Abstract
The objective of the present study was to isolate Actinobacteria, preferably Streptomyces spp. from the rhizosphere soils of three ethno-medicinal plants collected in Serbia (Papaver rhoeas, Matricaria chamomilla, and Urtica dioica) and to screen their antifungal activity against Candida spp. Overall, 103 sporulating isolates were collected from rhizosphere soil samples and determined as Streptomyces spp. Two different media and two extraction procedures were used to facilitate identification of antifungals. Overall, 412 crude cell extracts were tested against Candida albicans using disk diffusion assays, with 42% (43/103) of the strains showing the ability to produce antifungal agents. Also, extracts inhibited growth of important human pathogens: Candida krusei, Candida parapsilosis, and Candida glabrata. Based on the established degree and range of antifungal activity, nine isolates, confirmed as streptomycetes by 16S rRNA sequencing, were selected for further testing. Their ability to inhibit Candida growth in liquid culture, to inhibit biofilm formation, and to disperse pre-formed biofilms was assessed with active concentrations from 8 to 250 μg/mL. High-performance liquid chromatographic profiles of extracts derived from selected strains were recorded, revealing moderate metabolic diversity. Our results proved that rhizosphere soil of ethno-medicinal plants is a prolific source of streptomycetes, producers of potentially new antifungal compounds.
Similar content being viewed by others
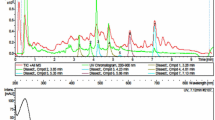
Avoid common mistakes on your manuscript.
Introduction
Soil is a nutritionally, biologically, and physically complex and diverse habitat. Its inhabitants are able to perform a broad range of metabolic processes and to produce an immense diversity of bioactive metabolites (Maleki et al. 2013). Soil microorganisms, such as bacteria and fungi, control the ecosystem by decomposition of nutrients and may serve as indicators of ecosystem health (Stamenov et al. 2018). Recent advances in exploring microbial diversity in soil have revealed the remarkably rich biosynthetic potential for the production of new natural products among microbial strains, especially within the group of Actinobacteria (Genilloud 2017). The genus Streptomyces is the largest antibiotic-producing genus of the phylum Actinobacteria and its representatives are widely distributed in soils, especially in those that are dry, not too acidic, and rich in organic matter (Waksman and Henrici 1943). The species belonging to the genus Streptomyces constitute 50% of the total population of soil actinomycetes and 75–80% of the commercially and medicinally useful antibiotics have been derived from this genus (Challis and Hopwood 2003). They produce compounds with antibacterial, antifungal, anti-infective, anticancer, and antitumor activity. Streptomyces spp. are thus recognized as industrially important organisms for their impressive ability to produce structurally and functionally diverse novel secondary metabolites (Mellouli et al. 2003; Williams and Mayfield 1971).
Diverse microbes of economic importance, including streptomycetes, are related to medicinal plants. Medicinal plants harbor a distinctive microbiome due to their unique and structurally divergent bioactive secondary metabolites that are most likely responsible for the high specificity of the associated microorganisms (Rios and Recio 2005). Plant rhizosphere soil contains a large phylogenetic diversity of microorganisms which have proven to be an unlimited source for potential drugs, agrochemicals, and biocatalysts (Köberl et al. 2013; Spasic et al. 2018). These microorganisms have been processed to produce hundreds of valued commercial products in order to provide efficient industrial biotechnological solutions (Golinska et al. 2015; Köberl et al. 2013).
Despite the long list of currently available antibiotics in the market, antifungal drugs constitute a small but significant group of molecules and they have an important role in the control of mycotic diseases. Only a limited number of antifungal agents are currently available for the treatment of life-threatening fungal infections (Vicente et al. 2003). The search for new, safer, and broad-spectrum antifungal drugs with greater potency has been progressing slowly. Therefore, the development of new antifungal agents, preferably naturally occurring with novel mechanisms of action, is an urgent medicinal need (Thakur et al. 2007). Keeping in view the extensive utility of actinomycetes for natural product biosynthesis, the aim of this study was to highlight the presence of Streptomyces spp. in different rhizosphere soils of ethno-medicinal plants collected in Serbia and to comprehensively evaluate their potential to produce secondary metabolites with antifungal activity.
Materials and methods
Sampling and isolation of Streptomyces species
Rhizosphere soil samples of medicinal plants were collected in Serbia during the years 2015 and 2016. Soil samples were stored at + 4 °C and transferred to the laboratory. The soil samples (1 g) were suspended in HNC medium (yeast extract 60 g/L, sodium dodecyl sulphate 0.5 g/L, CaCl2 0.5 g/L) and heated at 42 °C for 30 min (Schrey et al. 2012). Serial dilutions of the suspension were prepared by the 10-fold dilution method. For each of the 10−3, 10−4, and 10−5 dilutions of the suspension, aliquots of 0.1 mL were spread onto the surface of MSF (mannitol 20 g/L, soybean flour 20 g/L, agar 20 g/L) (Stankovic et al. 2013) and ISP2 (glucose 4 g/L, yeast extract 4 g/L, malt extract 10 g/L, agar 20 g/L), containing nystatin (4 μg/mL), nalidixic acid (10 μg/mL), and cycloheximide (5 μg/mL). Plates were incubated at 30 °C for 7 days, and sporulating colonies of distinct morphological appearance were subcultured in MSF medium to obtain pure isolates. Spore suspensions were prepared in glycerol (20%, v/v), maintained at − 80 °C, and used for the inoculation of cultures for further experiments (Kieser et al. 2000).
Morphological characteristics of isolates
Morphological characteristics such as aerial mass color and substrate mycelium were observed on MSF plates. The aerial mass was classified according to Bergey’s manual of systematic bacteriology in the following color series: gray (G), white (W), yellow (Y), blue (Bl), and mixed (Mix). Distinctive colors of the substrate mycelium were recorded as follows: beige (Bg), black (Bck), blue (Bl), biscuit (Bs), brown (Bw), orange (O), yellow (Y) (Vos et al. 2009).
