Abstract
Settlement, metamorphosis, survival, and growth of Haliotis asinina larvae exposed to settlement surfaces coated with biofilms of four local diatom species (Navicula seminulum, Navicula parva, Nitzschia alexandria, Amphora coffeaeformis) were assessed. After 24 h and 72 h, N. alexandria induced the highest settlement rate (p < 0.001). Larval settlement rate on slats coated with N. seminulum, and A. coffeaeformis was significantly higher after 72 h than after 24 h (p < 0.001). Metamorphosis was not higher than control after 24 h (p > 0.05) for any diatom treatment, but after 72 h, all diatoms induced a significantly higher metamorphosis rate than the control (p < 0.001). N. alexandria induced the highest metamorphic rate (15.71 %). Shell length of post-larvae was not significantly different on day 4 and day 10 (p > 0.05) in diatom film treatments, relative to control. By day 20, post-larvae survived best on slats with N. alexandria (79.86 %). Survival among treatments was the lowest on slats with N. seminulum (54.91 %), and no live larvae were found in the control. Post-larvae on slats with A. coffeaeformis showed the highest daily growth rate of shell length (DGsl) (87.44 μm d−1). Post-larvae on slats with N alexandria showed the lowest DGsl (55.66 μm d−1). Commercial nursery slats seeded with N. alexandria will result in high and consistent settlement, metamorphosis, and survival, while slats with A. coffeaeformis will ensure sufficient food for rapid growth of the H. asinina post-larvae.
Similar content being viewed by others
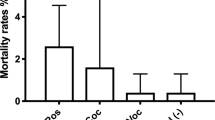
Avoid common mistakes on your manuscript.
Introduction
Abalone is a commercially exploited gastropod in the Asia–Pacific region. There are approximately 90 species of abalone, of which 15 have been cultured commercially (Sales and Janssens 2004) including H. rubra in Australia (Huggett et al. 2005), H. midae in South Africa (Genade et al. 1988), and H. iris in New Zealand (Tong et al. 1987; Moss and Tong 1992). In China, the main cultured abalone species are H. discus hannai Ino in the north and H. diversicolor Reeve in the south (Nie and Wang 2004; Zhang et al. 2004). H. asinina, a tropical abalone species, is the largest abalone species in the South China Sea. In China, the cultured experiment of H. asinine was reported in 2000 and its artificial seeds were successfully obtained in 2005 (Huang et al. 2002, 2007a, b). Currently, H. asinina has been considered as a potential cultured abalone species. A large-scale artificial cultured plan which has been initiated requires large quantities of H. asinina seeds. However, seed production output is restricted by low larval survival rates, abnormal metamorphosis, and poor larval settlement. In order to enhance seed production, it is necessary to improve larval settlement, metamorphosis, and larval survival utilizing various settlement cues in hatchery culture of the tropical abalone H. asinina.
Larval settlement and metamorphosis are influenced by chemical, biological, and physical cues. Chemical cues play an important role in determining the selection of habitat by invertebrate larvae (Hadfield and Paul 2001; Steinberg et al. 2001; Huggett et al. 2005). Chemicals such as gamma-aminobutyric acid and epinephrine can promote settlement and metamorphosis response of H. asinina larvae and increase survival rates after larvae settlement at the appropriate concentration, but low concentrations may be ineffective, and higher doses can cause abnormal development or be toxic, leading to death of post-larvae (Gapasin and Polohan 2004; Wang et al. 2010). In fact, production practice indicates that it is difficult to accurately control the concentration of such chemical substances in the large area waters of induction. Also, the cost of implementing this is severely high. In addition, chemical substances are unsuitable for inducing abalone larval to settle and metamorphose in industry because of environmental and legal concerns (Slattery 1992).
