Abstract
Neglected tropical diseases hinder social and economic growth in many tropical areas. We report leishmanicidal activities of isoflavanquinones and antifungal potentials of fractions from methanol root extract of Abrus precatorius. Agar dilution method was used to determine antifungal properties while 96-well dilution protocol was adopted for antileishmanial activities. Ethyl acetate fraction of A. precatorius root extract showed moderate activity against M. canis and F. solani with percentage inhibition of 42.5% and 55.0% respectively while other fractions were inactive. Crude extract, n-hexane, and ethyl acetate fractions demonstrated potent antileishmanial activities with IC50 values of 22.20 ± 0.540 μg/mL, 19.35 ± 0.670 μg/mL, and 6.32 ± 0.001 μg/mL respectively, against L. major. The ethyl acetate fraction, which was the most active fraction, was subjected to successive column chromatography followed by preparative recycling HPLC in order to isolate the active constituents. Structures were established by HR-ESIMS, 1D and 2D NMR (1H, 13C, COSY, NOESY, HMBC, HSQC) spectroscopy. Two compounds were obtained and identified as isoflavanquinones: abruquinone A (1) and abruquinone B (2). Compounds 1 and 2 demonstrated significant (p < 0.05) antileishmanial activity against L. major (IC50 6.35 ± 0.005 μg/mL and 6.32 ± 0.008 μg/mL respectively) and L. tropica (IC50 6.29 ± 0.015 μg/mL and 6.31 ± 0.005 μg/mL, respectively). This appears to be the first report of antileishmanial activity of compounds 1 and 2 from the genus Abrus against cutaneous leishmaniasis and validates the use of A. precatorius in treating dermatology diseases.
Similar content being viewed by others
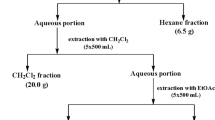
Avoid common mistakes on your manuscript.
Introduction
Many fungi and protozoa are implicated in a variety of human and animal diseases which result in retrogression in social and economic growth in many tropical areas. Leishmaniasis, one of the neglected tropical diseases, is a parasitic disease caused by genus Leishmania and transmitted via the bite of various species of sandflies (Reithinger et al. 2007). More than 20 species have been shown to be pathogenic in mammals, with affected hosts including domesticated and sylvatic animals presenting four main clinical syndromes: cutaneous leishmaniasis (CL), mucocutaneous leishmaniasis (MCL), visceral leishmaniasis/kala azar (VL), and post kala azar dermal leishmaniasis (PKDL) (Advait et al. 2014). Cutaneous leishmania caused by Leishmania major, Leishmania tropica, Leishmania mexicana, and Leishmania braziliensis is the second most severe form of Leishmaniasis, and is prevalent in Southwest Asia, Middle East, Central Asia, Africa, Southern Europe, Central, and Southern America; It has contributed to considerable global mortality, with reported 0.7 to 1.2 million cases (75%) occurring each year (Alvar et al. 2012). Despite the rise in the prevalence of this disease, available treatment drugs are inadequate and present with deleterious side effects, and resistance, hence the need for drug repurposing and novel drugs from natural product sources.
Abrus precatorius, a member of Fabaceae family, is a climbing woody shrub found in tropical regions such as Nigeria where it has many folkloric medicinal uses including diabetes management, hemoglobinuria, sore throat, rheumatism, skin infections, and jaundice treatment (Kishur et al. 2012). It is commonly called otuobiribiri or anya nwamba among the Ohafia clan in South East Nigeria. Various parts of the plant possess potent activities against infectious diseases and have been reported to have antibacterial (Holetz et al. 2002; Kalpesh et al. 2010), antimalarial and antiplasmodial (Gathirwa et al. 2011; Menan et al. 2006), and cytotoxic activities (Gadadhar and Karande 2013). A spectrum of secondary metabolites has been identified from A. precatorius, including triterpenoids (Choi et al. 1989; Xiao, et al. 2012), alkaloids (Ghosal and Dutta 1971), flavonoids (Bhardwaj et al. 1980), and isoflavanquinones (Song and Hu 1998). Here, we report bioassay-guided isolation of active isoflavanquinones from methanol root extract of A. precatorius fractions and their activity against L. major, L. tropica, and pathogenic fungi Trichophyton longifusis, Candida albicans, Candida glabarata, Fusarium solani, Microsporum canis, and Aspergillus flavus.
