Abstract
Key message
Chinese fir has strong transpiration and assimilation activity. Daily stem radial increments were closely related to moisture conditions. Tree growth responds to a climate in similar ways but with different magnitudes.
High temporal resolution dendrometric measurements offer a great opportunity to acquire valuable information about stem size dynamics at half-hour intervals and improve our understanding of climate influence on tree growth and physiological processes. In the present study, we continuously monitored intra-annual stem radial size changes with the help of automated dendrometers in pure Masson pine and Chinese fir forests widely distributed in subtropical China across two consecutive years of 2017 and 2018. The main goal of this study was to analyze intra-annual stem growth dynamics as well as their climatic forcing. Our results indicate a similar growth onset between Masson pine and Chinese fir but prolonged growth duration for Chinese fir in 2018. Large stem-size fluctuations with particularly strong stem shrinkage especially in summer were obtained for Chinese fir. The analysis of climatic forcing on daily stem radial increments indicated similar correlation trends with different magnitudes for the two species, with changes in the daily stem growth positively correlated with relative humidity (RH), precipitation, and soil water content (SWC), and negatively correlated with photosynthetically active radiation (PAR), vapor pressure deficit (VPD) and temperature factors. In general, daily stem radial growth is closely associated with moisture conditions. Lagging the climatic factors showed decreasing impacts on daily stem growth. We conclude that tree growth is primarily responsive to moisture-related climatic factors, and Chinese fir shows more robust transpiration and assimilation activity.
Similar content being viewed by others
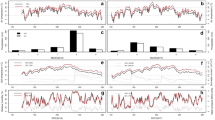
Avoid common mistakes on your manuscript.
Introduction
Subtropical forests in southern China play an important role in the dynamics and coupling of global carbon and hydrological cycles (Fang et al. 2001; Huang et al. 2018; Sun et al. 2019), with high forest coverage and a diverse range of vegetation types (Li et al. 2009; Yang et al. 2019). The subtropical forest also has great value in maintaining the regional economic sustainability of rural populations. However, subtropical forests face a major challenge from global climate change, making the quantification of tree growth and its climate response imperative.
Differential response of tree species to climate may alter the role of forests in future global biogeochemical cycles (Harvey et al. 2020). Tree growth has been described as an effect of control by limiting factors of the environment (Downes et al. 1999; Fritts 1976). Predicting how tree growth and forest productivity would respond to gradually changing climate at local scales will benefit from understanding seasonal climatic influences. Information on the climate sensitivity of tree growth, which is crucial for appropriate management approaches, is therefore urgently needed (Liu et al. 2018; van der Maaten et al. 2018). At present, numerous dendroclimatological studies have adopted tree-ring technologies to explore tree growth mechanisms responding to climate using monthly climatic data and annual tree-ring width (e.g., Harvey et al. 2020; Yu et al. 2018). Due to the typical annual resolution of dendroclimatology, the response of tree growth to climate was generally assessed at the inter-annual time scale. However, cambial activity and xylem enlargement occur on a short time-scale (Deslauriers et al. 2008; Huang et al. 2018), which is distinctly different from traditional dendroclimatological studies in terms of time resolution. Improved understanding of climatic drivers of tree growth can greatly benefit from intra-annual scale data and studies.
Dendrometers are widely used instruments that are installed on stems to obtain gross stem size (Fritts 1976). High-resolution dendrometer measurements can provide valuable information on tree stem radial variation by continuously monitoring stem radial variations at sub-hour and micrometer resolution, making it possible to study tree response to climatic influences at a high temporal resolution (Aldea et al. 2018). In contrast with stem wood anatomical approaches for studying intra-annual tree growth dynamics, such as the weekly micro-cores sampling method (Huang et al. 2018), continuous-measurement dendrometers record stem radial changes without an invasive sampling of the cambium and trunk (Deslauriers et al. 2011; Duchesne and Houle 2011). Therefore, high-resolution dendrometers are particularly suitable for long-term monitoring of tree growth, which ensures the recorded results are based on natural responses of trees to past and current factors (Gliniars et al. 2013). Physiologically, the recorded stem radial variations contain both irreversible periods of cambium growth as well as reversible periods of stem shrinkage and swelling related to water changes (Bouriaud et al. 2005; Deslauriers et al. 2003; Downes et al. 1999).
Automatic dendrometers are now commonly used in forest ecological studies. For example, dendrometers have been used for studying daily stem radial variations and seasonal growth dynamics of various tree species driven by climate around the world (Aldea et al. 2018; Fritts 1976; Smiljanic and Wilmking 2018; Wang et al. 2016). Furthermore, dendrometer analyses also focus on the long-term water status of plants (Barraclough et al. 2018; Schaefer et al. 2019; Zweifel and Hasler 2001). Thus, dendrometers have been shown to provide important insights into water movement and storage in plants, growth phenology, and climatic effects on stem size dynamics. Despite its valuable characteristics, dendrometers are usually installed on individual tree species with a limited number of trees due to the high cost, hindering comparison between species that might have different phenologies and physiologies (van der Maaten et al. 2018).
In the present study, we seek to understand the growth dynamics and climatic forcing in Masson pine (Pinus massoniana Lamb.) and Chinese fir (Cunninghamia lanceolata Hook.), which are the main afforestation and local native tree species widely distributed in subtropical China (Yu et al. 2003). Masson pine has played a critical role in vegetation and soil restoration over the past several decades because of its valuable ecological traits, such as high survivability and fast-growth in nutrient-impoverished soils (Huang et al. 2018; Kuang et al. 2008). Chinese fir, which is unique to subtropical China, is a vital commercial tree species with high growth rates and timber quality, creating considerable economic value (Chen et al. 2013; Huang et al. 2019). The planting history can be dated back a thousand years. With the large scale of afforestation and reforestation, the forest area of Chinese fir is increasing rapidly, mostly in plantations (Zhang et al. 2004). Understanding continuous stem size dynamics and the intra-annual growth response to climatic variation is crucially important for afforestation design and sustainable forest management under changing climate. However, stem radial variation on short time scales and the corresponding response to climatic factors are little known because of the lack of long-term monitoring. Furthermore, the species differences in radial variation patterns remain unexplored.