Abalone begin to feed on benthic diatoms after larvae settlement (Tutchulte and Connell 1988). Thus, the role of benthic diatoms as settlement substrates and diets of abalone post-larvae is irreplaceable in seed production process (Chen et al. 1977). Many studies have shown that species, density, and quality of diatoms will also affect larval settlement, metamorphosis, and survival as well as growth of post-larvae (Kawamura and Takami 1995; Kawamura et al. 1995, 1998a, b; Gorrostieta-Hurtado et al. 2009; Carbajal-Miranda et al. 2005; Xing et al. 2008). As inducers, benthic diatoms are safer and more easily controlled compared to other chemical substances. Settlement, metamorphosis, and growth of H. asinina larvae in response to some diatom species have been reported (Gallardo and Buen 2003; Gapasin and Polohan 2005), but the occurrence of larvae in response to local diatom species in the South China Sea is still poorly understood. This paper investigates the response of H. asinina larvae to different local monospecific benthic diatom species which have the potential to greatly increase larval settlement, metamorphosis, and subsequent seed production output.
Materials and methods
Haliotis asinina larvae
H. asinina broodstocks were maintained at an abalone farm in Sanya (18°13′N, 109°28′E), China. Those broodstocks with mature gonads were picked out and induced to spawn by thermal and UV light stimulation. Ovum and sperm were blended together at a ratio of 1:10. This resulted in insemination within 2 h after spawning. Redundant sperm was cleared away by filtered seawater (FSW) about 10 min after insemination. Fertilized eggs were then transferred into aerated fiberglass tanks (500 L) filled with FSW at a density of 30 mL−1 at 30 ± 1 °C and a salinity of 33. When fertilized eggs developed into the swimming larvae, they were siphoned at 15 mL−1 into other aerated fiberglass tanks containing FSW at the same temperature and salinity until subsequent experiments started.
Preparation of slats with diatoms
Four local monospecific inducing benthic diatom species (N. seminulum, N. parva, N. alexandria, A. coffeaeformis) were obtained from a marine biological laboratory at the Hainan University in Haikou, China (19°22′N, 110°102′E). To enable direct observation of the number of settled and metamorphosed larvae as well as attached diatoms under a dissecting microscope, flat transparent polyethylene slats were used. Settlement slats (20 × 20 cm) were placed in flow-through tanks, inoculated with monospecific diatom seeds, and then placed outside for about 1–2 weeks to encourage prior proliferation of the algae. To maintain the growth of diatoms, a compound fertilizer (including NaSiO3) was added to diatom rearing tanks every 4 days. The size and the density of diatom cells (cells number cm−2) were calculated before being used in experiments (Table 1).
Experimental design and methodology
The swimming larvae were counted and placed into fiberglass tanks (80 × 60 × 60 cm) at 3 mL−1 to test their response to four diatom species. Diatom-covered settlement slats were installed vertically in the fiberglass tank at the density of 20 pieces. Every diatom treatment group contained five replicate tanks. Those fiberglass tanks having settlement slats without diatoms were used as controls. In order to assess H.asinina larval settlement and metamorphosis, the number of settled and metamorphosed larvae was counted using a dissecting microscope after 24 and 72 h from the beginning of experiment. Post-larvae with their foot firmly attached to the substrates were regarded as settled and those that had fully shed their velar cilia and undergone peristomal shell secretion were considered metamorphosed (Hahn 1989; Bryan and Qian 1998; Gapasin and Polohan 2004). The formulas to calculate survival rate, settlement rate, and metamorphosis rate are given as:
In this period, a dark environment with light aeration was retained to encourage larvae to settle and metamorphose. From the fourth day, illumination by cool white fluorescent lights with a 12-h L/12-h photoperiod and a flow-through seawater with a flow rate of 5–10 Lh−1 as well as moderate aeration were supplied. The larval shell length (SL) and the post-larval survival rate were first measured on day 4. They were again measured on day 10 and day 20 (see Table 2). Daily growth rate of shell length (DGsl) was used in assessing larval growth and calculated as follows (Capinpin and Corre 1996):
where DGsl was the increase in shell length (μm), and n was the number of days spent rearing.
