Abstract
Berberine (BBR) administration is effective in the management of diabetic nephropathy, but the full mechanism of action has not been comprehensively established. This study investigated the effect of oral administration of BBR on fasting blood glucose (FBG) level, renal function (serum uric acid, urea, and creatinine) markers, arginase and adenosine deaminase (ADA) activities, and endogenous antioxidant level in streptozotocin (STZ)-induced diabetic nephropathy of rats. Non-diabetic and diabetic (induced with 50 mg/kg body weight (bwt) of STZ) rats were treated with 50 and 100 mg/kg bwt of BBR for 14 days. Thereafter, the rats were sacrificed and the kidney was isolated and homogenized. The supernatant was used for the determination of ADA and arginase activities, nitric oxide (NO), and malonylaldehyde (MDA) levels. Antioxidant status of the kidney (superoxide dismutase (SOD), glutathione peroxidase (GPx) activities, and glutathione (GSH) level) was determined. The result indicated that FBG level, as well as serum biomarkers of kidney function, was drastically reduced in diabetic rats treated with BBR when compared to the untreated diabetic rats. Induction of diabetes led to an increase in ADA and arginase activities, and MDA level, with a concomitant decrease of NO level and antioxidant status, compared with the normal control rats. However, treatment with BBR (50 and 100 mg/kg) reversed these effects. In conclusion, oral administration of BBR decreased FBG, ADA and arginase activities, and MDA level, and increased NO and antioxidant in diabetic rats.
Similar content being viewed by others
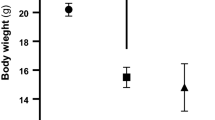
Avoid common mistakes on your manuscript.
Introduction
Diabetic nephropathy (DN) is one of the severe chronic complications of diabetes mellitus (DM) (Warren et al. 2019). It is characterized by proteinuria, mesangial expansion, glomerular basement membrane (GBM) thickening, persistent fibrosis, and a gradual decrease in renal function, which result from increased extracellular matrix (ECM) deposition at the glomerular level (Kato and Natarajan 2014). Several studies have related the development of kidney damage with the increased activities of arginase and adenosine deaminase (ADA), as well as increased oxidative stress (Ni et al. 2015; Akinyemi et al. 2014, 2017; Ademiluyi et al. 2014). Although persistent hyperglycemia had been linked to DN (Ni et al. 2015), no study had investigated the activities of arginase and ADA in the DN model.
According to the reports of Bauerle et al. (2011) and Akinyemi et al. (2017), adenosine, which is an endogenous signalling molecule, has been implicated in kidney function. Its production involves enzymatic phosphohydrolysis of ATP and/or AMP. Adenosine is involved in the regulation of glucose homeostasis by improving insulin secretion and also regulating β-cell homeostasis and activity (Antonioli et al. 2015). However, study indicates that the functionality of adenosine could be hampered by its reduction via the activity of adenosine deaminase (ADA), an enzyme that is present in the kidney, and catalyses the irreversible hydrolytic deamination of adenosine to inosine and 2-deoxyadenosine to 2-deoxyinosine, thereby depleting the concentration and functionality of adenosine (Akinyemi et al. 2017). Hence, an attempt to inhibit the activity of ADA has been proposed as a therapeutic approach for the prevention of kidney dysfunction in diabetic patients (Quezada et al. 2013; Antonioli et al. 2015).
Nitric oxide (NO) is an active endogenous vasodilator, involved in the regulation of renal function (Oboh et al. 2017). It is produced from catalytic activity of nitric oxide synthase (NOS), using ʟ-arginine as substrate (Oboh et al. 2018). Arginase also competes for ʟ-arginine with NOS to produce urea and ʟ-ornithine, thereby, reducing the production of NO (Oboh et al. 2018). According to the report of You et al. (2015), arginase activity increases during diabetic conditions, but, its inhibition has also been reported to have a therapeutic effect on DN. Studies have also shown that NO level decreases under oxidative stress, a major factor in the pathophysiology of diabetes (Rehman and Akash 2017; Oboh et al. 2018). Free radicals such as superoxide radical (O2−) can interact with NO to form peroxynitrite (ONOO), thereby depleting NO bioavailability (Oboh et al. 2016). Therefore, any attempt to alleviate oxidative stress or boost endogenous antioxidant levels could also be a good therapeutic strategy towards the management of DM and DN (Kashihara et al. 2010).