Consequently, this study was designed to use high-resolution dendrometers to monitor stem radial variations of Masson pine and Chinese fir over 2 years (2017–2018) in subtropical China. The specific goals were 1) to determine interspecific differences in intra-annual stem radial variation cycles and growth dynamics (onset, duration, and cessation); 2) to examine the major climatic factors driving species-specific stem radial growth.
Materials and methods
Study site description
This study was conducted at the Qianyanzhou (QYZ) ecological research station, in the south-central Jiangxi Province, subtropical China (115° 3′ 37.1″ E, 26° 45′ 1.5″ N). The area has a distinct subtropical monsoon climate. The average annual temperature from 2009 to 2018 was 19.4 °C, with daily maximum average temperatures of the warmest month and daily minimum average temperatures of the coldest month of 35.8 °C and 3.1 °C, respectively (Fig. 1). The annual precipitation ranges from 1100 to 1760 mm with a mean value of 1446 mm, and the lowest precipitation in early spring and autumn (Fig. 1). The forest is dominated by evergreen coniferous species such as Masson pine, Chinese fir, and slash pine (Pinus elliottii). The substrate is predominantly ultisols. The shrub layer mainly includes Loropetalum chinense, Camellia oleifera, and Vitex negundo var. cannabifolia, among others.
Climograms for the period of 2009 to 2018 of the study site. Numbers to the left of the y-axis indicate daily maximum average temperatures of the warmest month and daily minimum average temperatures of the coldest month, from top to bottom, respectively. Bars below the x-axis represent probable frost season (blue green), and frost-free season (no bars)
Data collection
Stem radial variation measurements
Four upright and healthy trees per species from pure Masson pine and Chinese fir forests were randomly selected for the installation of high-resolution automatic band dendrometers. We assumed the same climatic conditions for the two forests that are 5 km apart. The two forests were located on the southeastern and southern slope with an elevation of 92 m a.s.l. and 120 m a.s.l., respectively. The monitored Masson pine and Chinese fir trees respectively had an average diameter at breast height of 19.5 ± 1.4 cm and 14.3 ± 2.1 cm, tree age of 31 ± 3 years and 24 ± 2 years. In July 2016, we installed band dendrometers Type DC2 (range 15 mm, accuracy ± 2 μm, temperature coefficient < 0.1 μm/K, Ecomatik, Munich, Germany) in the selected trees to measure stem radial changes at breast height. Parts of the outermost bark were carefully removed to avoid damaging cambium and to reduce the influences of expansion and shrinkage due to changes in air humidity. Stem radial variations were automatically recorded every 30 min and stored in data loggers HOBO DL18. Measurements recorded in 2017 and 2018 were used in the analyses of this study. All raw data were converted to radial variations with an assumption that the selected tree stem is circular.
Climatic data records
To assess weather conditions in 2017 and 2018, daily meteorological data including daily average, maximum and minimum temperature (Tave, Tmax, Tmin, oC), total precipitation (Pre, mm), relative humidity (RH, %), photosynthetically active radiation (PAR, μmol/(m2·s)) were obtained from the QYZ climatic station. Soil temperature (ST) and soil water content (SWC) were acquired on-site at 10-cm depth using a small automated weather station. All raw records were at a half-hour scale. The daily vapor pressure deficit (VPD, kPa) was computed by air temperature (T) and RH using the following equation (Zhang et al. 2016):
Data analysis
To illustrate the daily pattern of dendrometer cycles, which is analogous to a sinusoidal wave (King et al. 2013), we calculated the differences between radial size series and the first value on a specific day, i.e., the first value of a day was artificially set to zero for all individual trees. Monthly mean half-hour data of stem radial variations were calculated by averaging the half-hour data during the same month across trees and years. From these waves, monthly mean daily maximum and minimum, as well as their time of occurrence was obtained.
The Gompertz equation is one of the most appropriate sigmoidal models to describe growth processes due to its inherent flexibility (Duchesne et al. 2012; Zeide 1993), and is commonly adopted in many dendrometer studies (eg., Deslauriers et al. 2003; Tian et al. 2017). To determine the growth onset and cessation of the two species, we first fitted the Gompertz equation to the daily averaged raw measurement data for individual trees and year. The form of the Gompertz equation including a parameter (Y0, the lower asymptote) that is indicative of the initial stem status at the beginning of the growing season to avoid arbitrary choices in the initial settings of the raw dendrometer data is given as:
where Y is the daily radial variations; A is the upper asymptote; β is the x-axis placement parameter; \(\kappa\) is the rate of change parameter; t is the time in days.
After fitting Gompertz equations, we computed their derivatives, i.e., the function of growth rates. The timing of growth onset and cessation was identified according to the dendrometer accuracy. More specifically, the growth onset and cessation dates were defined as the DOY (day of year) when growth rates exceeded and fell below 2 μm per day, respectively (Liu et al. 2018), and growth duration was calculated as the number of days between growth onset and cessation.
Raw dendrometer cycles comprise irreversible stem radial growth and a combination of water-related reversible stem expansion and shrinkage. To explore the climatic forcing of intra-annual tree stem growth, we need to extract the growth-induced expansion of the stem from raw data for each tree and year. The zero growth (ZG) concept by Zweifel et al. (2016) was applied to separate water-related and growth-related variations, which assumes that growth starts when stem radius exceeds the previous maxima and ends when the stem starts shrinking again (Fig. 2). More specifically, a stepwise zero-growth baseline as displayed in Fig. 2 was extracted by moving daily maxima to the next equal stem radius (Liu et al. 2019). Consequently, it is assumed that no growth happens during periods of stem shrinkage (Knusel et al. 2019).
