Abstract
Pheromones are considered to play an important role in broadcast spawning in aquatic animals, facilitating synchronous release of gametes. In oysters, the sperm has been implicated as a carrier for the spawn-inducing pheromone (SIP). In hatchery conditions, male pearl oysters (Pinctata maxima) can be stimulated to spawn through a variety of approaches (e.g. rapid temperature change), while females can only be induced to spawn through exposure to conspecific sperm, thus limiting development of targeted pairing, required for genetic research and management. The capacity for commercial production and improvement of genetic lines of pearl oysters could be greatly improved with access to a SIP. In this study, we prepared and sequenced crude and semi-purified P. maxima sperm extracts that were used in bioassays to localise the female SIP. We report that the P. maxima SIP is proteinaceous and extrinsically associated with the sperm membrane. Bioactivity from pooled RP-HPLC fractions, but not individual fractions, suggests that the SIP is multi-component. We conclude that crude sperm preparations, as described in this study, can be used as a sperm-free inducer of female P. maxima spawning, which enables for a more efficient approach to genetic breeding.
Similar content being viewed by others
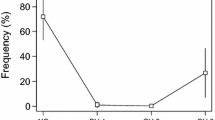
Avoid common mistakes on your manuscript.
Introduction
The silver-lipped pearl oyster (Pinctada maxima) produces high-quality large pearls. In Australia, wild oysters have traditionally been used for pearl production. Mantle tissue from a donor oyster, grafted with a nucleus, secretes a nacreous layer surrounding the implanted nucleus, ultimately producing a pearl within 2–3 years (Mamangkey and Southgate 2009). Increased production requirements have led to the development of technologies to produce hatchery-raised oysters to augment the wild stock for pearl cultivation.
At present, male P. maxima can be induced to spawn without exposure to conspecific sperm, by manipulating their water temperature, feed and water levels. The additional exposure to sperm will almost always hasten this response (D. Mills, Paspaley Pearls, 2011 pers. comm.). These sperm-free spawn induction techniques have never proven successful on sexually mature female P. maxima, and addition of conspecific sperm is required to induce spawning, although pre-spawning behaviours such as wide gaping and opening and shutting of valves can be observed prior to sperm application. The addition of conspecific sperm is the only known trigger that will induce spawning in female P. maxima.
Upon spawning, eggs are fertilised rapidly, limiting commercial and research development for two reasons. First, in commercial breeding operations, males and females are mass spawned in a single tank. When a single first male spawns, the presence of conspecific sperm triggers other males and females to spawn, resulting in a mixture of gametes from different broodstock. While this procedure is designed to create maximum genetic diversity within a batch, it creates bias towards fertilisation with the sperm from the initial spawning male, thus significantly reducing intended diversity (D. Mills, Paspaley Pearls, 2011 pers. comm.).
A second limitation to genetic improvement of hatchery-raised oysters is the inability to access spawned unfertilised P. maxima eggs. Gametes can be stripped from oysters and activated using ammonia, but this usually means killing the broodstock, and gametes are typically of lower quality than those naturally spawned (Rose 1990; Tanaka and Kumeta 1981). P. maxima sperm can currently be used to fertilise multiple females, thereby allowing for comparing genetic traits and gamete quality amongst the F1 offspring. However, the reverse situation is limited by the rapid fertilisation of eggs upon spawning (as they will only be released upon exposure to conspecific sperm). This process could be greatly simplified if naturally released eggs were available unfertilised, without the need to sacrifice the females to achieve this (Minaur 1969; Rose 1990).
Aquatic spawn-inducing pheromones (SIPs) have been implicated for many species, acting to synchronise gamete release, and the exact biochemical composition has been elucidated for some. Most is known from fish, where spawning pheromones may consist of a variety of steroids, prostaglandins, bile salts and amino acids (for review, see Chung-Davidson et al. 2010). Galtsoff (1938) first reported the presence of a SIP in oysters, describing the stimulation of spawning in the oyster Crassostrea virginica within seconds to 1 h after conspecific sperm application. Also in C. virginica, it was established that the SIP was proteinaceous and associated with the sperm membrane (Rice et al. 2002); procedures that can denature proteins such as multiple freeze-thawing, heat treatment and proteases resulted in a reduction of conspecific spawning activity by 68% upon conspecific sperm treatment.
In this study, we investigated the presence of a SIP in P. maxima. Our observations that P. maxima conspecific sperm can trigger spawning led to targeted analysis of the sperm and seminal fluid (milt). Using extraction and behavioural spawning assays, we report a SIP that is associated with the sperm membrane and that it remains bioactive even after lyophilisation and multiple freeze-thaw cycles.
Materials and Methods
Experimental Oysters
P. maxima were obtained from a pearl farm located in the north-eastern Kimberley’s of Western Australia. Wild, sexually mature oysters in ripe condition between 150 and 200 mm in shell height were collected. During peak spawning seasons for P. maxima (January to April and September to November), wild-caught oysters were chosen to provide sufficient genetic variation to eliminate potential bias in response to the bioassay that may have occurred from using a hatchery-derived cohort.
Panels of oysters were removed from the water; the individual oysters removed and opened using speculums. The oysters were visually assessed for suitable gonad maturity according to the scale originally described by Tranter (1958). Oysters with well-developed gonads were retained, cleaned to remove external fouling, placed back into tagged panels and returned to the water prior to transport.
