Abstract
Many regions in China experience air pollution episodes because of the rapid urbanization and industrialization over the past decades. Here we analyzed the effect of emission controls implemented during the G-20 2016 Hangzhou summit on air quality. Emission controls included a forced closure of highly polluting industries, and limiting traffic and construction emissions in the cities and surroundings. Particles with aerodynamic diameter lower than 2.5 μm (PM2.5) and ozone (O3) were measured. We also simulated air quality using a forecast system consisting of the two-way coupled Weather Research and Forecast and Community Multi-scale Air Quality (WRF-CMAQ) model. Results show PM2.5 and ozone levels in Hangzhou during the G-20 Summit were considerably lower than previous to the G-20 Summit. The predicted concentrations of ozone were reduced by 25.4%, whereas the predicted concentrations of PM2.5 were reduced by 56%.
Similar content being viewed by others
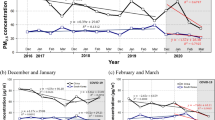
Explore related subjects
Discover the latest articles, news and stories from top researchers in related subjects.Avoid common mistakes on your manuscript.
Introduction
Many areas in China, such as the Beijing–Tianjin–Hebei region, the Pearl River Delta, and the Yangtze River Delta, experience air pollution episodes because of the rapid urbanization and industrialization over the past few decades (Zhao et al. 2013; Rohde and Muller 2015; Yan et al. 2015; Lu et al. 2016; Wang and Fang 2016; Huang et al. 2016; Hong et al. 2016). A number of major international events such as the 2008 Olympic Games, the 2014 Asia-Pacific Economic Cooperation summit (APEC), and the 2015 Grand Military Parade have been held in China, during which efforts were implemented to achieve good air quality (Xing et al. 2011; Li et al. 2014; Wen et al. 2016; Liu et al. 2016). To reduce air pollution during these events, a series of stringent emission control strategies, involving industries and power plants, motor vehicles, and even residential activities were enacted (Liu et al. 2016). Previous studies found that, during the Olympic Games, the APEC summit, and the Grand Military Parade, air quality improvements occurred after implementing a series of restrictive measures to reduce air pollution, which were termed “Olympic Blue,” “APEC Blue,” and “Parade Blue”, respectively (Wang et al. 2011; Huang et al. 2015; Sun et al. 2016). As one of the six largest city clusters in the world, the Yangtze River Delta encompasses the Shanghai municipality, Jiangsu, Anhui, and Zhejiang provinces and accounts for 20% of China’s Gross Domestic Product (http://en.people.cn/200407/08/eng20040708_148830.html). On the basis of long-term monitoring from 1980 to 2011 and 1-year field measurements in 2011–2012, Cheng et al. (2013) reported that visual range in the Yangtze River Delta experienced a sharp decrease from 13.2 to 10.5 km during 1980–2000. To improve the haze pollution in the Yangtze River Delta, it is necessary to decrease PM2.5 (particles with aerodynamic diameter lower than 2.5 μm) concentrations. Here, we evaluate the effectiveness of emission controls on PM2.5 and O3 concentrations in the Yangtze River Delta region.
Hangzhou, located in northwestern Zhejiang province in the south-central portion of the Yangtze River Delta and the capital and most populous city of Zhejiang Province, is one of the most renowned and prosperous cities in China due in part to its natural scenery. By the end of 2015, Hangzhou’s population had reached 9 million with an urbanization of ~75.3% (http://www.zj.stats.gov.cn/tjgb/rkcydcgb/201601/t20160128_168706.html). Due to the rapid urbanization and vigorous economic development over the past three decades, Hangzhou and its surroundings routinely experience air pollution and heavy haze (Sun et al. 2013; Yu et al. 2014a). For example, the mean concentrations of PM2.5 in Hangzhou ranged from 106 to 131 μg/m3 over September 2010 to July 2011 (Sun et al. 2013). The mean concentration of PM2.5 during a week-long heavy haze episode from December 3–9, 2013 was 293.4 ± 103.2 μg/m3 (Yu et al. 2014a). Li et al. (2015) showed that the surface O3 concentrations in the summer of 2013 in Hangzhou were significantly affected by the air pollution transport from the north Zhejiang province (29.6%). As well, the occurrence of heavy haze episodes in Hangzhou was found to be closely associated with the contribution of regional transport of air pollutants (Yu et al. 2014a).
