in potentially more frequent and costlier disease control. A MR risk generator was used to simulate disease progression. Empirical MR risk distribution functions were sampled to stochastically compare net benefits across scenarios, highlighting the potential impact of infrequent but significant disease incursions. Our results showed that the risk-based strategy was more effective at controlling the disease, especially for susceptible myrtle species in high-risk locations. The findings highlighted the essential role of fungicides in propagating highly MR-susceptible species, and that disease management, when responsive to risk, enhanced the efficiency of fungicide use. The Responsive strategy is discussed as an effective management option for nurseries under uncertainty of significant MR incursions. However, in less risky scenarios, the benefits of the responsive strategy were moderate, and operational considerations may favour the standard calendar-based approach. In such cases, the method provided here can help estimate the appropriate fungicide application interval and the associated MR risk.
Similar content being viewed by others
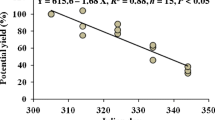
Avoid common mistakes on your manuscript.
Introduction
Agricultural systems by nature are subject to risk and uncertainty, which can negatively impact productivity and profitability (Moschini and Hennessy 2001; Vermeulen et al. 2013). Responsive management strategies mitigate these effects by enhancing the system’s robustness and resilience to fluctuating conditions (Hansen 2002; Ullah et al. 2016; Urruty et al. 2016).
When comparing management strategies, the appropriate analytical framework is important. Cost Benefit Analysis (CBA) is a conventional tool used to assess the economic efficiency of management options. In this context, economic efficiency refers to the optimal use and allocation of resources to maximize the net benefits. CBA achieves this by comparing the benefits of a potential intervention against its costs. Incorporating risk probabilistically allows for a more detailed understanding of potential outcomes. This is particularly important where impacts can vary widely and the likelihood of extreme values are of interest (Knoke et al. 2022; Monge et al. 2023; Rossing et al. 1994).
Probabilistic Cost Benefit Analysis (PCBA) is a valuable tool for evaluating agricultural management strategies in situations involving risk. It allows for the inclusion of variability and the trade-offs between different outcomes, with probabilities taken into account (Ruett et al. 2020). This study applied PCBA to a case study with an agricultural system under highly variable conditions. The focus was on plant nurseries in New Zealand, which have been impacted by a recently arrived fungal plant disease called myrtle rust (MR). Disease management strategies were compared using PCBA under varying levels of disease severity.
MR is a plant disease that has spread worldwide, including into Australasia and continues to spread (Narouei‐Khandan et al. 2020). The MR disease pandemic biotype of Austropuccinia psidii has previously invaded countries such as Australia and New Caledonia (Du Plessis et al. 2019), and since its local detection in 2017 has also affected New Zealand (Beresford et al. 2018; Ho et al. 2019). For New Zealand, this invasive fungus has affected some iconic native and endemic tree species within the Myrtaceae family (McCarthy et al. 2021), notably maire tawake (Syzygium maire; Swamp maire), ramarama (Lophomyrtus bullata), rohutu (L. obcordata) and pohutukawa (Metrosideros excelsa) (Soewarto et al. 2019). It also affects several exotic Myrtaceae species. Disease effects across a range of susceptible species bring economic challenges to local communities and the broader region, including to plant nurseries (Beresford and Wright 2022). MR causes foliage destruction and dieback of young, actively expanding leaf and stem tissues in susceptible species, and wind-borne spores allow it to spread rapidly within nurseries. Nursery managers must aim to exclude it completely from nurseries growing those species using primarily preventative fungicide sprays. Fungicides can control minor incursions, however, once MR has become well-established plant destruction may be the only viable solution (New Zealand Plant Producers Incorporated 2022).
Myrtles represent a key group within the plant species of New Zealand. This group encompasses both native and endemic species that hold significant importance for biodiversity, production, and cultural identity (Ballingall and Pambudi 2017; Beresford and Wright 2022; Velarde et al. 2019). Myrtle species play a pivotal role in new afforestation and forest restoration efforts. These efforts are evident in the expanding forest areas and active planting initiatives (Boffa Miskell Limited 2017; Marden and Lambie 2015; Simpson 1994). These initiatives are threatened by the arrival of MR and are reliant on plant nurseries for their continuation.
The New Zealand nursery industry
New Zealand’s diverse nurseries support both the economy and ongoing environmental restoration. Each nursery is unique, with its own blend of climatic conditions, operational capabilities, and customer demographics. Nurseries selling large quantities of myrtle species are particularly vulnerable to MR, and are the same nurseries contributing endemic and iconic myrtles to ecological afforestation initiatives (Velarde et al. 2019).
Smaller native plant nurseries primarily serve local customers, while medium and larger nurseries have a nationwide customer base (Ministry for Primary Industries 2022). These medium and larger nurseries cultivate diverse plant species for nationwide planting initiatives. Due to their extensive plant supply and potential production of MR-susceptible plants outside their natural range, the value at risk from MR in nurseries extends beyond that specific to their local environments.
Plant nurseries require careful management to ensure the health and vitality of the plants they house, and one of the key challenges they face is the management of diseases (Parke and Grünwald 2012; Valatin et al. 2022). Nursey conditions are characterised by an abundance of young, rapidly growing plants with new flush growth, and potentially favourable moisture conditions, which provide an ideal environment for the rapid spread and establishment of the MR disease (Beresford et al. 2020).
The arrival of a new disease in a nursery carries the potential for significant losses. In Australia, where MR has been present longer than in New Zealand, nurseries have seen increased management costs, increased reliance on regular chemical applications, and removed the more susceptible species from production and sale (Biosecurity New Zealand 2019; Carnegie and Pegg 2018). A similar picture is playing out in New Zealand. The presence of MR in New Zealand has had a considerable impact, as documented by Ballingall and Pambudi (2017), Velarde et al. (2019), and Beresford and Wright (2022).
Case study
This study examines New Zealand plant nurseries as a case study to assess the relative effectiveness of verifiable MR control strategies and their impact on plant production profits. Nurseries are the focus because of their vulnerability to MR, the legal requirement to control the disease, their importance to ecological restoration and afforestation of myrtle species, and the economic and cultural significance of myrtle species to New Zealand.