Materials and methods
Plant material
Young A. precatorius roots were harvested from a local farm in Umuoriehi Umuahia, South East Nigeria, and were authenticated at the herbarium unit of the Botany Department, Obafemi Awolowo University, Ile-Ife, Nigeria. A voucher specimen (IFE-17655) was deposited for future reference. The roots were thoroughly washed to remove sand and other contaminating particles, oven-dried (Uniscope SM9053) at 40 °C for 96 h to a constant weight. The dried samples were pulverized using a mechanized milling machine (grinder) and then stored individually in portions in air-tight containers for subsequent analysis.
Extraction and isolation
Pulverized A. precatorius roots (350 g) were macerated in 70% methanol in airtight transparent jars. Each jar containing 50 g of pulverized sample and 500 mL of 70% methanol (1:10) were shaken for 48 h in a shaker water bath (Uniscope SM101) at 40 °C. The crude methanol extract was obtained by percolation via accelerated filtration under vacuum at constant pressure and filtrate concentrated using a rotary evaporator (Stuart RE 300) under reduced pressure and controlled temperature (40 °C). The crude methanol extract (98.83 g) was reconstituted in distilled water (495 mL) and subjected to liquid–liquid fractionation in order of increasing polarity of solvents to obtain n-hexane (8.23 g), ethyl acetate (15.81 g), n-butanol (17.33 g), and aqueous (29.05 g) fractions respectively. These fractions were screened for antifungal and antileishmanial activities, following which the ethyl acetate fraction was subjected to bioassay-guided fractionation using column chromatography methods. The ethyl acetate fraction (15.81 g) was pre-absorbed in 31.62 g of silica gel (1:2). Wet packing of 375 g of activated silica gel (230–400 mesh) was done using hexane in a glass column (85 cm × 7 cm, 1D). Successive gradient elution starting with Hex-100% to DCM 100% to MeOH 50% v/v afforded 10 fractions based on TLC analysis with UV visualization at 254 nm and 380 nm; compound 1 was eluted with Hex:DCM (3:7 v/v) mobile phase solvent system. Fraction 5 was subjected to recycling preparative HPLC (LC–908 W) and eluted with Hex:EtOAc:MeOH (8:1.5:0.5 v/v) mobile phase system at a flow rate 4 mL/min to afford compound 2. HRESIMS, 1D (1H-NMR) and 2D NMR (13C, COSY, NOESY, HMBC, HSQC) spectroscopy techniques were done to characterize and elucidate the structures of compounds 1 and 2.