Cultivation of Streptomyces spp. and preparation of crude culture extracts
Spore suspensions (20 μL) of different Streptomyces spp. isolates were firstly inoculated into vegetative medium (maltose 15 g/L, tryptone soya broth 8 g/L, yeast extract 4 g/L, CaCO3 2 g/L) (Ilic-Tomic et al. 2015) and incubated on a shaker at 30 °C for 48 h, 180 rpm. This preculture was used for inoculation (1%, v/v) of two production media: JS (glucose 20 g/L, starch 20 g/L, mannitol 15 g/L, soybean flour 30 g/L, CaCO3 10 g/L) (Stankovic et al. 2013) and R2YE (K2SO4 0.25 g/L, MgCl4 × 6H2O 10 g/L, sucrose 103 g/L, glucose 10 g/L, casamino acids 0.1 g/L with additionally added 0.5% (w/v) KH2PO4 10 mL/L, 3.68%(w/v) CaCl2 × 2H2O 80 mL/L, 20% (w/v) L-proline 15 mL/L, 4 М TES buffer 100 mL/L, trace elements solution 2 mL/L, 1 M NaOH 5 mL/L, yeast extract 6.2 g/L) (Kieser et al. 2000). Cultures were grown in Erlenmeyer flasks (1:5, culture to volume ratio) containing coiled stainless steel springs for better aeration at 30 °C, 180 rpm for 7 days. Extraction of Streptomyces whole cultures with ethyl acetate (EtOAc) (1:1/v:v) was performed by vigorous mixing at 30 °C for 12 h. The EtOAc extract was separated from the cell debris by centrifugation (5000 rpm for 20 min at 4 °C; Eppendorf 5804R bench top centrifuge). The mycelium residue was afterwards extracted with methanol (MeOH) (1/10 of the original culture volume) by vigorous mixing at 30 °C for 30 min. The MeOH extract was separated from the cell debris by centrifugation (5000 rpm for 20 min at 4 °C; Eppendorf 5804R bench top centrifuge). Both extracts were then separately dried with anhydrous MgSO4, followed by drying under vacuum (BUCHI Rotavapor® R-300, Germany) and the dry mass of each extract was determined.
Antifungal activity of Streptomyces spp. extracts
Antifungal activity was tested by standard disc diffusion assays against type strains: C. albicans ATCC 10231, C. krusei ATCC 6258, C. parapsilosis ATCC 22019, C. glabrata ATCC 2001. Briefly, late stationary phase cells of individual test microorganisms were spread on Sabouraud dextrose agar plates (Hamid et al. 2014). The extracts were dissolved in dimethyl sulfoxide (DMSO) to a concentration of 50 mg/mL and applied to sterile discs (500 μg per disc) with the same amount of the DMSO solvent serving as a negative control. The plates were incubated at 30 °C. For the initial screen, the zones of inhibition were measured after 24 h. For the control purpose, nystatin (Acros Organics, New Jersey, USA) was dissolved in DMSO at 5 mg/mL and applied to sterile discs to achieve 50 μg per disc.
Minimal inhibitory concentrations (MICs) were determined for nine selected isolates according to CLSI broth microdilution guidelines (Clinical and Laboratory Standards Institute 2008, 2012). MICs of the examined extracts were determined in RPMI—1640 medium (Sigma, Adrich, USA). The MIC value corresponds to the lowest concentration that inhibited the growth of the respective test organism after 24 h at 37 °C for the examined extracts. The highest concentration of the examined extracts used in these assays was 250 μg/mL.
Antibiofilm activity was determined for nine selected isolates using C. albicans. Minimal biofilm inhibitory concentrations (MBIC) and minimal biofilm eradication concentrations (MBEC) of the extracts were studied by using a previously reported 96-well microtiter plate assay (Pierce et al. 2008). In the biofilm inhibition and biofilm eradication assays inoculums were 106 CFU/mL. Starting concentrations of the examined extracts were 250 μg/mL with two-fold serial dilutions following. The lowest concentration that inhibited biofilm formation was evaluated after incubation for 48 h at 37 °C. In biofilm eradication assays, pre-formed biofilms (24 h at 37 °C) were incubated for 24 h with decreasing concentrations of the examined extracts. Biofilm growth was analyzed by crystal violet (CV) staining of adherent cells and estimated as absorbance at 530 nm on Tecan Infinite 200 Pro multiplate reader (Tecan Group Ltd., Männedorf, Switzerland).
HPLC analysis of crude extracts of selected isolates
In order to estimate the metabolic complexity of the organic extracts of the investigated bacterial strains, samples were analyzed by analytical HPLC on a JASCO system consisting of a UV-1575 Intelligent UV/VIS Detector, DG-2080-53 3-Line Degasser, two PU-1580 Intelligent HPLC Pumps, AS-1550 Intelligent Sampler and HG-1580-32 Dynamic Mixer controlled by the Galaxie chromatography software (Version 1.8.6.1) provided by Jasco. A total of 20–50 μL of each extract was separated on a Eurosphere II 100-3 C18 A (150 × 4.6 mm) column with integrated pre-column manufactured by Knauer (KNAUER Wissenschaftliche Geräte GmbH, Berlin, Germany). Eluents included water (A) and acetonitrile (B) buffered with trifluoroacetic acid (0.1% TFA) at a flow rate of 1 mL/min. The gradient started with 5% B and reached 95% B by 23 min. Peaks were detected by UV-VIS diode array detector at 220 nm. Full UV spectra of the major metabolites were also recorded online.