The G-20 2016 Hangzhou summit, the 11th annual meeting of the heads of government, was held during September 3–5, 2016, in Hangzhou, China. During this period, the governments of Hangzhou and its surrounding provinces enforced a series of emission reductions, including a forced closure of highly polluting industries, and limiting traffic and construction emissions in the cities and surroundings. The air quality forecasting system applied here consists of the two-way coupled Weather Research and Forecast and Community Multi-scale Air Quality (WRF–CMAQ) model, which has been used to forecast air quality in Hangzhou regularly (Yu et al. 2015). The objectives of the present study are to combine the WRF–CMAQ model simulations with ground-based observations to evaluate the effectiveness of emission reduction measures during the G-20 2016 Hangzhou summit period and their impacts on air quality on both local and regional scales.
Experimental details
Description of the WRF–CMAQ modeling system
The two-way coupled WRF–CMAQ modeling system (Wong et al. 2012; Yu et al. 2014b) is used to forecast air quality in Hangzhou regularly (Yu et al. 2015). The system was developed by linking the WRF (Skamarock et al. 2008) and the CMAQ models (Wong et al. 2012; Yu et al. 2014b; Eder and Yu 2006). A brief summary relevant to the present study is presented here. The model configurations are the same as those described in Yu et al. (2014b). The modeling domain, as shown in Fig. 1, covers most of China and parts of East Asia with 12 km × 12 km grid resolution. Both WRF and CMAQ use 27 vertical layers. The physics package of the WRF3.4 (ARW) includes the Kain–Fritsch (KF2) cumulus cloud parameterization, the Asymmetric Convective Model (ACM2) for a planetary boundary layer (PBL) scheme, RRTMG shortwave and longwave radiation schemes, two-moment cloud microphysics, and the Pleim–Xiu (PX) land-surface scheme. The meteorological initial and lateral boundary conditions were derived from the Global Forecast System (GFS) model data. The carbon bond chemical mechanism (CB05) (Yarwood et al. 2005) is used to represent photochemical reaction pathways, and the AERO6 aerosol module of the CMAQ version 5.0 is used to represent aerosol microphysics. Predicted aerosol chemical composition includes sulfate, nitrate, ammonium, water, primary organic aerosol, secondary organic aerosol from both anthropogenic and biogenic origin, and elemental carbon (Yu et al. 2014b). The default chemical boundary conditions (BCONs) in the CMAQ model were used in the simulations. Anthropogenic emissions of SO2, NO x , CO, NMVOC, NH3, PM10 and PM2.5 over China and the rest of domain were estimated on the basis of the regional inventories MEIC for 2012 (www.meicmodel.org) and Emissions Database for Global Atmospheric Research (EDGAR): HTAP V2 (0.10 × 0.10), respectively. Biogenic VOC emissions were estimated on the basis of the MEGAN model (Guenther et al. 2012). Model forecast results of the second day are used to compare with the observations.
Concentrations of ozone (O3) and PM2.5 (particles with aerodynamic diameter lower than 2.5 μm) simulated by the WRF–CMAQ (based on the emission controls) with observed data overlaid (circles) at 14:00 (local time) on August 30, September 1 and September 3, 2016. The essential consistency between the model predictions and observations indicates that the spatial patterns of observed PM2.5 and O3 are captured reasonably well
Observations and model evaluation
Observations of hourly air pollutant (PM2.5 and O3) concentrations at 8 national monitoring stations in Hangzhou have been obtained, for which detailed information is available at the Web site of Ministry of Environmental Protection in China (http://datacenter.mep.gov.cn/). These hourly air pollutant data will be used to evaluate the model performance and analyze the effects of emission reductions on air quality in Hangzhou.