Half-hourly stem radius changes (high-frequency fluctuations) in 2017 and 2018 for 4 trees of each species with cumulative stem growth (rising stepped curves) deduced according to the ZG concept (Zweifel et al. 2016) which assumes no growth-induced irreversible stem expansion during periods of shrinkage
Additionally, day-to-day stem radial increments were computed by calculating the difference between two consecutive days using continuous daily stem growth data following the ZG concept (Zweifel et al. 2016). To remove individual long-term growth trends and make daily stem variations comparable among trees, a min–max normalization (0–1 normalization) method was used. Namely, the difference between actual daily growth and year-round minimum value is divided by the difference between year-round maximum and minimum daily variations. The removal of growth trends makes it possible to assess the sensitivity and responses of trees to external environments, avoiding the effects of different absolute growth rates. We, therefore, made climate-growth analyses by correlating the averaged normalized daily stem radial growth against the climatic factors to investigate the climatic forcing pattern throughout the main growing season across the two years. Specifically, Spearman correlation coefficients between diurnal stem radial growth and diurnal climatic factors as well as diurnal stem radial increment and previous-day climatic factors were calculated. The climatic factors lagged up to 2 days were used to illustrate hysteresis effects. Furthermore, moving-window Spearman correlation analysis was used to examine the possible seasonality of climate-growth relationships. The moving window was thereby set to 21 days.
All statistical analyses and graphing were performed using the R software (version 3.6.1, R Core Team).
Results
Variations of meteorological factors
Climatic conditions were similar between the two studied years (Fig. 3). The annual Tave in 2017 and 2018 were comparable, with values of 18.6 ± 8.0 and 18.7 ± 8.6 °C, respectively. Monthly mean air temperature ranged from 7.7 (December) to 28.6 °C (July), and from 6.1 (January) to 28.9 °C (July), respectively. Maximum air temperatures exceeded 38 °C in June or July (Fig. 3a, b). The annual total precipitation in the two years was 1418 and 1400 mm, respectively. From March to August, the amounts of precipitation in 2017 were higher than in 2018, with values of 1049 and 890 mm, respectively. The difference between years was caused by more precipitation in March and June in 2017. RH ranged from 63.8% to 100% in 2017 and from 50 to 100% in 2018. The variation trends of vapor pressure deficit are inverse to relative air humidity, with values ranging from 0 to 0.90 kPa in 2017 and from 0 to 1.45 kPa in 2018 (Fig. 3c, d). PAR showed a normal distribution shape with higher values in the mid-year and lower values at the beginning and end of the year.
Soil temperatures varied with air temperatures but with lower diurnal amplitudes. The paired-samples Wilcox test showed that soil temperatures at 10 cm depth were significantly higher in Masson pine forests in both years, while there was no significant difference between the two years for these species. In contrast, soil water content showed a significant difference between both species and years. The average soil water content in the Chinese fir forest was higher in the two years, which is opposite to soil temperature (Fig. 4).
Soil temperature (a) and soil water content (b) at 10 cm depth for Masson pine and Chinese fir forests during 2017 and 2018. The length of the box indicates the 25th–75th percentile, the vertical lines attached to the box represent the minimum and maximum values, the dot inside the box represents the mean value, the horizontal line inside the box represents the median, outliers are marked as asterisks. The violin represents a probability density. ns indicates non-significant (p > 0.05), *** indicates highly significant (p < 0.001)
Stem growth dynamics and seasonal growth pattern
The annual variability of stem radius is characterized by rapid growth in the spring, followed by a progressive rise in summer, a fluctuating plateau in autumn, and a slight decrease during the winter months (Fig. 2). Although individual trees followed highly similar growth patterns within years and species, differences existed in cumulative growth (Fig. 2), e.g., Chinese fir showed distinctly larger annual increments in 2017. Relatively constant annual growth was observed for Masson pine, whereas higher growth variation was found in Chinese fir over the two years.
The daily accumulated stem radial growth modeled using a Gompertz function and derived growth rates are displayed in Fig. 5. The fitting results showed intra-annual accumulated stem growth of each tree across species and year can be well explained by the Gompertz function. The time of growth onset and cessation, and cumulative annual increments describing the stem growth progress are presented in Fig. 6. On average, both species synchronously started growth at approximately DOY 30 (Jan 30) in 2017, whereas the initiation in 2018 was 6 days earlier for Masson pine and 10 days later for Chinese fir. The ending time of Masson pine was at approximately DOY 227 (Aug 15) and the cessation was 113 days later for Chinese fir in 2017. In contrast, the cessation was relatively consistent (DOY 257 and 263, Sep 14 and 20) for the two species in 2018. Consequently, the average duration of the growing season was 196 and 231 days for Masson pine, 310 and 222 days for Chinese fir, in 2017 and 2018, respectively. The average annual stem radial increments of the two years were approximately 1.7 and 1.9 mm for Masson pine, 3.6 and 2.1 mm for Chinese fir, respectively. Relative fast stem growth occurred in April and May. The main overlapped period of stem growth was from March to mid-August, as the main growth period.