To better reflect the effects of different diatom species on H. asinina post-larval survival, a formula set forth by Wang et al. (2010) was used to calculate the survival rate and was adjusted to give:
Where LN was the number of settled larvae remaining alive, and TN was the total of larvae settled on slats 72 h after the beginning of experiment.
The water temperature was maintained at 30 ± 1 °C by heating rods, and the salinity was about 33 in all tanks throughout the experiment. Sampling was done by randomly taking five settlement slats of every tank from every diatom treatment group.
Data analysis
Statistical analyses of the data were performed using the SPSS Statistical Package (SPSS Science, Chicago, Illinois). Briefly, the raw data on survival rate, settlement rate, growth rate, or metamorphosis rate were first tested for normality of distribution using the Kolmogorov–Smirnov and Shapiro–Wilk tests and for homogeneity of the variance using the Levene’s test. If raw data failed these tests, square root or natural logarithm transformations were then performed. When both the normality and homogeneity were met, the differences in survival, settlement, and metamorphosis among the groups were analyzed using a one-way analysis of variance (ANOVA) followed by Tukey’s HSD multiple comparisons.
Results
The settlement rate of H. asinina larvae on all slats with diatoms was significantly higher than that of the control after 24 h (p < 0.001) and 72 h (p < 0.001) (Fig. 1). Among four diatom species tested, the highest larval settlement rate of 19.12 % was observed on slates with N. alexandria and was significantly higher than on slates with three other diatom species after 24 h (p < 0.001). The lowest settlement rate (11.52 %) was observed on slats with A. coffeaeformis, but the difference was not significant compared to slats of the two other diatom species (p > 0.05). After 72 h, the settlement rate on slats with diatoms increased and the highest larval settlement rate still occurred on slats with N. alexandria (20.16 %, p < 0.001). However, the lowest settlement rate (11.52 %) occurred on slats with N. parva and was significantly lower than slats with N. seminulum and A. coffeaeformis (p < 0.05). There was no significant difference in the larval settlement rate between slats of N. seminulum and A. coffeaeformis (p > 0.05). Furthermore, the larval settlement rate on slats with N. seminulum, N. parva, and A. coffeaeformis after 72 h was significantly higher than after 24 h (p < 0.001) (Fig. 1), while such significant differences were not observed on slats with N. alexandria and in the control (p > 0.05). The settlement rate of the control remained low and was not different at 24 h (0.81 %) than after 72 h (1.02 %).
Settlement (%) of Haliotis asinina larvae in response to salts with different mono-specific benthic diatoms. Data plotted are means ± SD of five replicates for a period of 24 and 72 h. The different letters on the top of the columns indicate significant differences (1-way ANOVA and Tukey’s HSD test α = 0.05): a < b < c < d < e
After 24 h, larval metamorphosis response was not discovered on any of the slats with diatoms or in the control. After 72 h, the larval metamorphosis rate was the highest on slats with N. alexandria (15.71 %) lowest on slats with A. coffeaeformis (10.54 %) (Fig. 2). Larval metamorphosis rate had no significant difference among slats with N. seminulum, N. parva, and A. coffeaeformis (p > 0.05), but were significantly lower than those on slats with N. alexandria. Although the larval metamorphosis rate on all slats with diatoms was significantly higher than that of the control (only 1.07 %) (p < 0.001). Overall, it was still very low and ranged between 10.54–15.71 %.