Plant producers and central government agencies tasked with biosecurity have taken action to mitigate MR threats and manage the disease (Jay et al. 2003; Beresford et al. 2019). Government- requirements preventing the transportation of the disease in addition to expectations for industry best practices place operational constraints on nursery businesses, ultimately impacting nursery business’s profitability (Bate et al. 2016). MR can be present in plants without visible signs (Beresford et al. 2020). Therefore, legal requirements must be verified through specific and verifiable MR control measures for compliance. The cost of those measures varies with the number of plants, their value, and the associated operational costs.
This study builds on previous literature, testing whether responsive management, through intensified monitoring and response, can mitigate disease impact by providing early detection and focused fungicide application (Ruett et al. 2020).
Methods
This research evaluates the economic impacts of MR on plant nurseries by contrasting two disease control strategies. The first is a time-dependent calendar-based approach routinely employed by nursery managers for a range of plant diseases (Beresford and Wright 2022). The second is a responsive risk-based approach as proposed by Beresford and Wright (2020), in which infection risk and disease development rate under changeable weather conditions are measured so that increasing risk can be responded to with increasing fungicide control. We compare the strategies using three exemplar myrtle species differing in MR susceptibility: ramarama (highly susceptible), pohutukawa (moderately susceptible), and manuka (Leptospermum scoparium) (resistant). The comparison is done across multiple locations within New Zealand to test how changing weather affects the predicted MR progression and the financial outcome for plant nurseries growing myrtle species under these two MR control strategies.
The Myrtle Rust Process Model (Beresford et al. 2018) is a nursery decision support tool used for weather-risk-based fungicide management and underpins the risk-based approach by Beresford and Wright (2022). Here the tool was used in a stochastic implementation to provide MR risk sequences for a Monte Carlo PCBA analysis of New Zealand plant nurseries. Net benefits were calculated as the MR impacts avoided by using the Beresford (2022) responsive management approach, offset by any change in fungicide application costs. The framework was designed to best reflect New Zealand plant nurseries using interviews with plant nursery managers to inform the framework structure and assumptions.
Plant nursery interviews
Face-to-face and phone interviews were conducted with relevant commercial and community plant nursery managers who grow and sell myrtle species. Appropriate nurseries were found through the New Zealand Plant Producers Incorporated (NZPPI).
Through the interviews, a targeted email survey was designed, and distributed to a wider group of nurseries across New Zealand. Interviews and surveys were supplemented by advertised plant prices and associated specifications, as well as a previous MR nursery survey (The New Zealand Forest Research Institute 2023). Further information on the interviews and surveys can be found in the supplementary material.
Nursery managers considered MR control a low-cost operation. Fungicide was required for other diseases. Therefore, the arrival of MR as a new fungal pathogen required additional plants to be incorporated into existing disease control operations, generally requiring minimal additional costs.
However, where MR prevention and control failed and a MR incursion happened, nursery costs were significant. Despite incursions being infrequent, 85% of nurseries interviewed had at some point experienced a MR incursion. The severity of those incursions ranged from minor to significant in terms of impact. Incursion impacts came from needing to keep plants longer under disease control, which could include repotting costs, and in the worst cases having to destroy plants. The CBA here has been designed to include these rare but potentially significant impacts and reflect the descriptions of the impacts provided.
Analysis framework
Based on the nursery interviews and surveys a CBA framework was designed to reflect the financial implications of MR and its control. A key assumption was that because the risk-based system was aligned with MR risk, it would effectively manage the disease, making incursions only possible under the calendar-based approach. It was assumed the risk-based approach would avoid all MR impacts. That is, whenever calendar-based fungicide applications fell short of the risk-based applications in a fortnight avoided impacts were calculated, as depicted in the bottom section of Fig. 1. Conversely, when the number of calendar-based fungicide applications met or surpassed the risk-based thresholds, no avoided impacts were assumed.
The year 2022 was selected for simulation as the most recent year with complete data available. Analysis was done on a fortnightly time scale to reflect the temporal resolution of MR progression and to provide sufficient daily MR risk data points within each time step. To define fortnights, months were split into two, one before and including the 15th day and one after.
The study by Beresford and Wright (2022) found that the risk-based strategy enhanced the efficiency of fungicide use, requiring fewer sprays to achieve an equivalent level of disease control. Despite being more responsive, by matching fungicide applications to measured risk the strategy had the potential to reduce disease management costs, a contradiction to the Cost–Benefit Analysis (CBA) expectation of a more thorough management approach requiring higher costs.
Stochastic MR risk influenced the change in the risk-based frequency of fungicide application and the likelihood of incursion under a calendar-based strategy. The process (Fig. 1) compared the costs of fungicide applications with the associated avoided incursion impacts. The comparison is quantified as the net benefits of risk-based fungicide application over the calendar-based approach. Net benefit (\(\widetilde{net\, benefit}\)) was calculated as the avoided impacts (\(\widetilde{avoided\, impact}\)) minus the change in fungicide application costs (\(\widetilde{cost}\)), as shown in Eq. (1), and provided the main result across the scenarios investigated.
The stochastic component of both costs and benefits were driven by the cumulative MR risk, which in turn decided the number of MR risk thresholds exceeded. The threshold value was a key input controlling the rate of fungicide applications required for disease control. It was specific to plant species because of varying disease susceptibility, and to fungicide active ingredients through varying effectiveness. The common reliance on thresholds in calendar-based and risk-based assessments made it more efficient to simulate fungicide strategy costs and benefits simultaneously.
Myrtle rust risk
A stochastic MR risk generator was constructed from data downloaded from the weather risk tool developed for the NZPPI (MetWatch Weather and Disease Platform 2023). The steps were downloading calculated MR risk from 2016 to 2022 for ten regional weather stations (Fig. 2), characterising MR risk for simulation, and simulating fortnightly MR risk, as described in the supplementary material. Characterising risk and simulation were done using the Simetar add-on for Microsoft Excel widely used in the ecological economics risk and uncertainty literature (Monge et al. 2018; Monge and McDonald 2020).
