Abstract
Rain-on-Snow (ROS) events can cause severe snowmelt hazards such as river flooding, avalanches, and landslides that have significant impacts on various sectors. The influence of climate change on the frequency of ROS events in Japan was investigated using climate projections obtained from the Database for Policy Decision making for Future climate change (d4PDF). The projected future climate in the regional model simulations showed an increase in the ROS events over the mountainous areas in Hokuriku (Sea of Japan side of Central Japan) and Hokkaido (Northern Japan) regions, where a higher amount of snowfall will still occur in the future. Characteristics of ROS events such as rainfall, snowmelt, and related runoff were also enhanced in these regions. Self-organizing maps (SOMs) were applied using the surface atmospheric circulation data to determine the dominant ROS-related weather patterns (WPs) in the present and future climate. The SOMs showed that some WPs had a significant effect on the cause of the ROS events. The differences in the impacts of climate change between the WPs were evaluated to understand the future changes in runoff and snowmelt associated with ROS events. The SOM analysis suggests that the increase in the occurrence of ROS events and the resultant enhancement in their characteristics in the future-climate projection can be attributed to the changes in the dominant ROS-related WPs (from cyclonic to cold-surge type) corresponding to variations in the freezing point line. These findings can inform water hazard and water resource management plans that aim to withstand regional climate change.
Similar content being viewed by others
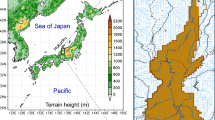
Avoid common mistakes on your manuscript.
1 Introduction
The phenomenon of rainfall on snow is called a Rain-on-Snow (ROS) event that can cause severe hazards such as river flooding, snow avalanches, and landslides because of a combination of rain and snowmelt. These ROS events have significant impacts on various sectors, especially with regards to water resources management, flood forecasting, and risk management (e.g. Freudiger et al. 2014; McCabe et al. 2007; Sui and Koehler 2001). The ROS events and the related disasters occur not only during the snowmelt season in the early spring (Ishii 2019), but also during the snowfall season in the winter (Sui and Koehler 2001). The impacts of ROS events on floods have been investigated in previous studies (e.g. Freudiger et al. 2014; Sui and Koehler 2001; Surfleet and Tullos 2013) using hydrological modeling and observation datasets, as these events can cause severe floods during snowmelt seasons (Floyd and Weiler 2008; Ishii 2019). During the ROS events, which are dependent on the amount and temperature of the rainwater falling onto the snow, large volumes of snow can melt rapidly and generate considerable water fluxes (Ishii et al. 2017; Whitaker and Sugiyama 2005) in a catchment zone. For example, the 2017 Oroville dam crisis in California was exacerbated by rapid snowmelt in relation to an ROS event in addition to the extreme rainfall (White et al. 2019). Contrarily, the resultant snowmelt in winter could have a direct influence on decreased spring snowfall and consequently, on water storage and supply in snowmelt-dominated regions.
ROS events have complex generation mechanisms which depend on snow depth, precipitation types (rain or snow), and air temperature (Freudiger et al. 2014; McCabe et al. 2007). Increasing atmospheric temperatures may decrease the frequency of ROS events in mid-latitude regions (McCabe et al. 2007) in the future by reducing the amount of snowfall and number of snow-covered days. In contrast, in mountainous and high-latitude regions, an increase in the ROS frequency is projected due to increasing rainfall days (Jeong and Sushama 2018). Despite the increase in temperatures, snow cover in these regions will exist during most of the cold seasons since air temperatures will generally remain below the freezing point (0 °C). Therefore, the risk of an ROS flood will vary with the future warming climate, and it is extremely important to evaluate how it will change at a regional scale.
Previous studies have investigated ROS events and their long-term changes using observation datasets in relation to rising air temperatures (e.g., Cohen et al. 2015; McCabe et al. 2007; Pall et al. 2019). Climate change impacts on ROS events have also been investigated using projections based on global and regional climate models (Bieniek et al. 2018; Jeong and Sushama 2018; Musselman et al. 2018) even though they are for limited areas. Detailed regional scale studies predicting how ROS events will change in the future climate are not yet available for most parts of the world facing such risks. Understanding the spatial distribution, climatic behavior, variability, and drivers of atmospheric circulation of ROS events is important for better planning of flood disaster prevention and water resource management.