Bioassays
Antifungal activity bioassay
Agar dilution method (Choudhary et al. 1995) was used to determine the antifungal activities of fractions from A. precatorius root methanol extracts against human fungi pathogens T. longifusis, C. albicans, C. glabarata, F. solani, M. canis, and A. flavus. Briefly, 24 mg of A. precatorius root were dissolved in 1-ml sterile DMSO serving as a stock solution. Sabouraud dextrose agar (SDA) was used for the growth of fungus. Media with acidic (pH 5.5–5.6) containing a relatively high concentration of glucose or maltose 2% was prepared by mixing 32.5 gm/500 ml distilled water, steamed to dissolve the contents and dispensed as volumes 4 mL into screw cap tubes and autoclaved at 121 °C for 15 min. Tubes were allowed to cool to 50 °C and non-solidified SDA was loaded with 66.6 μl of fractions pipetted from the stock solution to give a final concentration of 400 μg/mL of the media. Tubes were allowed to solidify in slanting position at room temperature and inoculated with 4-mm diameter piece of fungus removed from a 7-day-old culture of fungus. An agar surface streak was employed for non-mycelial growth while other media supplemented with DMSO and reference antifungal drugs (miconazole and amphotericin B) were used as negative and positive controls respectively. The tubes were incubated at 27 °C for 7 days. Growth in the extract amended media was determined by measuring linear growth (mm) and growth inhibition calculated with reference to the negative control using the formula:
Antileishmanial activity bioassay
Ninety-six (96) well dilution protocol was adopted for the study as described by (Yoshie, et al. 2013) with slight modification. Briefly, 950-μL RPMI-1640 media (Invitrogen, Darmstadt, Germany) supplemented with 50-mL heat-inactivated fetal calf serum (FCS; PAA, Pasching, Austria) and 5 ml 200-mM l-glutamine (Biochrom AG, Berlin, Germany) were added to 50 μL DMSO. A total of 180 μL of RPMI-1640 media was introduced into the first well and 100 μL into the remaining wells. Twenty microliters of test fractions and compounds 1 and 2 were added, followed by serial dilution. A total of 100 μL suspension of test organisms were added in all wells and sealed with novax sealing tape, incubated for 72 h at 25 °C. Amphotericin and pentamidine served as standard drugs. Growth inhibition was determined by counting the number of cells in Neubauer chamber, IC50 determined using EZ-Fit™ (Perrella Scientific software, USA) and percentage inhibition calculated using the formula:
Statistical analysis
Data were analyzed using EZ-Fit™ (Perrella Scientific software, USA) and expressed as mean ± SD. P < 0.05 at 95% confidence level was considered statistically significant for differences in means.
Results
A bioassay-guided fractionation, followed by repeated chromatographic purification of MeOH soluble fraction of A. precatorius root MeOH extract afforded two active compounds 1 and 2. Compound 1 was obtained from fraction 3 following elution with Hex:DCM (3:7 v/v) mobile phase solvent system on silica gel column chromatography, analyzed on TLC Hex:EtOAc:MeOH (6.9:3:0.1 v/v) solvent system and developed using 20% H2SO4 spray reagent to give a clear yellow spot visible at 254 nm. Compound 1 (17.8 mg) appeared as orange needles. Compound 2 was obtained following purification by normal phase recycling preparative HPLC using Hex:EtOAc:MeOH (8:1.5:0.5 v/v) mobile phase system with retention time (tR) 58.2 min (10 mg), analyzed on TLC using Hex:EtOAc:MeOH (8:1.5:0.5) v/v solvent system and developed using 20% H2SO4 spray reagent. Compound 2 appeared as brownish sticky mass. The molecular formulae of 1 and 2 were established as C19H20O7 and C20H22O8 (calculated [M+H]+m/z 360.2200 and m/z 390.2200) for molecular ions m/z 360.12 [M+H]+ and 390.13 [M+H]+, respectively, by HRESIMS (JEOL600HI). Their isoflavanquinone-based carbon skeletons were established by 1D and 2D NMR data corresponding to previously reported data (Kuo et al. 1995; Song and Hu 1998) and identified as 6,7,3′,4′tetramethoxy-2′,5′-isoflavanquinone also known as abruquinone A (Fig. 1) and 2,3-dimethoxy-5-(6,7,8-trimethoxy-3,4-dihydro-2H-1-benzopyran-3-yl)cyclohexa-2,5-diene-1,4-dione also known as abruquinone B (Fig. 2) respectively. Both isoflavanquinones were isolated fully characterized and evaluated for L. major and L. tropica.