While ten locations were analysed, four were used in the results to explore MR risk in more detail.
-
1.
Kerikeri, located in the northern part of New Zealand near the coast, is characterised by warm temperatures and humidity. These conditions are conducive for MR, making Kerikeri a high-risk area.
-
2.
Havelock North, on the East Coast of the North Island of New Zealand, experiences colder temperatures than Kerikeri. However, it is affected by predominant westerly winds which create a drier climate (Manzello 2020). This climate also presents a high risk for MR in warmer months whenever it is not too dry.
-
3.
Cromwell, situated inland in the lower part of the South Island of New Zealand, experiences greater seasonal temperature fluctuations due to its inland location just east of New Zealand’s Southern Alps. The generally cool temperatures and lower humidity make it a very low-risk location for MR.
-
4.
Gore, in the southernmost region of New Zealand, is on average colder than Cromwell. Despite the cooler temperatures, Gore has a higher risk than Cromwell due to more coastal climate.
These four regions provide a representative overview of the potential risk of MR across a range of climates.
A two-stage stochastic model of daily MR risk was fitted; first simulating risk occurrence, and where there was MR risk, determining its magnitude. As MR risk is influenced by temperature and humidity this approach was based on precipitation modelling due to the common inputs (Benoit et al. 2022; Franzke and Czupryna 2020). This two-stage approach provided a system for representing the fortnightly proportion of zero-value risk days.
For stage 1, simulated risk occurrence was binary, using zero for non-risk days or one for positive MR risk days. Bernoulli distributions were calibrated to the fortnightly probability of positive daily MR risk by location and sampled for stochastic generation.
For stage 2, where risk was positive, risk magnitude was simulated by adding daily MR risk residuals to 2022 fortnightly means. Daily residuals were sampled from fortnightly distributions by location. For locations with sufficient positive MR risk values, the Kolmogorov-Smirnoff test revealed the Beta distribution as the most appropriate distribution. The test worked by comparing the distances between the original data’s Cumulative Distribution Function (CDF) and test CDFs. The test is effective in assessing distribution fit for both parametric and non-parametric distributions (Montefalcone et al. 2021). For low-risk locations, due to the scarcity of positive risk scores, fortnightly residuals were either pooled into monthly groupings to fit a parametric distribution or, in the most extreme cases pooled into 1 group covering the full data set for an empirical distribution (i.e., non-parametric alternative). MR risk modelling is described further in the supplementary material.
The stochastic generator provided annual profiles of cumulative MR risk using a daily time step. The generator was used to simulate multiple MR risk series for Monte Carlo analysis. For each of the ten locations, 500 full-year Monte Carlo simulations of daily MR risk were performed starting on July 1st and ending on June 30th. A July 1 to June 30 year was used, starting and ending in the lowest-risk period (winter) to minimise the effects of initial risk values.
Nursery model
Their method used a threshold value to determine how often fungicides should be applied under increasing MR risk. The threshold value provides an adjustment mechanism for disease control effectiveness, depending on the fungicide used and the plant species. For this study, a nursery fungicide application approach consistent with theirs was assumed, so threshold adjustment provided a method to control for the effect of plant species susceptibility.
Three myrtle species were included in the analysis by adjusting threshold values. Those species covered the range from most susceptible to most resistant. Each species had a specific risk threshold to include in risk and fungicide application requirement calculations for CBA, as shown in the supplementary material. Beresford and Wright (2022) established a risk threshold for ramarama of 0.5, which was used in this study. Their findings provide a robust estimate of susceptibility. Relative susceptibilities across pohutukawa and manuka were based on questions in nursery manager surveys regarding the number of missed fungicide applications that would cause concern. On average nursery managers perceived pohutukawa as half as susceptible as ramarama, and manuka as having 10% of the susceptibility. Consequently, thresholds of 1 and 5 were used for these two species respectively.
Thresholds were used with cumulative simulated MR risk from the stochastic generator to assess the incursion impacts avoided by the risk-based approach. Incursions were assumed for the calendar-based approach in fortnights where cumulative risk exceeded more successive species-specific thresholds than there were fungicide applications.
Two levels of incursion were considered. Small incursion costs for labour covering the time taken for pruning infected shoots were included when MR risk exceeded more thresholds than fungicide applications. Large incursion costs of additional weekly sprays and a no-sale period where the plants could not be sold for a month were included when thresholds exceeded twice the number of fortnightly calendar-based applications. Additionally, where the large-incursion no-sale period pushed the sale outside the planting season, additional plant storage, maintenance, and repotting costs were included for holding plants for the next sale season. Those costs were partially offset by an increase in value due to the larger plant size in the following season. Further detail on incursion assumptions is in the supplementary material. The effects of the number of thresholds required for large incursions as well as the rate of increase in plant value with size is explored further in sensitivity analysis.
PCBA was used assuming a nursery was growing 1000 plants of the specified species, to be sold in 2.5 L pots. Plant size measured by pot volume was used to determine plant value, based on regression of plant specifications and value from the nursery data collected. Further information on the nursery assumptions, including operational costs is included in the supplementary material.
In its simplest form, calendar-based fungicide application involves maintaining a consistent fungicide application interval throughout the year. However, based on the interviews and surveys, nursery managers using a calendar-based system vary their fungicide scheduling with a cessation of applications during the colder winter months, and additional fungicide applications when an incursion is observed. To reflect this, it was assumed that nursery managers in North Island locations do not routinely apply fungicides in July. Further south, in South Island locations, fungicide application ceased in June, July, and August. This assumption is included in Table 1. Also, additional fungicide applications were included following large incursions. These are referred to as reactive fungicide applications. Reactive fungicide applications consisted of four additional applications, once per week for four weeks. Reactive applications were accounted for in incursion triggers, adding them to the fungicide count, and reducing the likelihood of subsequent incursions in the following month. This system represents the practices of most nursery managers following a calendar-based fungicide application strategy.
Scenarios
Scenarios used for analysis with the nursery model were defined by plant species, calendar-based fungicide application intervals and location, with location affecting the assumed weather and associated disease progression. Scenarios are presented in Table 2. A total of 120 unique combinations were used to compare the responsive risk-based fungicide strategy to regular calendar-based scheduled applications.