DNA isolation and sequencing of the 16S rRNA gene
Genomic DNA was isolated by a previously described method (Nikodinovic et al. 2003). The 16S rRNA sequence was amplified from genomic DNA using universal bacterial primers 27F (5′-AGAGTTTGATCCTGGCTCAG-3′) and 1492R (5′-GGTTACCTTGTTACGACTT-3′) (Reysenbach et al. 1994). PCR amplification was performed in a 2720 Thermal Cycler (Applied Biosystems, Thermo Fisher Scientific, Waltham, USA) using KAPA Taq PCR kit (KAPA Biosystems, USA) following the manufacturer’s protocol. PCR products were purified using a PCR purification kit (Qiagen, Germany). Sequencing was performed with a BigDye™ Terminator v3.1 Cycle Sequencing Kit (Applied Biosystems, Thermo Fisher Scientific, Waltham, USA) on an Applied Biosystems 3130 Genetic Analyzer. 16S rRNA gene sequences were identified using BLASTN program (Altschul et al. 1997).
16S rRNA gene phylogeny
The obtained forward and reverse sequences were assembled by the SeqMan Pro software (DNASTAR Inc., Madison, USA). Alignment of sequences and homologous sequences taken from GeneBank was performed with the Clustal W 2.0 algorithm (Larkin et al. 2007). The phylogenetic tree was constructed by the maximum likelihood algorithm using Jukes-Cantor distance correction and Bootstrap resampling method, all included in the MEGA 7 package (Kumar et al. 2016). The tree was rooted using the 16S rRNA gene sequence of Bacillus subtilis 168 (NC000964.3) as an outgroup. Sequences of the nearest type strains, as well as the outgroup strain, were taken from GeneBank. 16S rRNA gene sequences (at least 1400 nt) were deposited in GeneBank under accession numbers MF11788, MF11800, MF11796, MF11789, and MH128156–MH128160.
Results
Streptomyces spp. isolation
Rhizosphere soil samples were collected from three ethno-medicinal plants (P. rhoeas, poppy; M. chamomilla, chamomile; and U. dioica, nettle) and treated with conditions that facilitate streptomycetes isolation. Pre-treatment of soil samples (including the choice of media, temperature, antibiotics, and duration of incubation) was performed in order to induce streptomycetes species growth and sporulation. A total of 103 sporulating isolates, presumably streptomycetes, were isolated: 50, 33, and 20 isolates from poppy, chamomile, and nettle, respectively. Selected colonies were slow growing, aerobic, glabrous or chalky, heaped, folded, and with aerial and substrate mycelia of different colors. In addition, all excreted an earthy odor of geosmin, characteristic for Streptomyces spp.
In accordance with the aerial mycelium color, 103 isolates were grouped in the following series: white (57%), gray (31%), and yellow (11%). Beside these, one isolate had blue aerial mass and one was recorded as mix of colors (pink, blue, and white color of colonies). With substrate mycelia of isolated strains, a broadened spectrum of colors was observed: yellow (29%), biscuit (18%), beige (17%), brown (15%), orange (10%), black (5%), blue (4%), and gray (2%), with comparable distribution for each plant (Fig. 1).
Antifungal activity of Streptomyces spp.
All 103 soil isolates were cultivated in two nutrient rich, but distinctively different media, JS and R2YE. Seven days old whole cultures were extracted with EtOAc and the mycelial residues were subsequently extracted with MeOH. In total, 412 extracts from these cultivations (JS-EtOAc, JS-MeOH, R2YE-EtOAc, and R2YE-MeOH for each isolate) were screened for antifungal activity with disc diffusion assays against C. albicans ATCC10231 with 21% (88/412) determined as positive (data not shown). Out of 206 extracts obtained from JS medium, 32% (66/206) were active, while 11% (22/206) were active from cultures grown in R2YE. Out of the EtOAc extracts, 29% (59/206) were active, while 14% (29/206) active extracts were recorded when methanol was used for residual mycelium extraction (data not shown). Overall, the active extracts were associated with 43 different isolates (Supplementary Table 1).
After this initial screening, the 43 isolates that showed inhibitory activity against C. albicans ATCC 10231 were selected for further antifungal activity assessment against three non-albicans Candida type strains namely, C. krusei, C. parapsilosis, and C. glabrata (Supplementary Table 1, Fig. 2). All extracts from these 43 strains (172 in total) were included in the screen. Growth inhibitory activity against all three non-albicans Candida species was detected with comparable sensitivity (Fig. 2a). Based on the relative numbers of active isolates, C. albicans was the most sensitive strain tested (Fig. 2a). The analysis of only JS-EtOAc extracts showed that 50% of the strains isolated from P. rhoeas rhizosphere had the ability to produce antifungal agents (25/50) while in the case of M. chamomilla and U. dioica rhizosphere isolates, this number was lower, roughly one third of the isolated strains (11/33, 7/20, respectively) (Fig. 2b).
Antifungal activity. a Antifungal activity of extracts against Candida albicans and three non-albicans Candida type strains (C. krusei, C. parapsilosis, and C. glabrata). b Distribution of isolates that showed activity against C. albicans from different rhizospheres. c Sensitivity of different Candida strains against active isolates obtained from three different plants
It was shown that the origin of the strains could not directly be correlated to the activity of the derived extracts on tested Candida strains (Fig. 2c). C. albicans was 1.5-fold more sensitive in the case of extracts from P. rhoeas isolates, while C. parapsilosis was more sensitive when extracts from M. chamomilla rhizosphere isolates were used (Fig. 2c).