Berberine (BBR) is an isoquinoline alkaloid obtained from numerous herbal plants such as Coptis chinensis (Coptis or Goldthread), Hydrastis canadensis (goldenseal), Berberis aquifolium (Oregon grape), Berberis aristata (Tree Turmeric), Berberis vulgaris (Barberry), and Arcangelisia flava (Singh and Mahajan 2013). BBR is widely known for its anti-oxidative, anti-apoptotic, antidiabetic, anticancer, and anti-inflammatory and cognitive potentials (Ni et al. 2015; Chen et al. 2016; de Oliveira et al. 2016). Numerous studies have documented the protective roles of BBR and its possible mechanism of actions towards the management of DN (Ni et al. 2015; Kumaş et al. 2019; Chen et al. 2016; de Oliveira et al. 2016), but none has ever reported the effect BBR treatment on the activities of ADA and arginase as well as NO level in the kidney of diabetic rats. This study focused on elucidating the therapeutic mechanisms of BBR treatment in the amelioration of DN by investigating the effect of oral BBR administration on the activities of ADA and arginase, as well as NO level in the kidney homogenate of STZ-induced diabetic rats in an experimental model that evaluated hyperglycemia, oxidative stress, and renal dysfunction in relation to nephropathy.
Materials and methods
Chemicals
5,5′-dithio-bis-2-nitrobenzoic acid (DTNB), Tris buffer, reduced glutathione, adenosine, ʟ-arginine, Coomassie brilliant Blue G, streptozotocin (STZ), and berberine hydrochloride were obtained from Sigma-Aldrich (St. Louis, MO, USA). Acarbose was purchased from Glenmark Generics (Europe) pharmaceutical limited. Thiobarbituric acid (TBA) and trichloroacetic acid (TCA) were procured from Sigma-Aldrich, Inc. (St Louis, MO, USA). Sodium carbonate, ammonium sulphate, sodium nitroprusside, potassium acetate, sodium dodecyl sulphate (SDS), iron (II) sulphate and disodium hydrogen phosphate, and sodium dihydrogen phosphate were sourced from BDH Chemicals Ltd. (Poole, England).
Experimental animals
Fifty-six adult male rats weighing between 200 and 230 g were obtained from the animal house of the Faculty of Veterinary Medicine, University of Ibadan. After 2 weeks of acclimatization, they were randomly divided into 7 groups (n = 8). The animals were kept in wire mesh cages under a controlled light cycle (12-h light/12-h dark); they were fed with commercial rat chow with free access to water ad libitum. All institutional and national guidelines for the care and use of laboratory animals were followed. All the experimental animals received humane care, according to the criteria outlined in the Guide for the Care and Use of Laboratory Animals (National Institutes of Health 2011).
Induction of diabetes
The rats (n = 48) were made diabetic via intraperitoneal (i.p.) injection of a single dose (50 mg/kg bwt) of freshly prepared STZ (10 mg/0.2 ml) in citrate buffer (0.1 M, pH 4.5). The dose was reported by Parisa and Siamak (2011) and Kumaş et al. (2019). After 72 h, blood glucose test was conducted, and rats with blood glucose 250 mg/dl were considered diabetic and were used for this study. The ethical regulations are in accordance with national and institutional guidelines for the protection of animal welfare during experiments.
Experimental design
The fifty-six rats (48 diabetes and 8 normal) were, thereafter, divided randomly into 7 treatment groups (n = 8).
-
Group I: Normal control rats received citrate buffer
-
Group II: Normal rats treated with 50 mg/kg BBR (10 mg/0.2 ml) through oral gavage
-
Group III: Normal rats treated with 100 mg/kg BBR (20 mg/0.2 ml) through oral gavage.
-
Group IV: Untreated diabetic rats
-
Group V: Diabetic rats treated with 50 mg/kg of BBR (10 mg/0.2 ml) through oral gavage
-
Group VI: Diabetic rats treated with 100 mg/kg of BBR (20 mg/0.2 ml) through oral gavage
-
Group VII: Diabetic rats treated with 25 mg/kg of acarbose (ACA, 5 mg/0.2 ml) through oral gavage
The normal rats were orally treated daily for 14 days with citrate buffer (1 ml/kg bwt of rats) in group I (normal control) and BBR at 50 and 100 mg/ml in group II and group III (treated control) respectively. The diabetic rats were not treated in group IV (negative diabetic control). Group V and VI were diabetic rats treated with 50 and 100 mg/kg of BBR, respectively, to assess the treatment of BBR effect (de Oliveira et al. 2016). Group VII served as positive control and was orally treated with acarbose, which is a standard hypoglycemic drug, having inhibitory effects on α-amylase and α-glucosidase activity (Adefegha et al. 2021a, b). Fasting blood glucose (FBG) levels were monitored on days 1, 4, 7, 10, and 14 during the treatment. At the end of the treatment, on day 15, the rats were anaesthetized with isofluorane, and thorax was opened up to collect the blood via cardiac puncture into the plain bottle. The kidney was isolated, transferred to a clean glass plate on ice, and washed with buffer. The right kidney was and used for the biochemical assay, while the left kidney was fixed in 10% formalin for the histological examination.
Sample collection
The cardiac blood collected was allowed to clot and serum was harvested, by centrifuging for 10 min at 5000 × g. Right kidney collected was weighed, rinsed with cold saline, and homogenized with five volumes of 0.1 M of phosphate buffer (pH 7.4) (Oboh et al. 2018). The homogenate was subsequently centrifuged at 5000 × g for 10 min to collect the supernatant that was used for the determination of biochemical assays (Oboh et al. 2020).