Calendar-based intervals ranged from 7 to 28 days as well as the reactive-only scenario. The range of fungicide application intervals was selected to cover the range evident in a survey investigating how nurseries in New Zealand protect Myrtle species (The New Zealand Forest Research Institute 2023). The ‘reactive-only’ scenario, with fungicide applications exclusively following large incursions, provided a test for species and location combinations where MR can be managed without chemical sprays. It allowed the identification of location and species combinations suitable for chemical-free MR control where zero large incursions were simulated.
Sensitivity analysis
Two key inputs were identified for sensitivity analysis: (1) The rate of value increase for larger plants when they are kept for an extended period to control MR. (2) The difference between the number of risk thresholds exceeded and fungicide applications to justify a large incursion. Those inputs had importance in the PCBA model as well as uncertainty around their value, requiring sensitivity analysis to test their potential influence on this study’s findings. Their effects on the net benefits were explored with scenarios of ramarama plants grown in high-risk (Kerikeri) and low-risk (Gore) locations.
Results
Stochastic myrtle rust generator
Generated daily risk profiles (Fig. 3) confirmed Kerikeri as the highest-risk location, producing a cumulative risk requiring 32 risk-based fungicide applications in a year for the most susceptible species. In comparison, Cromwell was the lowest risk location requiring on average one fungicide application once every 2.5 years for the same species. The rate of accumulation of the MR risk index for Havelock North and Gore was between those two locations, following a trend of higher risk in warmer northern locations.
Simulated incursions
The shortest calendar-based fungicide application interval tested was 7 days, and this had the strongest disease control effect. Under this interval, for ramarama, most locations had average rates of large incursions between 0.6 and 1 per year. The exceptions included Cromwell and Gore where no large incursions were simulated. No large incursions were simulated at any location under the 7-days interval for pohutukawa or manuka, suggesting that for all but the most susceptible species, regular fungicide application can control MR. For the most susceptible species, however, the efficacy of regularly scheduled fungicide application was unreliable, with some large incursion predicted across locations even for the most conservative treatment.
The average number of large incursions for each of the ten locations across the three plant species groups (Fig. 4) confirmed ramarama as the most MR-susceptible species, as the darker shade indicates a high risk of large incursions. Pohutukawa had a much lower risk, as the shade is much lighter with lighter shades for the warmer Northern regions. Manuka had the lowest risk. No shading for manuka indicates no large incursions across locations and fungicide application frequencies.
Simulated annual average MR large incursion counts across ten regions, defined by aggregated territorial authority boundaries. Counts are presented for three levels of susceptability; ramarama (highly susceptible), pohutukawa (moderately susceptible), and manuka (resistant) plant species, under the 14-days and 28-days preventative intervals, as well as the reactive-only fungicide application strategy
Contrasting across fungicide application intervals (Fig. 4), the lightest shade indicates a low average risk of large incursions for all species except ramarama. The 28-days fungicide application interval is less effective, as the dark areas indicate a high risk for ramarama across North Island locations, and lighter areas indicate moderate risk across most South Island locations. The reactive-only fungicide application is the least effective, as the dark areas indicate a high risk for ramarama across the country, except for the colder dry inland area around Cromwell. For pohutukawa under a reactive-only fungicide application strategy, there is some moderate risk in northern regions and some low-level risk through central North Island areas. This indicates that chemical-free MR control may be appropriate for resistant and moderately susceptible species in most locations across New Zealand, as indicated by no large incursions under the reactive-only strategy.
Cost benefit analysis
Costs
Costs were determined through differences in fungicide application frequency relative to the calendar-based system. Where risk-driven fungicide application increased fungicide frequency there was a cost associated. Conversely, where it reduced fungicide frequency there was a cost saving through more efficient fungicide use.
The risk-based system caused minimal additional costs. The average cost of shifting to a risk-based system in Kerikeri was NZ$300 for 1000 medium-sized ramarama plants, or 2% of the plant value when comparing a 28-days interval. In contrast, for ramarama in Gore and pohutukawa and manuka plants across both locations the risk-based fungicide application reduced fungicide applications, and provided cheaper disease control. Further details on the variation in costs across simulations are provided in the supplementary material.
Benefits
Gross benefits were derived from avoided impacts. That is the incursion expense avoided by matching fungicide applications to measured risk. Benefits were highest for scenarios with warmer more northern locations and for MR susceptible plant species where MR disease progression exceeded the level of disease control provided by the calendar-based system.
The benefits of fungicide application varied with location, plant species susceptibility, and the length of calendar-based intervals. Among the species studied, ramarama, as the most susceptible, showed significantly higher benefits across different locations and application intervals. Specifically, in Kerikeri, the benefits for ramarama compared to a 28-days interval on average were NZ$12,280 per year for 1000 medium-sized plants, representing 94% of the plant value. The 95% quantile range across simulations ranged from NZ$7600 to NZ$13,000. In contrast, other species in Kerikeri and Gore had much lower annual benefits, consistently below NZ$500 across all simulations. Further details on the variation in benefits across simulations and the avoided incursion costs that make up those benefits are provided in the supplementary material.
Net benefits
Net benefits represent the overall advantage of a responsive risk-based fungicide application strategy for managing the risk of MR. They were calculated as the benefits from avoided impacts minus the additional fungicide costs. Net benefits and their associated probabilities are useful for risk-informed decision-making and are a distinctive feature of a PCBA, constituting the main result of this study.
The cumulative probability of net benefits based on 500 simulations for 1000 plants of each species for Kerikeri and Gore shows the likelihoods of net benefits (Fig. 5). As expected, there is a positive correlation across both locations and susceptibility between net benefits and increasing risk of incursion. Ramarama had the greatest net benefits from a risk-based fungicide application strategy across high-risk and low-risk scenarios. The lines for ramarama in the lightest shade are consistently further right in the graphs showing greater proportions of simulations in the high-net-benefits section of the plot.