Most of the ROS-related floods are reported in the mountainous areas of Hokuriku (Whitaker and Sugiyama 2005) and the northern part of Japan (Ishii 2019), both of which experience extremely heavy snowfall. From winter to spring these regions are colder than the rest of Japan increasing the likelihood of occurrence of ROS events Ando and Ueno (2015). investigated the changes in winter rainfall and snowfall in central Japan by analyzing the ratios of snowfall and rainfall events in recent decades. However, climatological studies that focus on the drivers of atmospheric circulation and how they are being affected by the influence of global warming on ROS events in Japan are unavailable.
ROS events can be attributed to synoptic-scale atmospheric circulation drivers with a nonlinear relationship to meteorological factors. Synoptic-scale background weather conditions determine precipitation type (rainfall or snowfall) events on a daily timescale. Classifying synoptic weather patterns (WPs) by characterizing the statistical properties of meteorological variables at a local scale provides insights to the origins of the ROS events. Previous studies have used WP classification to gain an insight into the dominant extreme precipitation patterns (Brigode et al. 2013; Ohba et al. 2015) and have discussed pattern-by-pattern changes under global warming (Ohba and Sugimoto 2019, 2020). In this study, a WP classification technique was applied to examine the impacts of global warming on the relationships between ROS and synoptic-scale WPs during the winter season in Japan. Climate change impacts on ROS may vary among WPs, which would lead to regional variations in future ROS events.
Our main objective is to investigate the projected future changes in the ROS events due to global warming with respect to the present climatic conditions in Japan. Future changes in ROS events are further investigated by evaluating them with respect to the relevant meteorological/climatological variables, such as rainfall and snow depth. Projected changes to the snowmelt and runoff caused by changes in future ROS events are also investigated.
2 Data and methods
2.1 Data
The atmospheric simulation data used in this study for historical and projected climatic conditions was taken from the Database for Policy Decision making for Future climate change (d4PDF: Mizuta et al. 2017; Kawase et al. 2019). The d4PDF consists of outputs obtained from a general circulation model (d4PDF-GCM) that is dynamically downscaled for the Japanese region using a regional climate model (d4PDF-RCM). The horizontal grid spacings of the d4PDF-GCM and d4PDF-RCM are 60 and 20 km, respectively. The 60-km resolution d4PDF-GCM data was used exclusively for the self-organizing map (SOM) and the corresponding 20 km downscaling data (d4PDF-RCM) was used for regional/local-scale analysis in Japan. Notably, the 20-km regional climate model (RCM) uses the MRI/JMA Simple Biosphere model (MJ-Sib: Hirai et al. 2007) as its land surface model, providing hydrological variables such as runoff and snow melt for each grid.
In this study, 40-member historical simulations (1951–2010) and 60-member (with six cases for each 10-member ensemble) + 4-K future-climate projections (2051–2110) were used to analyze the climate change impacts. The details of the experimental setup for the simulations are described by Kawase et al. (2016) and Mizuta et al. (2017). The ensemble members were obtained from the perturbed lower boundary, with initial conditions of a 60-km GCM. As for the + 4-K future-climate simulations, the climatological warming patterns of sea surface temperature (SST) derived from the six climate models (CCSM4, MIROC5, MPI-ESM-MR, HadGEM2-AO, GFDL-CM3, and MRI-CGCM3 contributing to Coupled Model Intercomparison Project Phase 5) were added to the observational SSTs after removing the long-term component. The SST difference between the RCP8.5 and historical simulations was used as the warming pattern. The validation of precipitation in d4PDF is discussed in Endo et al. (2017), Kawase et al. (2018), and Ohba and Sugimoto (2019) for summer, and Kawase et al. (2016) and Ohba and Sugimoto (2020) for winter.
The WP classification technique was applied to the daily mean surface zonal and meridional winds obtained from the d4PDF-GCM. Raw surface wind vectors within a specific region (123° E–148° E, 26° N–48° N) were used.