The antifungal activities of fractions of A. precatorius root methanol extracts are presented in Table 1. MeOH soluble fractions from the root of A. precatorius were assayed against human pathogenic fungi T. rubrum, C. albicans, A. niger, M. canis, F. solani, and C. glabrata. All fractions showed no activity against assayed fungi except the ethyl acetate fraction which showed moderate activity against M. canis and F. solani with percentage inhibition of 42.5% and 55.0% respectively. Crude extract and butanol fraction showed an insignificant inhibition of 20% and 5% respectively against F. solani (Table 1).
Antileishmanial activities of fractions of A. precatorius root methanol extracts and compounds 1 and 2 are presented in Table 2. The crude extract, n-hexane, and ethyl acetate fractions of root methanol extracts of A. precatorius demonstrated potent antileishmanial activities with IC50 values of 22.20 ± 0.540 μg/mL, 19.35 ± 0.670 μg/mL, and 6.32 ± 0.001 μg/mL respectively, against L. major, compared with those observed for the standard drug, Amphotericin B (IC50 0.39 ± 0.001 μg/mL) and pentamidine (IC50 3.15 ± 0.005 μg/mL). n-Butanol and aqueous fractions showed low activity against L. major with an IC50 value of 66.90 ± 0.450 μg/mL and ˃ 100.00 μg/mL respectively compared with standard controls. From the present study, ethyl acetate fraction among all assayed fractions was the most active fraction and was further subjected to fractionation and successive chromatographic purifications to afford compounds 1 and 2. Compounds 1 and 2 demonstrated significant (p < 0.05) potent activity when evaluated against L. major (IC50 6.35 ± 0.005 μg/mL and 6.32 ± 0.008 μg/mL, respectively) and L. tropica (IC50 6.29 ± 0.015 μg/mL and 6.31 ± 0.005 μg/mL, respectively) compared with those observed for the standard drugs amphotericin B (IC50 0.40 ± 0.007 μg/mL) and pentamidine (IC50 3.21 ± 0.005 μg/mL) respectively (Table 2).
Discussions
M. canis is a zoophilic dermatophyte and is among the most common dermatophytes associated with tinea capitis and tinea corporis in humans, especially children and ringworms in pets (Lunder 1992). F. solani is involved in most cases of systemic fusariosis and corneal infections and is one of the most common agents in disseminated and cutaneous infections especially in immuno-compromised patients (Kerry et al. 2008). Ethyl acetate fraction as observed in this study showed potent growth inhibition of M. canis and F. solani and may be a good drug source candidate against dermatology infections caused by these fungi spp. This finding is congruent with a similar report by Lubna et al. (2018).
Cutaneous leishmaniasis (CL) is one of the most frequent skin disorders in the New World, and accounts for around 60% of all cases in nonendemic areas (Androula and Helena 2010); most of CL cases in the Old World are caused by two Leishmania species: L. major and L. tropica. Two distinct morphologies characterize the life cycle of the Leishmania parasites: the elongated and flagellated promastigote, found in the alimentary tract of the female sand fly vector, and the round nonmotile amastigote, present in the bloodstream and tissues of the mammalian host. The parasites persist in macrophages present in the spleen, bone marrow, liver, and lymph nodes and induce extensive inflammation and increased hematopoiesis (Max et al. 2013). Pentamidine, one of the currently prescribed treatment drugs present with deleterious safety concerns such as insulin-dependent diabetes mellitus, hypoglycemia, hypotension, renal toxicity, and myocarditis (Michael et al. 1985) while the deoxycholate form of amphotericin B has many adverse effects including infusion reactions, nephrotoxicity, hypokalemia, and myocarditis (Messori et al. 2013).
Leishmaniasis drugs have had poor economic returns as endemic areas are typically impoverished despite increasing patient population. In order to bolster the pipeline, significant effort has been applied in repurposing drugs from different indications. In this study, our results show the potential use of isoflavaquinones namely abruquinone A and B isolated from the roots of A. precatorius in the treatment of Leishmaniasis. Earlier work has reported the anti-inflammatory, antiplatelet and antitubercular, antiparasitic, and antimicrobial properties of these compounds and other related compounds from the genus Abrus (Aziz et al., 2011; Chutima et al. 2004; Kuo et al. 1995; Yoshie et al. 2013). To the best of our knowledge, this is the first report of antileishmanial activity of compounds 1 and 2 from the genus Abrus against cutaneous leishmaniasis.