Cumulative probability distribution of net benefits of changing to risk-based fungicide application schedules from 14-days and 28-days calendar-based application intervals over varying MR susceptibility for ramarama (highly susceptible), pohutukawa (moderately susceptible), and manuka (resistant) plant species. Costs were calculated for a nursery growing 1000 plants. Kerikeri is used as a high-risk location and in contrast Gore as a low-risk location
For most combinations of species, location, and calendar-based fungicide application intervals, net benefits were consistently modest, generally below NZ$380 per annum (Fig. 5). Exceptions were for ramarama across all locations and calendar-based fungicide application intervals, and for pohutukawa specifically in Kerikeri compared to a 28-days fungicide application interval. Which, based on its average impact, was a moderate to low-risk scenario but included a small number of simulations with high impacts.
For those scenarios with moderate to low average risk, high-impact incursions were the outliers. Simulations predominantly provided low-net-benefit results. For the example of pohutukawa in Kerikeri, only 1.5% of simulations predicted high net benefits. However, those few exceeded NZ$10,000 or 45% of plant value, reflecting the potential for rare but high-impact MR incursions. Similarly, for ramarama in Gore under a 14-days fungicide application interval, a small subset of 2.5% of simulations demonstrated much higher net benefits, exceeding NZ$6700 per annum or 52% of plant value. The remaining simulations yielded net benefits below NZ$290. The occurrence of rare but high-impact incursions highlights the advantage of the responsive risk-based fungicide application strategy in providing a mechanism to control and mitigate infrequent but high-impact events.
The cumulative net benefit probabilities have distinct steps due to the binary nature of fungicide application triggers and incursions, and the large associated change in impacts. Where a large incursion was simulated it provided a step change in net benefits through disease management costs as well as potentially postponing seedling sales into when seasonal demand for seedlings ceased. Those simulations incurred additional storage and re-potting costs for keeping seedlings for the following planting season. The steps in net benefits (Fig. 5) reflect a progression from scenarios without large incursions to those with large incursions, and for ramarama in some scenarios a second step where large incursions cause the retention of plants until the subsequent planting season and the associated increase from avoided incursions.
Across the 10 locations, the most MR susceptible species, ramarama, consistently had the greatest net benefits from switching to a risk-based fungicide application strategy (Fig. 6). The spatial variation in average et benefits is shown for the 14-days, and 28-days intervals and the reactive-only fungicide application strategies. The darker shade indicates higher net benefits. Pohutukawa and manuka had a much lower net benefit. Despite pohutukawa having a noticeably higher risk of incursions, the average annual net benefit for growing 1000 pohutukawa plants is not discernible from the benefit for the MR-resistant manuka.
Simulated annual average net benefits across ten regions, defined by aggregated territorial authority boundaries. Net benefits are for 1000 plants over three levels of susceptibility; ramarama (highly susceptible), pohutukawa (moderately susceptible), and manuka (resistant), under 14-days, and 28-days fungicide application intervals, and a reactive-only fungicide application strategy
Contrasting across fungicide application intervals (Fig. 6) net benefits for switching to risk-based fungicide applications from a 14-days fungicide application interval are the lowest, with the lighter shades indicating a comparatively low net benefit. The net benefits for risk-based fungicide applications compared with a 28-days application interval had a higher value, with darker areas showing a high net benefit. Following the trend, the risk-based strategy had the highest net benefit when compared to the reactive-only fungicide application strategy, as the dark shade indicates a high risk of incursion costs for ramarama across New Zealand, except for the colder dry inland area in the South of New Zealand around Cromwell.
Sensitivity analysis
Sensitivity analysis was used across two important but uncertain variables for Kerikeri as a high-risk location, and Gore, as a low-risk location. Those variables are the plant value coefficient, which defines the value of a plant after an incursion based on the change in pot size, and the incursion factor, which defines the number of MR risk thresholds exceeded relative to calendar-based fungicide applications to justify a large incursion. Sensitivity was tested for 1000 ramarama plants across a range of plant value coefficients and incursion factors (Fig. 7).
The plant value coefficient has similar importance as the incursion factor for ramarama. The plant value coefficient decides what plants can be sold for following a large incursion that causes plants to be kept for a long period requiring repotting and an associated increase in plant and pot size, and therefore plant value. The range from 0 to 20 represents a range from no change in plant value to an increase of NZ$20 for every additional litre of pot size. The value used in this study of NZ$7.4/L is equivalent to the average increase empirically evident in plant value data minus 20%, representing an assumed less-receptive market for larger plants later in the planting season. The net benefit results of this study are in the middle of the sensitivity analysis and indicate sensitivity of net benefits to the plant value coefficient is around 35%.
The range in net benefits was wide. For ramarama plants grown in Kerikeri they ranged from NZ$2200 to NZ$12,700 and from NZ$100 to NZ$4600 in Gore. There is a distinct and significant change in net benefits from a risk-based fungicide application schedule due to the incursion factor as it reaches 100%. Because the threshold counts being compared are low-value integers, incursion factors under 100% result in all incursions being classed as large. Once a factor of 100% or more is used, the risk-based cumulative score must pass two or more thresholds to trigger a large incursion. Most fortnights have zero or one calendar-based fungicide application. So, the effects of raising the threshold higher after 100% are minimal. The sensitivity analysis shows that the definition of when a large incursion is triggered makes a large difference with values of either 0% or 100%. However, there are no other meaningful options, and the 100% value used in this study was most suitable.
Discussion
This study examined the net benefits of the responsive risk-based approach compared to calendar-based fungicide application intervals. In low-risk simulations, matching calendar-based intervals to location and species-specific risk was possible, as shown by the low net benefits for those cases. However, while the benefits were small in these cases, the risk-based strategy consistently held value across locations and plant species due to improved fungicide efficiency.
The highest benefits from the responsive risk-based approach were for weather and species combinations carrying the risk of high-impact incursions, even if the potential incursions might be infrequent. The high impacts of those large incursions meant that if only one incursion was avoided, the strategy provided value. This aligns with the experience of the nursery managers. As reported in the surveys, the management of MR is not usually a major burden, requiring marginal additional cost. However, on the rare occasion that MR progresses faster than it can be managed, the costs ramp up quickly. Avoiding those infrequent but significant MR impacts is where a responsive strategy provides value.