Metamorphosis (%) of Haliotis asinina larvae in response to salts with different mono-specific benthic diatoms. Data plotted are means ± SD of five replicates for a period of 24 and 72 h. The different letters on the top of the columns indicate significant differences (1-way ANOVA and Tukey’s HSD test α = 0.05): a < b < c
All of treatment groups displayed a high larval survival rate up to 93.68 % (Fig. 3) and had no significant differences among them (p > 0.05) on day 4. On day 10, the rate slightly decreased on slats with N. parva, N. alexandria, and A. coffeaeformis (p > 0.05) and significantly decreased on slats with N. seminulum and in the control (p < 0.001). Slats with N. alexandria displayed the highest survival rate of 87.44 %, and the control displayed the lowest survival rate of 3.29 %. On day 20, post-larvae survived best on slats with N. alexandria (79.86 %). Survival was the lowest on slats with N. seminulum (54.91 %), and no live larvae were found in the control. The post-larval survival rate on slats with N. seminulum was significantly lower than on slats with three other diatom species (p < 0.001). There was no significant difference in the larval survival rate between slats with N. alexandria and N. parva (p > 0.05). Similar results were observed between slats with N. parva and A. coffeaeformis (p > 0.05).
Survival (%) of Haliotis asinina larvae in response to salts with different mono-specific benthic diatoms. Data plotted are means ± SD of five replicates for Day 4, Day 10 and Day 20. The different letters on the top of the columns indicate significant differences (1-way ANOVA and Tukey’s HSD test α = 0.05): a < b < c < d < e
The shell length of post-larvae for all five treatments was similar on day 4 (p > 0.05) (Table 2). By day 10, the shell length of the post-larvae on slats with diatoms had increased (p < 0.001). Control data were not evaluated due to death of most of the post-larvae. Daily growth rate of shell length (DGsl) of post-larvae was higher on slats with N. seminulum than that slats with three other diatom species, but this difference was not significant (Fig. 4) (p > 0.05). At the end of this experiment, slats with A. coffeaeformis produced the largest post-larvae with an average of 1431.3 μm in shell length (Table 2). Post-larvae on slats with N. alexandria were the smallest with an average of 1103.1 μm in shell length. DGsl of post-larvae on day 20 was higher than that day 10 (p < 0.001). Post-larvae on slats with A. coffeaeformis showed the highest DGsl of 87.44 μm d−1. This was significantly higher than that on slats with other diatom species (p < 0.001). Furthermore, DGsl of post-larvae on slats with N. seminulum was significantly higher than that on slats with N. parva and N. alexandria (p < 0.001), but these significant differences were not detected between slats with N. parva and N. alexandria (p > 0.05) (Fig. 4).
Daily growth rates of shell length (DGsl) (%) of Haliotis asinina larvae in response to salts with different mono-specific benthic diatoms. Data plotted are means ± SD of five replicates for Day 10 and Day 20. The different letters on the top of the columns indicate significant differences (1-way ANOVA and Tukey’s HSD test α = 0.05): a < b < c < d
Discussion
In the absence of a suitable substrate, abalone larvae will postpone settlement and remain swimming for some time (Morse et al. 1984). Undoubtedly, benthic diatoms can enhance settlement of abalone larvae. However, the inducing mechanism is not yet clearly known, and a consensus has not been reached. Many previous studies indicate that the effectiveness of diatoms inducing abalone larvae to settle depends on species specific and/or composition of extracellular mucus which is secreted by diatoms and does not merely depend on simple species combinations (Matthews and Cook 1995; Takami et al. 1997a, b). As confirmation, H. laevigata larvae settled better on films of monospecific diatoms than on films of mixed diatoms, especially films of N. ramosissima alone. H. rubra larvae settled better on films of Amphora sp. alone than on mixed films of Cocconeis sp. and Amphora sp, while they settled worse on films of Amphora sp. alone than on mixed films of Navicula and Amphora Daume et al. (1999, 2000). In addition, Dordon et al. (2004) demonstrated that the chemical acceptor of abalone larvae could receive and identify extracellular mucus released into the water by diatom species and could impel larvae to select different diatom biofilms to settle in.
These results indicated that N. alexandria could quickly induce H. asinina larvae to settle in a short time, and the composition of extracellular mucus which is secreted by this diatom species might be more attractive for H. asinina larvae than other diatom species tested in this experiment. Though other diatom treatments also displayed higher larval settlement rate than the control (p < 0.001), their induced effects were slow and inefficient.