Stem growth modeled by Gompertz function (a) and derived daily growth rate (b) for Masson pine and Chinese fir, respectively. Shaded regions indicate main growth periods. Dots and lines in top panels indicate daily means of communicative stem growth and Gompertz function fitted curves, respectively. The dashed line in the bottom panels represents stem growth rates equal to 2 μm per day, corresponding to the dendrometer accuracy
Growth onset time, cessation time, duration, and annual growth in 2017 and 2018 for Masson pine and Chinese fir, respectively. The length of the box indicates the 25th–75th percentile, the vertical lines attached to the box represent the minimum and maximum values, the dot inside the box represents the mean value, the horizontal line inside the box represents the median, and outliers are marked as asterisks. ** and *** indicates significance level at p < 0.01 and p < 0.001 by paried t test, respectively
Monthly mean daily radial cycles over a full year reveal variations among months and species (Fig. 7). The amplitude and phase of these cycles varied along the course of 12 months with conspicuous differentiation between hot and cold seasons, i.e., summer and winter. Hot months have much larger amplitudes than cold months. Summer and winter are characterized by an inconspicuous phase shift in the daily cycle. During summer days, local maximum values of Masson pine and Chinese fir occur at around 04:00 and 08:00, respectively, followed by local minima between 15:00 and 17:00 for both species. During winter days, both species showed local maxima and minima between 09:00 and 11:00 and between 17:00 and 19:00, respectively. A comparison of the two species showed a large fluctuation of daily radial cycles for Chinese fir, suggesting that Chinese fir trees are more sensitive to tree water deficit during spring than Masson pine. In particular, high shrinkage was observed during the hot months. The year-round variation patterns contain daily amplitude maxima in August and minima in December for Masson pine, and maxima in September and minima in December for Chinese fir, respectively.
Correlations between growth and climate
During the main growth periods of both species in both years, we studied the climatic forcing of daily stem growth through calculating correlation coefficients between averaged normalized daily stem radial increment and climatic data. Highly similar correlation patterns between both species and years were obtained in the present study (Fig. 8). Diurnal correlation patterns indicated that daily stem increment was significantly associated with Tmax (negative), precipitation (positive), RH (positive), PAR (negative), VPD (negative), SWC (positive) for both species in 2017 and 2018, while significant negative associations with Tave, Tmin, ST were observed for Masson pine in 2017 and 2018, and Chinese fir in 2018. When considering the lag effects, the strength of correlation patterns generally declined and seldom changed direction.
Spearman correlations between normalized daily stem radial increments (calculated according to ZG concept by Zweifel et al (2016)) of Masson pine and Chinese fir, and climatic factors. Coefficients are calculated for the main growth periods (March to mid-August) of 2017 and 2018, respectively, while considering lag-effects up to 2 days (indicated by different colors). Gray dashed lines represent the significance at the 0.05 level
To further understand the prominent climatic factors affecting stem growth, moving-window correlation analyses (21 days) were applied to calculate the correlation between the averaged normalized daily stem radial increment and the current-day climatic data (Tmax, precipitation, RH, PAR, VPD, and SWC) in 2017 and 2018 (Fig. 9). The correlation results demonstrated the above-mentioned high coincidence in the climatic forcing of the changes in the daily stem radius of the two species. In both years, stem radial growth changes of both species were positively correlated with precipitation and RH, negatively correlated with PAR and VPD, but no continuous correlations with Tmax and SWC during the main growth periods. Notably, in 2017 a long period of strong positive correlations with RH and precipitation and strong negative correlations with PAR and VPD was found for Masson pine. Between the two years, similar climatic responses can be found, especially with RH, PAR, and VPD for Chinese fir during March and mid-August.
Moving-window correlations (21 days window) between normalized daily stem radial increments (calculated according to ZG concept by Zweifel et al (2016)) of Masson pine and Chinese fir, and selected diurnal climatic factors with highest correlation coefficients in Fig. 8. Gray dashed lines represent the significance at the 0.05 level. The discontinuous line of Masson pine is caused by consecutive zero values
Discussion
Automated high-resolution dendrometers can provide valuable information about actual growth in tree diameter (irreversible cycle) and water status (reversible hydrological cycle) (Aldea et al. 2018; Zweifel et al. 2005). In the present study, we monitored Masson pine and Chinese fir for two years to analyze stem radial variation cycles and their climate responses across species and years. Our results found differences in stem radial growth dynamics and variation cycles across species and years. However, the climatic forcing of stem radial growth was highly similar across species and years.
Seasonal patterns of stem radial growth
The growth rates and/or annual increments of Masson pine and Chinese fir showed large differences in 2017 but high similarity in 2018. The distinct differences may result from Chinese fir having a faster recovery from tree water deficit or short-lived drought (Guney et al. 2020). From Figs. 2 and 9, we found Masson pine endures a long period of zero values representing no growth while Chinese fir had discrete–continuous growth indicating better recovery during the same period. Furthermore, transpiration and assimilation activity is assumed to be high when daily shrinkage is high (Guney et al. 2020). As displayed in this study, Chinese fir has a substantial daily stem radial fluctuation and large daily shrinkage (Fig. 7). When considering external factors, the stand of Chinese fir may grow better on more fertile sites. In contrast, Masson pine is always a pioneer tree species of barren sites. Under extreme conditions, Masson pine trees may maintain physiological processes primarily with very low growth rates.
Stem radius began to increase in late January to early February, which is earlier than micro-coring results of Masson pine in south subtropical China, where xylem activation started in mid to late February (Huang et al. 2018). The discrepancy may be attributed to differences in measurement methods or microclimates. Since the micro-core method can monitor tree growth at the cell level, intra-annual stem dynamics through dendrometers in combination with regular micro-cores could move us to better document and understand the mechanisms by which climate modulates wood formation and tree stem growth (Cocozza et al. 2016).
In comparison with Masson pine, growth onset approximately 2 weeks later was observed for Chinese fir in 2018. We thought that could be explained in terms of temperature thresholds. Since threshold temperature is a limiting factor favoring the beginning of xylogenesis (Huang et al. 2018; King et al. 2013; Saderi et al. 2019), we inferred that the lower soil temperature before growth onset in Chinese fir forests may delay the onset. Low soil temperatures reduce enlargement and turgor of xylem cells due to inhibition in water uptake and tree transpiration (Liu et al. 2016, 2018).