The downside of a responsive strategy is primarily operational. The regularity of scheduled fungicide applications makes them more suitable for a nursery business by removing the requirement to monitor weather conditions and allowing a simple regularly scheduled system. Furthermore, calendar-based fungicide applications were shown in simulation to manage the risk of large incursions, provided the frequency is sufficient to exceed the variation in risk resulting from the location’s weather patterns and plant MR susceptibility. The regular calendar-based strategy has the downside of unneeded fungicide applications when the weather is unsuitable for MR. Unneeded fungicide applications place additional fungicides in the nursery environment and carry costs, although, those costs are small.
The findings here are predicated on the assumption that reacting to MR risk and applying fungicide whenever a threshold is surpassed eliminates the possibility of an incursion. This assumption, while necessary for the analysis, is not strictly accurate. There will be at least a small chance of an incursion even with a tailored system such as the proposed risk-based system from Beresford and Wright (2022).
This study utilised two levels of incursion. In reality, each incursion will differ in severity and respond to management controls based on various conditions, including temperature, humidity, and local spore loading. Spore loading, which has not been included in this analysis, can significantly impact the severity of the infection and its control (Beresford and Wright 2022). Where there are more MR spores landing on potential host plants, the pathogen will be more aggressive and harder to control. There is currently insufficient data to quantify this effect. Our research is calibrated to the field trials conducted by Beresford and Wright (2022) for ramarama, and in doing so assumes a uniform spore loading nationally, equivalent to that of the field trial in Beresford and Wright (2022).
In addition to spore loading, this work assumes a linear MR response to increasing disease risk, scaling up with the number of MR risk thresholds surpassed, and scaling down with fungicide applications applied. While reflective of the findings in Beresford and Wright (2022), this approach is approximate. Incursion progression and disease severity will likely follow a logistic function whereby, under conditions that favour MR, it will ramp up much more quickly than our assumption. Conversely, it will likely ramp up more slowly in cold and dry conditions.
This study indicates a risk-based fungicide application strategy is effective. However, managers can include interventions beyond fungicide and removing infected material. The spacing of plants and irrigation and fertiliser management are two interventions that can help control MR within a nursery (Beresford and Wright 2022). While fungicide application is expected to be the most effective option, there are additional options to aid disease management. Although, their effectiveness remains untested (Beresford and Wright 2022).
Weekend-only spraying is appealing for nursery managers, staff, and customers as it minimises exposure to chemical spraying operations. The result of no large incursions for pohutukawa and manuka across the country under 7-days fungicide application cycles suggests weekend-only spraying is a viable option for nurseries, other than for those growing the most susceptible species.
The expected success of the risk-based approach has implications for controlling new incursions. When MR was first discovered in New Zealand in 2017 the initial response was aimed at eradication, with enforced destruction of infected plants (Department of Conservation 2018). This study highlights the potential effectiveness of chemical controls as a lower-impact alternative to plant destruction for incursion responses.
For the reactive-only fungicide application strategy, with no scheduled applications, the only location with no simulated incursions for ramarama was Cromwell. While this suggests the climate there is too cold for MR even on susceptible species, it does not necessarily mean the location is suitable for the economic propagation of those species. All areas where the climate is suitable for propagating ramarama likely require chemical sprays to prevent MR. This finding is particularly relevant for community nurseries, which typically do not use chemical sprays and often rely on volunteer labour. Spraying may not be possible in these settings as many volunteers lack the qualifications for chemical spraying and are usually averse to using chemical sprays. Moreover, volunteer nurseries depend on people’s motivation to complete work, which is often driven by ecological restoration goals. The inability to restore a species due to the emergence of a new disease could significantly impact this motivational drive.
The MR risk simulations presented here represent current weather patterns based on 2022 fortnightly means. It does not account for potential future weather scenarios considering climate change. The effects of those could be assessed for MR disease impacts in future research.
Conclusions
This study used simulation with PCBA to compare two MR control strategies in New Zealand plant nurseries: a responsive risk-based approach from Beresford and Wright (2022), and a regular-interval calendar-based approach. Simulations showed the risk-based approach to be more effective at preventing MR incursions and efficient in fungicide use, providing high net benefit in moderate to high-risk scenarios by avoiding infrequent but high-impact MR incursions.
There were consistently positive net benefits simulated across scenarios. However, in most scenarios, the benefits were small. For those situations, operational factors may favour a calendar-based approach. The regularly scheduled fungicide applications fit more easily into a nursery business, for example, allowing weekend-only fungicide spraying to occur outside regular business hours and away from customers and staff. Ultimately, selecting a fungicide control strategy should align with the individual nursery’s business model, considering the risk associated with local weather conditions and the species grown. The methods presented here can guide the appropriate fungicide application interval for calendar-based systems, matching it to the nursery conditions and the desired level of risk.
A key finding was that propagation of highly MR-susceptible species without frequent enough fungicide application risks serious economic consequences. Furthermore, nurseries that do not use or do not have access to chemical fungicides may be limited in the species they can grow due to the presence of MR.
The findings presented here highlight the benefits of a responsive risk-based MR control strategy and can help inform the design of MR control systems. However, while the assumptions used are considered robust, when considering the implications of this research it is essential to acknowledge the assumptions and listed limitations.
The study demonstrated the value of PCBA as a tool for evaluating responsive management strategies under risk and uncertainty, and the potential of risk-based fungicide application as a robust and efficient option for nursery managers. Extrapolating these findings more widely suggests that agricultural systems dependent on variable factors like weather can benefit from responsive systems. Such systems use measured risk factors to inform management, improving efficiency and are capable of avoiding rare but significant adverse events, minimising the associated impacts.