Biochemical assays
Determination of fasting blood glucose
Tail vein blood, collected after sniping the tail tip, was used in the estimation of FBG with an auto-coding glucose metre and test strip containing glucose oxidase (Finetest, Infopia, South Korea).
Determination of total protein content
The protein content of the supernatant from kidney homogenate was measured by the method of Bradford (1976) using bovine serum albumin (BSA) as standard.
Determination of serum urea, creatinine, and uric acid levels
Serum urea, creatinine, and uric acid levels were estimated by using commercially available kits from RANDOX Laboratories Ltd., Crumlin, County Antrim, UK, and the procedures stated therein were strictly followed.
Determination of malondialdehyde (MDA) level
The MDA level in the supernatant from the kidney homogenate was determined by the method described by Ohkawa et al. (1979) with slight modifications. The reaction mixture contained 200 μl of the homogenate or standard (MDA 0.03 mM), 200 μl of 8.1% sodium dodecyl sulphate (SDS), 750 μl of acetic acid solution (2.5 M HCl, pH 3.5), and 750 μl of 0.8% TBA was incubated at 100 °C for 60 min. The absorbance was measured at 532 nm and the MDA level was expressed as nmol MDA/mg protein.
Determination of superoxide dismutase and glutathione peroxidase activities, and glutathione (GSH) level
Superoxide dismutase (SOD) activity was determined by the method of Alía et al. (2003) using adrenaline (0.06 mg/ml) as substrate. Glutathione peroxidase (GPx) activity was assayed by the method of Paglia and Valentine (1967), while the glutathione (GSH) level was determined using Ellman’s reagent (19.8 mg of 5,5′ dithiobisnitrobenzoic acid in 100 ml of 0.1% sodium citrate) (Ellman 1959).
Determination of adenosine deaminase activity
ADA activity was measured in kidney homogenate using the method of Giusti and Galanti (1984), as described by Olasehinde et al. (2020), where 50 μL of kidney homogenate was added to 21 mM of the substrate (adenosine, pH 6.5) and incubated at 37 °C for 1 h. Absorbance was read at 620 nm.
Determination of arginase activity
Kaysen and Strecker (1973) method as reported by Dada et al. (2020) was adopted for the determination of arginase activity in the kidney homogenate of normal and STZ-induced diabetic rats, using 50 mM ʟ-arginine as the substrate to quantify the produced urea intensity at 450 nm.
Determination of nitric oxide level
Briefly, the mixture of kidney homogenate (150 μL), distilled water (450 μL), and Griess reagent (600 μL) was incubated at room temperature for 10 min and the absorbance was read at 540 nm. The concentration of nitrite in the supernatant was determined from the sodium nitrite standard curve and expressed as μmol/mg protein (Green et al. 1982).
Histopathological studies
The kidney that was fixed in formalin was processed for histological sectioning (Avwioro 2002). The thin section (5 microns) of the tissue obtained was mounted on glass slides and stained with haematoxylin and eosin (H&E). Thereafter, the slides were observed in the optical microscope to evaluate possible damage in the kidney tissue.
Statistical analysis
GraphPad (version 5.0) prism was used for statistical analysis of the experimental results. The data were summarized as mean ± standard error of the mean (SEM). The variation in mean value was analysed for significance level using one-way ANOVA with Tukey post hoc test. Level of significance was accepted at p < 0.05, p < 0.01, p < 0.001.
Results
Effect of oral administration of berberine on fasting blood glucose level of normal and diabetic rats
Increased level of fasting blood glucose (FBG) was observed in untreated diabetic rats (p < 0.05) relative to the normal control (Fig. 1). The FBG level of normal rats treated with 50 and 100 mg/kg BBR, respectively, ranged between 62 and 69 mg/dl, which was significantly lower (p < 0.05) when compared to normal control rats (80 and 84 mg/dl). Reduced FBG level was observed in diabetic rats treated with ACA (278.0 to 88 mg/dl) at the end of the experiment. That of the diabetic rats treated with 50 mg/kg of BBR had 124 mg/dl, while that of 100 mg/kg BBR treated diabetic rats was reduced from 291.0 to 102.2 mg/dl at the end of the experimental period. Both FBG levels of the normal rats treated with 50 mg/kg BBR and that of the 100 mg/kg BBR treated normal rats were not different (p > 0.05) when compared, but were lower (p < 0.05) relative to the FBG level of the normal control rats. Interestingly, the FBG level of diabetic rats treated with ACA was not different (p > 0.05) compared to the FBG level of normal control rats at the end of the experimental period.