Growth cessation for Masson pine was much earlier than Chinese fir in 2017 but similar in 2018, which resulted in differences in growth duration. Duchesne et al. (2012) showed that climatic factors cannot adequately account for the growth cessation of individual trees, which may be mostly determined by complicated intrinsic tree physiological processes, like cell division, differentiation, and death. However, a micro-coring study showed that the cessation of wood formation is likely to be influenced by environmental factors, such as photoperiod, with drought stress hastening it (Saderi et al. 2019).
Monthly mean daily stem size variations showed clear increasing amplitudes of the daily cycles in summer days with high temperature and protracted sunshine. In line with this finding, other high-resolution dendrometer studies also report that daily maximum amplitudes appear in hot months (e.g., King et al. 2013; Wang et al. 2016). Higher daily mean air temperature and greater amounts of radiation promote transpiration (McKenney and Rosenberg 1993), leading to increased canopy water demands. However, because of the lag in water absorption responding to the onset of transpiration and the lag prolonged with increasing distance between crown and stem base, an individual tree may meet water needs by consuming its water storage in elastic tissues of the bark and rigid xylem (Zweifel and Hasler 2001), thus causing large daily variations.
Larger fluctuations of monthly mean daily cycles in Chinese fir (Fig. 7) may suggest that during a daily period, Chinese fir expend their internal stem water reserves more quickly than Masson pine (King et al. 2013). On the other hand, Chinese fir may have higher transpiration supporting the larger annual increment. Additionally, Chinese fir may store less water in internal issues than Masson pine, which would be consistent with the fact that Masson pine has a better ability to tolerate drought and to retain more water (Yu et al. 2003).
Climatic driving daily stem growth
In addition to similar growth patterns and daily stem size variations, the climatic forcing of daily stem dynamics was also particularly similar for the two studied species (Figs. 8 and 9). The normalized daily stem radial increment in association with diurnal climatic factors gave significant correlations. The strongest positive relationships were found for RH and precipitation, and the strongest negative relationships were observed for VPD, PAR, and Tmax. In line with previous dendrometer studies (Deslauriers et al. 2003; Duchesne and Houle 2011; van der Maaten et al. 2018), stem radial growth is therefore expected to be maximal during periods of abundant precipitation and higher humidity as well as during periods of lower temperature and PAR. Furthermore, the differences in moving correlation results between the two years are possibly caused by specific climate conditions.
The highest positive correlations were found with RH, whereas the strongest negative correlation tended to be with VPD, especially during the rapid growing periods (Fig. 9). RH and VPD are both influenced by precipitation and temperature, representing direct metrics of moisture conditions (Zhang et al. 2016). Air moisture is therefore regarded as the major factor affecting stem radial dynamics for the two species in subtropical China. With the increase of temperature and solar radiation after 06:00 am, RH begins to decline, as clearly illustrated in Fig. 10 using three-day data series. A decrease in RH together with an increase in VPD are likely to gradually enhance tree transpiration and photosynthesis and promote upward water transportation, thus inhibiting cell enlargement and expansion because of a drop in cell turgor pressure of the stem (Deslauriers et al. 2003; Fritts 1976; Major and Johnsen 2001). As temperature and solar radiation decline in the late afternoon, transpiration and photosynthesis from the leaves occur more slowly than water absorption in the roots, resulting in a simultaneous decline in leaf water potential and increase in trunk water potential (Fritts 1976; Zhang et al. 2016). As a consequence, the significant correlation between stem radial variability and RH indicates stem radius changed coincidentally with the variations of solar radiation.
Precipitation, furthermore, is also an important climatic factor in stem radial variations, since it promotes tree growth and/or internal water supplement (King et al. 2013). However, reversible expansion of cambium through rapid water replenishment could generate an overestimation of the impacts of precipitation. High PAR negatively affects stem radial variations likely related to leaf photosynthesis, which could be explained by two mechanisms. Firstly, the declines of PAR reduce the rate of photosynthesis and decrease the leaf water potential, further decreasing stem water mobilization and thus contributing to water remaining in the conduit to decrease contraction (King et al. 2013; Zhang et al. 2016). Secondly, high PAR is prone to inducing light saturation, leading to low light-use efficiency. Leaf photosynthesis rates also decline at this time, which could result in carbon starvation and negative consequences for carbohydrate formation.
Temperature influences daily stem variations in complex ways such as indirectly changing tree water status over VPD and directly controlling tree respiration and assimilation processes (Downes et al. 1999; van der Maaten et al. 2018). However, the limitation of temperature to stem radial growth is often found in temperate and cold climates, particularly limiting growth onset and cessation (Begum et al. 2013; Deslauriers et al. 2008; Downes et al. 1999; Rossi et al. 2008). After reaching a certain threshold temperature and entering into the growing season, stem radial growth is generally thought to become limited by climatic factors other than temperature (Duchesne and Houle 2011). For example, Downes et al (1999) found a positive correlation between stem radial growth and temperature in spring when water was not limiting, which then decreases in strength and becomes negative during summer. In the present study, air temperature alone showed comparably weak effects on stem radial growth (Fig. 8). For the three listed temperature variables, the strongest negative correlations were found with maximum air temperature for both species. Additionally, significant negative correlations were also found with daily average and minimum temperatures for Masson pine in both years and for Chinese fir in 2018. The negative response of daily stem radial increment to temperature, as indicated by example data in Fig. 10b, can be caused by transpiration and respiration rates increasing with rising air temperature (Lewis et al. 1999; Zweifel et al. 2001), thus, in turn, promoting water loss and decreasing carbohydrates available for tree stem growth (Liu et al. 2018; Zhang et al. 2016). Furthermore, soil water content showed a relatively intense impact on the daily stem increment of Masson pine (Fig. 8), suggesting that the water loss by transpiration and the water uptake from the soil is more balanced (Guney et al. 2020), as demonstrated by Fig. 7.
