Abstract
This study examines the extent to which people with different past thermal experience and “climatocultural” history systematically report different outdoor thermal sensations and thermal comfort patterns. After constructing two distinct climatocultural groups co-inhabiting the research setting (native Israelis vs. representatives of colder regions), and comparing their relative thermal preferences in both short-term and long-term observational experiments, we confirmed the existence of a strong correlation between affiliation to a certain climatocultural group and outdoor thermal sensation. It was shown that the degree of this correlation was not a constant value, and the strength of the difference in thermal sensation between different groups might change subject to different environmental conditions and possibly expectations. Under some environmental conditions, i.e., stressful but not extreme, the differences may be accentuated, while under others, i.e., either comfortable or extremely stressful, the scope of difference may diminish. Short-term acclimation may to some extent offset the differences between separate climatocultural groups. The study also showed that the meaning of the thermal comfort scale itself may be perceived unevenly between representatives of different groups.
Similar content being viewed by others
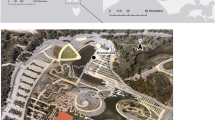
Avoid common mistakes on your manuscript.
Introduction
It is well understood that the level of thermal stress imposed on a person by the physical environment is related to the energy balance between that person’s body and the surroundings. This balance results primarily from energy exchanges in the form of radiation and convection, and under warm conditions is modulated by sweat evaporation. A pedestrian in an urban environment is typically exposed to conditions of sun and wind that decisively influence this energy balance, and therefore a detailed accounting of the various heat fluxes, rather than a simple record of temperature, is more likely to offer a realistic portrayal of the thermal environment experienced by a person outdoors.
Human thermal physiology models based on these exchanges have become increasingly abundant and in some cases complex (Zhang et al. 2001), but at the same time such models have never been able to fully predict the thermal comfort responses of individuals in a particular setting. This inability lies in the fact that the notions of thermal comfort, sensation, and perception are much more complex than what is described in any physical or physiological model—including, as they do, psychological and experiential aspects which may be stochastic and dynamic in nature.
Accordingly, while models such as the Index of Thermal Stress (ITS) have been shown to robustly describe the thermal environment in terms that are relevant to subjective thermal sensation, these descriptions have also been shown to be context dependent (Saaroni et al. 2015). For example, Pearlmutter et al. (2014) found that under hot and dry conditions, pedestrians in open spaces have a greater tolerance for thermal variations than subjects who are placed in the controlled conditions of a climate chamber. It has been suggested that such limitations on the capacity of physiological models to universally predict individual thermal comfort responses are intrinsic to the modeling approach and that these limitations are governed by the intricacy of such notions as thermal “perception,” which differs from thermal “sensation” in that it involves not only the detection of a stimulus in the environment, but also the interpretation of this information (Brager and de Dear 2003; Zhang and Zhao 2008; De Dear 2010). Thus the relationship between physical stress and human perception inevitably includes non-physical factors, such as expectations which have become engrained due to prior psychological conditioning—a phenomenon referred to as thermal adaptation (Gosling et al. 2014). The link between perception, expectations, adaptation, and prior psychological conditioning is profound. The existence of a self-reinforcing feedback between expectations and perceptions is well-known (Plous 1993), and expectations are predicated on historical information and past environment, while adaptation also occurs as people learn from experience (Sterman 2000). A detailed description of various cognitive and affective theories and models, which highlight the relationship between perception, expectations, and adaptation, is given by Brennan et al. (2014).
Many of these theories confirm the link between past experiences and sociocultural background, on the one hand, and human perception, on the other. Examples include Bronfenbrenner’s sociocultural “ecological systems model” and Kollmuss and Agyemans’ “model of pro-environmental behaviour,” which account for sociocultural factors and link them to human perceptions and behaviors, and Vlek’s “needs, opportunities and abilities” model, in which culture directly affects human abilities, intentions, behavioral control and behavior, and subjective well-being (Brennan et al. 2014).
Both short-term (directly affected by immediate thermal loads) and long-term (influenced by sociocultural factors) thermal adaptation are important to consider when explaining differences in perceived thermal comfort, especially among groups of people for whom built environments are designed. This is because the assumption of an “ideal temperature” for all, by which people wearing similar clothes and doing similar activities are thought to be equally comfortable with the same conditions no matter where they are in the world, does not account for the variations and complexities of people’s day-to-day reality in different cultures and climates (Wilhite et al. 1996; Nakano et al. 2002; Chappells and Shove 2004; Yamtraipat et al. 2005; Indraganti and Rao 2010).
Traditional urban settings often evolved in tandem with a lifestyle that provided behavioral protection from challenging extremes, with inhabitants using clothing and various mechanisms provided by the built environment as part of their comfort strategy. It has been suggested that the introduction of technologies such as mechanical cooling and motorized transport have had radical impacts on the culture within and around buildings, that have tended to cut the traditional links between regional culture and climate—favoring instead those of the “international” culture of air-conditioned life styles and buildings (Schiller and Evans 1998; Roaf 2006; Pearlmutter 2007). This “universality” has been called into question by a multitude of studies; for example, Stoops (2002) indicated that Portuguese office workers are content with a much wider range of seasonal variation (up to 5 °C) than Swedes, who expect indoor temperatures to waver by no more than half a degree.