Many previous studies have shown that the larval settlement rate of H. asinina is very low and variable, ranging from 0 to 12 % by using mixed species of diatoms growing naturally on corrugated plastic sheets as settlement substrates (Singhagraiwan and Doi 1993; Jarayabhand and Paphavasit 1996; Fermin and Gapasin 2000). Our research showed a higher larval settlement rate compared to those results. Gallardo and Buen (2003) have also reported that H. asinina larvae settling on sheets of Navicula alone is greater than that on sheets of mixed diatoms. This might imply that H. asinina larvae prefer to settle on slats with monospecific diatoms rather than settle on slats with mixed diatoms.
Normal metamorphosis after larvae settlement determines the success of the seedlings. Likewise, metamorphosis response of abalone larvae to monospecific diatoms depends on the amount and composition of extracellular substances produced by diatom species and/or is species specific. Feeding those diatoms which is not useful for the metamorphosis of some abalone species may be possibly useful for other abalone species. Moreover, diatom species differ in their capacity to secrete extracellular mucus (Takami et al. 1997a, b; Gapasin and Polohan 2005). Our results showed that N. alexandria was the best inducer for H. asinina larval metamorphosis among four diatom species tested, but was not species specific. This was because three other diatom species equally induced a higher metamorphosis rate than the control (p < 0.001). Meanwhile, the capacity of N. alexandria secreting extracellular mucus might be higher compared to that of N. parva, A. coffeaeformis, and N. seminulum. In addition, the capacity of diatom species secreting extracellular mucus was not related to the size of diatom cells, because the A. coffeaeformis cells which were the largest and their density was not the lowest among the four diatom species (Table 1) induced the lowest metamorphosis rate (Fig. 2). Similarly, N. seminulum which has a higher cell density and almost the same size of cell compared to N. alexandria induced a lower metamorphosis rate as well (Table 1).
Kawamura and Takami (1995), Kawamura et al. (1995) and Takami and Kawamura (2003) found that the larvae of H. discus hannai and H. iris on films of different monospecific diatoms or mixed diatoms have a similar survival rate and growth rate within 1 to 2 weeks after larvae settlement. Because abalone post-larvae are incapable of digesting diatoms at this age, they have attributed this to possible benefits of residual yolk and the use of a greater amount of mucus produced by diatoms and that the amount of mucus is more abundant at higher cell densities for the conspecific diatom. With the increasing age of abalone larvae, when nutrients from the yolk are depleted, abalone post-larvae must gain nutrients from the diet to maintain rapid growth (Kawamura et al. 1998b). Therefore, abalone post-larval survival and growth depend on the dietary value, and the amount of benthic diatoms supplied (Gorrostieta-Hurtado and Searcy-Bernal 2004; Gorrostieta-Hurtado et al. 2009).
Results of the current study indicate concur, H. asinia larvae may assimilate nutritional substances from the yolk and mucus secreted by diatoms to meet their growth needs from day 4 to day 10, but the survival and growth were dependant on diatom species tested. Unexpectedly, N. seminulum yielded significantly decreased survival. According to Martinez-Ponce and Searcy-Bernal (1998) and Day et al. (2004), a grazing pressure below the optimum increased the density of diatoms in the biofilm which can result in a low survival rate due to the proliferation of bacteria and protozoa. In contrast, if the density of diatoms is low, post-larval feed intake will be deficient and the survival rate will be lower than at higher densities. Thus, to achieve optimum post-larval development, it is necessary to provide the appropriate amount of diatoms. The low survival rate of H. asinia post-larvae on slats with N. seminulum was thought to be due to its overly high cell densities. We did not find an overproduction of protozoa on slats with N. seminulum.