Given that 50 μg of clinically used antifungal nystatin when loaded onto disc gives the zone of clearance of 10 mm with C. albicans, we have selected strains that showed inhibition zones of 5 mm and higher against C. albicans, no matter if they were grown in JS or R2YE medium or which type of solvent was used for the extract generation. The additional criterion was the ability to inhibit at least three out of four used Candida strains, regardless of inhibition zones diameter. Based on these selection criteria, nine strains were selected for further examination (Supplementary Tables 1 and 2 and Fig. 3). The selected strains originated from all three examined rhizospheres and they showed different spectra of activity upon growth in JS (Fig. 3b) and R2YE media (Fig. 3c).
Selection of Streptomyces strains showing anti-Candida activity. a Morphology of nine selected strains at MSF plates. b Antifungal efficacy of extracts derived from nine selected strains cultivated in JS medium. c Antifungal efficacy of extracts derived from nine selected strains cultivated in R2YE medium. Shaded fields represent turbid zone of Candida growth inhibition and symbol “/” means no inhibition zone
BV283, BV291, BV295, and BV305 were isolated from rhizosphere of P. rhoeas. Antifungal activity of isolate BV283 was detected against all tested Candida spp. in both media, except for the R2YE-MeOH extract, with growth inhibition zones varying from 3 to 13 mm. Strain BV295 had similar properties in a sense that antifungal activity was detected in both media but the growth inhibition zones were smaller (1 to 7 mm). Still, there are few important differences between strains BV283 and BV295. The first difference between these two strains was related to JS-MeOH extract, active only against C. krusei in the case of strain BV295 and the second one is the lack of activity against C. glabrata for both R2YE extract of the BV295 strain. Also, the activity of R2YE-MeOH extracts against three Candida spp. was detected with BV295 strain. The antifungal activity of isolate BV291 was related to the JS-EtOAc extract. It was detected against three of four tested Candida spp. but it was selected for further analysis because of the largest inhibition zone against C. krusei (10 mm). Antifungal compound(s) were produced when isolate BV305 was grown in both media; the moderate growth inhibition zones (2 to 8 mm) against all four Candida strains were detected with EtOAc extracts, while MeOH extracts were active only against C. albicans and C. parapsilosis with growth inhibition zones varying between 2 and 3 mm.
BV340 and BV410 originated from the M. chamomilla rhizosphere. The antifungal activity of isolate BV340 against all tested Candida spp. was mainly related to MeOH extract when the strain was grown in JS medium, with the exception of JS-EtOAc extract active against C. albicans. On the other hand, when isolate BV340 was grown in R2YE medium antifungal activity against three of four used Candida spp. was related to EtOAc extract. Zones of Candida spp. growth inhibition varied from 2 to 6 mm. Strain BV410 produced antifungal compound(s) only in JS medium, successfully extracted only with EtOAc. Clear zones of fungal growth inhibition, varying from 2 to 7 mm, were observed in disk assays against all tested Candida spp. except for C. krusei.
The selected strains originating from the U. dioica rhizosphere (BV221, BV229, and BV231) produced antifungal compound(s) only in JS medium. Clear growth inhibition zones (from 3 to 10 mm) against C. krusei, C. parapsilosis, and C. glabrata were detected with EtOAc and MeOH extracts of both BV229 and BV231.
For nine selected isolates, MIC, MBIC, and MBEC were determined (Table 1). Two methanolic (BV231, BV283) and one ethyl acetate extract (BV410) were active on C. albicans ATCC 10231 in liquid culture, although a wider range of activities was detected in disc assays (Fig. 3b, c). This difference in disc diffusion assay and liquid dilution method when assessing antifungal properties may not be that surprising, as bacterial extracts are complex mixes of structurally diverse compounds that behave differently due to their physical-chemical properties under different conditions. This phenomenon has been reported on numerous occasions when Candida spp. susceptibilities were tested even for the clinically used antifungals (Kostiala and Kostiala 1984; Kumar et al. 2015). The lowest concentration that inhibited C. albicans growth was 8 μg/mL for the EtOAc extract of BV410. This extract also had the ability to inhibit formation of C. albicans biofilm at 125 μg/mL (Table 1). EtOAc extracts of BV283 and BV295 showed strong inhibition of biofilm formation at concentrations of 31 and 62 μg/mL, respectively. On the other hand, only two extracts showed influence on already formed Candida biofilms at the highest tested concentrations (BV305 and BV340).
HPLC analysis of crude extracts
JS-EtOAc and JS-MeOH extracts of nine selected strains were analyzed by analytical HPLC. HPLC chromatograms of JS-EtOAc extracts of nine selected strains were more heterogeneous (Fig. 4) in comparison to corresponding JS-MeOH extracts that showed no or rather small peaks in HPLC chromatograms (data not shown). The chromatograms of the EtOAc extracts derived from BV221, BV229, BV231, BV283, BV295, and BV340 showed high similarity during initial chemical screening although they originated from different plant rhizospheres. All of them showed major peaks at 13.7 min and 15.4 min with the same UV profile. Also, around 22.5 min, there was a group of minor peaks with identical UV profile among these different extracts. The BV291 EtOAc extract had a major peak at 12.3 min, but production of this compound seemed rather low. UV analysis of the major products in the BV305 EtOAc extract showed that the compounds from 14 to 20 min were all highly related to each other, having identical UV profiles. The BV410 EtOAc showed distinctive peaks with UV profile indicating the presence of conjugated double bonds at 15.31 min, 16.37 min, and 19 min.
Phylogenetic relatedness of selected Streptomyces strains
16S rRNA sequencing was used for confirmation that the selected soil isolates belong to the Streptomyces genus. Also, in this way, it was possible to estimate relatedness of selected streptomycetes from rhizospheres of ethno-medicinal plants that showed antifungal activity. A phylogenetic tree was constructed with maximum likelihood statistical method and B. subtilis as an outgroup (Fig. 5). Three distinct branches were observed with BV283, BV295, BV231, BV229, and BV221 grouping in one, BV410 in another and BV291 and BV340 in a separate branch (Fig. 5).