Prior to assessing the effects of emission control schemes on air quality, WRF-CMAQ was evaluated against the ground-based observations. In parallel with the observed hourly PM2.5 and O3 observations, concurrent hourly model concentrations at 8 monitoring sites in Hangzhou were averaged. The normalized mean bias (NMB) and correlation coefficient (R) were used to assess model performance based on paired observational and simulated data (Yu et al. 2006; Zhang et al. 2006). NMB reflects the degree of agreement between the simulated and measured values, and R indicates the extent of the relationship between simulated and observational values. Two simulation scenarios were set: one to simulate pollutant concentrations in the absence of emission reductions (denoted as “model”) and another to simulate pollutant concentrations with emission control (denoted as “model-ctr”).
Emission control schemes
For the emission control schemes, the Yangtze River Delta region (including Zhejiang province, Shanghai municipality, Jiangsu province, and Anhui province) was subject to emission controls for the G-20 2016 Hangzhou summit. Table 1 lists the emission sources involved in the reduction measures and their estimated reduction percentages for four different provinces before and during the summit obtained on the basis of internal document (i.e., Collaborative Environmental Air Quality Guarantee Scheme for the Yangtze River Delta Region and Its Surrounding areas during the G-20 2016 Hangzhou Summit, http://futures.hexun.com/2016-06-21/184510358.html). The amount of required emission reductions was dependent upon the distance to the G-20 Summit venue. As shown, industrial and power plant emissions in Shanghai, Jiangsu, and Anhui were reduced by 40% from August 24 to September 6. In the Zhejiang province from August 24 to September 1, in addition to required 50% reduction of the industrial emissions, power plants and residential, motor vehicle emissions were also required to be reduced by 50% for the period of August 28 to September 1, as shown in Table 1. For the G-20 Summit period from September 1 to 6, the reduction percentage required in Zhejiang province for industry, power plants, residential, and motor vehicle emissions increased from 50 to 65%.
Results and discussion
Evaluation of the model performance before, during, and after the G-20 Summit
Figure 1 shows spatial distributions of simulated O3 and PM2.5 overlaid with observed data before and during the G-20 Summit at 14:00 LT on August 30, September 1 and 5, 2016. As shown, there is essential consistency between the model predictions and observations, indicating that the spatial patterns of observed PM2.5 and O3 are captured reasonably well. Figure 2 shows time series of observations and simulations for O3 and PM2.5 in the absence and presence of emission reductions during the period from August 26 to September 15. Model predictions with emission reductions (“model-ctr”) give a much closer agreement with the observations for both PM2.5 and O3 than those without emission reductions. For the entire study period, the correlations (NMB) between predictions and observations are 0.73 (−17.7%) and 0.67 (24.4%) for O3 and PM2.5, respectively, for the simulations with the emission reductions, as compared to values of 0.40 (−28.5%) and 0.37 (59.5%) in the absence of emission reductions. In addition, predictions under the targeted emission controls are much closer to the observations of PM2.5 and O3 than those without the emission controls, as indicated by both time series and scatter plots in Fig. 2.
Time series of observations and simulations with (O3-model-ctr, PM2_5-model-ctr) and without (O3-model, PM2_5-model) emission controls and the corresponding scatter plots between observations and predictions during August 26–September 15, 2016: a time series comparison for O3, b time series comparison for PM2.5, c scatter plots for O3 and d scatter plots for PM2.5. The correlation equations are also shown in the scatter plots. The “model” and “model-ctr” represent the results in the absence and presence of emission reductions, respectively. The “obs” represents observations. The average simulated concentrations of O3 and PM2.5 without emission reductions were significantly higher than the observed values during the G-20 Summit (a, b), indicating significant improvement of air quality
To assess the effects of emission reductions during the G-20 Summit 2016 in Hangzhou, the entire study period was separated into three subperiods: before the G-20 Summit (from August 26 to September 3), namely the start of the implementation of emission reduction; during the G-20 Summit (from September 4 to September 5), during which the more stringent emission reduction strategy was carried out, and after the G-20 Summit (from September 6 to September 15), during which the emission reduction was stopped (Fig. 2). During the G-20 Summit, the average observed O3 concentration was 82.4 μg/m3, as compared to 126.8 μg/m3 before the G-20 Summit but still higher than after the G-20 Summit (75.2 μg/m3) (Fig. 2a). The very low O3 concentrations for the periods of September 9–12 and September 14–15 caused the average low O3 concentration after the G-20 Summit as indicated in Fig. 2a. Figure 2b shows that the average observed PM2.5 concentration during the G-20 Summit (25.9 μg/m3) was somewhat lower than that before the G-20 Summit (33.5 μg/m3) because of more stringent emission reduction strategies during the G-20 Summit, with the highest PM2.5 concentration of 35.8 μg/m3 after the G-20 Summit. The average simulated concentrations of O3 and PM2.5 without emission reductions were significantly higher than the observed values during the G-20 Summit (Figs. 2a, 3b), indicating significant improvement of air quality.