Blood glucose level of normal and STZ-induced diabetic rats treated with oral administration of berberine (BBR). Key: Group I: Normal control rats received citrate buffer. Group II: Normal rats treated with 50 mg/kg BBR through oral gavage. Group III: Normal rats treated with 100 mg/kg BBR through oral gavage. Group IV: Untreated 50 mg/kg STZ-induced diabetic rats. Group V: Diabetic rats treated with 50 mg/kg of BBR through oral gavage. Group VI: Diabetic rats treated with 100 mg/kg of BBR through oral gavage. Group VII: Diabetic rats treated with 25 mg/kg of acarbose (ACA) through oral gavage
The effect of oral administration of berberine on serum urea, creatinine, and uric acid levels in the diabetic rats
Figure 2A−C reveal the levels of urea, creatinine, and uric acid, respectively, in the serum of STZ-induced diabetes in rats. On the other hand, diabetic rats treated with BBR (50 and 100 mg/kg) and ACA (25 mg/kg) reversed these effects.
Effect of oral administration of berberine (BBR) on urea (A), creatinine (B), and uric acid (C) levels in the serum of normal and STZ-induced diabetic rats. Bars represent mean ± SEM (n = 8). Values are statistically different at **p < 0.001, *p < 0.05 versus normal control rats; ##p < 0.01, #p < 0.05 versus STZ-induced diabetic rats. Key: Group I: Normal control rats received citrate buffer. Group II: Normal rats treated with 50 mg/kg BBR through oral gavage. Group III: Normal rats treated with 100 mg/kg BBR through oral gavage. Group IV: Untreated 50 mg/kg STZ-induced diabetic rats. Group V: Diabetic rats treated with 50 mg/kg of BBR through oral gavage. Group VI: Diabetic rats treated with 100 mg/kg of BBR through oral gavage. Group VII: Diabetic rats treated with 25 mg/kg of acarbose (ACA) through oral gavage
The effect of berberine on MDA level, SOD and GPx activities, and GSH level in the kidney of diabetic rats
In Fig. 3A, MDA level was increased in the kidney homogenate in untreated diabetic rats (p < 0.01) when compared with the normal control rats. In normal rats, administration of BBR (50 and 100 mg/kg) inhibited MDA production but was not different from that of normal control rats. As evidenced in Fig. 3B−D, the diabetic rats had a significant (p < 0.01) decrease in the activities of SOD and GPx, and GSH level, when compared with the control rats. Upon treatment with BBR (50 and 100 mg/kg) and ACA (25 mg/kg), activities of antioxidant enzymes, and level of GSH were increased with varying significant differences in comparison with untreated diabetic rats.
Effect of oral administration of berberine (BBR) on malonylaldehyde (MDA) level (A), superoxide dismutase activity (B), glutathione peroxidase (GPx) activity (C), and glutathione (GSH) level (D) in the kidney homogenate of normal and STZ-induced diabetic rats. Bars represent mean ± SEM (n = 8). Values are statistically different at ***p < 0.001, **p < 0.01, *p < 0.05 versus normal control rats; ###p < 0.01, ##p < 0.01, #p < 0.05 versus STZ-induced diabetic rats. Key: Group I: Normal control rats received citrate buffer. Group II: Normal rats treated with 50 mg/kg BBR through oral gavage. Group III: Normal rats treated with 100 mg/kg BBR through oral gavage. Group IV: Untreated 50 mg/kg STZ-induced diabetic rats. Group V: Diabetic rats treated with 50 mg/kg of BBR through oral gavage. Group VI: Diabetic rats treated with 100 mg/kg of BBR through oral gavage. Group VII: Diabetic rats treated with 25 mg/kg of acarbose (ACA) through oral gavage
The effect of berberine on ADA and arginase activities, and NO level in the kidney of diabetic rats
Figure 4A depicts the effect of BBR on ADA activity. Untreated diabetic rats have increased ADA activity compared to normal control. The normal rats treated with 50 mg/kg BBR as well as 100 mg/kg BBR treated normal rats had reduced ADA activity compared to normal control rats. However, there was a significant (p < 0.05) reduction in ADA activity of 100 mg/kg BBR-treated normal rats when compared to the normal control rats. Diabetic rats treated with BBR (50 and 100 mg/kg bwt) and acarbose had reduced ADA activity, but diabetic rats treated with 100 mg/kg bwt had the highest (p < 0.01) reduction of ADA activity. From Fig. 4B, arginase activity was higher (p < 0.05) in untreated diabetic rats compared to normal control rats. This effect was reversed significantly (p < 0.01) in diabetic rats treated with 100 mg/kg of BBR and ACA. All the normal rats treated with BBR (50 and 100 mg/kg bwt) have reduced arginase activity, but not significantly different from normal control rats. Figure 4C reveals that NO level was boosted in normal rats, most especially in 100 mg/kg treated normal rats (p < 0.05) compared to normal control rats. Untreated diabetic rats have a depleted level of NO (p < 0.05) relative to normal control rats; however, the diabetic rats treated with BBR, most especially that of 100 mg/kg treated rats, had a significant (p < 0.05) increase of NO level.