2.2 Definition of Rain-on-Snow events
An ROS day in d4PDF is defined as a day with both a daily precipitation (rainfall) above 10 mm and a snow depth of more than 10 cm for a given grid cell. However, there is no consensus on the definition of ROS across the world and hence, this precipitation/snow-depth range differs in the studies carried out in different countries (most meteorological studies on ROS have used a lesser value e.g., snow depth ~ 10 mm). However, as Japan is a very mountainous country with minimal flat land (Fig. 1) and experiences heavy snowfall within small regions, these higher values were employed. The ROS-associated daily rainfall, snowmelt, and runoff are referred to as ROS-rainfall (intensity of ROS events), ROS-snowmelt, and ROS-runoff, respectively.
2.3 SOM technique
Self-organizing map (SOM) is a type of artificial neural network that is based on unsupervised learning, which can effectively classify various nonlinear WPs. The SOM technique was developed by Kohonen (1982), as a nonlinear pattern recognition technique that can project high-dimensional data onto a two-dimensional map that is visually comprehensible. This technique has been used in previous climatological studies to gain spatially organized weather patterns. For details, please refer to the work of Ohba et al. (2016a). Previous studies (e.g., Ohba et al. 2015) have examined the relationships between local weather variables and large-scale WPs, and these relationships have been applied for weather/climate forecasting (e.g., Cavazos et al. 2002; Ohba et al. 2016b, 2018). In these studies, clearly defined WPs are successfully extracted from nonlinear atmospheric elements using SOMs. The advantages of using this approach in meteorology have also been discussed in the works of Reusch et al. (2007) and Gibson et al. (2017). The WP classification using SOMs is useful for identifying complex nonlinear interrelationships between WPs and climatic factors. A torus-type SOM (Ito et al. 2000) is used instead of a conventional SOM as the former has no map edges. The SOM projection process has been described in detail elsewhere (Gibson et al. 2017; Ohba et al. 2016a, b; Ohba and Sugimoto 2019, 2020).
Daily surface wind vectors from the d4PDF-GCM are used as the input vectors of SOM. To classify large-scale WPs while minimizing computational costs, the atmospheric variables from the GCM are used for the SOM analysis. Each element in the array is denoted as a node with one reference vector, where each reference vector represents a generalized pattern of input vectors. We use a 20 × 20-grid SOM with 400 reference vectors to classify 400 WPs. The number of input vectors is 324,000 (90 days × 60 years × 60 ensembles for the historical and future simulations; 30-member historical and 30-member future climate simulations are used to make the SOM). We also test another number of SOM nodes (such as 15 × 15) and atmospheric variables such as the sea-level pressure (SLP) to confirm they do not significantly affect the results obtained in this study. In this study, a single SOM is trained with the two climate simulations to directly compare the impact of climate change on the ROS in the same WP. Both simulations are merged to train the SOM with the same weighting. Such work has been conducted in other SOM studies and results in what is often termed a “master SOM” (Cassano et al. 2007). Although the resultant SOM obtained from the two climates are almost identical, it is preferable to use a single SOM to directly compare the future and present climates on an equal footing. The use of a master SOM resulted in less total discrepancy (defined by the integrated Euclid distance between the original data and best match node) compared to the SOM obtained from the present climate.
3 Results
3.1 Future changes in Rain-on-Snow events
Figure 2 shows the distributions of annual ROS days in Japan for the present and future climates, and their differences that were derived from the d4PDF-RCM data. The d4PDF-RCM simulated a large number of ROS days especially in the central to northeast region of Japan facing the Sea of Japan (Hokuriku and west of Tohoku regions) and in the northernmost region of Japan (Hokkaido region). Snowmelt hazards during an ROS event were reported for these regions in previous studies (Ishii 2019; Whitaker and Sugiyama 2005). The present-climate simulations show that in the southern and western regions of Japan facing the Pacific Ocean, little to no ROS events can occur (Fig. 2a). The spatial distribution of the ROS events (associated with snow-depth) could be attributed to mountainous effects, that is, orographic enhancement (suppression) of snowfall to the windward (leeward) side of the mountains (Fig. 1 for the topography of Japan).
The future-climate simulations showing the changes in the spatial distributions of the ROS events are presented in Fig. 2b and c. Most of the regions show a decrease in ROS days in the future owing to an increase in air temperature and the resultant decrease in the days with snow cover on the ground. There are strong regional variations in the projected ROS frequency, where the values decreased in the coastal plains/low-altitude areas and increased in the high-mountainous regions. Such ROS decreases in coastal areas were also observed in other regions (Pall et al. 2019). However, the increases in the ROS days were evident in the mountainous Hokuriku (Hida mountain-range known as the northern Japanese alps and Echigo mountain-range) and Hokkaido (Ishikari mountains) regions.