In conclusion, this study demonstrates potent antileishmanial activities of isoflavanquinones isolated from methanol soluble fractions of roots of A. precatorius as well as antifungal potentials of the ethyl acetate fraction and therefore, validates the folkloric use of A. precatorius in treating various dermatology diseases of fungal and protozoan origin. Compounds 1 and 2 can provide viable alternative chemical space towards drug development against neglected tropical diseases especially cutaneous leishmaniasis. In vivo antileishmanial studies are being considered against promastigotes and amastigotes of various Leishmania species.
References
Advait SN, Shilpi K, Arun BK, Frantisek S, Andriy B, Casey JM, Valentina M (2014) Recent developments in drug discovery for leishmaniasis and human African trypanosomiasis. Chem Rev 114:11305–11347
Alvar J, Ve'lez I, Bern C, Herrero M, Desjeux P, Cano J, den Boe M (2012) Leishmaniasis worldwide and global estimates of its incidence. PLoS One 7(5):e35671
Androula P, Helena CM (2010) Leishmaniasis, an emerging infection in travelers. Int J Inf Secur 14:e1032–e1039
Aziz AR, Volodymyr S, Surendra KJ, Babu LT, Shabana IK, Melissa RJ, Ilias M (2011) Antiparasitic and antimicrobial isoflavanquinones from Abrus schimperi. Nat Prod Commun 6(11):1645–1650
Bhardwaj D, Bisht M, Mehta C (1980) Flavonoids from Abrus precatorius. Phytochemistry 19:2040–2041
Choi Y, Hussain R, Pezzuto J, Kinghorn A, Morton J (1989) Abrusosides A-D, four novel sweet-tasting triterpene glycosides from the leaves of Abrus precatorius. J Nat 52:1118–1127
Choudhary M, Dur-e-Shahwar PZ, Jabbar A, Ali I, Atta-ur-Rahman (1995) Antifungal steroidal lactones from Withania coagulance. Phytochemistry 40(4):1243–1246
Chutima L, Sinenart S, Prasat K (2004) Antitubercular and antiplasmodial constituents of Abrus precatorius. Planta Med 70:276–278
Gadadhar SS, Karande AA (2013) Abrin immunotoxin: targeted cytotoxicity and intracellular trafficking pathway. PLoS One 8(3):e58304
Gathirwa J, Rukunga G, Mwitari P, Mwikwabe N, Kimani C, Muthaura C, Omar S (2011) Traditional herbal antimalarial therapy in Kilifi District, Kenya. J Ethnopharmacol 134:434–442
Ghosal S, Dutta S (1971) Alkaloids of Abrus precatorius. Phytochemistry 10:195–198
Holetz FB, Perrini GL, Sanches NR, Coritez DAG, Nakamura CV, Filho BPD (2002) Screening of some plants used in the Brazilian Folk Medicine for the treatment of Infectious Diseases. Mem I Oswaldo Cruz 97:7
Kalpesh M, Mehta M, Mendpara N, Garmit S, Shah G (2010) Determination of antibacterial activity of MIC of crude extract of Abrus precatorius L. Bitotechnol Adv 10:2
Kerry O, Deanna AS, Annette F, Dora M, Michael GR, Mary EB, David MG (2008) Molecular phylogenetic diversity, multilocus haplotype nomenclature, and in vitro antifungal resistance within the Fusarium solani dpecies complex. J Clin Microbiol 46(8):2477–2490
Kishur SC, Ramakant S, Pradeep SP, Vidyadhish AK (2012) Pharmacological activities of Abrus precatorius Linn – a review. Int J Ayurvedic Herb Med 2(2):336–348
Kuo SC, Chen SC, Chen LH, Wu JB, Wang JP, Teng CM (1995) Potent antiplatelet, anti-inflammatory and antiallergic isoflavanquinones from the roots of Abrus precatorius. Planta Med 61(4):307–312