Effect of oral administration of berberine (BBR) on adenosine deaminase activity (A), arginase activity (B), and nitric oxide (NO) level (C) in the kidney homogenate of normal and STZ-induced diabetic rats. Bars represent mean ± SEM (n = 8). Values are statistically different at **p < 0.001, *p < 0.05 versus normal control rats; ##p < 0.01, #p < 0.05 versus STZ-induced diabetic rats. Key: Group I: Normal control rats received citrate buffer. Group II: Normal rats treated with 50 mg/kg BBR through oral gavage. Group III: Normal rats treated with 100 mg/kg BBR through oral gavage. Group IV: Untreated 50 mg/kg STZ-induced diabetic rats. Group V: Diabetic rats treated with 50 mg/kg of BBR through oral gavage. Group VI: Diabetic rats treated with 100 mg/kg of BBR through oral gavage. Group VII: Diabetic rats treated with 25 mg/kg of acarbose (ACA) through oral gavage
The effect of oral administration of berberine on the histopathology of the kidney
Figure 5 reveals the photomicrograph of the kidney where group I (a) showed normal histoarchitecture of the kidney with the glomeruli and the tubules well delineated. Group II (b), which is normal rats treated with 50 mg/kg BBR, also revealed good histology when compared with (group III) normal rats treated with 100 mg/kg BBR (c) and diabetic rats treated with ACA (group VII), where the tubules are not distinct enough. Group IV (e) on the other hand shows signs of necrosis, especially within the glomeruli (yellow outlines). Groups V (f), group VI (g), and group VII (h) also show improved histology, but a better one is seen in group VI with the tubules clearly revealing better nephroprotective property of oral administration of BBR.
Haematoxylin and eosin of kidney tissue of groups I, II, III, IV, V, VI, and VII respectively. The group I shows normal histoarchitecture of the kidney with the glomeruli and the tubules well delineated. Group II also reveals good histology when compared with group III and group VII where the tubules are not distinct enough. Group IV the other hand shows sign of necrosis especially within the glomeruli (yellow outlines). Groups V, VI, and VII, respectively, also show improved histology but a better one is seen in group VI with the tubules clearly revealed indicating its better protective property. G, glomerulus; P, proximal convoluted tubule; D, distal convoluted tubule; stain, haematoxylin and eosin; magnification, × 400; STZ, streptozotozin; BER, berberine; ACA, acarbose. Key: Group I: Normal control rats received citrate buffer. Group II: Normal rats treated with 50 mg/kg BBR through oral gavage. Group III: Normal rats treated with 100 mg/kg BBR through oral gavage. Group IV: Untreated 50 mg/kg STZ-induced diabetic rats. Group V: Diabetic rats treated with 50 mg/kg of BBR through oral gavage. Group VI: Diabetic rats treated with 100 mg/kg of BBR through oral gavage. Group VII: Diabetic rats treated with 25 mg/kg of acarbose (ACA) through oral gavage
Discussion
The present study evaluates the renoprotective mechanism of oral administration of BBR in the management of DN model rats. Accordingly, the fasting blood glucose (FBG) level was high in the untreated diabetic rats than in the normal control (NC) rats. This suggested that the diabetic model was successful. After oral administration of BBR, the FBG level was reduced closer to that of normal control. Interestingly, diabetic rats treated with 100 mg/kg of BBR had reduced (p < 0.05) FBG compared with that of 50 mg/kg BBR-treated diabetic rats. This suggests that oral administration of BBR at 50 mg/kg can reduce FBG, but 100 mg/kg dosage had a better effect and, thus, could prevent the progression of DN (Zhang et al. 2010; Liang et al. 2019).
A significant increase in serum urea, creatinine, and uric acid levels of diabetic rats pointed towards kidney damage/dysfunctions (Ademiluyi et al. 2014; Kumaş et al. 2019). The increased levels of these biomarkers confirm that diabetes-induced damage to the kidney. However, oral administration of BBR significantly improved kidney function by lowering urea, creatinine, and uric acid levels, when compared with the untreated diabetic rats. This indicates that BBR possesses antidiabetic properties and is effective towards the management of DN; the observation agreed with the study reported by Wu et al. (2012).
Oxidative stress triggers chronic kidney disease via redox imbalance and free radical production, thus leading to a low antioxidant defence mechanism (Chirino and Pedraza-Chaverri 2009; Daenen et al. 2019). The mechanism of diabetes-induced oxidative stress involves an overwhelming production of reactive oxygen species (ROS) in tissues and cellular components, and/or decreased antioxidants, thereby, causing damage to membranes, DNA, and proteins (Kashihara et al. 2010). This effect could partly be responsible for reduced NO level in the diabetic rats, as radicals such as superoxide radical (O2¯) react with NO to form peroxynitrite (ONOO¯) and other peroxyl radicals such as MDA (Ademiluyi et al. 2016; Adefegha et al. 2021a, b). This is evidently observed in this study, as the elevated MDA level was observed in the kidney of untreated diabetic rats. MDA has been regarded as a major biomarker of oxidative stress (Sodipo et al. 2021). Also, a large number of oxidized lipids, such as hydroperoxide, have been linked to the progression of DN (Zwołińska et al. 2006). The observed decrease in endogenous antioxidant (SOD and GPx activities, and GSH level) in the kidney tissue of untreated diabetic rats as against that of the control and treated normal rats, further, confirmed the occurrence of oxidative damage and renal injury, possibly caused by the excessive production of ROS. However, treatment with BBR (50 and 100 mg/kg) reversed these effects and also decreased MDA level with a concomitant increase in the antioxidant defence mechanism via an increase in the activities of SOD and GPx, and GSH level, when compared with the untreated diabetic rats. Interestingly, the improved antioxidant defence system is significant in diabetic rats treated with oral administration 100 mg/kg BBR.