One of the implications of this shift is a relative inattention to the thermal quality of the outdoor urban environment, and the subsequent replacement of pedestrian activity with a greater reliance on air-conditioned vehicles and interior spaces. Such practices foster high energy consumption and adversely affect the biological fitness of people by substituting the human body’s own thermoregulatory processes with energy-intensive appliances (de Dear and White 2008).
In response to the “one-size-fits-all” approach and its various implications for long-term sustainability, the idea of an “adaptive approach” to thermal comfort initially evolved as a way to modify the prescribed “comfort zone” for buildings in different climatic zones around the world by accounting for prevailing conditions outdoors (de Dear and Brager 1998; Humphreys and Nicol 1998; Nicol and Humphreys 2002). Though for some time its focus was limited to indoor spaces (Golany 1996), the adaptive concept eventually expanded to include outdoor comfort as well—since although they are less amenable to climatic control, comfortable open spaces can contribute inestimably to the quality of life within cities (Höppe 2002; Nikolopoulou and Steemers 2003; Spagnolo and de Dear 2003; Kenawy and ElKadi 2011). In fact, it has become increasingly apparent that the dynamic complexity of the outdoor environment, which amplifies the limitations of simplified physiological models, makes the consideration of regional adaptation all the more important—as illustrated in several recent case studies in hot humid and hot dry climates (Lin et al. 2011; Middel et al. 2016; Ndetto and Matzarakis 2017).
In a more general sense, the adaptive approach takes into account the various adaptations—be they physiological, psychological, or behavioral—that play a key role in determining subjective thermal sensation and perception (Yao et al. 2009). There is a recognition that the physical environment informs the norms and behavioral patterns of geographically distinct groups, and that these in turn influence the expectations and thermal perceptions—i.e., the long-term acclimation—of individual members of that group (Triandis 1994; Knez and Thorsson 2007). Numerous studies, many of which were summarized in a review by van Hoof (2008) have highlighted the differences in thermal preferences between subjects based on the circumstances of their culture and acclimation—and it is these “climatocultural factors” that underlie the present analysis.
The effect of climatocultural factors on thermal perception has been incorporated within the adaptive model of thermal comfort. Auliciems (1981), an early pioneer of the research of this model, argued that two interacting components—climatocultural norms/practices and past thermal environments—directly influence thermal expectations as well as thermal sensations, preferences and satisfaction. Interestingly, he considered that climatocultural norms/practices are modified by behavioral and technological adjustments, as well as by existing heat/cold loads on the body, thus forming, with other factors, a system of feedback loops of interaction (Auliciems 1981). It is logical that such a system is complex, dynamic, and non-linear, which enhances uncertainty in uncovering the specifics of thermal interactions and defining the nature of influencing factors. This makes it difficult for a researcher to discern the nature of a factor which might be instrumental in shaping a specific thermal perception. One can mostly uncover the existence of variations in thermal perceptions and link these variations to some broader categories, like the afore-mentioned “climatocultural” factors.
Thus, while we consider in this study the role of short-term acclimation (resulting from changes in the immediate thermal environment), our primary focus is on the type of long-term acclimation which derives from an individual’s “thermal history” and is expressed in terms of fundamental thermal expectations. We hypothesized that the thermal perception and preferences of two distinct co-resident climatocultural groups (native-born Israelis, on the one hand, and non-Israelis with a primary past experience of living in a much colder environment, on the other) may systematically differ, even as the individual members of each group are influenced by current environmental conditions and personal expectations. The main research question of this paper is therefore as follows: Could affiliation to different climatocultural groups correspond with variations in outdoor thermal perception?
Methods
From the introductory remarks above, it is clear that the potential differences in thermal perception between population groups living in diverse climatic regions have been examined in prior studies. There is little evidence, however, of experimental research in which the thermal preferences of people with qualitatively different climatocultural backgrounds have been compared systematically when located in the same climatic setting. In this study, we make use of a unique setting in Israel that could be considered a “climatocultural laboratory” to do just this.
The Sede-Boqer campus of Ben-Gurion University of the Negev is a relatively self-contained community located in the arid Negev region of southern Israel. The region is characterized by hot dry summers (average daily temperature range of 18–32 °C in July) and predominantly clear days in winter, though with temperatures that are more severe than in most of Israel, which has a generally mild Mediterranean climate. In its capacity as an international graduate school, the campus hosts a diverse student population which includes not only native-born Israelis but also an appreciable number of visitors from foreign countries who live in Israel for a short period (of two or more years). This arrangement presented the opportunity of comparing the thermal preferences of different population groups whose members have undergone long-term “acclimation” in sharply different thermal environments and practices over the course of their lives, but whose perceptions and preferences may be observed in a single common location where they both dwell for some period.