Discussion
Soil represents a natural habitat of many organisms living together and some of them are producers of very useful chemical compounds, including antifungal agents. Past research regarding medicinal plants was focused on the detection of bioactive phytochemicals, but in the last decade, the focus has shifted towards endophytic or rhizosphere associated bacteria, due to the recognition that a significant number of phytotherapeutic compounds are actually produced by associated microbes or through interaction with their host (Hartmann et al. 2008). For example, munumbicins and kakadumycins, both wide-spectrum antibiotics, were confirmed to be produced by Streptomyces spp. associated with Kennedia nigriscans and Grevillea pteridifolia, respectively (Castillo et al. 2003; Castillo et al. 2002).
In this study, we aimed to isolate Actinobacteria, preferably streptomycetes, from rhizosphere soil of common ethno-medicinal plants collected in Serbia (P. rhoeas, M. chamomilla, and U. dioica) as these bacteria are an established source of novel antibiotics. In total, 103 soil sporulating bacteria, presumably streptomycetes, were isolated. The methodology that was used, with appropriate pretreatment and the choice of isolation media that combined selective isolation procedures with specific antibiotics increased the range of isolated Streptomyces strains, when compared to previously used methodology (Djokic et al. 2011). The number and diversity of actinomycetes isolated from P. rhoeas rhizosphere were significantly higher than from other rhizosphere soils. Previous studies have shown that the diversity of actinomycetes in rhizosphere soils is positively correlated to the level of organic matter and depended on the species of plant (Khamna et al. 2009a). The presence of streptomycetes plays an important role in plant rhizospheres because they affect plant growth either by nutrient assimilation or by secondary metabolite production (Khamna et al. 2009b). All obtained isolates grew on MSF media showing morphology typical of streptomycetes (Vos et al. 2009). The color of the substrate mycelium and aerial spore mass varied and can be considered to indicate species diversity of the isolates. This study showed that the white color series of mature sporulated aerial mycelium dominated with 57% of isolated strains, although there are reported examples showing the highest occurrence of streptomycete-like strain of the gray aerial mycelium (Alimuddin et al. 2011).
All obtained Streptomyces isolates were screened for their ability to produce antifungal compounds. We used fundamentally different media (JS and R2YE medium) in order to stimulate isolates to produce a variety of antifungal agents. JS medium was previously proven as a strong stimulator of secondary metabolism in Streptomyces strains; also, medium similar to R2YE was previously successfully used for the production of antimicrobial compounds by streptomycetes (Ilic et al. 2005; Stankovic et al. 2013). In addition, two solvents (ethyl acetate and methanol) were used to maximize the chance of isolation and detection of potential active compounds. Out of 412 different extracts, 88 (21%) were active against C. albicans in disc diffusion assays. These extracts originated from 43 different isolates, making the percentage of active isolates 42%. This percentage was higher in comparison with studies of the antifungal activity of streptomycetes isolated from rhizosphere soil of date palm in Tunis (26%) or those isolated from the rhizosphere of sixteen medicinal plants collected in Lamphun, Thailand (7%), but on the other hand, it was in accordance with previously obtained data referring to streptomycetes isolated from the soils of southeastern Serbian region, with 45% isolates showing antifungal potential with a total of 20 isolates screened (Fguira et al. 2012; Ilic et al. 2005; Khamna et al. 2009b). Streptomycetes with their nutrient cycling, antifungal activity, and ability to thrive in harsh conditions likely play a role in stimulating plant growth, increasing yield and reducing pathogens as well as biotic or abiotic stresses (Jog et al. 2014).
We found that JS medium is better in triggering the production of antifungals in comparison to R2YE. This observation corroborates observations from the previous studies that found that culture medium is a key factor in secondary metabolite biosynthesis in different Actinomyces genera (Bode et al. 2002). It is line with so-called OSMAC (one strain ± many compounds) approach where nature’s chemical diversity is revealed by systematic alteration of easily accessible cultivation parameters (for example, media composition) in order to increase the number of secondary metabolites available from one microbial source. Using this approach, it was shown that when Streptomyces sp. (strain A1), primarily characterized as a producer of rubromycins, was grown in a soybean meal/mannitol medium, it produced streptazoline and streptazones A and B1, while no rubromycins could be detected (Bode et al. 2002). Regardless of the cultivation medium, we obtained more active extracts after whole culture extraction with EtOAc in comparison to MeOH extraction of residual mycelium. When the biosynthetic capacity of 146 marine Salinispora and Streptomyces strains differently grown and extracted was analyzed, the significance of the choice of the extraction method was clearly demonstrated (Crusemann et al. 2017). Extraction was carried out sequentially with three solvents of increasing polarity (EtOAc, n-butanol, and MeOH), which enabled the capture of a common core metabolome, but solvent-specific metabolites were also discovered. These results support our finding that usages of different extraction solvents will greatly enhance the molecular diversity beyond the antifungal activity detected.