Oyster Transport and Maintenance
Collected oysters were packed between layers of damp hessian in insulated containers and transported by seaplane to the hatchery and immediately transferred into seawater, approximately 4 h after packing. Female oysters were placed together in two 780-l tanks of ambient seawater (28 °C) and fed a mixture of live microalgae. Males were separated into individual buckets containing cooled seawater at 23–25 °C and not fed. Males will commonly spawn following a stressful event (e.g. transport) and particularly when placed in warm water and fed. In addition, a male spawning in the presence of another will often trigger the other males to spawn. As stripped sperm was required for the bioassays, males were placed into separate tanks to minimise the risk of mass spawning.
Stripping of Sperm and Preparation of Crude Sperm and Milt
To collect stripped sperm, male oysters were sacrificed by slicing a knife between the shell and the adductor muscle and then viscera, containing gonad cells, was removed. Scalpel incisions were made across the gonad and fluid was drained into a sterile container. Sperm from multiple males was mixed and stored at 4 °C prior to use. Approximately 20 ml of gonad fluid was harvested from each male.
-
(a)
Whole live stripped sperm: P. maxima sperm was stripped from males for bioassay on females, and then compared with females treated with no sperm (negative controls). Treatments administered with 0.5-ml live, stripped sperm were considered a positive control in all remaining bioassays. Fresh oysters were sacrificed and stripped on the day of each bioassay to ensure that sperm was at the same level of activity. Three to six males were stripped and the sperm pooled to reduce variability between bioassays regarding sperm quality. Naturally released sperm was not used in any bioassay.
-
(b)
Sperm and sperm milt: Seminal fluid was collected and centrifuged at 4000×g for 30 min at 4 °C to provide sperm-free milt (Agelopoulou et al. 2004; Jonáková et al. 2007; Rice et al. 2002). The seminal fluid was carefully separated from the sperm pellet by gentle pipetting into another vial kept on ice. A sample of concentrated sperm (sperm pellet) was examined under a light microscope (Leica compound microscope) to ensure that sperm cells had not ruptured. Sperm pellets and sperm milt were frozen separately at − 80 °C prior to transport to the laboratory on dry ice for processing.
Preparation of Experimental Treatments: Semi-Purified (SP) 1–8
Treatments were prepared using stripped P. maxima sperm as follows, Fig. 1.
-
SP1-Sperm milt after semi-purification: Sperm milt was thawed, acidified to 0.1% triflouroacetic acid (TFA; Merck) and processed through a Sep-20 cm3 (5 g) C18 column cartridges (Waters Corp) according to the manufacturer, to remove hydrophobic molecules. Water-soluble molecules were eluted with 60% CH3CN/0.1%TFA into a sterile tube, then snap frozen in dry ice and lyophilised (freeze-dried) prior to use in spawning bioassay. Treatments were administered at equivalent to 0.5 ml of stripped sperm.
-
SP2-Isolation of sperm membrane-associated molecules, method 1 for semi-purification, similar to extraction protocols used on C. virginica sperm, after Rice et al. (2002). Mechanical homogenisation was used to extract molecules associated with the sperm cell. Sperm pellets were thawed and refrozen three times with dry ice to rupture sperm cells. Pellets were then resuspended in 0.1% TFA, homogenised (Qiagen tissue disruptor; Qiagen) for 10 min and sonicated for 5 min at the highest setting. Samples were then centrifuged for 30 min at 4000×g to produce sperm membrane pellets and supernatant. Sperm membrane pellets were refrozen and lyophilised for use in treatments SP3 and SP4. Supernatant was semi-purified through a Sep-Pak and eluates were lyophilised. Approximately half was stored at − 80 °C for use in bioassays, and the other half was further purified using reverse phase high-performance liquid chromatography (RP-HPLC (SP5)). Treatments were administered at equivalent to 0.63 ml of stripped sperm per dose.
-
SP3-Sperm pellet membranes: Sperm membranes were retained following SP2 purification. Bioassay of lyophilised membranes was performed to assess the effectiveness of protocol used for SP2 (method 1) to remove SIP from the sperm membrane. Treatments were administered at equivalent to 1.116 ml of stripped sperm per dose.
-
SP4-Sperm pellet membranes treated with proteinase K: Remaining sperm membranes from SP3 were treated with proteinase K (500 μg/ml; Sigma) at 40 °C for 24 h, to remove sperm proteins. Treated samples were then boiled for 10 min to inactivate the proteinase K activity. The sample was then frozen at − 20 °C until bioassay. Treatments were administered at equivalent to 1.116 ml of stripped sperm per dose.
-
SP5-RP-HPLC-purified fractions of sperm membrane-associated molecules extracted by method 1: lyophilised semi-purified samples obtained following SP2 (method 1) were further purified by C18 RP-HPLC, a technique for separating smaller biomolecules (< 2000 Da) into fractions based on their hydrophobicity (Cummins et al. 2011). Samples were resuspended in 0.1% TFA and purified by semi-preparative Zorbax C18 RP-HPLC (1 × 25-cm column) using a two-step linear gradient (0–10% CH3CN/0.1% TFA for 5 min, then 10–60% CH3CN/0.1% TFA for 50 min, with a flow rate of 2 ml/min. Fractions were manually collected into 50-ml falcon tubes, at intervals of 5 min, so that the samples were split into 10 fractions. Fractions were lyophilised and frozen at − 20 °C until used in bioassay as pooled groups: 10–30, 30–50, 30–40 and 40–50 min.