Predicted reductions of hourly O3 (top) and PM2.5 (bottom) concentrations in the Yangtze River Delta region with and without the emission controls for the three periods (i.e., August 31–September 2, September 3–5, and September 6–9, 2016). During the G-20 Summit period, O 3 concentrations were reduced by more than 20 μg/m3 (or 25.4%) in Hangzhou and to a lesser extent in surrounding areas such as Shanghai. PM 2.5 reductions exceeded 20 μg/m3 (or 56.1%) in Hangzhou and to a lesser extent in surrounding Yangtze River Delta region
Impacts of emission control schemes on local air quality in Hangzhou
Figure 3 shows the geographical distributions of predicted reduction of hourly O3 and PM2.5 concentrations in the Yangtze River Delta region during three periods (i.e., August 31–September 2, September 3–5, and September 6–9, 2016) obtained by the difference between the model simulations in the presence and absence of emission controls. During the G-20 Summit period, O 3 concentrations were reduced by more than 20 μg/m3 (or 25.4%) in Hangzhou and to a lesser extent in surrounding areas such as Shanghai (Fig. 3). PM 2.5 reductions exceeded 20 μg/m3 (or 56.1%) in Hangzhou and to a lesser extent in surrounding Yangtze River Delta region, as shown in Table 1 and Fig. 3. Reductions of hourly PM2.5 and O3 in Fig. 2 showed noticeable trends; reduction of hourly levels increased gradually during August 31–September 3, and reaching a maximum during the G-20 Summit.
Conclusion
To prepare for the G-20 Hangzhou summit, held from September 3 to 5, 2016, in Hangzhou, China, governments of Hangzhou and its surrounding provinces (Shanghai, Jiangsu, and Anhui) enforced a series of air pollutant emission reductions. Ground-based observations show that the air quality in Hangzhou during the G-20 2016 Hangzhou summit was considerably improved, most likely due to efficient emission controls across the Yangtze River Delta region. Observations of PM2.5 and O3 at 8 monitoring sites in Hangzhou were used to evaluate simulations from the WRF-CMAQ model and assess the impact of emission controls on air quality in Hangzhou. Simulated results under the targeted emission controls are much closer to the observations of PM2.5 (R = 0.67, NMB = −8.7%) and O3 (R = 0.73, NMB = 4.6%) than those without emission controls. During the G-20 Summit period, O 3 and PM2.5 concentrations were reduced by 20.1 μg/m3 (or 25.4%) and 20.5 μg/m3 (or 56.1%), respectively, in Hangzhou, on the basis of the comparison of the model simulations without and with the emission controls.