When taking hysteresis effects of climatic forcing on daily stem variations into consideration, correlation relationships changed in intensity (Fig. 8) rather than direction, which are not compatible with published similar dendrometer studies (Downes et al. 1999; van der Maaten et al. 2013, 2018). This could be due to the methods of extracting daily stem dynamics from raw measurements, namely the ZG concept and stem cycle approach, respectively. In comparison with diurnal effects, however, lagging the climatic factors hardly improves the significance of the correlations for considered climatic factors. For example, a very explicit synchronous change with lagging as much as a few hours between stem radius and RH was observed (Fig. 10a). Further, lagging effects can also help in understanding the complexity of the interaction between climate and physiological processes. For example, Downes et al. (1999) suggest that temperature would immediately control metabolic rates but have lagged effects on stem radial growth through photosynthesis and transpiration, showing multiple effects.
Interspecific similarity in response to climatic forcing
We expected species-specific responses to climatic forcing in stem radial increment due to species-specific light and water demands, nutrient-use efficiency, or growth phenology. Our findings revealed a similar trend but species differences in strength of response to the same climatic variables. The observed similarity in climate responses could be explained by stem radial changes at short-term resolution. This implies that tree stem radial dynamics, regardless of species, may respond consistently to climatic forcing even if the strengths of climate-growth relationships vary among trees (van der Maaten et al. 2018). Consequently, synchronous or similar shrinking and swelling cycles were commonly observed for trees grown in one site, which is in accordance with previous relevant studies (Aldea et al. 2018; Fritts 1976; van der Maaten et al. 2018; Vandegehuchte et al. 2014).
Intra-annual dendrometer monitoring series offers information about two different signals, the irreversible stem size growth due to division and enlargement of wood and bark cells in the cambium, and the reversible stem shrinkage and swelling of the stem on account of imbalances between transpiration and water uptake (Duchesne and Houle 2011; Zweifel et al. 2005, 2006). Differentiating the wood formation and stem rehydration components of dendrometric cycles is remarkably difficult due to rapid and constant changes in tree water potential (Makinen et al. 2003). Failing to consider the intra-seasonal variation in tree water status may bias the estimates of tree growth (King et al. 2013), thus limiting the application of automated high-resolution dendrometers in future long-term growth monitoring programs. To better understand and quantify stem increment on short time scales, further research that focused on explicitly distinguishing true wood formation and stem growth from dendrometric cycles appears to be urgently needed. Sap-flow measurements might help to remove reversible stem hydrological cycles (Oberhuber et al. 2015; Wullschleger et al. 2004).
Understanding the growth response of tree species to meteorological factors benefits from long-term monitoring of radial growth, but unfortunately, our dendrometer measurements were prematurely ended after 2 years. Severe pinewood nematode disease in 2019 led to death of large numbers of Masson pine trees including the trees we were monitoring. Therefore, only two consecutive years of data were analyzed in our study. Despite comparisons of species and climate records with growth for only two years, our results yield generally reasonable and consistent climatic response characteristics for the two species. Additional years with more extreme weather (e.g. drought) would expand the range of conditions for which tree growth response could be assessed.
Conclusion
In the present study, we analyzed the stem growth characteristics (growth onset, cessation, duration, and annual cumulative stem radial increments) and daily variations with their response to climatic forcing for Masson pine and Chinese fir in subtropical China based on automated dendrometric measurements collected in 2017 and 2018. We found similar growth onset time between two species but prolonged growth duration and large annual cumulative radial increments for Chinese fir in 2017, attributing to strong transpiration and assimilation activity of Chinese fir. We suggest that stem radial size dynamics, irrespective of species, are closely associated with moisture conditions and indicate moisture plays a major role in tree stem growth. Similar correlation trend but different magnitudes of correlation coefficients between Masson pine and Chinese fir demonstrating climatic factors affect tree growth in the same way with different intensities. More studies are needed to combine the micro-coring approach and sap-flow measurements to profoundly understand growth rhythms and environmental responses using the corrected dendrometer series.
References
Aldea J, Bravo F, Vazquez-Pique J, Rubio-Cuadrado A, del Rio M (2018) Species-specific weather response in the daily stem variation cycles of mediterranean pine-oak mixed stands. Agric for Meteorol 256:220–230
Barraclough AD, Zweifel R, Cusens J, Leuzinger S (2018) Daytime stem swelling and seasonal reversal in the peristaltic depletion of stored water along the stem of Avicennia marina (Forssk.) Vierh. Tree Physiol 38:965–978
Begum S, Nakaba S, Yamagishi Y, Oribe Y, Funada R (2013) Regulation of cambial activity in relation to environmental conditions: understanding the role of temperature in wood formation of trees. Physiol Plant 147:46–54