Increased activity of ADA in the kidney had been ascribed to kidney toxicity as well as defects in the DNA of cells and immune system (Kumar et al. 2015). In addition, increased ADA activity in this study could mean a decreased level of adenosine, a renoprotective molecule. Extracellular adenosine primarily functions as a signalling molecule and mediates diverse physiological functions (Adefegha et al. 2021a, b). Several studies have established the level of adenosine in hypoxia, inflammation, or acute renal injury (Yap and Lee 2012; Bowser et al. 2017). In this study, treatment with BBR prevented increased ADA activity in the kidney of diabetic rats. This apparently proves that BBR has a nephroprotective role against DN, and the ability to inhibit/reduce ADA activity could be an added mechanism of the renoprotective ability of BBR.
Several studies have reported that the inhibition of arginase activity improved kidney function in DN (You et al. 2013; Akinyemi et al. 2017). Significant elevation of arginase activity was observed in the kidney of untreated diabetic rats compared with that of normal control rats. This effect could result in low availability of ʟ-arginine, as a substrate for nitric oxide synthase (NOS) activity, hence, induce reduced NO level. Therefore, pharmacological inhibition of renal arginase activity in BBR-treated rats implies that BBR could mediate renal tissue protection. Hence, this study revealed an important role of arginase activity in the pathogenesis of renal injury and provided evidence for arginase inhibition as a potential therapeutic modality for treating DN. In this study, diabetic rats treated with BBR (50 and 100 mg/kg) had a significant reduction of arginase activity compared with that of untreated diabetic rats. Inhibition of arginase activity could increase the availability of ʟ-arginine for the production of NO by NOS activity.
The changes in the architecture observed in the histological study of kidney tissue of diabetic rats further revealed the renal damage caused by oxidative stress as a result of diabetes. Also, the observed necrosis within the glomeruli of the diabetic rats is an indication of DN compared with the normal control rats, which showed normal histoarchitecture of the kidney with the glomeruli and the tubules well delineated. However, treatment with BBR (50 and 100 mg/kg) ameliorated histological distortion compared with that of the untreated diabetic rats. Overall, histological examination showed that BBR (50 and 100 mg/kg) caused reconstruction, repair, and recovery of damaged kidneys in diabetic rats. Interestingly, diabetic rats treated with 100 mg/kg BBR showed a better protective effect.
Conclusions
This study revealed that oral administration of BBR normalized fasting blood glucose level and kidney function (urea, creatinine, and uric acid) makers in diabetic rats. The ability of oral administration of BBR to inhibit ADA and arginase activities, and MDA production and improve antioxidant status with increasing NO level could be a mechanism against DN.
References
Adefegha SA, Oboh G, Dada FA, Okeke BM, Oyeleye SI (2021a) Berberine mitigates diabetes-induced erectile dysfunction in rats through modulation of antioxidant status and critical enzyme activity. Comp Clin Pathol 30(2):181–189
Adefegha SA, Oboh G, Dada FA, Oyeleye SI, Okeke BM (2021b) Berberine modulates crucial erectogenic biomolecules and alters histological architecture in penile tissues of diabetic rats. Androlia 53(7):e14074
Ademiluyi AO, Oboh G, Agbebi O, Oyeleye SI (2014) Dietary inclusion of sorghum (Sorghum bicolour) straw dye protects against cisplatin-induced nephrotoxicity and oxidative stress in rats. Pharma Biol 52:829–834
Ademiluyi AO, Oyeleye SI, Oboh G (2016) Biological activities, antioxidant properties and phytoconstituents of essential oil from sweet basil (Ocimum basilicum L.) leaves. Comp Clinl Pathol 25:169–176
Akinyemi AJ, Oboh G, Ademiluyi AO, Araoye OO, Oyeleye SI (2014) Dietary inclusion of local salt substitutes induces oxidative stress and renal dysfunction in rats. Rev Environ Health 29:355–361
Akinyemi AJ, Onyebueke N, Faboya OA, Onikanni SA, Fadaka A, Olayide I (2017) Curcumin inhibits adenosine deaminase and arginase activities in cadmium-induced renal toxicity in rat kidney. J Food Drug Anal 25:438–446