-
SP6-Isolation of sperm membrane-associated molecules, method 2: extraction under high pH was used in an effort to increase the yield of proteins from the sperm membrane, after Rice et al. (2002). Sperm pellets (6 × 12.67 g) were partially thawed and resuspended in 25 ml of 1 M sodium carbonate (Na2CO3) solution at pH 12.65. Samples were then homogenised (Qiagen tissue disruptor) for 5 min on ice. They were then placed on a rocker at 4 °C for 30 min, then placed in a sonicator bath for 10 min at the highest level at room temperature, prior to further homogenisation for 5 min. Samples were then centrifuged for 30 min at 4000×g at 4 °C. Supernatant was removed for semi-purification using Sep-Pak C18 cartridges. Molecules were eluted with 60% CH3CN and lyophilised. Approximately half was stored at − 80 °C for use in bioassays and the other half was further purified using RP-HPLC (SP7). Treatments were administered at equivalent to 1 ml of stripped sperm per dose.
-
SP7-RP-HPLC-purified fractions of sperm membrane-associated molecules extracted by method 2: lyophilised membrane-associated molecules following SP6 (method 2) were resuspended in 0.1% TFA, then fractionated by RP-HPLC. RP-HPLC conditions were 0–10% CH3CN/0.1% TFA over 5 min, then 10–60% over 50 min. Fractions were collected in 1 min intervals and lyophilised for use in bioassay as pooled groups: 30–36, 37–43 and 44–51 min. Groups 37–43 and 44–51 min were also repeated in bioassay at double concentration.
-
SP8-Sperm pellet membranes: Sperm membranes were retained following SP6 purification. Bioassay of lyophilised membranes was performed to assess the effectiveness of protocol used for SP6 (method 2) to remove SIP from the sperm membrane.
Experimental System and Spawning Bioassays
Tanks (3 × 1000 l) were filled with seawater and heated to 32 °C the day prior to bioassay to allow thermal manipulation of oysters. Microalgae for feeding oysters were grown in a temperature-controlled algae lab at the DAC. An 80-l tub was set up in the experimental room with an airline and stone to maintain the microalgae in suspension on the day of each bioassay. There was a capacity to use forty 18-l experimental tubs for each bioassay; the number used varied between bioassays depending on oyster availability.
All spawning bioassays were conducted using identical spawn induction techniques across all treatments over a 1-day period. Variables included the addition of experimental treatments to the tubs and the number of oysters used in each treatment group.
Females were randomly allocated to 18-l individual tubs containing seawater at 28 °C and fed microalgae. After 1 h, animals were given a water exchange by draining the water from the tubs and refilling with seawater at 32 °C. Tanks were slowly refilled to ensure minimal stress. The process of draining and refilling all 40 experimental tubs took approximately 1 h. Animals were then fed again and treatments were administered. All treatments were administered by 1-ml syringe, within close proximity to the entrance to the oyster’s inhalant siphon.
The standard spawning bioassay requires a waiting period of 1 h after each water exchange and addition of treatment, allowing sufficient time for females to react. After 1 h, females that had not spawned had their tank drained, then half filled with seawater at 32 °C to ensure that oysters were only partially submerged and left for another 10 min. Tubs were then completely refilled and animals fed microalgae. Females that had spawned were marked and no seawater was exchanged. After 1 h, the treatment procedure was repeated for females that had not spawned. If females had not spawned by 1 h of this third treatment, they were considered to be non-responsive to treatments, or the treatment was not functional, and the bioassay was terminated. Time taken to spawn, after initial addition of treatment, was recorded for each spawning female.
Lyophilised treatments were resuspended in filtered seawater to a concentration equivalent to 0.5-ml whole stripped sperm.
Treatments were tested over a series of bioassays, meaning several batches of oysters were used over the duration of the project. As receptivity to spawning triggers showed variation between different batches of oysters, a relative treatment spawn % was calculated to show comparison between treatment spawn rates with their corresponding controls.
Sperm Membrane Pellet Preparation and Protein Analysis
Seawater containing P. maxima sperm was collected from 10 individuals and stored in 250-ml tubes at − 80 °C, prior to further analysis. Samples were removed from − 80 °C, thawed at room temperature, then centrifuged for 30 min at 4000×g at 4 °C. The supernatant was discarded, and pellets were processed by freeze-thaw three times. Pellets were then resuspended in 1-ml 0.1% TFA then centrifuged for 30 min at 4000×g at 4 °C. The supernatant was discarded, and pellets were sonicated on ice (4 × 30-s bursts at the highest level). Then, the sample was centrifuged for 30 min at 16,000×g at 4 °C, the supernatant removed and membrane pellet lyophilised.