In order to map this “climatocultural diversity” and construct distinct climatocultural groups for comparison, research participants were selected among the Sede-Boqer student population, which can be distinguished not only by its multinational character, but also by a fairly high degree of uniformity in age (young adults) and status (university students). As a first step, 105 in-depth interviews were conducted with individual students with a special emphasis on their individual climatic history, personal evaluation of the Sede-Boqer climate and general thermal preferences. From these interviews, it was possible to map the climatocultural diversity of the population and to construct two distinct groups. Group 1 consisted of locally born Israelis and residents of nearby countries with no long-term experience of living in distinctly cold climates, and group 2 consisted of non-Israelis who were raised and had prolonged or prevailing experience of living in cold-climate countries (primarily in northern Europe) as illustrated in Fig. 1. Each group included 45–50 subjects in total, with between 20 and 30 from each group participating in each of the individual experiments described below.
Geographical distribution of the survey sample, with circle size indicating the relative proportions of participants from group 1 (red) and group 2 (blue) countries (ISR = Israel, JOR = Jordan/PA, TUR = Turkey, ARM = Armenia, RUS = Russia, BLR = Belarus, DEU = Germany, FRA = France, and USA = northern regions of United States)
Using these two highly refined focus groups, two types of thermal comfort surveys were conducted—the first extending over a lengthy period in order to generate a large volume of responses, and the second concentrated in two single-day experiments which compared participant responses under identical conditions.
Extended remote-response survey
In this survey, we remotely collected daily thermal comfort votes of subjects in the two focus groups, who were asked to report electronically (via e-mail or instant messaging) on their current thermal sensation. Using this procedure, all responses were time-stamped, allowing for accurate recording of the exact time and date that the responses were received. Respondents were instructed to report their “comfort vote” once each day at a time of their choosing, but only under the condition that they were present at the Sede-Boqer campus, and had remained stationary for at least several minutes in an outdoor location which was (a) in the shade (i.e., protected from direct solar radiation), and (b) protected from the wind as far as possible, by utilizing shelter from adjacent buildings.
While the specific site of voting was unknown, the location of all respondents was limited to the built-up area of the Sede-Boqer campus (within a radius of several hundred meters, and with a fairly even distribution of uniform one- and two-story buildings, shown in Fig. 2b, c, which also describe the locations of the second, location-based, type of experiments). In lieu of micro-scale information, but with some confidence that differences in radiant loading and wind effects would be minimal, the assessment of environmental conditions in this extensive survey was limited to the measurement of screen-height air temperature within the campus, recorded continuously at 10-min intervals. Metabolic activity and the clothing insulation were assumed to be typical for the season and time of day with respondents in a resting state and protected from direct sun and wind. The decision to limit the range of variables was dictated by the need to gather large volumes of data without relying on subjects to fill in lengthy forms or report at known locations equipped with meteorological devices, and in total 2055 responses were recorded (1249 in winter and 806 in summer).
The period of reporting was divided into a cold winter season period (November 2010–January 2011), and a hot summer season period (June–July 2011). The response consisted of a thermal comfort “vote” using a seven-point scale (1 = cold, 2 = cool, 3 = comfortably cool, 4 = comfortable/neutral, 5 = comfortably warm, 6 = warm, and 7 = hot) in winter, with the additional category of “very hot” (8) added in summer.
Location-based group experiments
Two single-day experiments were held in which the members of both focus groups recorded their comfort votes simultaneously, while sitting or standing together in a series of defined locations. The cold-season experiment was held in late winter (March 2011) and the hot-season experiment was held in late summer (September 2011).
Each experiment consisted of thermal comfort voting in a series of four locations (Fig. 2), with temperature measurements made simultaneously at each point. Initially the respondents underwent a 30-min period of “conditioning” inside the climate-controlled conditions of a classroom space, recording their comfort votes using the previously mentioned comfort scale. The entire group then moved to a location outside the room and recorded individual outdoor comfort votes, and the process was repeated at a second point nearby. Finally, the subjects returned to the room and cast a final comfort vote. In total, 172 votes were recorded in winter and 176 in summer. The use of two independent experiments of identical type, held at different times of the year (winter and summer) but at the same premises, allowed for a broad comparison of the thermal perceptions of participants by means of a “thermal vote” in both outdoor and indoor environments.
Statistical analysis
Statistical analyses were performed using one- or two-way analysis of variance (ANOVA) depending on the experimental design. For testing statistical differences between groups, ANOVA was combined with the Student t test and Tukey-Kramer HSD test. Bivariate Pearson correlation (for normally distributed data) or Spearman’s rank correlation analysis (for non-normally distributed data) were used to analyze correlations. Univariate linear regression analysis was performed to show the dependence of thermal comfort responses on temperature with respect to subjects’ climatic origin and gender.
Furthermore, data were analyzed for homogeneity of variance and linearity of the residuals (residuals plotted over fitted values). All analyses were performed using JMP software (8.0, SAS Institute Inc.) for Windows. Analysis of covariance (ANCOVA) was used to compare Y-intercepts and slopes of linear regression lines (http://vassarstats.net/).