Alía M, Horcajo C, Bravo L, Goya L (2003) Effect of grape antioxidant dietary fiber on the total antioxidant capacity and the activity of liver antioxidant enzymes in rats. Nutr Res 23:1251–1267
Antonioli L, Blandizzi C, Csóka B, Pacher P, Haskó G (2015) Adenosine signalling in diabetes mellitus—pathophysiology and therapeutic considerations. Nature Rev Endocrinol 11:228
Avwioro OG (2002) Histochemistry and tissue pathology. Clavarianum Press
Bauerle JD, Grenz A, Kim JH, Lee HT, Eltzschig HK (2011) Adenosine generation and signaling during acute kidney injury. J Am Soc Nephrol 22:14–20
Bowser JL, Lee JW, Yuan X, Eltzschig HK (2017) The hypoxia-adenosine link during inflammation. J Appl Physiol 123(5):1303–1320
Bradford MM (1976) A rapid and sensitive method for the quantitation of microgram quantities of protein utilizing the principle of protein-dye binding. Anal Biochem 72:248–254
Chen X, Zhang Y, Zhu Z, Liu H, Guo H, Xiong C, Xie K, Zhang X, Su S (2016) Protective effect of berberine on doxorubicin-induced acute hepatorenal toxicity in rats. Mol Med Rep 13:3953–3960
Chirino YI, Pedraza-Chaverri J (2009) Role of oxidative and nitrosative stress in cisplatin-induced nephrotoxicity. Exp Toxicol Pathol 61:223–242
Dada FA, Oyeleye SI, Adefegha SA, Oboh G (2020) Extracts from almond (Terminalia catappa) leaf and stem bark mitigate the activities of crucial enzymes and oxidative stress associated with hypertension in cyclosporine A‐stressed rats. J Food Biochem 13435.
Daenen K, Andries A, Mekahli D, Van-Schepdael A, Jouret F, Bammens B (2019) Oxidative stress in chronic kidney disease. Ped Nephr 34(6):975–991
de Oliveira JS, Abdalla FH, Dornelles GL et al (2016) Berberine protects against memory impairment and anxiogenic-like behavior in rats submitted to sporadic Alzheimer’s-like dementia: involvement of acetylcholinesterase and cell death. Neurotox 57:241–250
Ellman GL (1959) Tissue sulfhydryl groups. Arch Biochem Biophy 82:70–77
Giusti G, Galanti B (1984) Colorimetric method In: Bergemeyer HU (ed) Methods of enzymatic analysis Verlag Chemie Weinheim 315 – 323
Green LC, Wagner DA, Glogowski J, Skipper PL, Wishnok JS, Tannenbaum SR (1982) Analysis of nitrate nitrite and [15N] nitrate in biological fluids. Anal Biochem 126:131–138
Kashihara N, Haruna YK, Kondeti VS, Kanwar Y (2010) Oxidative stress in diabetic nephropathy. Curr Medic Chem 17:4256–4269
Kato M, Natarajan R (2014) Diabetic nephropathy—emerging epigenetic mechanisms. Nature Rev Nephrol 10:517
Kaysen GA, Strecker HJ (1973) Increased arginase activity levels caused by nitric oxide synthase dysfunction. New Eng J Med 323:1234–1238
Kumar S, Agarwal R, Bal A, Sharma K, Singh N, Aggarwal AN, Verma I, Rana SV, Jha V (2015) Utility of adenosine deaminase (ADA) PCR & thoracoscopy in differentiating tuberculous & non-tuberculous pleural effusion complicating chronic kidney disease. Ind J Med Res 141:308–314
Kumaş M, Eşrefoğlu M, Karataş E, Duymaç N, Kanbay S, Ergün IS, Üyüklü M, Koçyiğit A (2019) Investigation of dose-dependent effects of berberine against renal ischemia/reperfusion injury in experimental diabetic rats. Nefrologia 39:411–423
Liang Y, Xu X, Yin M, Zhang Y, Huang L, Chen R, Ni J (2019) Effects of berberine on blood glucose in patients with type 2 diabetes mellitus: a systematic literature review and a meta-analysis. Endocrine J 66(1):51–63
National Institutes of Health (2011) Guide for the care and use of laboratory animals 8th edition Washington (DC): National Academia Press (US)
Ni WJ, Ding HH, Zhou H, Qiu YY, Tang LQ (2015) Renoprotective effects of berberine through regulation of the MMPs/TIMPs system in streptozocin-induced diabetic nephropathy in rats. Eur J Pharmacol 764:448–456
Oboh G, Ademiluyi AO, Oyeleye SI, Olasehinde TA, Boligon AA (2017) Modulation of some markers of erectile dysfunction and malonaldehyde levels in isolated rat penile tissue with unripe and ripe plantain peels: identification of the constituents of the plants using HPLC. Pharm Biol 55:1920–1926
Oboh G, Akinyemi AJ, Oyeleye IS, Williamsnelson K (2016) Protective effect of phenolic extracts from two species of miracle berry leaves (Thaumatococcus daniellii and Megaphrynium macrostachyum) on some pro-oxidant induced oxidative stress in rat pancreas in vitro. J Appl Pharm Sci 6:118–124