Lyophilised membrane pellets were gently resuspended and washed three times with wash buffer (20 mM Tris–HCl, 150 mM NaCl, pH 7.6) and then incubated in 2-ml buffer (20 mM Tris–HCl, 150 mM NaCl, 10 mM CaCl2, 1 M D-arabinose (Sigma-Aldrich, St. Louis, MO), pH 7.6) for trypsin shaving (Solis et al. 2010; Taverna et al. 2007). The sample was then digested with 10-μg sequencing grade trypsin (Promega, Madison, WI) for 20 min at 37 °C with gentle rocking. Biological duplicates were performed. The digestion was stopped by adjusting the pH of the sample to < 3 by adding 10% formic acid. Membrane residues were removed by centrifugation at 1500×g for 15 min at 4 °C. Supernatant was then collected and further centrifuged at 12,000×g for 15 min 4 °C, and the resulted supernatant was lyophilised using a SpeedVac vacuum concentrator and desalted using ZipTip C18 (Millipore, Bedford MA) prior to LC-MS/MS analysis.
The tryptic peptides were analysed by LC-MS/MS on a Shimadzu Prominance Nano HPLC (Kyoto, Japan) coupled to a Triple Tof 5600 mass spectrometer (AB SCIEX, Concord, Canada) equipped with a nano electrospray ion source. Detailed method has been reported previously (Wang et al. 2016). Briefly, 10 μl was injected onto a 50 mm × 300 μm C18 trap column (Agilent Technologies, Sydney, Australia), which was then placed in line with 150 mm × 75 μm 300SBC18, 3.5um nano HPLC column (Agilent Technologies, Australia) for mass spectrometry analysis. Linear gradients of 1–60% solvent B over 30 min at 300 ml/min flow rate, followed by a steeper gradient from 60 to 80% solvent B in 5 min, were used for peptide elution. Solvent A consisted of 0.1% formic acid (aq) and solvent B contained 90/10 acetonitrile/0.1% formic acid (aq). The ion spray voltage was set to 2400 V, nebuliser gas 1 (GS1) 12 and interface heater at 150 °C. Full scan TOFMS data was acquired over the mass range 350–1800 and for product ion ms/ms 100–1800. Ions observed in the TOF-MS scan exceeding a threshold of 100 counts and a charge state of + 2 to + 5 were set to trigger the acquisition of product ion. The data was acquired and processed using Analyst TF 1.5.1 software (AB SCIEX, Concord, Canada).
Trypsin Shaved Protein Identification
The LC-MS/MS data were imported to the PEAKS studio (Bioinformatics Solutions Inc., Waterloo, ON, Canada, version 7.0). The database used for the analysis in this study was derived from an in-house P. maxima transcriptome. De novo sequencing of peptides, database search and characterising specific PTMs were used to analyse the raw data; false discovery rate (FDR) was set to ≤ 1%, and [− 10*log(p)] of proteins was calculated accordingly where p is the probability that an observed match is a random event. The PEAKS used the following parameters: (i) precursor ion mass tolerance, 0.1 Da; (ii) fragment ion mass tolerance, 0.1 Da (the error tolerance); (iii) tryptic enzyme specificity with two missed cleavages allowed; (iv) monoisotopic precursor mass and fragment ion mass; (v) a fixed modification of cysteine carbamidomethylation; and (vi) variable modifications including lysine acetylation, deamidation on asparagine and glutamine, oxidation of methionine and conversion of glutamic acid and glutamine to pyroglutamate. The identified proteins were used as queries for BLASTp searching (NCBI; www.ncbi.nlm.nih.gov) with an e-value cut-off 10−3.
Results
Exposure of P. maxima females to stripped P. maxima sperm, in an initial bioassay, stimulated 80% of females to spawn (n = 20) within 0.5–4.5 h (mean 112 min ± 18.9 min), compared to 0% spawning for females not exposed to stripped sperm (n = 20). For all following spawning assays, live-stripped sperm was used as the positive control. Treatment SP1 (sperm milt) did not induce any spawning, and all spawning was induced within sperm membrane-associated treatments or by whole sperm. The results of all milt and sperm extract treatments on P. maxima females spawning are presented in Table 1, showing spawn percentages induced by treatments and corresponding positive controls.
Analysis of Female P. maxima Spawning in Response to Semi-Purified and RP-HPLC Sperm Membrane Fractions Using Initial Protein Extraction Protocol
Treatment SP2 (sperm membrane-associated molecules, method 1) showed some bioactivity, inducing 7% of female oysters to spawn after 135 min [21% of spawn rate of positive control oysters (after mean 196 min ± 60 min)]. SP2 extracts were further purified by RP-HPLC and fractions collected between 10 and 50 min (SP5); in assay, these showed a low level of bioactivity. A representative RP-HPLC chromatogram of SP5 is shown in Fig. 2a, and pooled RP-HPLC fractions at 10–30, 30–50 min and 30–40 and 40–50 min were tested for spawning induction. Only pooled fractions 30–50 min triggered spawning, in 7.6% of females, approximately 240 min post-treatment. SP3 (sperm pellet membranes) induced spawning in 30% of females after mean 133 min ± 4 min [75% of spawn rate of positive control oysters (after mean 134 min ± 27 min)]. Sperm membranes treated with proteinase K (SP4) did not induce any spawning.