Results
Initial differences between groups
The intake-interviews allowed us to identify a number of initial differences in thermal perception and preference that were indicated between the members of groups 1 (of “warm-country” origin) and 2 (of “cold-country” origin).
Responses to the question “Do you consider yourself a ‘heat-loving’ person (who prefers more exposure to warm environments) or a ‘cold-loving’ person (who prefers more exposure to cool environments)?” revealed the predominance of heat-loving persons among group 1 and cold-loving persons among group 2 (Fig. 3). This initial self-definition by the group members reinforced our distinction between the two groups as representing individuals who have indeed undergone long-term thermal acclimation to relatively “warm” and “cold” conditions.
Thermal preference among the representatives of group 1 and group 2. The two groups (n = 92) showed a significant difference at α = 0.95. (See statistical data in Appendix, Table 1). In this figure and throughout the paper, “TH” stands for “thermal history”
It was also found, to a statistically significant degree, that subjects in group 1 perceive winter in Sede-Boqer as colder than those from group 2 (Fig. 4a), while subjects from group 1 perceive summer in Sede-Boqer as more comfortable than those from group 2 (Fig. 4b).
Evaluation of Sede-Boqer climates by the representatives of groups 1 and 2 in winter (a) and summer (b). The two groups (N = 91, for winter evaluation and N = 88 for the summer evaluation) showed significant difference at α = 0.95. (See statistical data in Appendix, Table 1)
When asked to evaluate the overall climate of Sede-Boqer, the bulk of subjects from both group 1 and group 2 gave a response of “comfortable” (Fig. 5).
Evaluation of the overall climate in Sede-Boqer by the representatives of groups 1 and 2. Fisher’s exact test showed significant difference between the groups (n = 87) at α = 0.95. (See statistical data in Appendix, Table 1)
Differences in remotely observed thermal comfort responses
From data collected in the extended seasonal survey based on electronic voting, a number of differences in thermal perception may be observed between groups 1 and 2. The basic distribution of votes for the two groups is shown in Fig. 6 for winter (a) and summer (b).
The distribution of remotely observed thermal votes for groups 1 and 2, in a winter and (b) summer. Fisher’s exact test and t test with votes treated as continuous variables showed significant difference between the groups [for winter: n = 1249 (group 1 = 622; group 2 = 627); for summer: n = 806 (group 1 = 403; group 2 = 403)] at α = 0.95. (See statistical data in Appendix, Table 1)
In winter, group 1 members recorded 359 of its 622 votes (58%) within the comfort range (as represented by the votes “comfortably cool,” “comfortable/neutral,” and “comfortably warm”), while nearly 85% of group 2 votes were in this same comfort range. Figure 6a clearly shows that in winter, perceptions in group 2 are on average closer to the point of thermal neutrality than those of group 1, which fall much more heavily on the “colder” side of the thermal spectrum.
In summer, the opposite may be seen: the proportion of total votes within the comfort range for group 1 (52%) is much higher than that of group 2 (only 18%). While the average perception in the former is well above neutrality it is still within the comfort zone, whereas the average for the latter is clearly outside this zone, in the range of significant discomfort due to overheating.
In Figs. 7 and 8, the distribution of comfort votes in the two groups is shown as a function of the simultaneously measured air temperature. The regression lines in Fig. 7 show this relation in winter, and it is clear that subjects from group 1 perceive a low-temperature environment as significantly colder and less comfortable than those in group 2. However, it is also clear that the subjective thermal sensation of group 1 rises more rapidly with respect to measured temperature—such that at higher (i.e., milder) temperatures, the difference between the perceptions of the groups diminishes. While at the lowest temperatures, the average difference in perception is approximately two steps on the comfort scale (from 1 to 3, which is equivalent to the total change in group 2’s average response over the temperature range), the average difference over the winter temperature range is approximately half this, or one step.
Relation between air temperature (Tair in °C) and thermal comfort vote for group 1 (red) and group 2 (blue) in winter. The analysis of variance and Fisher’s test showed that the linear fit for group 1 (vote = 0.29 + 0.18*Tair; Prob < .0001*, n = 622), was significantly different (Prob < .0001*) from that of group 2 (vote = 2.68 + 0.08* Tair; Prob < .0001*, n = 627). ANCOVA analysis showed a significant difference between the two regression lines (for both slope and Y-intercept)
Relation between air temperature (Tair in °C) and thermal comfort vote for group 1 (red) and group 2 (blue) in summer. The analysis of variance and Fisher’s test showed that the linear fit for group 1 (vote = 0.92 + 0.16*Tair; Prob < .0001*, n = 403), was significantly different (Prob < .0001*) from that of group 2 (vote = 1.79 + 0.17*Tair; Prob < .0001*, n = 403). ANCOVA analysis showed a significant difference between the Y-intercepts of the two regression lines
Interestingly, the two lines converge within the range of temperatures typically considered to be comfortable (above 20 °C), and within the previously mentioned comfort range on the perceived thermal sensation scale (intersecting slightly above the neutral point of “4”). This indicates that the two groups experience this range of temperature in a similar way, and that it could be considered as a comfortable outdoor optimum (acceptable for both groups) in winter.