Bouriaud O, Leban J-M, Bert D, Deleuze C (2005) Intra-annual variations in climate influence growth and wood density of Norway spruce. Tree Physiol 25:651–660
Chen GS, Yang ZJ, Gao R, Xie JS, Guo JF, Huang ZQ, Yang YS (2013) Carbon storage in a chronosequence of Chinese fir plantations in southern China. For Ecol Manage 300:68–76
Cocozza C, Palombo C, Tognetti R, La Porta N, Anichini M, Giovannelli A, Emiliani G (2016) Monitoring intra-annual dynamics of wood formation with microcores and dendrometers in Picea abies at two different altitudes. Tree Physiol 36:832–846
Deslauriers A, Morin H, Urbinati C, Carrer M (2003) Daily weather response of balsam fir (Abies balsamea (L.) Mill.) stem radius increment from dendrometer analysis in the boreal forests of Québec (Canada). Trees 17:477–484
Deslauriers A, Rossi S, Anfodillo T, Saracino A (2008) Cambial phenology, wood formation and temperature thresholds in two contrasting years at high altitude in southern Italy. Tree Physiol 28:863–871
Deslauriers A, Rossi S, Turcotte A, Morin H, Krause C (2011) A three-step procedure in SAS to analyze the time series from automatic dendrometers. Dendrochronologia 29:151–161
Downes G, Beadle C, Worledge D (1999) Daily stem growth patterns in irrigated Eucalyptus globulus and E. nitens in relation to climate. Trees 14:102–111
Duchesne L, Houle D (2011) Modelling day-to-day stem diameter variation and annual growth of balsam fir (Abies balsamea (L.) Mill.) from daily climate. For Ecol Manage 262:863–872
Duchesne L, Houle D, D’Orangeville L (2012) Influence of climate on seasonal patterns of stem increment of balsam fir in a boreal forest of Québec, Canada. Agric for Meteorol 162:108–114
Fang JY, Chen AP, Peng CH, Zhao SQ, Ci LJ (2001) Changes in forest biomass carbon storage in China between 1949 and 1998. Science 292:2320–2322
Fritts HC (1976) Tree rings and climate. Academic Press, New York
Gliniars R, Becker GS, Braun D, Dalitz H (2013) Monthly stem increment in relation to climatic variables during 7 years in an East African rainforest. Trees 27:1129–1138
Guney A, Zweifel R, Turkan S, Zimmermann R, Wachendorf M, Guney CO (2020) Drought responses and their effects on radial stem growth of two co-occurring conifer species in the Mediterranean mountain range. Ann for Sci 77:105. https://doi.org/10.1007/s13595-020-01007-2
Harvey JE, Smiljanic M, Scharnweber T, Buras A, Cedro A, Cruz-Garcia R, Drobyshev I, Janecka K, Jansons A, Kaczka R, Klisz M, Laanelaid A, Matisons R, Muffler L, Sohar K, Spyt B, Stolz J, van der Maaten E, van der Maaten-Theunissen M, Vitas A, Weigel R, Kreyling J, Wilmking M (2020) Tree growth influenced by warming winter climate and summer moisture availability in northern temperate forests. Glob Change Biol 26(4):2505–2518
Huang JG, Guo XL, Rossi S, Zhai LH, Yu BY, Zhang SK, Zhang MF (2018) Intra-annual wood formation of subtropical Chinese red pine shows better growth in dry season than wet season. Tree Physiol 38:1225–1236
Huang YQ, Deng XW, Zhao ZH, Xiang WH, Yan WD, Ouyang S, Lei PF (2019) Monthly radial growth model of Chinese Fir (Cunninghamia lanceolata (Lamb.) Hook.), and the relationships between radial increment and climate factors. Forests 10:757. https://doi.org/10.3390/f10090757
King G, Fonti P, Nievergelt D, Buntgen U, Frank D (2013) Climatic drivers of hourly to yearly tree radius variations along a 6 degrees C natural warming gradient. Agric for Meteorol 168:36–46
Knusel S, Conedera M, Zweifel R, Bugmann H, Etzold S, Wunder J (2019) High growth potential of Ailanthus altissima in warm and dry weather conditions in novel forests of southern Switzerland. Trees 33:395–409
Kuang YW, Sun FF, Wen DZ, Zhou GY, Zhao P (2008) Tree-ring growth patterns of Masson pine (Pinus massoniana L.) during the recent decades in the acidification Pearl River Delta of China. For Ecol Manage 255:3534–3540
Lewis JD, Olszyk D, Tingey DT (1999) Seasonal patterns of photosynthetic light response in Douglas-fir seedlings subjected to elevated atmospheric CO2 and temperature. Tree Physiol 19:243–252
Li L, Huang ZL, Ye WH, Cao HL, Wei SG, Wang ZG, Lian JY, Sun I-F, Ma KP, He FL (2009) Spatial distributions of tree species in a subtropical forest of China. Oikos 118:495–502
Liu XS, Nie YQ, Luo TX, Yu JH, Shen W, Zhang L (2016) Seasonal shift in climatic limiting factors on tree transpiration: evidence from sap flow observations at Alpine Treelines in Southeast Tibet. Front Plant Sci 7:1018. https://doi.org/10.3389/fpls.2016.01018
Liu XS, Nie YQ, Wen F (2018) Seasonal dynamics of stem radial increment of Pinus taiwanensis Hayata and its response to environmental factors in the Lushan mountains Southeastern China. Forests 9:387. https://doi.org/10.3390/f9070387
Liu XS, Wang CS, Zhao JK (2019) Seasonal drought effects on intra-annual stem growth of Taiwan pine along an elevational gradient in subtropical China. Forests 10:1128. https://doi.org/10.3390/f10121128
Major JE, Johnsen KH (2001) Shoot water relations of mature black spruce families displaying a genotype x environment interaction in growth rate. III. Diurnal patterns as influenced by vapor pressure deficit and internal water status. Tree Physiol 21:579–587
Makinen H, Nojd P, Saranpaa P (2003) Seasonal changes in stem radius and production of new tracheids in Norway spruce. Tree Physiol 23:959–968
McKenney MS, Rosenberg NJ (1993) Sensitivity of some potential evapotranspiration estimation methods to climate change. Agric for Meteorol 64:81–110