References
Ballingall J, Pambudi D (2017) Economic impacts of Myrtle rust: A dynamic CGE assessment (p. 19) [NZIER report to Ministry for Primary Industries]
Bate AM, Jones G, Kleczkowski A, MacLeod A, Naylor R, Timmis J, Touza J, White PC (2016) Modelling the impact and control of an infectious disease in a plant nursery with infected plant material inputs. Ecol Model 334:27–43. https://doi.org/10.1016/j.ecolmodel.2016.04.013
Benoit L, Sichoix L, Nugent AD, Lucas MP, Giambelluca TW (2022) Stochastic daily rainfall generation on tropical islands with complex topography. Hydrol Earth Syst Sci 26(8):2113–2129. https://doi.org/10.5194/hess-26-2113-2022
Beresford RM, Turner R, Tait A, Paul V, Macara G, Zhidong DY, Lima L, Martin R (2018) Predicting the climatic risk of myrtle rust during its first year in New Zealand. New Zealand Plant Prot 71:332–347. https://doi.org/10.30843/nzpp.2018.71.176
Beresford RM, Smith G, Ganley B, Campbell R (2019) Impacts of myrtle rust in New Zealand since its arrival in 2017. New Zealand Gard J 22(2):5–10
Beresford RM, Shuey LS, Pegg GS (2020) Symptom development and latent period of Austropuccinia psidii (myrtle rust) in relation to host species, temperature, and ontogenic resistance. Plant Pathol 69(3):484–494. https://doi.org/10.1111/ppa.13145
Beresford RM, Wright PM (2022) Risk-based fungicide management for myrtle rust in nurseries (PFR SPTS No. 22715). Plant Food Res https://mpi.govt.nz/dmsdocument/54247/direct
Beresford RM (2023) Vulnerability of New Zealand Myrtles to Myrtle Rust https://myrtlerust.com/disease/plant/host-vulnerability
Biosecurity New Zealand. (2019). New Zealand Myrtle Rust Strategy 2019–2023. Ministry for Primary Industries https://myrtlerust.org.nz/assets/Uploads/Myrtle-Rust-Strategy-web3.pdf
Boffa Miskell Limited (2017) Mānuka & Kānuka Plantation Guide. Ministry for Primary Industries https://www.mpi.govt.nz/dmsdocument/17371-manuka-kanuka-plantation-guide
Carnegie AJ, Pegg GS (2018) Lessons from the incursion of myrtle rust in Australia. Annu Rev Phytopathol 56:457–478. https://doi.org/10.1146/annurev-phyto-080516-035256
Department of Conservation (2018) New approach to manage myrtle rust https://www.doc.govt.nz/news/media-releases/2018/new-approach-to-manage-myrtle-rust/
Du Plessis E, Granados GM, Barnes I, Ho W, Alexander B, Roux J, McTaggart AR (2019) The pandemic strain of Austropuccinia psidii causes myrtle rust in New Zealand and Singapore. Australas Plant Pathol 48:253–256. https://doi.org/10.1007/s13313-019-0624-x
Franzke CLE, Czupryna M (2020) Probabilistic assessment and projections of US weather and climate risks and economic damages. Climatic Change 158(3–4):503–515. https://doi.org/10.1007/s10584-019-02558-8
Hansen JW (2002) Realizing the potential benefits of climate prediction to agriculture: issues, approaches, challenges. Agric Syst 74(3):309–330. https://doi.org/10.1016/S0308-521X(02)00043-4
Ho W, Baskarathevan J, Griffin R, Quinn B, Alexander B, Havell D, Ward N, Pathan A (2019) First report of myrtle rust caused by Austropuccinia psidii on Metrosideros kermadecensis on Raoul Island and on M excelsa in Kerikeri New Zealand. Plant Dis 103(8):2128
Jay M, Morad M, Bell A (2003) Biosecurity, a policy dilemma for New Zealand. Land Use Policy 20(2):121–129. https://doi.org/10.1016/S0264-8377(03)00008-5
Knoke T, Gosling E, Reith E, Gerique A, Pohle P, Valle Carrión L, Ochoa Moreno WS, Castro LM, Calvas B, Hildebrandt P (2022) Confronting sustainable intensification with uncertainty and extreme values on smallholder tropical farms. Sustain Sci 17(5):1977–1994. https://doi.org/10.1007/s11625-022-01133-y
Manzello SL (2020) Encyclopedia of wildfires and wildland-urban interface (WUI) fires. Springer
Marden M, Lambie S (2015) Plot-based, growth performance of space-planted mānuka (Leptospermum scoparium) on marginal land, and vulnerability to erosion [MPI Technical Paper No: 2015/19]. Ministry for Primary Industries https://www.mpi.govt.nz/dmsdocument/8650/direct
Matsumoto M, Nishimura T (1998) Mersenne twister: a 623-dimensionally equidistributed uniform pseudo-random number generator. ACM Transactions Model Comput Simul (TOMACS) 8(1):3–30. https://doi.org/10.1145/272991.272995
McCarthy JK, Wiser SK, Bellingham PJ, Beresford RM, Campbell RE, Turner R, Richardson SJ (2021) Using spatial models to identify refugia and guide restoration in response to an invasive plant pathogen. J Appl Ecol 58(1):192–201. https://doi.org/10.1111/1365-2664.13756
McKay MD, Beckman RJ, Conover WJ (2000) A comparison of three methods for selecting values of input variables in the analysis of output from a computer code. Technometrics 42(1):55–61. https://doi.org/10.1080/00401706.2000.10485979
Ministry for Primary Industries (MPI) (2022) Native Nursery Survey 2022: Main Report https://www.mpi.govt.nz/dmsdocument/58294-Native-Nursery-Survey-2022-Main-Report
Monge JJ, McDonald GW (2020) The economy-wide value-at-risk from the exposure of natural capital to climate change and extreme natural events: the case of wind damage and forest recreational services in New Zealand. Ecol Econ 176:106747. https://doi.org/10.1016/j.ecolecon.2020.106747
Monge JJ, Daigneault AJ, Dowling LJ, Harrison DR, Awatere S, Ausseil A-G (2018) Implications of future climatic uncertainty on payments for forest ecosystem services: the case of the East Coast of New Zealand. Ecosyst Serv 33:199–212. https://doi.org/10.1016/j.ecoser.2018.04.010
Monge JJ, Dowling LJ, Wegner S, Melia N, Cheon PE, Schou W, McDonald GW, Journeaux P, Wakelin SJ, McDonald N (2023) Probabilistic risk assessment of the economy-wide impacts from a changing wildfire climate on a regional rural landscape. Earth’s Future 11(10):e2022EF003446. https://doi.org/10.1029/2022EF003446