RP-HPLC of sperm preparations. a A representative RP-HPLC profile of water-soluble sperm membrane-associated molecules (SP5) extracted using the initial protein extraction method. Fractions were pooled at 5 min intervals, then lyophilised. RP-HPLC conditions were 0–10% CH3CN/0.1%TFA over 5 min, then 10–60% over 50 min, then 60–80% over 5 min. b A representative RP-HPLC profile of water-soluble sperm membrane-associated molecules (SP7), extracted using the secondary protein extraction method. Fractions were collected at 1 min intervals and lyophilised. RP-HPLC conditions were 0–10% CH3CN/0.1%TFA over 5 min, then 10–60% over 50 min, then 60–80% over 5 min. mAU: map absorbance units
Analysis of Female P. maxima Spawning in Response to Semi-Purified and RP-HPLC Fractions of Semi-Purified Sperm Extracts Using Secondary Protein Extraction Protocol
Treatment SP6 (sperm membrane-associated molecules, method 2) induced spawning in 30% of experimental oysters after mean 92 min ± 17 min [75% of spawn rate of positive control oysters (after mean 134 min ± 27 min)]. Fractions of this extract (SP7), separated by RP-HPLC and collected between 30 and 51 min, showed some bioactivity. A representative RP-HPLC chromatogram is shown in Fig. 2b, demonstrating a complex mixture of biomolecules. Spawning was triggered in 10% of animals treated with combined RP-HPLC fractions between 30 and 36 min after 35 min (12.5% of positive control oyster spawn rate (after mean 103 min ± 22 min)). No spawning was observed when females were treated with pooled RP-HPLC 37–43 min fractions and 44–51 min. When these two treatments were repeated at double the dosage, they induced 30 and 11% spawning after mean 197 min ± 57 min and 90 min, respectively [30 and 11% of positive control oyster spawn rate (after mean 85 min ± 18 min)]. SP8 (sperm pellet membranes) induced spawning in 80% of females after mean 105 min ± 15 min [80% of spawn rate of positive control oysters (after mean 85 min ± 18 min)].
LC-MS Analysis of Sperm Membrane-Association Proteins
P. maxima sperm were collected and the membranes ‘shaved’ (with trypsin) to elucidate membrane-associated proteins. LC-MS/MS analysis identified 27 proteins (Table 2 and File S1 for sequence information). The majority of the identified proteins match to proteins associated with cell structure (i.e. tubulin, actin and tektin) and regulation of cell metabolism (i.e. arginine kinase, ATP synthase beta subunit). One sperm protein identified has similarity to a protein described in Crassostrea virginica as an atrial natriuretic peptide (ANP) receptor 1. Domain analysis indicates that it contains domains consistent with the purple sea urchin (Strongycentrotus purpuratus) speract receptor (Fig. S1), including a ligand-binding domain of membrane bound guanylyl cyclases (which are activated by sperm-activating peptides), adenylate and guanylate cyclase catalytic domain and a cyclase homology domain. Another P. maxima sperm protein consisting of 438 amino acids did not have any significant similarity to any known proteins in the NCBI database.
Discussion
In aquatic molluscs, synchronous broadcast spawning is essential to ensure successful fertilisation of gametes. The pheromones that signal conspecifics to initiate spawning are largely unknown, yet hold the key for manipulating animal behaviour. The aim of this study was to initially determine the existence of a sperm-associated SIP in the pearl oyster P. maxima. From there, biochemical purification approaches and behavioural analyses were used to drill down into the nature of the SIP. These findings provide new insight towards the development of a sperm-free stimulant that can reliably induce spawning in female P. maxima, paving a path towards revolutionising broodstock management and oyster farming.
Female P. maxima have never been documented to spawn under hatchery conditions without being exposed to conspecific sperm. We confirmed this in a bioassay that examined spawning responses of females exposed to live, conspecific sperm, compared with unexposed females; all females were exposed otherwise to identical manipulations. The results confirmed the presence of a SIP in P. maxima sperm, as it induced spawning in 80% of females. This spawn percentage is considered a highly acceptable spawn rate in the aquaculture industry, as natural variability of oyster health and sexual maturity are contributing factors to spawning success; it is uncommon for 100% of females to spawn in hatchery conditions.
The absolute requirement for the presence of sperm to induce spawning in P. maxima proved it an excellent species for bioassays that could narrow down the nature of a sperm-associated SIP. Similar studies have been conducted on C. virginica; however, there both males and females can spawn in response to spawn-induction manipulation alone (without the addition of sperm) (Galtsoff 1938; Rice et al. 2002), making it harder to ascertain the role of the SIP.
All milt components were tested separately from sperm cells in spawning bioassays. Centrifugation was used to separate sperm cells from milt, as was done in the study on C. virginica (Rice et al. 2002). Milt components did not induce spawning in female P. maxima, indicating that the SIP is in the sperm itself. Following the C. virginica membrane extraction guidelines (Rice et al. 2002), homogenisation was similarly performed to release extrinsic bioactive molecules from P. maxima sperm membranes, facilitating further purification by Sep-Pak and RP-HPLC. Spawning was induced using Sep-Pak-purified supernatant obtained from homogenised sperm cells (see SP2), although at a relatively low percentage compared with the positive controls (live sperm). Those P. maxima sperm membranes remaining from homogenisation (as concentrated pellets, see SP3) induced spawning with similar success rates to that of positive controls. These results indicated that P. maxima SIP is associated with the sperm membrane, consistent with results reported for C. virginica (Galtsoff 1938; Rice et al. 2002).