A similar comparison in the summer season (Fig. 8) shows that subjects from group 2 perceive conditions as significantly warmer than representatives of group 1. Here, the two regression lines are approximately parallel, indicating that this relationship is fairly constant over the range of summer temperatures (approximately 19–38 °C), and there is no point at which the comfort levels converge, as there was in the winter—even at 25 °C where they both had identical comfort levels in the winter. As in winter, the average gap between the groups is about one step on the comfort scale.
Differences in thermal comfort responses in location-based group experiments
As mentioned, the single-day group experiments involved a series of four comfort votes, with vote 1 indoors, votes 2 and 3 at different points outdoors, and vote 4 indoors. The four votes were separated by 5-min intervals, and the results of the experimental observations are shown in Fig. 9 for the winter experiment and Fig. 10 for summer.
Comparison of location-based comfort votes by voting session in winter, under varying environmental conditions. Temperatures: vote 1 (indoor) = 19.5 °C; vote 2 (outdoor) = 14.8 °C; vote 3 (outdoor) = 14.7 °C; vote 4 (indoor) = 20.0 °C. (See statistical data in Appendix, Table 2)
Comparison of location-based comfort votes by voting session in summer, under varying environmental conditions. Temperatures: vote 1 (indoor) = 27.0 °C; vote 2 (outdoor) = 30.9 °C; vote 3 (outdoor) = 29.7 °C; vote 4 (indoor) = 28.0 °C. (See statistical data in Appendix, Table 2)
In general, it may be seen once again that there is a clear difference between representatives of the two groups with respect to thermal sensation, with the average comfort vote lower for group 1 than for group 2 in nearly all cases. In winter, this means that group 1 is more sensitive to cold conditions, reporting an average sensation farther from the comfort zone, and in summer, group 2 is shown to be more sensitive to hot conditions with its higher average vote farther from the comfort zone. These differences are especially pronounced for the votes cast in the uncontrolled conditions outdoors, with the two groups showing a relative “equalization” when returning to the climatized indoor space—though in summer the differences persisted indoors as well.
Thus the transition from one thermal setting to another, in this case from indoors to outdoors and back, may (as in the case of vote 4 in winter) or may not (as in vote 4 in summer) act as a factor for leveling off the difference between the groups. It seems that the almost identical voting patterns in vote 4 in winter could be explained by a type of short-term acclimation, since when subjects returned to the comfortable environment of the warm room their reported thermal sensation expressed a similar level of pleasantness regardless of group. In contrast, their return in summer to the indoor space—whose temperature of 28 °C was only 2° lower than outdoors—did not produce such a “universally satisfying” condition and thus resulted in a perpetuation of the differences between the groups.
It is worth mentioning that these inter-group differences in thermal perception do not appear to have been influenced by differences in the clothing worn by participants in the two groups. In order to evaluate the possibility of such an influence, subjects were asked to describe their garments based on standard options, and ANOVA and t test analysis of the responses did not show any significant difference between the groups (at p < 0.05, α = 0.95). The colors of garments were also not significantly different, with both groups reporting an even distribution between neutral and light colors.
Interpretation of the comfort scale by the two groups
Numerous researchers have recognized cultural and linguistic differences in the interpretation of standard comfort scales (Cena et al. 1990; Pitts 2006; Humphreys 2008). In order to examine this possibility, subjects from both groups were asked to provide an answer to the following question: “When you look at each term on the scale, what impression does it give you?” Possible answers comprised the following variants: “preferred,” “comfortable,” “acceptable,” “uncomfortable,” and “very stressful”.
The results of this questioning are shown in Fig. 11 and indicate clear differences between the two groups in terms of their interpretation of the comfort scale. The colder side of the scale is interpreted with a significantly higher level of acceptability by group 2, and conversely the warmer side is interpreted as more acceptable to group 1—among which some representatives treat even the “hot” and “very hot” categories as “acceptable”.
Interpretation of the thermal comfort scale categories by members of the two groups: VS—very stressful, UC—uncomfortable, A—acceptable, C—comfortable, P—preferable. (See statistical data in Appendix, Table 3)
Interestingly, the intermediate voting scale categories of “comfortably warm” and “comfortably cool” receive a large number of “preferred” and “comfortable” ratings, especially from members of group 2. Even the categories of “cool” and “warm” are sometimes interpreted as “comfortable” rather than merely “acceptable”. This reinforces the notion that the zone of “thermal comfort” ranges considerably over both sides of the neutral point among a certain segment of the population.
It is somewhat surprising that the “comfortable” point is not always interpreted as being “preferred,” and for some people is just “acceptable”—which supports the idea that thermal “neutrality” might be treated by some people as a condition which is in fact lacking in interest or stimulation (Heschong 1979; de Dear 2011).