Oboh G, Ogunbadejo MD, Ogunsuyi OB, Oyeleye SI (2020) Can gallic acid potentiate the antihyperglycemic effect of acarbose and metformin? Evidence from streptozotocin-induced diabetic rat model. Arch Physiol Biochem 1–9
Oboh G, Oyeleye SI, Akintemi OA, Olasehinde TA (2018) Moringa oleifera supplemented diet modulates nootropic-related biomolecules in the brain of STZ-induced diabetic rats treated with acarbose. Metab Brain Dis 33:457–466
Ohkawa H, Ohishi N, Yagi K (1979) Assay for lipid peroxides in animal tissues by thiobarbituric acid reaction. Anal Biochem Exp Med 95:351–358
Olasehinde TA, Oyeleye SI, Ibeji CU, Oboh G (2020) Beetroot supplemented diet exhibit anti-amnesic effect via modulation of cholinesterases, purinergic enzymes, monoamine oxidase and attenuation of redox imbalance in the brain of scopolamine treated male rats. Nutr Neurosci 1–15
Paglia DE, Valentine WN (1967) Studies on the quantitative and qualitative characterization of erythrocyte glutathione peroxidase. J Lab Clin Med 70:158–169
Parisa H, Siamak S (2011) Effects of Hypericum perforatum extract on diabetes-induced learning and memory impairment in rats. Phyto Res 25:544–549
Quezada C, Alarcon S, Jaramillo C, Munoz D, Oyarzun C, San Martin R (2013) Targeting adenosine signaling to treatment of diabetic nephropathy. Curr Drug Targets 14:490–496
Rehman K, Akash MSH (2017) Mechanism of generation of oxidative stress and pathophysiology of type 2 diabetes mellitus: how are they interlinked? J Cell Biochem 118:3577–3585
Singh IP, Mahajan S (2013) Berberine and its derivatives: a patent review (2009–2012). Expert Opin Ther Pat 23:215–231
Sodipo MA, Oyeleye SI, Oboh G, Agbede JO, Oluwamukomi MO (2021) Hypoglycemic effect of biscuits produced from flour blends of three medicinal foods on high-fat diet-streptozotocin-induced diabetic rats. J Food Biochem 45(3):e13334
Warren AM, Knudsen ST, Cooper ME (2019) Diabetic nephropathy: an insight into molecular mechanisms and emerging therapies. Expert Opinion on Therapeutic Targets 23(7):579-591
Wu D, Wen W, Qi CL, Zhao RX, Lü JH, Zhong CY, Chen YY (2012) Ameliorative effect of berberine on renal damage in rats with diabetes induced by high-fat diet and streptozotocin. Phytomed 19:712–718
Yap SC, Lee HT (2012) Adenosine and protection from acute kidney injury. Curr Opinion Nephrol Hyper 21:24
You H, Gao T, Cooper TK, Morris SM Jr, Awad AS (2013) Arginase inhibition mediates renal tissue protection in diabetic nephropathy by a nitric oxide synthase 3-dependent mechanism. Kid Int 84(6):1189–1197
You H, Gao T, Cooper TK, Morris SM Jr, Awad AS (2015) Arginase inhibition: a new treatment for preventing progression of established diabetic nephropathy. Am J Physiol-Renal Physiol 309:447–455
Zhang H, Wei J, Xue R, Wu JD, Zhao W, Wang ZZ, Wang SK, Zhou ZX, Song DQ, Wang YM, Pan HN (2010) Berberine lowers blood glucose in type 2 diabetes mellitus patients through increasing insulin receptor expression. Metab 59(2):285–292
Zwołińska D, Grzeszczak W, Szczepańska M, Kiliś-Pstrusińska K, Szprynger K (2006) Lipid peroxidation and antioxidant enzymes in children on maintenance dialysis. Ped Nephr 21:705–710
Acknowledgements
The authors acknowledge Professor Ganiyu Oboh of Functional Foods and Nutraceuticals Research Laboratory, Biochemistry Department, School of Science, Federal University of Technology Akure, Nigeria, for providing the laboratory facilities to carry out this study.
Author information
Authors and Affiliations
Corresponding author
Ethics declarations
Ethical approval
The investigation was performed according to the Animal Ethics Committee Guidelines for the use of experimental animals (Ethic code FUTA/ETH/2020/014).
Informed consent
Informed consent was obtained from all individual participants in this study.
Conflict of interest
The authors declare no competing interests.
Additional information
Publisher's Note
Springer Nature remains neutral with regard to jurisdictional claims in published maps and institutional affiliations.
Rights and permissions
About this article
Cite this article
Adefegha, S.A., Dada, F.A., Oyeleye, S.I. et al. Effect of oral berberine administration on the renal profiles of adenosine deaminase, arginase, and nitric oxide in streptozotocin-induced diabetic nephropathy of rats. Comp Clin Pathol 31, 255–263 (2022). https://doi.org/10.1007/s00580-022-03329-1
Received:
Accepted:
Published:
Issue Date:
DOI: https://doi.org/10.1007/s00580-022-03329-1