References
Cheng Z, Wang S, Jiang J, Fu Q, Chen C, Xue B, Yu J, Fu X, Hao J (2013) Long-term trend of haze pollution and impact of particulate matter in the Yangtze River Delta, China. Environ Pollut 182(6):101–110. doi:10.1016/j.envpol.2013.06.043
Eder B, Yu SC (2006) A performance evaluation of the 2004 release of Models-3 CMAQ. Atmos Environ 40:4811–4824. doi:10.1016/j.atmosenv.2005.08.045
Guenther AB, Jiang X, Heald CL, Sakulyanontvittaya T, Duhl T, Emmons LK, Wang X (2012) The model of emissions of gases and aerosols from nature version 2.1 (MEGAN2.1): an extended and updated framework for modeling biogenic emissions. Geosci Model Dev 5(6):1–58. doi:10.5194/gmd-5-1471-2012
Hong YW, Chen JS, Deng JJ, Tong L, Xu LL, Niu ZC, Yin LQ, Chen YT, Hong ZY (2016) Pattern of atmospheric mercury speciation during episodes of elevated PM2.5 levels in a coastal city in the Yangtze River Delta, China. Environ Pollut 218:259–268. doi:10.1016/j.envpol.2016.06.073
Huang K, Zhang X, Lin Y (2015) The “APEC Blue” phenomenon: regional emission control effects observed from space. Atmos Res 164:65–75. doi:10.1016/j.atmosres.2015.04.018
Huang ZJ, Ou JM, Zheng JY, Yuan ZB, Yin SS, Chen DH, Tan HB (2016) Process contributions to secondary inorganic aerosols during typical pollution episodes over the Pearl River Delta region, China. Aerosol Air Qual Res 16:2129–2144. doi:10.4209/aaqr.2015.12.0668
Li SW, Li HB, Luo J, Li HM, Qian X, Liu MM, Bi J, Cui XY, Ma LQ (2014) Influence of pollution control on lead inhalation bio-accessibility in PM 2.5: a case study of 2014 Youth Olympic Games in Nanjing. Environ Int 94:69–75. doi:10.1016/j.envint.2016.05.010
Li H, Li L, Cheng H, An J, Yan R, Huang H, Wang Y, Lu Q, Wang Q, Lou S, Wang H, Zhou M, Tao S, Qiao L, Chen M (2015) Ozone source apportionment at urban area during a typical photochemical pollution episode in the summer of 2013 in the Yangtze River Delta. Environ Sci (in Chinese) 36(1):1–10. doi:10.13227/j.hjkx.2015.01.001
Liu HR, Liu C, Xie ZQ, Li Y, Huang X, Wang SS, Xu J, Xie PH (2016) A paradox for air pollution controlling in China revealed by “APEC Blue” and “Parade Blue”. Sci Rep-UK 6:34408. doi:10.1038/srep34408
Lu XC, Yao T, Fung JC, Lin CQ (2016) Estimation of health and economic costs of air pollution over the Pearl River Delta region in China. Sci Total Environ 566–567:134–143. doi:10.1016/j.scitotenv.2016.05.060
Rohde RA, Muller RA (2015) Air pollution in China: mapping of concentrations and sources. PLoS ONE. doi:10.1371/journal.pone.0135749
Skamarock WC, Klemp JB, Dudhia J, Gill DO, Barker DM, Duda MG, Huang X-Y, Wang W, Powers JG (2008) A description of the advanced research WRF version 3, Technical Note TN-475+STR, NCAR
Sun GJ, Yao L, Jiao L, Shi Y, Zhang QY, Tao M, Shan G, He Y (2013) Characterizing PM2.5 pollution of a subtropical metropolitan area in China. Atmos Clim Sci 3:100–110. doi:10.4236/acs.2013.31012
Sun Y, Wang ZF, Wild O, Xu WQ, Chen C, Fu PQ, Du W, Zhou LB, Zhang Q, Han TT, Wang QQ, Pan XL, Zheng HT, Li J, Guo XF, Liu JG, Worsnop DR (2016) “APEC Blue”: secondary aerosol reductions from emission controls in Beijing. Sci Rep 6:20668. doi:10.1038/srep20668
Wang ZB, Fang CL (2016) Spatial-temporal characteristics and determinants of PM2.5 in the Bohai Rim Urban Agglomeration. Chemosphere 148:148–162. doi:10.1016/j.chemosphere.2015.12.118
Wang W, Jariyasopit N, Schrlau J, Jia Y, Tao S, Yu TW, Dashwood RH, Zhang W, Wang X, Simonich SL (2011) Concentration and photochemistry of PAHs, NPAHs, and OPAHs and toxicity of PM2.5 during the Beijing Olympic Games. Environ Sci Technol 45:6887–6895. doi:10.1021/es201443z
Wen W, Cheng SY, Chen XF, Wang G, Li S, Wang XQ, Liu XY (2016) Impact of emission control on PM2. 5 and the chemical composition change in Beijing-Tianjin-Hebei during the APEC summit 2014. Environ Sci Pollut R 23:4509–4521. doi:10.1007/s11356-015-5379-5