The dietary value of different benthic diatom species varies due to differences in digestibility and nutritional values (Kawamura and Takami 1995; Kawamura et al. 1995, 1998a). Algae containing a higher proportion of essential fatty acids (EFA: C20:5n–3 and C20:4n–6) are regarded as having a higher nutritional value in the diet of abalone because they must be derived from the diet of consumers (Nelson et al. 2002). In our study, DGsl of post-larvae on slats with A. coffeaeformis was significantly higher than on slats with other diatom species on day 20 (p < 0.001). According to Huang et al. (2007a, b) and Tang (2007), their findings showed that the highest percentage of EFA was discovered in A. coffeaeformis and that percentage was similar in three other diatom species. However, DGsl of post-larvae on slats with N. seminulum were significantly higher than on slats with N. parva and N. alexandria (p < 0.001), and the lowest DGsl was detected on slats with N. alexandria. We speculate that the high DGsl on slats with N. seminulum resulted from a low post-larvae survival rate and higher cell densities. The low DGsl on slats with N. alexandria might be due to its low digestibility. Haliotis fed different species of Nitzschia differed in their digestibility (Kawamura and Takami 1995; Kawamura et al. 1995, 1998a): one species of Nitzschia spp. (8.5 %) and N. ovalis (32.3 %) yielded low digestibility, while another Nitzschia spp. yielded high digestibility (92.7 %). In the current study, the diatom species, N. alexandria, may have been poorly digested. In addition, Kawamura et al. (1998b) reported that H. iris post-larvae that are >600–800 μm SL are able to digest diatoms and therefore grow more rapidly. In this study, we estimated the SL of H. asinia post-larvae that are able to digest diatoms were about 500 μm by analyzing the data of H. asinia post-larval DGsl and SL on day 10 and day 20.
In conclusion, our studies clearly show that N. alexandria induced H. asinia larvae to settle and metamorphose at higher rates than three other local diatom species. N. alexandria can also support a high larval survival rate, but it is unsatisfactory in quickly promoting post-larval growth compared to A. coffeaeformis. Future studies must aim to find a suitable proportion of N. alexandria and A. coffeaeformis to apply to hatchery culture of H. asinina.
References
Bryan PJ, Qian PY (1998) Introduction of larval attachment and metamorphosis in the abalone Haliotis diversicolor (Reeve). J Exp Mar Biol Ecol 223:39–51
Capinpin EC, Corre KG (1996) Growth rate of the Philippine abalone, Haliotis asinina fed an artificial diet and macroalgae. Aquaculture 144:81–89
Carbajal-Miranda MJ, Sanchez-Saavedra MDP, Simental JA (2005) Effect of monospecific and mixed benthic diatom cultures on the growth of red abalone postlarvae Haliotis rufescens (Swainson 1822). J Shellfish Res 24:401–405
Chen SJ, Chen M, Lu HK (1977) On the foods of abalone A preliminary report on the culture of benthic diatoms. Acta Zool Sin 23(1):47–53 (Abstract in English)
Daume S, Brand-Gardner S, Woelkerling WJ (1999) Settlement of abalone larvae (Haliotis laevigata Donovan) in response to non-geniculate coralline red algae (Corallinales, Rhodophyta). J Exp Mar Biol Ecol 234:125–143
Daume S, Krsinich A, Farrell S, Gervis M (2000) Settlement, early growth and survival of Haliotis rubra in response to different algal species. J Appl Phycol 12:479–488
Day R, Gilmour P, Huchette S (2004) Effects of density and food supply on post-larval abalone: behaviour, growth and mortality. J Shellfish Res 23:1009–1018