Lubna M, Syed AS, Rashida A, Syed GS, Rahil A (2018) Antibacterial and antifungal activities of the polyphenolic fractions isolated from the seed coat of Abrus precatorius and Caesalpinia crista. Nat Prod Res 32(23):2835–2839
Lunder M (1992) Is Microsporum canis infection about to become a serious dermatological problem? Dermatology 184:87–89
Max G, Mark H, Wiliam E, Thomas H, John SL, Elizabeth RS, Richard JS (2013) Review: drug discovery algorithm for cutaneous leishmaniasis. Am J Trop Med Hyg 88(2):216–221
Menan H, Banzouzi J, Hocquette A, Pelissier Y, Blache Y, Kone M, Valentin A (2006) Antiplasmodial activity and cytotoxicity of plants used in West Africa traditional medicine for the treatment of malaria. J Ethnopharmacol 105:131–136
Messori A, Fadda V, Maratea D, Trippoli S, Marinaj C (2013) Nephrotoxicity of different formulations of amphotericin B: summarizing evidence by network meta-analysis. Clin Infect Dis 57(12):1783–1784
Michael S, Michael AK, Richard BB (1985) Pentamidine: a review. Clin Infect Dis 7(5):625–634
Reithinger R, Dujardin J, Louzir H, Pirmez C, Alexander B, Brooker S, Lockwood D (2007) Cutaneous leishmaniasis. Clin Dermatol 25:203–211
Song C, Hu Z (1998) Abruquinone A, B, D, E, F, and G from the root of Abrus precatorius. Acta Bot Sin 40:734–739
Xiao Z, Wang F, Sun A, Li C, Huang C, Zhang S (2012) A new triterpenoid saponin from Abrus precatorius Linn. Molecules 17:295–302
Yoshie H, Melanie R, Samad NE, Stefanie Z, Tsholofelo M, Dashnie N, Mathias H (2013) Antiprotozoal isoflavanquinones from Abrus precatorius ssp. Africanus. Planta Med 79:492–498
Acknowledgment
EEO acknowledges the World Academy of Sciences for the advancement of Science in Developing Countries (TWAS), Trieste, Italy, for ICCBS-TWAS Fellowship (2018) at the H.E.J. Research Institute of Chemistry, ICCBS, University of Karachi, Karachi, Pakistan.
Funding
This research work was fully funded by the International Centre for Chemical and Biological Sciences, ICCBS, University of Karachi, Karachi, Pakistan and The World Academy of Science (TWAS) Trieste, Italy, for the advancement of Science in Developing Countries.
Author information
Authors and Affiliations
Contributions
EEO conceptualized and designed the research work; EEO carried out the assays, analyzed data, and wrote the article manuscript, MSA elucidated the chemical structures; MSA, ORO, and FDO proofread the article manuscript.
Corresponding author
Ethics declarations
Ethical approval
Not required
Competing interests
The authors declare that they have no competing interests.
Additional information
Publisher’s note
Springer Nature remains neutral with regard to jurisdictional claims in published maps and institutional affiliations.
Rights and permissions
About this article
Cite this article
Okoro, E.E., Ahmad, M.S., Osoniyi, O.R. et al. Antifungal and antileishmanial activities of fractions and isolated isoflavanquinones from the roots of Abrus precatorius. Comp Clin Pathol 29, 391–396 (2020). https://doi.org/10.1007/s00580-019-03073-z
Received:
Accepted:
Published:
Issue Date:
DOI: https://doi.org/10.1007/s00580-019-03073-z