The spectrum of candidiasis has changed with the emergence of non-albicans Candida spp. and their antifungal resistance. Several published reports agree that Candida tropicalis is the most common isolate (48%), followed by C. parapsilosis (27.4%), C. albicans (22.5%), C. krusei (0.88%), and C. glabrata (0.88%) (Capoor et al. 2005). In this study, three non-albicans species were also used as test microorganisms. Among the strains that already showed antifungal activity against C. albicans, 46.5% were (20/43) also active against C. glabrata, the most sensitive tested non-albicans species, whereas these percentages for C. krusei and C. parapsilosis were 35% (15/43), and 30% (13/43), respectively. The potent inhibitory activity against C. albicans grown in liquid culture was detected for two analyzed crude extracts (283 JS-MeOH and 410 JS-EtOAc) with MIC values of 32 and 8 μg/mL, respectively. These prominent antifungal activities of crude extracts, complex mixtures of various compounds, imply that purified compound(s) would have even better antifungal potency. There are examples in the literature where crude extracts or purified antifungal compound(s) from different soil Streptomyces spp. had strong inhibitory effect against C. albicans, with MIC values comparable to well-known antifungal drugs amphotericin B or fluconazole (Cordova-Davalos et al. 2017; Vartak et al. 2014). We also detected prominent ability to impair C. albicans biofilm formation or to eradicate preformed Candida biofilms in vitro for several crude cell extracts. The best biofilm inhibition activity was related to both EtOAc and MeOH extract of BV283 isolate grown in JS medium. Similarly, it was previously shown that among forty Streptomyces spp. isolated from different soil samples in Egypt, only one had high biofilm inhibition activity (Sheir and Hafez 2017). Also, among sixty randomly selected Streptomyces spp. from Germplasm Bank of Actinomycetes one isolate, Streptomyces sp. GCAL-25 had a strong effect on C. albicans biofilm formation (Cordova-Davalos et al. 2017). All these findings confirm the importance of soil isolates as a source of prominent antifungal metabolites active against C. albicans biofilms, one of its most important virulent factors.
Our results of the HPLC analyses are consistent with our phylogenetic analysis, since extracts with similar HPLC profiles showed close relatedness between 16S rRNA sequences. By contrast, BV305 and BV410 are distant from other isolated strains consequently resulting in unique metabolic profiles during the HPLC analyses. Phylogenetic analysis also confirmed close relationships among isolates that originate from U. dioica rhizosphere: Streptomyces sp. BV221, Streptomyces sp. BV229, Streptomyces sp. BV231. Those strains showed high similarity with Streptomyces sp. BV283 and Streptomyces sp. BV295 that originate from P. rhoeas rhizosphere. Notably, Streptomyces sp. BV283 although similar to BV295, BV221, BV229, and BV231 as judged by HPLC “fingerprint” and phylogenetic analysis (Figs. 4 and 5) showed stronger bioactivity properties (Fig. 3 and Table 1) which may not be surprising considering the potential number of gene clusters responsible for bioactive compounds synthesis that each Streptomyces spp. can bear (Ikeda et al. 2003). Another group of phylogenetically similar streptomycetes is composed of Streptomyces sp. BV305 and Streptomyces sp. BV291 (originate from P. rhoeas rhizosphere) and Streptomyces sp. BV340 (originate from U. dioica). On the other hand, Streptomyces sp. BV410 showed a lack of close relations with other selected isolates, but it is phylogenetically close to Streptomyces europaeiscabiei.
In conclusion, Streptomyces strains isolated and identified within this study are capable of producing a diversity of specialized metabolites with pronounced antifungal activity, highlighting that rhizosphere soil of ethno-medicinal plants is a prolific source of metabolically talented streptomycetes—producers of potentially new antifungal compounds. In-depth studies on the chemical constituents of the strains that produced the most promising antifungal activity profiles are currently ongoing in our laboratories.
References
Alimuddin A, Widada J, Asmara W, Mustofa M (2011) Antifungal production of a strain of Actinomycetes spp isolated from the rhizosphere of cajuput plant: selection and detection of exhibiting activity against tested fungi. I J Biotech 16:1–10. https://doi.org/10.22146/ijbiotech.7829
Altschul SF, Madden TL, Schäffer AA, Zhang J, Zhang Z, Miller W, Lipman DJ (1997) Gapped BLAST and PSI-BLAST: a new generation of protein database search programs. Nucleic Acids Res 25:3389–3402
Bode HB, Bethe B, Hofs R, Zeeck A (2002) Big effects from small changes: possible ways to explore nature’s chemical diversity. Chembiochem 3:619–627. https://doi.org/10.1002/1439-7633(20020703)3:7<619::AID-CBIC619>3.0.CO;2-9
Capoor MR, Nair D, Deb M, Verma PK, Srivastava L, Aggarwal P (2005) Emergence of non-albicans Candida species and antifungal resistance in a tertiary care hospital. Jpn J Infect Dis 58:344–348
Castillo U, Harper J, Strobel G, Sears J, Alesi K, Ford E, Lin J, Hunter M, Maranta M, Ge H, Yaver D, Jensen J, Porter H, Robison R, Millar D, Hess W, Condron M, Teplow D (2003) Kakadumycins, novel antibiotics from Streptomyces sp NRRL 30566, an endophyte of Grevillea pteridifolia. FEMS Microbiol Lett 29:183–190. https://doi.org/10.1016/S0378-1097(03)00426-9
Castillo U, Strobel G, Ford E, Hess W, Porter H, Jensen J, Albert H, Robison R, Condron M, Teplow D, Stevens D, Yaver D (2002) Munumbicins, wide-spectrum antibiotics produced by Streptomyces NRRL 30562, endophytic on Kennedia nigriscans. Microbiology 148:2675–2685. https://doi.org/10.1099/00221287-148-9-2675
Challis GL, Hopwood DA (2003) Synergy and contingency as driving forces for the evolution of multiple secondary metabolite production by Streptomyces species. Proc Natl Acad Sci U S A 100(Suppl 2):14555–14561. https://doi.org/10.1073/pnas.1934677100
Clinical and Laboratory Standards Institute (2008) Reference method for broth dilution antifungal susceptibility testing of yeasts—third edition: approved standard M27-A3. CLSI W, PA, USA
Clinical and Laboratory Standards Institute. (2012) Reference method for broth dilution antifungal susceptibility testing of yeasts: Fourth informational supplement M27-S4. CLSI W, PA, USA.