Rice et al. (2002) established that the SIP in C. virginica sperm was proteinaceous. They found that protein-denaturing procedures, such as multiple freeze-thawing, heat treatment and proteases, resulted in a reduction of conspecific spawning activity by 68%. To investigate whether P. maxima SIP is likely to be (or contain) a protein or a peptide, sperm membranes were incubated with proteinase K. This was effective in fully abolishing spawning activity, when compared to sperm membranes not treated with proteinase K, confirming that the P. maxima SIP is proteinaceous or includes a proteinaceous component. Aquatic pheromones characterised to date consist of amino acids, peptides or proteins (Cummins et al. 2011; Cummins et al. 2005; Kikuyama et al. 1995; Rice et al. 2002; Snell and Nacionales 1990), prostaglandins (Appelt and Sorensen 2007; Eom et al. 2009), steroids (Stacey et al. 1989) or bile acids (Li et al. 2002). Peptides and proteins that act as sex attraction pheromones and aggression pheromones have been discovered in several molluscs. For example, the protein attractin has been purified from several Aplysia spp. and B. leachii and found to induce aggregation and mating (Cummins et al. 2005; Painter et al. 2003). The abalone H. asinina secretes water-soluble proteins in mucous, enabling detection and trail following of conspecifics (Kuanpradit et al. 2012). The longfin squid L. pealeii has a contact pheromone that triggers male-male aggression that is also proteinaceous (Cummins et al. 2011). Female L. pealeii embed this protein pheromone into the surface of their eggs, which they lay on spawning grounds. Males, visually attracted to the eggs, become extremely aggressive towards one another upon contact with the pheromone through their sensory arm suckers. A 10 kDa protein was characterised from homogenised egg capsules which induced this aggressive response in behavioural bioassays. These studies helped to lay a platform for similar molecular characterisation of the proteinaceous SIP in P. maxima sperm.
Once the P. maxima SIP had been confirmed as proteinaceous and associated with the sperm membrane, purification procedures were modified to select for these membrane-associated proteins. Sperm membrane proteins can be either integral to the cell membrane (intrinsic membrane proteins), as is the SIP in C. virginica sperm, or associated with the surface lipid layer on the membrane (extrinsic membrane proteins) (Rice et al. 2002; Vertomenn et al. 2011). Extrinsic membrane proteins can be removed from membranes by homogenisation techniques, but intrinsic proteins cannot (Rice et al. 2002).
As supernatant from Sep-Pak purified, homogenised sperm cells (SP2) induced spawning, it was deemed likely that the P. maxima SIP was extrinsic to the sperm membrane. These biomolecules, however, induced a low percentage of spawning, so it was concluded that mechanical homogenisation alone was not entirely effective in releasing the P. maxima SIP from membranes, or that the SIP could have an intrinsic component as well. For this reason, the protocol was modified (high pH 12.65; SP6) in an attempt to extract more extrinsic membrane-associated proteins. This pH increase prior to mechanical homogenisation prevents ruptured sperm membranes from resealing, enhancing the release of soluble proteins attached to the membrane lipid layer (Vertomenn et al. 2011). Bioassays using these purified fractions yielded higher spawn rates than those extracted from the initial extraction protocol SP2. This indicated that secondary protocol SP6 may have succeeded in extracting more bioactive protein from the sperm membranes. However, sperm membranes remaining after protein extractions were still highly active, indicating that not all of the SIP was extracted from membranes.
Since sperm membranes themselves induced a greater spawning response than extracts following semi-purified extract treatments, it seems likely that the P. maxima SIP could be primarily membrane intrinsic, or exists as a combination of extrinsic and intrinsic membrane bound molecules. Potentially, relatively small amounts of intrinsic active protein may have been released through our extraction procedures, enough to induce spawning of some animals. Similar extrinsic membrane protein extraction methods (NaCO3 pH wash, > 11), used by Rice et al. (2002) on C. virginica sperm resulted in no induction of oyster spawning.
In order to further separate the sperm extracts based on hydrophobicity, homogenised sperm extracts from SP2 were semi-purified by Sep-Pak; then, biomolecules were separated into fractions by RP-HPLC. Initial bioassay of RP-HPLC fractions of homogenised sperm membrane extracts (SP5) revealed spawn-inducing bioactivity within RP-HPLC fraction 30–50 min. When this fraction was further divided into two groups (30–40 and 40–50 min) for testing, no spawning was observed with either. This may be explained through synergistic activities of bioactive molecules. Based on pheromone studies in other animal species, multi-component pheromones are very common (Boo 1998; Tillman et al. 1999; Fine and Sorenson 2008). This may also explain why fractions of purified P. maxima sperm extracts resulted in low percentage spawning responses in bioassays, compared to that of positive controls and sperm membranes. It is possible that these fractions contained component/s of the SIP, but not its entirety. That is, there is a synergistic relationship between several molecules throughout the 30–50-min fraction.
Bioassay of RP-HPLC fractions extracted by the secondary protocol (SP6) revealed that a number of pooled fractions induced spawning (RP-HPLC min 30–36, 37–43 and 44–51). Similar testing had previously proved ineffective when extraction was carried out by mechanical homogenisation alone (SP5). The relatively low percentage of spawns in these groups compared to positive controls and combined semi-purified membrane associated molecules from SP6 indicate that the SIP has the greatest bioactivity when all components are present; however, some activity is also present after separation. This supports the idea that the P. maxima SIP is likely to be a multi-component pheromone, present within RP-HPLC fractions 30–50 min.