Discussion
It is quite evident that the level of comfort or discomfort experienced by a person at a given time and place depends not only on the physical nature of the surrounding environment, but on the personal circumstances affecting that person’s individual perception of the environment. It is also clear that a better understanding of individual preferences can contribute the design of spaces which are less thermally stressful for those using them. If, however, an individual’s thermal perception is governed by idiosyncratic rather than systematic factors, then it remains beyond the capacity of the designer to account for the non-physical aspects of that individual’s thermal comfort.
In this study, we have attempted to systematically describe the differences in thermal perception that apply not only to individuals, but to groups of individuals who share a common thermal history thanks to the physical and cultural features of environment in which they have undergone long-term acclimation. Drawing on the diverse geographical background of an international student population, we were able to simultaneously compare the thermal responses of two distinct climatocultural groups when the members of those groups were situated in the same physical location. The series of experiments conducted in order to make this comparison was designed to capture both the breadth of thermal sensation over an extended period of time, and the precise differences in thermal sensation that can only be observed in a synchronized setting.
From analysis and interpretation of the results, we observe a strong correlation in each experiment between affiliation to a certain climatocultural group and perceived thermal sensation. The observed correlations indicate that members of group 1—young adults whose primary life experience is from Israel and neighboring countries in the “warm” eastern Mediterranean region—are better adapted to hot summer conditions than members of group 2, whose life experience is from cold regions such as northern Europe. Conversely, group 2 is better adapted to cold winter conditions. This “adaptation” is expressed by fact that on average, group 1 perceives summer conditions as less “hot” and more “comfortable” than does group 2, and the opposite is evident in winter. We interpret these differences in perceived thermal sensation to result primarily from divergent expectations, which are deeply engrained due to long-term thermal acclimation.
While these findings may not be surprising, it is significant that statistically clear patterns which conform to our theoretical assumptions do in fact emerge from several independent sets of data. Clearly these differences in group thermal perception cannot be identified by observing individual group members or groups which are too small or heterogeneous. By comparing two distinct groups, each of which exhibits a high level of internal homogeneity, and by performing this comparison in a spatially and temporally synchronous setting, we may attribute a certain degree of confidence to the patterns identified.
At the same time, we see evidence that these patterns expressing long-term acclimation may be overridden by strong acclimation processes in the short-term. This was seen when respondents returned from outdoor voting in winter to the sharply contrasting environment of a heated room, which apparently nullified the differences in thermal sensation that the two groups had experienced previously. In fact the balance of evidence from the various experiments suggests that perceptual differences between groups are diminished when conditions are seen as universally comfortable (i.e., in the heart of the “comfort zone”) rather than open for interpretation, and it is possible that these differences would also diminish when conditions become sufficiently extreme to be perceived as universally uncomfortable.
Our empirical results also indicate that the differences in reported thermal sensation between groups are not just physiological (i.e., due to the reception of thermal signals by the body’s sensory mechanisms) or even psychological (i.e., due to the cognitive interpretation of these signals as “hot” or “cold”). Rather they seem to also reflect cultural differences in the interpretation of the terms used to describe these sensations, i.e., the acceptability of “warm” or “cool” as preferred states. This finding could have methodological significance since thermal comfort research is often based on questionnaires using a verbal comfort scale to represent the thermal preferences of respondents.
While it might be expected that such cultural differences would be manifested in actual adaptive behavior, we did not find evidence of this in terms of the clothing worn by participants in the study—which did not reflect any significant differences between groups. This lack of differentiation may reflect a type of cultural adaptation operating over a relatively short time scale.
There is ample evidence that the meanings of “comfort” are continuously changing, and that what is considered comfortable in a particular culture may vary over time. Still, understanding differences in thermal perception due to climatocultural factors and long-term conditioning can be useful for improving thermal comfort models and formulating design guidelines that are appropriate for different contexts (Chappells and Shove 2004). This is especially relevant for pedestrian comfort in outdoor spaces—which has historically received less attention than indoor comfort, but which may have substantial consequences for the long-term sustainability of a rapidly urbanizing human society in a changing global environment.
Conclusions
Knez and Thorsson (2006) argue that “different geographical/climatic zones can be defined as different cultures.” Recognizing that the deeply intrinsic mutual relationships between cultural norms and physical environments can shape not only attitudes and expectations, but also perceptions, they suggest the existence of “different environment-related behavioural, emotional and cognitive consequences related to thermal comfort assessments of outdoor environments for persons living in different cultures” (Knez and Thorsson 2006, p. 259). In this paper, we have offered experimental evidence supporting the assertion that different climatocultural backgrounds may indeed be instrumental in causing differences in thermal perception.