Cordova-Davalos LE, Escobedo-Chavezávez KG, Evangelista-Martínez Z (2017) Inhibition of Candida albicans cell growth and biofilm formation by a bioactive extract produced by soil Streptomyces strain GCAL-25. Arch Biol Sci 70:387–396. https://doi.org/10.2298/ABS170908057C
Crusemann M, O’Neill EC, Larson CB, Melnik AV, Floros DJ, da Silva RR, Jensen PR, Dorrestein PC, Moore BS (2017) Prioritizing natural product diversity in a collection of 146 bacterial strains based on growth and extraction protocols. J Nat Prod 80:588–597. https://doi.org/10.1021/acs.jnatprod.6b00722
Djokic L, Narancic T, Nikodinovic-Runic J, Savic M, Vasiljevic B (2011) Isolation and characterization of four novel Gram-positive bacteria associated with the rhizosphere of two endemorelict plants capable of degrading a broad range of aromatic substrates. Appl Microbiol Biotechnol 91:1227–1238. https://doi.org/10.1007/s00253-011-3426-9
Fguira L, Bejar S, Mellouli L (2012) Isolation and screening of Streptomyces from soil of Tunisian oases ecosystem for nonpolyenic antifungal metabolites. Afr J Biotechnol 11:7512–7519. https://doi.org/10.5897/AJB11.2180
Genilloud O (2017) Actinomycetes: still a source of novel antibiotics. Nat Prod Rep 34:1203–1232. https://doi.org/10.1039/c7np00026j
Golinska P, Wypij M, Agarkar G, Rathod D, Dahm H, Rai M (2015) Endophytic actinobacteria of medicinal plants: diversity and bioactivity. Antonie van Leeuwenhoek 108:267–289. https://doi.org/10.1007/s10482-015-0502-7
Hamid ME, Assiry MM, Joseph MR, Haimour WO, Abdelrahim IM, Al-Abed F, Fadul AN, Al-Hakami AM (2014) Candida and other yeasts of clinical importance in Aseer region, southern Saudi Arabia. Presentation of isolates from the routine laboratory setting. Saudi Med J 35:1210–1214
Hartmann A, Rothballer M, Schmid M (2008) Lorenz Hiltner, a pioneer in rhizosphere microbial ecology and soil bacteriology research. Plant Soil 312:7–14. https://doi.org/10.1007/s11104-007-9514-z
Ikeda H, Ishikawa J, Hanamoto A, Shinose M, Kikuchi H, Shiba T, Sakaki Y, Hattori M, Ōmura S (2003) Complete genome sequence and comparative analysis of the industrial microorganism Streptomyces avermitilis. Nat Biotech 21:526–531. https://doi.org/10.1038/nbt820
Ilic-Tomic T, Genčić MS, Živković MZ, Vasiljevic B, Djokic L, Nikodinovic-Runic J, Radulovic NS (2015) Structural diversity and possible functional roles of free fatty acids of the novel soil isolate Streptomyces sp. NP10. Appl Microbiol Biotechnol 99:4815–4833
Ilic S, Konstantinovic S, Todorovic Z (2005) UV/VIS analysis and antimicrobial activity of Streptomyces isolates. F U Med Biol 12:44–46
Jog R, Pandya M, Nareshkumar G, Rajkumar S (2014) Mechanism of phosphate solubilization and antifungal activity of Streptomyces spp. isolated from wheat roots and rhizosphere and their application in improving plant growth. Microbiology 160:778–788. https://doi.org/10.1099/mic.0.074146-0
Khamna S, Yokota A, Lumyong S (2009a) Actinomycetes isolated from medicinal plant rhizosphere soils: diversity and screening of antifungal compounds, indole-3-acetic acid and siderophore production. World J Microbiol Biotechnol 25:649–655. https://doi.org/10.1007/s11274-008-9933-x
Khamna S, Yokota A, Peberdy J, Lumyong S (2009b) Antifungal activity of Streptomyces spp. isolated from rhizosphere of Thai medicinal plants. Int J Integr Biol 6:143–147 http://cmuir.cmu.ac.th/jspui/handle/6653943832/5775
Kieser T, Bibb MJ, Buttner MJ, Chater KF, Hopwood DA (2000) Practical Streptomyces Genetics. John Innes Foundation, Norwich, UK.