P. maxima SIP was found to be highly resistant to degradation since freezing, pH change (approximately pH 2–pH 12.65) and lyophilisation did not destroy bioactivity. All sperm was frozen prior to purification (− 70 °C), and yet the SIP maintained bioactivity, as did the SIP of C. virginica (Rice et al. 2002). Dramatic pH change of sperm from P. maxima and C. virginica showed that both SIPs were also resistant to denaturation by pH (Rice et al. 2002). Also, Sep-Pak purification and RP-HPLC methodologies, which included the chemicals acetonitrile and trifluoroacetic acid, did not destroy its activity. This is consistent with the characterisation of the contact pheromone of squid (Loligo pealeii) (Cummins et al. 2011) and attractin from sea hare (Aplysia californica) (Painter et al. 2003), which consist of peptides or small proteins purified through Sep-Pak from target tissues. These similar studies provided a level of confidence in using such methods to purify a SIP from P. maxima sperm without destroying it.
Our analysis of the P. maxima sperm membrane proteins revealed numerous proteins closely associated with sperm cell structure and cell metabolism. Since the function of the latter relates to intracellular processes, these are likely contaminants that were co-purified during sample preparation. However, a speract-like receptor was identified that likely localises to the oyster sperm membrane. To date, this cell-surface receptor has only been described in echinoderms where it is activated by egg-associated sperm-activating peptides, such as speract (Lessios 2011). Sperm-activating peptides are species-specific and 75 different peptides have been determined from 15 sea urchin species (Suzuki 1995). The P. maxima speract-like receptor may also help facilitate sperm chemotaxis in oysters although the identity of an egg-associated sperm-activating peptide is currently unknown. We conclude that its presence on P. maxima sperm membranes implicates it as a candidate spawn inducer if detected by mature oysters. In addition, a protein that appears to be specific to P. maxima is a candidate by virtue of the fact that a SIP would benefit the species if species-specific.
A significant outcome of this study concerns the finding that P. maxima sperm membranes hold a SIP, capable of inducing spawning in female and male conspecifics. Due to its resilient nature, stripped sperm can easily be prepared to form a stable, crude product for use in pearl oyster aquaculture, which now provides a biotechnology tool for greater control over spawning and a more efficient approach to advance genetic breeding.
Conclusions
In conclusion, we have confirmed that (1) P. maxima females do not spawn without the addition of a SIP present in conspecific sperm, (2) the SIP is associated with the sperm membrane, (3) the SIP is proteinaceous and (4) the SIP is likely a multi-component pheromone located within RP-HPLC 30–50 min (using gradient conditions specified in methods section). Lyophilised sperm membranes were found to be an excellent crude biotechnology product to induce spawning in male and female P. maxima without the addition of live sperm. This in itself provides industry with a sperm-free stimulant, highly effective in inducing male and female spawning that can be used the induction of controlled spawning as well as for genetic family line research and management. Finally, we provide a list of proteins identified from P. maxima sperm membrane preparations, including a speract-like receptor and novel protein.
References
Agelopoulou B, Cary PD, Pataryas T, Aleporou-Marinou V, Crane-Robinson C (2004) The sperm-specific proteins of the edible oyster (European flat oyster (Ostrea edulis)) are products of proteolytic processing. Biochim Biophys Acta 1676(1):12–22
Appelt CW, Sorensen PW (2007) Female goldfish signal spawning readiness by altering when and where they release a urinary pheromone. Anim Behav 74(5):1329–1338
Boo KS (1998) Variation in sex pheromone composition of a few selected lepidopteran species. J Asia Pac Entomol 1(1):17–23
Chung-Davidson Y-W, Huertas M, Li W 2010 A review of research in fish pheromones. Chem Commun Crustac 467–482. https://doi.org/10.1007/978-0-387-77101-4_24
Cummins SF, Schein CH, Xu Y, Braun W, Nagle GT (2005) Molluscan attractins, a family of water-borne protein pheromones with interspecific attractiveness. Peptides 26(1):121–129
Cummins SF, Boal JG, Buresch KC, Kuanpradit C, Sobhon P, Holm JB, Degnan BM, Nagle GT, Hanlon RT (2011) Extreme aggression in male squid induced by a β-MSP-like pheromone. Curr Biol 21(4):322–327
Eom J, Jung YR, Park D (2009) F-series prostaglandin function as sex pheromones in the Korean salamander, Hynobius leechii. Comp Biochem Physiol A Mol Integr Physiol 154(1):61–69
Fine J, Sorenson PW (2008) Isolation and biological activity of the multi-component sea lamprey migratory pheromone. J Chem Ecol 34(10):1259–1267