Our findings allow us to conclude that people with disparate climatic origins do indeed undergo long-term thermal acclimation, and as a result may perceive “warm” and “cold” seasonal conditions in contrasting ways. Results of the long-term experiment make it clear that in winter, thermal perceptions of those acclimated to a cold climate are closer to neutrality than those acclimated to a warm climate, and in summer, those with a warm-climate acclimation perceive discomfort far less than cold-climate subjects. These conclusions are further strengthened by results of the location-based experiment, which illustrate that inter-group differences in thermal perception are prominent even when observed under identical conditions—perhaps the strongest indication that climatocultural adaptation is a tangible and even quantifiable phenomenon.
The finding that different groups also interpret the meaning of the comfort scale in different ways adds a layer of nuance to these conclusions, but does not contradict them. In the “Introduction” section, we touched upon the dynamic complexity that characterizes thermal perception. Such complexity is marked by non-linearity (when effects are not directly proportional to causal elements); dynamicity (when changes occur over time); interrelated and often delayed feedback loop interactions (more precisely, the network of such interactions); path dependency (when past matters, so the talk about climatocultural origins is highly relevant for thermal perception); emergence (when new features in systems arise), and other complex mechanisms (Byrne and Callaghan 2014). The domains of thermal comfort and thermal perception fully follow the logic (or the lack thereof) of complex dynamic systems.
While fully acknowledging the intricate complexity and uncertainty embedded in the mechanism of thermal perception, we nevertheless advocate for its further study. It is precisely these multiple layered and dynamically complex interactions that can, and should, challenge researchers to develop more sophisticated research designs and approaches to data interpretation. For example, choice experiments (involving choices or actions, rather than stated preferences or descriptions) could remove the linguistic mediation that might be at play, while multi-level modeling might partition variance in thermal comfort between not only physical and climatocultural components, but, also, individual ones.
We consider that the burgeoning field of research into subjective thermal perception is of practical importance, especially in the light of growing globalization and cultural diversity of our cities. Thus, according to Kenawy and ElKadi (2011), in order to make urban open spaces successful, this diversity should not be neglected. They argue that “cultural diversity becomes one of the new variables that should be added to the subjective nature of thermal comfort. It is important that the urban planner take into consideration the thermal comfort of a wide range of people from different cultural background…” (Kenawy and ElKadi 2011, p 246). So, our paper is an invitation for the next round of research to unpack the components and dynamics within this “black box” of sociocultural and past-dependent groupings.
References
Auliciems A (1981) Towards a psycho-physiological model of thermal perception. Int J Biometeorol 25:109–122
Brager GS, de Dear RJ (2003) Historical and cultural influences on comfort expectations. In: Cole R, Lorch R (eds) Buildings, culture and environment: informing local and global practices. Blackwell, London, pp 177–201
Brennan L, Binney W, Parker L, Aleti T, Nguyen D (2014) Social marketing and behaviour change: models, theory and applications. Edward Elgar Publishing, Cheltenham
Byrne D, Callaghan G (2014) Complexity theory and the social sciences: the state of the art. Routledge, London
Cena KM, Ladd PG, Spotila JR (1990) A practical approach to thermal comfort surveys in homes and offices: discussion of methods and concerns. ASHRAE Trans 96(1):853–857
Chappells H, Shove E (2004) Comfort: a review of philosophies and paradigms. Future Comforts Project, UK ESRC programme. http://www.lancs.ac.uk/fass/projects/futcom/fc_litfinal1.pdf. Accessed 22 Feb 2017
de Dear RJ (2010) Thermal comfort in natural ventilation – a neurophysiological hypothesis. In: Proceedings of Conference: Adapting to Change: New Thinking on Comfort, Cumberland Lodge, Windsor, UK, 9–11 April 2010
de Dear RJ (2011) Revisiting an old hypothesis of human thermal perception: alliesthesia. Build Res Inf 39(2):108–117
de Dear RJ, Brager GS (1998) Developing an adaptive model of thermal comfort and preference. ASHRAE Trans 104(1):145–167
de Dear RJ, White S (2008) Residential air conditioning, thermal comfort and peak electricity demand management. In: Proceedings of Conference: Air Conditioning and the Low Carbon Cooling Challenge, Cumberland Lodge, Windsor, UK, 27–29 July 2008
Golany GS (1996) Urban design, morphology and thermal performance. Atmos Environ 30(3):455–465
Gosling SN, Bryce EK, Dixon PG, Gabriel KMA, Gosling EY, Hanes JM, Hondula DM, Liang L, Mac-Lean PAB, Muthers S, Nascimento ST, Petralli M, Vanos JK, Wanka ER (2014) A glossary for biometeorology. Int J Biometeorol 58:277–308