Kostiala AAI, Kostiala I (1984) Broth dilution and disc diffusion methods in the susceptibility testing of pathogenic Candida albicans against four antimycotics. Mycopathologia 87(1–2):121–127. https://doi.org/10.1007/BF00436640
Köberl M, Schmidt R, Ramadan E, Bauer R, Berg G (2013) The microbiome of medicinal plants: diversity and importance for plant growth, quality and health. Front Microbiol 4:400. https://doi.org/10.3389/fmicb.2013.00400
Kumar D, Bhattacharyya S, Gupta P, Banerjee G, Singh M (2015) Comparative analysis of disc diffusion and E-test with broth micro-dilution for susceptibility testing of clinical Candida isolates against amphotericin B, fluconazole, voriconazole and caspofungin. J Clin Diagn Res 9(11):DC01–DC04
Kumar S, Stecher G, Tamura K (2016) MEGA7: Molecular evolutionary genetics analysis version 7.0 for bigger datasets. Mol Biol Evol 33:1870–1874. https://doi.org/10.1093/molbev/msw054
Larkin M, Blackshields G, Brown N, Chenna R, McGettigan P, McWilliam H, Valentin F, Wallace I, Wilm A, Lopez R, Thompson J, Gibson T, Higgins DG (2007) Clustal W and Clustal X version 2.0. Bioinformatics 23:2947–2948. https://doi.org/10.1093/bioinformatics/btm404
Maleki H, Dehnad A, Hanifian S, Khani S (2013) Isolation and molecula identification of Streptomyces spp. with antibacterial activity from Northwest of Iran. Bioimpacts 3:129–134. https://doi.org/10.5681/bi.2013.017
Mellouli L, Ben Ameur-Mehdi R, Sioud S, Salem M, Bejar S (2003) Isolation, purification and partial characterization of antibacterial activities produced by a newly isolated Streptomyces sp. US24 strain. Res Microbiol 154:345–352. https://doi.org/10.1016/s0923-2508(03)00077-9
Nikodinovic J, Barrow KD, Chuck JA (2003) High yield preparation of genomic DNA from Streptomyces. BioTechniques 35:932–936
Pierce CG, Uppuluri P, Tristan AR, Wormley FL Jr, Mowat E, Ramage G, Lopez-Ribot JL (2008) A simple and reproducible 96-well plate-based method for the formation of fungal biofilms and its application to antifungal susceptibility testing. Nat Protoc 3:1494–1500. https://doi.org/10.1038/nprot.2008.141
Reysenbach AL, Wickham GS, Pace NR (1994) Phylogenetic analysis of the hyperthermophilic pink filament community in Octopus Spring, Yellowstone National Park. Appl Environ Microbiol 60:2113–2119
Rios JL, Recio MC (2005) Medicinal plants and antimicrobial activity. J Ethnopharmacol 100:80–84. https://doi.org/10.1016/j.jep.2005.04.025
Schrey SD, Erkenbrack E, Früh E, Fengler S, Hommel K, Horlacher N, Schulz D, Ecke M, Kulik A, Fiedler H-P, Hampp R, Tarkka MT (2012) Production of fungal and bacterial growth modulating secondary metabolites is widespread among mycorrhiza-associated streptomycetes. BMC Microbiol 12:164–164. https://doi.org/10.1186/1471-2180-12-164
Sheir DH, Hafez MA (2017) Antibiofilm activity of Streptomyces toxytricini Fz94 against Candida albicans ATCC 10231. Microbial Biosystems 2:26–39. https://doi.org/10.21608/mb.2017.5255
Spasic J, Mandic M, Radivojevic J, Jeremic S, Vasiljevic B, Nikodinovic-Runic J, Djokic L (2018) Biocatalytic potential of Streptomyces spp. isolates from rhizosphere of plants and mycorrhizosphere of fungi. Biotechnol Appl Biochem. https://doi.org/10.1002/bab.1664
Stamenov D, Djuric S, Hajnal Jafari T, Ćirić V, Manojlovic M (2018) Microbiological activity in the soil of various agricultural crops in organic production. Contemp Agr 67:34–39. https://doi.org/10.2478/contagri-2018-0005
Stankovic N, Senerovic L, Bojic-Trbojevic Z, Vuckovic I, Vicovac L, Vasiljevic B, Nikodinovic-Runic J (2013) Didehydroroflamycoin pentaene macrolide family from Streptomyces durmitorensis MS405T: production optimization and antimicrobial activity. J Appl Microbiol 115:1297–1306. https://doi.org/10.1111/jam.12326
Thakur D, Yadav A, Gogoi BK, Bora TC (2007) Isolation and screening of Streptomyces in soil of protected forest areas from the states of Assam and Tripura, India, for antimicrobial metabolites. J Myc Méd 17:242–249. https://doi.org/10.1016/j.mycmed.2007.08.001
Vartak A, Mutalik V, Parab RR, Shanbhag P, Bhave S, Mishra PD, Mahajan GB (2014) Isolation of a new broad spectrum antifungal polyene from Streptomyces sp. MTCC 5680. Lett Appl Microbiol 58:591–596. https://doi.org/10.1111/lam.12229
Vicente MF, Basilio A, Cabello A, Peláez F (2003) Microbial natural products as a source of antifungals. Clin Microbiol Infect 9:15–32. https://doi.org/10.1046/j.1469-0691.2003.00489.x
Vos P, Garrity G, Jones D, Krieg NR, Ludwig W, Rainey FA, Schleifer K-H, Whitman W (2009) Bergey’s Manual of Systematic Bacteriology. Volume 3: The Firmicutes. Second edition. Springer-Verlag New York, New York, USA.
Waksman SA, Henrici AT (1943) The nomenclature and classification of the Actinomycetes. J Bacteriol 46:337–341
Williams ST, Mayfield CI (1971) Studies on the ecology of actinomycetes in soil III. The behaviour of neutrophilic streptomycetes in acid soil. Soil Biol Biochem 3:197–208. https://doi.org/10.1016/0038-0717(71)90015-0
Acknowledgements
PM D’Agostino would like to thank the TUM University Foundation Fellowship for funding. TAM Gulder thanks the DFG for further funding (GU 1233/1-1 and CIPSM).
Funding
This work was supported by the Ministry of Education, Science and Technological Development of Serbia (Grant No 173048) and the DAAD (Deutscher Akademischer Austauschdienst, Bilateral Project with Republic of Serbia to J Nikodinović-Runic and TAM Gulder – 2016/2017).
Author information
Authors and Affiliations
Corresponding author
Ethics declarations
This manuscript does not contain human studies or experiments using animals.
Conflict of interest
The authors declare that they have no conflict of interest.
Additional information
Publisher’s note
Springer Nature remains neutral with regard to jurisdictional claims in published maps and institutional affiliations.
Electronic supplementary material
ESM 1
(DOCX 33 kb)
Rights and permissions
About this article
Cite this article
Mojicevic, M., D’Agostino, P.M., Nikodinovic-Runic, J. et al. Antifungal potential of bacterial rhizosphere isolates associated with three ethno-medicinal plants (poppy, chamomile, and nettle). Int Microbiol 22, 343–353 (2019). https://doi.org/10.1007/s10123-019-00054-8
Received:
Revised:
Accepted:
Published:
Issue Date:
DOI: https://doi.org/10.1007/s10123-019-00054-8