Wong DC, Pleim J, Mathur R, Binkowski F, Otte T, Gilliam R, Pouliot G, Xiu A, Young JO, Kang D (2012) WRF-CMAQ two-way coupled system with aerosol feedback: software development and preliminary results. Geosci Model Dev 5:299–312. doi:10.5194/gmd-5-299-2012
Xing J, Zhang Y, Wang SX, Liu XH, Cheng SH, Zhang Q, Chen YS, Streets DG, Jang C, Hao JM, Wang WX (2011) Modeling study on the air quality impacts from emission reductions and atypical meteorological conditions during the 2008 Beijing Olympics. Atmos Environ 45:1786–1798. doi:10.1016/j.atmosenv.2011.01.025
Yan RC, Yu SC, Zhang QY, Li PF, Wang S, Chen BX, Liu WP (2015) A heavy haze episode in Beijing in February of 2014: characteristics, origins and implications. Atmos Pollut Res 6:867–876. doi:10.5094/apr.2015.096
Yarwood G, Rao S, Yocke M, Whitten GZ (2005) Final report-updates to the carbon bond chemical mechanism: CB05,Rep. RT-04-00675, 246 pp., Yocke and Co., Novato, California. Available at: http://www.camx.com/publ/pdfs/CB05_Final_Report_120805.pdf
Yu SC, Eder B, Dennis R, Chu SH (2006) New unbiased symmetric metrics for evaluation of air quality models. Atmos Sci Lett 7:26–34. doi:10.1002/asl.125
Yu SC, Zhang QY, Yan RC, Wang S, Li PF, Chen BX, Liu WP, Zhang XY (2014a) Origin of air pollution during a weekly heavy haze episode in Hangzhou, China. Environ Chem Lett 12:543–550. doi:10.1007/s10311-014-0483-1
Yu SC, Mathur R, Pleim J, Wong D, Gilliam R, Alapaty K, Zhao C, Liu X (2014b) Aerosol indirect effect on the grid-scale clouds in the two-way coupled WRF-CMAQ: model description, development, evaluation and regional analysis. Atmos Chem Phys 14(20):11247–11285. doi:10.5194/acp-14-11247-2014
Yu SC, Li PF, Yan RC, Wang S, Liu WP (2015) Air quality real-time forecast of PM2.5 in Hangzhou metropolitan city with the WRF-CMAQ and WRF/Chem systems: model development and evaluation. In: 14th Annual CMAS conference, October 3–7, Chapel Hill, NC, USA
Zhang Y, Liu P, Pun B, Seigneur C (2006) A comprehensive performance evaluation of MM5-CMAQ for summer 1999 Southern Oxidants Study Episode, Part I. Evaluation protocols, databases, and meteorological predictions. Atmos Environ 40:4825–4838. doi:10.1016/j.atmosenv.2005.12.043
Zhao PS, Dong F, He D, Zhao XJ (2013) Characteristics of concentrations and chemical compositions for PM2.5 in the region of Beijing, Tianjin, and Hebei, China. Atmos Chem Phys 13:4631–4644. doi:10.5194/acp-13-4631-2013
Acknowledgements
This work was partially supported by the Department of Science and Technology of China (No. 2016YFC0202702, No. 2014BAC22B06) and National Natural Science Foundation of China (No. 21577126). This work was also supported by the Joint NSFC–ISF Research Program (No. 41561144004), jointly funded by the National Natural Science Foundation of China and the Israel Science Foundation. Part of this work was also supported by the “Zhejiang 1000 Talent Plan” and Research Center for Air Pollution and Health in Zhejiang University. YZ acknowledges the support from DOE (DE-SC0006695) and NSF (AGS-1049200) at NC State, USA. The views expressed in this presentation are those of the author(s) and do not necessarily represent those of the US EPA.
Author information
Authors and Affiliations
Corresponding author
Rights and permissions
About this article
Cite this article
Li, P., Wang, L., Guo, P. et al. High reduction of ozone and particulate matter during the 2016 G-20 summit in Hangzhou by forced emission controls of industry and traffic. Environ Chem Lett 15, 709–715 (2017). https://doi.org/10.1007/s10311-017-0642-2
Received:
Accepted:
Published:
Issue Date:
DOI: https://doi.org/10.1007/s10311-017-0642-2