Dordon N, Shpigel M, Harpaz S (2004) The settlement of abalone (Haliotis discus hannai Ino) larvae on culture layers of different diatoms. J Shellfish Res 23(2):561–568
Fermin AC, Gapasin RSJ (2000) Post-larvae density and photoperiod effects on the settlement and metamorphosis of the donkey-ear abalone, Haliotis asinina (Linne, 1758). In: Proceedings of the 10th tropical marine mollusc programme (TMMP) congress and workshop. Mar Biol Center Spec Publ, pp 231–234
Gallardo WG, Buen SMA (2003) Evaluation of mucus, Navicula, and mixed diatoms as larval settlement inducers for the tropical abalone Haliotis asinine. Aquaculture 221:357–364
Gapasin RSJ, Polohan BB (2004) Induction of larval settlement and metamorphosis in the donkey-ear abalone, Haliotis asinina Linnaeus, by chemical cues. Hydrobiologia 519:9–17
Gapasin RSJ, Polohan BB (2005) Response of the tropic abalone, Haliotis asinina, larvae on combinations of attachment cues. Hydrobiologia 548:301–306
Genade AB, Hirst AL, Smit CJ (1988) Observations on the spawning, development and rearing of the South African abalone Haliotis midae Linn. S A J Mar Sci 6:3–12
Gorrostieta-Hurtado E, Searcy-Bernal R (2004) Combined effect of light condition (constant illumination or darkness) and diatom densities on post-larval survival and growth of the abalone Haliotis rufescens. J Shellfish Res 23:1001–1008
Gorrostieta-Hurtado E, Searcy-Bernal R, Anguiano-Beltran C, Garcia-Esquivel C, Balenzuela-Espinoza E (2009) Effect of darkness on the early post-larval development of Haliotis corrugate abalone fed different diatom densities. Cienci Marina 35(1):113–122
Hadfield MG, Paul VJ (2001) Natural chemical cues for settlement and metamorphosis of marine-invertebrate larvae. In: McClintock JB, Baker JB (eds) Marine chemical ecology. Florida, Boca Raton, pp 431–461
Hahn KO (1989) Handbook of culture of ablalone and other marine gastropods. Florida, Boca Raton, p 348
Huang B, Wang XB, Liu JJ (2002) Studies on feasibility of Haliotis Asinine cultivated in man controlled environment. Mar Sci 26(7):40–41
Huang B, Deng ZR, Wang XB, Zhou Z (2007a) Embryonic development of abalone (Haliotis asinine Linnaeus) in the Chinese seas. Mar Sci 31(4):68–72
Huang HZ, Kang YY, Liang JR, Gao YH (2007b) Analysis of fatty acid composition in five strains of diatoms used as the food of abalone. Plant physiol Commun 43(2):349–354
Huggett MJ, de Nys R, Williamson JE, Heasman M, Steinberg PD (2005) Settlement of larval blacklip abalone, Haliotis rubra, in response to green and red macroalgae. Mar Biol 147:1155–1163
Jarayabhand P, Paphavasit N (1996) A review of the culture of tropical abalone with special reference to Thailand. Aquaculture 140:59–68
Kawamura T, Takami H (1995) Analysis of feeding and growth rate of newly metamorphosed abalone Haliotis discus hannai fed on four species of benthic diatom. Fish Sci 61:357–358
Kawamura T, Saido T, Takami H (1995) Dietary value of benthic diatoms for the growth of post-larval abalone Haliotis discus hannai. J Exp Mar Ecol 194:189–199
Kawamura T, Roberts RD, Nicholson CM (1998a) Factors affecting the food value of diatom strains for post-larval abalone Haliotis iris. Aquaculture 160:81–88
Kawamura T, Roberts RD, Takami H (1998b) A review of the feeding and growth of post-larval abalone. J Shellfish Res 17:615–625
Martinez-Ponce D, Searcy-Bernal R (1998) Grazing rates of red abalone (Haliotis rufescens) post-larvae feeding on the benthic diatom Navicula incerta. J Shellfish Res 17:627–630
Matthews I, Cook PA (1995) Diatom diet of abalone post-larvae (Haliotis mindae) and the effect of pre-grazing the diatom overstorey. Mar Freshw Res 46:545–548