Oberhuber W, Hammerle A, Kofler W (2015) Tree water status and growth of saplings and mature Norway spruce (Picea abies) at a dry distribution limit. Front Plant Sci 6:703. https://doi.org/10.3389/fpls.2015.00703
Rossi S, Deslauriers A, Griçar J, Seo J-W, Rathgeber CBK, Anfodillo T, Morin H, Levanic T, Oven P, Jalkanen R (2008) Critical temperatures for xylogenesis in conifers of cold climates. Glob Ecol Biogeogr 17:696–707
Saderi S, Rathgeber CBK, Rozenberg P, Fournier M (2019) Phenology of wood formation in larch (Larix decidua Mill) trees growing along a 1000-m elevation gradient in the French Southern Alps. Ann for Sci 76:89. https://doi.org/10.1007/s13595-019-0866-3
Schaefer C, Roetzer T, Thurm EA, Biber P, Kallenbach C, Pretzsch H (2019) Growth and tree water deficit of mixed Norway spruce and European beech at different heights in a tree and under heavy drought. Forests 10:577. https://doi.org/10.3390/f10070577
Smiljanic M, Wilmking M (2018) Drivers of stem radial variation and its pattern in peatland Scots pines: a pilot study. Dendrochronologia 47:30–37
Sun T, Ma M, Wang X, Wang Y, Du H, Xiang Y, Xu Q, Xie Q, Wang D (2019) Mercury transport, transformation and mass balance on a perspective of hydrological processes in a subtropical forest of China. Environ Pollut 254:113065. https://doi.org/10.1016/j.envpol.2019.113065
Tian QY, He ZB, Xiao SC, Peng XM, Ding AJ, Lin PF (2017) Response of stem radial growth of Qinghai spruce (Picea crassifolia) to environmental factors in the Qilian Mountains of China. Dendrochronologia 44:76–83
van der Maaten E, Bouriaud O, van der Maaten-Theunissen M, Mayer H, Spiecker H (2013) Meteorological forcing of day-to-day stem radius variations of beech is highly synchronic on opposing aspects of a valley. Agric for Meteorol 181:85–93
van der Maaten E, Pape J, van der Maaten-Theunissen M, Scharnweber T, Smiljanic M, Cruz-Garcia R, Wilmking M (2018) Distinct growth phenology but similar daily stem dynamics in three co-occurring broadleaved tree species. Tree Physiol 38:1820–1828
Vandegehuchte MW, Guyot A, Hubeau M, De Groote SRE, De Baerdemaeker NJF, Hayes M, Welti N, Lovelock CE, Lockington DA, Steppe K (2014) Long-term versus daily stem diameter variation in co-occurring mangrove species: environmental versus ecophysiological drivers. Agric for Meteorol 192:51–58
Wang WB, Zhang F, Yuan LM, Wang QT, Zheng K, Zhao CY (2016) Environmental factors effect on stem radial variations of Picea crassifolia in Qilian Mountains Northwestern China. Forests 7:210. https://doi.org/10.3390/f7100210
Wullschleger SD, McLaughlin SB, Ayres MP (2004) High-resolution analysis of stem increment and sap flow for loblolly pine trees attacked by southern pine beetle. Can J for Res 34:2387–2393
Yang YF, Wang F, Jin J, Hu SL, Gao Y (2019) Carbon storage potential and seasonal dynamics of phytolith from different vegetation types in a subtropical region, China. Environ Sci Pollut Res 26:29834–29844
Yu FY, Guo XB, Xu XZ (2003) Water status of bare-root seedlings of Chinese fir and Masson pine. J for Res 14:51–55
Yu J, Liu QJ, Meng SW, Zhou G, Shah S, Xu ZZ (2018) Summer temperature variability inferred from tree-ring records in the central Hengduan Mountains, southeastern Tibetan Plateau. Dendrochronologia 51:92–100
Zeide B (1993) Analysis of growth equations. For Sci 39:594–616
Zhang RB, Yuan YJ, Gou XH, Zhang TW, Zou C, Ji CR, Fan ZA, Qin L, Shang HM, Li XJ (2016) Intra-annual radial growth of Schrenk spruce (Picea schrenkiana Fisch et Mey) and its response to climate on the northern slopes of the Tianshan Mountains. Dendrochronologia 40:36–42
Zhang XQ, Kirschbaum MUF, Hou ZH, Guo ZH (2004) Carbon stock changes in successive rotations of Chinese fir (Cunninghamia lanceolata (lamb) hook) plantations. For Ecol Manage 202:131–147
Zweifel R, Haeni M, Buchmann N, Eugster W (2016) Are trees able to grow in periods of stem shrinkage? New Phytol 211:839–849
Zweifel R, Häsler R (2001) Dynamics of water storage in mature subalpine Picea abies: temporal and spatial patterns of change in stem radius. Tree Physiol 21:561–569
Zweifel R, Item H, Häsler R (2001) Link between diurnal stem radius changes and tree water relations. Tree Physiol 21:869–877
Zweifel R, Zimmermann L, Newbery DM (2005) Modeling tree water deficit from microclimate: an approach to quantifying drought stress. Tree Physiol 25:147–156
Zweifel R, Zimmermann L, Zeugin F, Newbery DM (2006) Intra-annual radial growth and water relations of trees: implications towards a growth mechanism. J Exp Bot 57:1445–1459
Acknowledgements
The authors thanked Huang Yuanfen for his kind support in data collection and thanked anonymous reviewers for their valuable comments and suggestions to improve the quality of the paper. This research was supported by the project of the Field Station Alliance of Chinese Academy of Sciences (KFJ-SW-YW034).
Author information
Authors and Affiliations
Contributions
FX and WH conceived and designed the experiment. ZB and KL performed the fieldwork. DX, LQ, and YF helped provide and analyze climate data. MS wrote the manuscript with contributions from ZB.
Corresponding author
Ethics declarations
Conflict of interest
The authors declare no conflict of interest.
Additional information
Communicated by Leavitt.
Publisher's Note
Springer Nature remains neutral with regard to jurisdictional claims in published maps and institutional affiliations.
Rights and permissions
About this article
Cite this article
Meng, S., Fu, X., Zhao, B. et al. Intra-annual radial growth and its climate response for Masson pine and Chinese fir in subtropical China. Trees 35, 1817–1830 (2021). https://doi.org/10.1007/s00468-021-02152-5
Received:
Accepted:
Published:
Issue Date:
DOI: https://doi.org/10.1007/s00468-021-02152-5