Montefalcone G, Abitbol MH, Kodwani D, Grumitt R (2021) Inpainting CMB maps using partial convolutional neural networks. J Cosmol Astropart Phys 2021(03):055. https://doi.org/10.1088/1475-7516/2021/03/055
Moschini G, Hennessy DA (2001) Uncertainty, risk aversion, and risk management for agricultural producers. Handb Agric Econ 1:87–153. https://doi.org/10.1016/S1574-0072(01)10005-8
Narouei-Khandan H, Worner S, Viljanen S, Van Bruggen A, Jones E (2020) Projecting the suitability of global and local habitats for myrtle rust (Austropuccinia psidii) using model consensus. Plant Pathol 69(1):17–27. https://doi.org/10.1111/ppa.13111
New Zealand Plant Producers Incorporated (2022) Myrtle Rust Garden Retail and Landscape Guidance https://nzppi.co.nz/Pages/SYSTEM/Utility/Download.aspx?id=f824fc18-2f05-468b-ba08-e066f53610ef&newtab=1
NZBHNSC (2016) New Zealand’s Biological Heritage National Science Challenge, Ngā Koiora Tuku Iho Proposal. Accessed https://bioheritage.nz/wp-content/uploads/2019/05/NZBH-NSC-Strategy-Booklet-A5-Screen-Version.pdf
Parke JL, Grünwald NJ (2012) A systems approach for management of pests and pathogens of nursery crops. Plant Dis 96(9):1236–1244. https://doi.org/10.1094/PDIS-11-11-0986-FE
MetWatch Weather Disease Platform (2023) Daily Weather https://nzppi.metwatch.nz/
Rossing W, Daamen R, Jansen M (1994) Uncertainty analysis applied to supervised control of aphids and brown rust in winter wheat. part 1. quantification of uncertainty in cost-benefit calculations. Agric Syst 44(4):419–448
Ruett M, Whitney C, Luedeling E (2020) Model-based evaluation of management options in ornamental plant nurseries. J Clean Prod 271:122653
Simpson PG (1994) Pohutukawa and biodiversity (Conservation Advisory Science Notes 100). Department of Conservation Wellington
Soewarto J, Giblin F, Carnegie A (2019) Austropuccinia psidii (myrtle rust) global host list. Version 2. Australian network for plant conservation, Canberra, ACT
The New Zealand Forest Research Institute (2023) Protecting Myrtaceae from myrtle rust in Aotearoa, New Zealand: Disease impact and control in nurseries. [Unpublished].
Ullah R, Shivakoti GP, Zulfiqar F, Kamran MA (2016) Farm risks and uncertainties: sources, impacts and management. Outlook Agric 45(3):199–205. https://doi.org/10.1177/0030727016665440
Urruty N, Tailliez-Lefebvre D, Huyghe C (2016) Stability, robustness, vulnerability and resilience of agricultural systems. A Rev Agronomy Sustain Dev 36:1–15
Valatin G, Price C, Green S (2022) Reducing disease risks to British forests: an exploration of costs and benefits of nursery best practices. For Int J For Res 95(4):477–491. https://doi.org/10.1093/forestry/cpac003
Velarde SJ, Grant A, Bellingham PJ, Richardson SJ, Wegner S, Soliman T (2019) MPI 18607 Project Report
Vermeulen SJ, Challinor AJ, Thornton PK, Campbell BM, Eriyagama N, Vervoort JM, Kinyangi J, Jarvis A, Läderach P, Ramirez-Villegas J (2013) Addressing uncertainty in adaptation planning for agriculture. Proc Natl Acad Sci 110(21):8357–8362. https://doi.org/10.1073/pnas.1219441110
Acknowledgements
The authors gratefully acknowledge Kathryn Hurr at New Zealand Plant Producers Incorporated for her support and feedback and for connecting the authors with relevant nursery managers. That appreciation extends to those nursery managers for insights into realised and potential MR impacts on their businesses. Further acknowledgement goes to Simon Wegner from Scion for project management, Dr Kwasi Adusei-Fosu, Dr Michael Bartlett, and Dr Julia Soewarto from Scion, along with Dr Beccy Ganley from Plant and Food Research for their expert advice and insights. Lastly, the authors thank the Associate Editor and article reviewers for their constructive feedback, taking the time to explore the details of this work, and enhancing the quality of the article. All contributions to this research have been valuable and are gratefully received.
Funding
This work was funded by the New Zealand Biological Heritage National Science Challenge (NZBHNSC 2016) in a sub-contract (NRT-Risk Assessment QT-10330) within Research Aim 4; ‘Social, cultural and economic characterisation’.
Author information
Authors and Affiliations
Contributions
All authors contributed to the study’s conception and design. Material preparation, data collection and analysis were performed by LD and JM. The first draft of the manuscript was written by LD and reviewed by JM through multiple iterations and RB in the final versions. All authors commented on the final versions and have read and approved the final manuscript.
Corresponding author
Ethics declarations
Conflict of interest
The authors have no relevant financial or non-financial interests to disclose.
Additional information
Publisher's Note
Springer Nature remains neutral with regard to jurisdictional claims in published maps and institutional affiliations.
Supplementary Information
Below is the link to the electronic supplementary material.
Rights and permissions
Springer Nature or its licensor (e.g. a society or other partner) holds exclusive rights to this article under a publishing agreement with the author(s) or other rightsholder(s); author self-archiving of the accepted manuscript version of this article is solely governed by the terms of such publishing agreement and applicable law.
About this article
Cite this article
Dowling, L., Monge, J. & Beresford, R. Probabilistic economic analysis of a weather-based adaptive disease management strategy-the case of myrtle rust in New Zealand nurseries. Biol Invasions 26, 3621–3637 (2024). https://doi.org/10.1007/s10530-024-03398-z
Received:
Accepted:
Published:
Issue Date:
DOI: https://doi.org/10.1007/s10530-024-03398-z