Heschong L (1979) Thermal delight in architecture. MIT Press, Cambridge
Höppe P (2002) Different aspects of assessing indoor and outdoor thermal comfort. Energ Build 34:661–665
Humphreys MA (2008) Why did the piggy bark? Some effects of language and context on the interpretation of words used in scales of warmth and thermal preference. In: Proceedings of Conference: Air Conditioning and the Low Carbon Cooling Challenge, Cumberland Lodge, Windsor, UK, 27–29 July 2008
Humphreys M, Nicol F (1998) Understanding the adaptive approach to thermal comfort. ASHRAE Transactions, Symposia, pp 991–1004
Indraganti M, Rao KD (2010) Effect of age, gender, economic group and tenure on thermal comfort: a field study in residential buildings in hot and dry climate with seasonal variations. Energ Build 42(3):273–281
Kenawy I, ElKadi H (2011) Diversity and thermal comfort in outdoor places. International Journal of Diversity in Organisations 11(1):237–248
Knez I, Thorsson S (2006) Influences of culture and environmental attitude on thermal, emotional and perceptual evaluations of a public square. Int J Biometeorol 50:258–268
Knez I, Thorsson S (2007) Thermal, emotional and perceptual evaluations of a park: cross-cultural and environmental attitude comparisons. Build Environ 43(9):1483–1490
Lin T, de Dear RJ, Hwang R (2011) Effect of thermal adaptation on seasonal outdoor thermal comfort. Int J Climatol 31(2):302–312
Middel A, Selover N, Hagen B, Chhetri N (2016) Impact of shade on outdoor thermal comfort – a seasonal field study in Tempe, Arizona. Int J Biometeorol 60:1849–1861
Nakano J, Tanabe S, Kimura K (2002) Differences in perception of indoor environment between Japanese and non-Japanese workers. Energ Build 34:615–621
Ndetto EL, Matzarakis A (2017) Assessment of human thermal perception in the hot-humid climate of Dar es Salaam, Tanzania. Int J Biometeorol 61:69–85
Nicol JF, Humphreys MA (2002) Adaptive thermal comfort and sustainable thermal standards for buildings. Energ Build 34:563–572
Nikolopoulou M, Steemers K (2003) Thermal comfort and psychological adaptation as a guide for designing urban spaces. Energ Build 35:95–101
Pearlmutter D (2007) Architecture and climate: the environmental continuum. Geography Compass 1(4):752–778
Pearlmutter D, Jiao D, Garb Y (2014) The relationship between bioclimatic thermal stress and subjective thermal sensation in pedestrian spaces. Int J Biometeorol 58:2111–2127
Pitts A (2006) The languages and semantics of thermal comfort. In: Proceedings of NCEUB conference: comfort and energy use in buildings – getting them right, Cumberland Lodge, Windsor, UK, 27–30 April 2006
Plous S (1993) The psychology of judgment and decision making. McGraw Hill, New York
Roaf S (2006) Comfort, culture and climate change. In: Proceedings of NCEUB conference: comfort and energy use in buildings – getting them right, Cumberland lodge, Windsor, UK, 27–30 April 2006
Saaroni H, Pearlmutter D, Hatuka T (2015) Human-biometeorological conditions and thermal perception in a Mediterranean coastal urban park. Int J Biometeorol 59:1347–1362
Schiller S, Evans JM (1998) Sustainable urban development: design guidelines for warm humid cities. Urban Design International 3(4):165–184
Spagnolo J, de Dear RJ (2003) A field study of thermal comfort in outdoor and semi-outdoor environments in subtropical Sydney Australia. Build Environ 38:721–738
Sterman J (2000) Business dynamics: systems thinking and modeling for a complex world. Irwin/McGraw-Hill, Boston
Stoops J (2002) An illustration of expectation differences in office thermal comfort. ACEEE Summer Study, Asimolar
Triandis HC (1994) Culture and social behavior. McGraw-Hill, New York
van Hoof J (2008) Forty years of Fanger’s model of thermal comfort: comfort for all? Indoor Air 18:182–201
Wilhite H, Nakagami H, Masuda T, Yamaga Y, Haneda H (1996) A cross-cultural analysis of household energy-use behaviour in Japan and Norway. Energy Policy 24(9):795–803
Yamtraipat N, Khedari J, Hirunlabh J (2005) Thermal comfort standards for air conditioned buildings in hot and humid Thailand considering additional factors of acclimatization and education level. Sol Energy 78:504–517
Yao R, Li B, Liu J (2009) A theoretical adaptive model of thermal comfort – adaptive predicted mean vote (aPMV). Build Environ 44:2089–2096
Zhang Y, Zhao R (2008) Overall thermal sensation, acceptability and comfort. Build Environ 43:44–50
Zhang H, Huizenga C, Arens E, Yu T (2001) Considering individual physiological differences in a human thermal model. J Therm Biol 26:401–408
Acknowledgements
The authors would like to thank Wolfgang Motzafi-Haller for his skill and knowledge in installing and maintaining the measuring equipment.
Author information
Authors and Affiliations
Corresponding author
Rights and permissions
About this article
Cite this article
Brychkov, D., Garb, Y. & Pearlmutter, D. The influence of climatocultural background on outdoor thermal perception. Int J Biometeorol 62, 1873–1886 (2018). https://doi.org/10.1007/s00484-018-1590-7
Received:
Revised:
Accepted:
Published:
Issue Date:
DOI: https://doi.org/10.1007/s00484-018-1590-7