Morse ANC, Froyd CA, Morse DE (1984) Molecules from cyanobacteria and red algae that induce settlement and metamorphosis in the mollusc Haliotis rufescens. Mar Biol 81:293–298
Moss GA, Tong LA (1992) Effect of stage of larval development on the settlement of the abalone, Haliotis iris. N Z J Mar Freshw Res 26:69–73
Nelson MM, Leighton DL, Phleger CF, Nichols PD (2002) Comparison of growth and lipid composition in the green abalone, Haliotis fulens, provided specific macroalgal diets. Comp Biochem Physiol 131(B):695–712
Nie ZQ, Wang SP (2004) The status of abalone culture in China. J Shellfish Res 23:941–945
Sales J, Janssens GPJ (2004) Use of feed ingredients in artificial diets for abalone: a brief update. Nutr Abstr Rev 74(7):13N–21N
Singhagraiwan T, Doi M (1993) Seed production and culture of a tropical abalone, Haliotis asinina Linne. Eastern Marine Fisheries Development Center (EMDEC), Thailand and Japan International Cooperation Agency (JICA), pp 32
Slattery M (1992) Larval settlement and juvenile survival in the red abalone (Haliotis rufescens) an explanation of inductive cues and substrate selection. Aquaculture 102:143–153
Steinberg PD, de Nys R, Kjelleberg S (2001) Chemical mediation of surface colonization. In: McClintock JB, Baker BJ (eds) Marine chemical ecology. Boca Raton, Florida, pp 355–387
Takami H, Kawamura T (2003) Dietary changes in the abalone Haliotis discus hannai, and relationship with the development of the digestive organ. Jarq-Japan Agric Res Quart 37:89–98
Takami H, Kawamura T, Yamashita Y (1997a) Contribution of diatoms as food sources for post-larval abalone Haliotis discus hannai on a crustose coralline algae. Moll Res 18(2):143–152
Takami H, Kawamura T, Yamashita Y (1997b) Survival and growth rates of post-larval abalone Haliotis discus hannai fed conspecific trail mucus and/or benthic diatom Coconeis scutellum var. parva. Aquaculture 152:129–138
Tang N (2007) The research of biochemical compsition of eight benthic diatoms. Dissertation, Dalian university pp 47–52
Tong LJ, Moss GA, Illingworth J (1987) Enhancement of a natural population of the abalone, Haliotis iris, using cultured larvae. Aquaculture 62:67–72
Tutchulte TC, Connell JH (1988) Feeding behaviour and algal food of three species of abalones (Haliotis) in southern California. Mar Ecol Progress Ser 49:57–64
Wang XB, Bai Y, Huang B (2010) Effects of chemical cues on larval survival, settlement and metamorphosis of abalone Haliotis asinina. J Oceanol Limnol 28(6):1261–1265
Xing RL, Wang CH, Cao XB, Chang YQ (2008) Settlement, growth and survival of abalone, Haliotis discus hannai, in response to eight monospecific benthic diatoms. J Appl Phycol 20:47–53
Zhang GF, Que HY, Liu X, Xu HS (2004) Abalone mariculture in China. J Shellfish Res 23:947–950
Acknowledgments
This study was funded by the National Natural Science Foundation of China (No. 31360105), the Key Scientific and Technological Project of Hainan Province (No. 080137), and the Key Project of Hainan University (No. hd09 × m10), China.
Author information
Authors and Affiliations
Corresponding author
Rights and permissions
About this article
Cite this article
Ding, J.j., Huang, B., Hu, Y.q. et al. The effects of different monospecific benthic diatoms on larval settlement, metamorphosis, survival, and growth of Haliotis asinina Linnaeus in the South China Sea. Aquacult Int 25, 367–377 (2017). https://doi.org/10.1007/s10499-016-0035-8
Received:
Accepted:
Published:
Issue Date:
DOI: https://doi.org/10.1007/s10499-016-0035-8