Galtsoff P (1938) Physiology of reproduction of Ostrea virginica II. Stimulation of spawning in the female oyster. Biol Bull 75(2):286–307
Jonáková V, Manásková P, Tichá M (2007) Separation, characterization and identification of boar seminal plasma proteins. J Chromatogr B 849(1–2):307–314
Kikuyama S, Toyoda F, Ohmiya Y, Matsuda K, Tanaka S, Hayashi H (1995) Sodefrin: a female-attracting peptide pheromone in newt cloacal glands. Science 267(5204):1643–1645
Kuanpradit C, Stewart MJ, York PS, Degnan BM, Sobhon P, Hanna PJ, Chavadej J, Cummins SF (2012) Characterization of mucus-associated proteins from abalone (Haliotis) – candidates for chemical signaling. FEBS J 279:437–450
Lessios HA (2011) Speciation genes in free-spawning marine invertebrates. Integr Comp Biol 51(3):456–465
Li W, Scott AP, Siefkes MJ, Yan H, Liu Q, Yun S-S, Gage DA (2002) Bile acids secreted by male sea lamprey that acts as a sex pheromone. Science 296(5565):138–141
Mamangkey NGF, Southgate PC (2009) Regeneration of excised mantle tissue by the silver-lip pearl oyster, Pinctada maxima (Jameson). Fish Shellfish Immunol 27(2):164–174
Minaur J (1969) Experiments on the artificial rearing of the larvae of the Pinctada maxima (Jameson) (Lamellibranchia). Aust J Mar Freshwat Res 20:175–187
Painter SD, Clough B, Black S, Nagle GT (2003) Behavioral characterization of attractin, a water-borne peptide pheromone in the genus Aplysia. Biol Bull 25(1):16–25
Queensland South Sea Pearls (2011) Artificial spat culture in Australia. http://www.qssp.com.au/spat_culture%20.htm. Viewed 10 March 2011
Reddy GVP, Guerrero A (2010) New pheromones and insect control strategies. In: Gerald L (ed) Vitamins & hormones, vol. 83. Academic Press, Oxford, pp. 493–519
Rice P, Ray SM, Painter SD, Nagle GT (2002) Intrinsic membrane protein in oyster sperm stimulates spawning behaviours in Crassostrea virginica: implications for aquaculture. J Shellfish Res 21(2):715–718
Rose RA (1990) A manual for the artificial propagation of the silverlip or goldlip pearl oyster, Pinctada maxima, (Jameson) from Western Australia’ Fishries Department, Western Australian Marine Research Laboratories, P.O. Box 20, North Beach, WA 6020, Australia
Snell TW, Nacionales MA (1990) Sex pheromone communication in Brachionus plicatilis (rotifera). Comp Biochem Physiol A Physiol 97(2):211–216
Solis N, Larsen MR, Cordwell SJ (2010) Improved accuracy of cell surface shaving proteomics in Staphylococcus aureus using a false-positive control. Proteomics 10:2037–2049
Stacey NE, Sorensen PW, Van Der Kraak GJ, Dulka JG (1989) Direct evidence that 17[alpha],20[beta]-dihydroxy-4-pregnen-3-one functions as a goldfish primer pheromone: Preovulatory release is closely associated with male endocrine responses. Gen Comp Endocrinol 75(1):62–70
Suzuki N (1995) Structure, function and biosynthesis of sperm-activating peptides and fucose sulfate glycoconjugate in the extracellular coat of sea urchin eggs. Zool Sci 12(1):13–27
Tanaka Y, Kumeta M (1981) Successful artificial breeding of the silver lip pearl oyster, Pinctada maxima (Jameson). Bull Natl Res Inst Aquac (Jpn) 2:21–28
Taverna F, Negri A, Piccinini R, Zecconi A, Nonnis S, Ronchi S, Tedeschi G (2007) Characterization of cell wall associated proteins of a Staphylococcus aureus isolated from bovine mastitis case by a proteomic approach. Vet Microbiol 119:240–247
Tillman JA, Seybold SJ, Jurenka RA, Blomquist GJ (1999) Insect pheromones—an overview of biosynthesis and endocrine regulation. Insect Biochem Mol Biol 29(6):481–514
Tranter DJ (1958) Reproduction in Australian pearl oyster (Lamellibranchia). II. Pinctada albina (Lamarck): Gametogenesis. Aust J Mar Freshwat Res 9:144–158
Vertomenn A, Panis B, Swennen R, Carpentier SC 2011 Challenges and solutions for the identification of membrane proteins in nonmodel plants. J Proteomics
Wang T, Zhao M, Rotgans BA, Strong A, Liang D, Ni G, Limpanont Y, Ramasoota P, Mcmanus DP, Cummins SF (2016) Proteomic Analysis of the Schistosoma mansoni Miracidium. PLoS One 11:e0147247
Acknowledgements
We would like to thank Paspaley Pearling Company for providing the pearl oysters used in this study, the Darwin Aquaculture Centre, Northern Territory Department of Primary Industry and Resources for the use of their facilities, and the University of the Sunshine Coast for use of the Genecology labs.
On behalf of all authors, the corresponding author states that there is no conflict of interest.
Author information
Authors and Affiliations
Corresponding author
Electronic supplementary material
Rights and permissions
About this article
Cite this article
Taylor, A., Mills, D., Wang, T. et al. A Sperm Spawn-Inducing Pheromone in the Silver Lip Pearl Oyster (Pinctada maxima). Mar Biotechnol 20, 531–541 (2018). https://doi.org/10.1007/s10126-018-9824-6
Received:
Accepted:
Published:
Issue Date:
DOI: https://doi.org/10.1007/s10126-018-9824-6