The spatial distribution of seasonal means of ROS days (Fig. 3) for winter (DJF: December–January–February) and spring (MAM: March–April–May) were analyzed to confirm the seasonal evolution of the ROS events and their changes. Similarly, this analysis was performed for summer (JJA: June–July–August) and fall (SON: September–October–November) (Fig. S2). The spatial distributions of the ROS days vary among the seasons. The ROS events in the present climate occur only slightly in the central and northern regions that face the Sea of Japan during JJA-SON (Fig. S2a), while are more widespread in winter and spring (Fig. 3a). The total occurrence of ROS throughout Japan peaks during MAM in the present climate, while it peaks during DJF in the + 4 K warming projection (Fig. 3b).
Seasonal means of ROS days obtained from the d4PDF-RCM during the winter season (December–January–February) and spring (March–April–May): a present climate simulations, b + 4-K future climate simulations, and c their differences. Black dot implies the region where the snow depth in the future climate is under 10 cm
In the future-climate simulation, the spatial distribution of ROS days substantially decreases from spring (Fig. 3c) to fall (Fig. S2c) in most of the regions. These decreases are caused by the projected future decreases in snow depth (dotted line, in Fig. 2c and 3c) that is associated with a northward shift of the freezing line (Fig. S1b). However, in winter in the projected + 4 K future-climate projection, a substantial increase in the ROS events over Hokuriku and Hokkaido regions was observed along with large amounts of snowfall (Fig. S3b) and increases in rainfall frequency. The mean snow depth for the two regions in the future-climate projection is almost similar to that in the present climate since air temperatures will still remain lower than the freezing point (Fig. S1). The increase in frequency and the northward and mountainward areal extent of ROS events are broadly consistent with previous studies in other regions (Rennert et al. 2009; Jeong and Sushama 2018; Putkonen and Roe 2003).
In addition to the frequency of the ROS events, changes in the intensity of ROS is also an important concern. Therefore, detailed changes in the characteristics of the ROS events (i.e., rainfall and snowmelt, and their related runoff) were also analyzed. Figures 4 and 5 show the mean ROS-rainfall, snowmelt, runoff, and its 50-year return value (RV50) for DJF and MAM, respectively. Overall, spatial patterns of the projected changes to the four ROS characteristics are relatively similar to those of the ROS days for the corresponding region (Fig. 3c). The results display a general enhancement of the ROS characteristics for higher latitude and elevation bands, and a decrease for the lowest elevation band in central and southern Japan. In addition to the increase in the ROS days, the ROS characteristics are also enhanced over the Hokuriku and Hokkaido regions in the future-climate projections, especially in the winter (Fig. 4).
Climatology of seasonal-mean (DJF: December–January–February) obtained from the d4PDF-RCM: a ROS-rainfall, b ROS-snowmelt (mm/day), c ROS-runoff (mm/day), and d RV50 of ROS-runoff for present and future climate simulations and their differences. Only regions with seasonal mean ROS days exceeding 0.2 are plotted
Climatology of seasonal-mean (MAM: March–April–May) obtained from the d4PDF-RCM: a ROS-rainfall, b ROS-snowmelt (mm/day), c ROS-runoff (mm/day), and d RV50 of ROS-runoff for present and future climate simulations and their differences. Only regions with seasonal mean ROS days exceeding 0.2 are plotted
Northern Japan and the mountainous central parts also show an increase in the ROS runoff and snowmelt as well as an increase in ROS days, yet the pattern is slightly different from the changes in ROS rainfall (quantity). The ROS rainfall is projected to increase the most in the future in the central to north-eastern part of Japan throughout the seasons (Figs. 4a and 5a). Since the ROS related total precipitation (rain + snow) is projected to decrease in the future during the winter (Fig. S5a), the increase in ROS rainfall could be mainly attributed to the phase change in precipitation from snow to rain corresponding to warming temperatures. These changes are consistent with the northward shift of the rain-snow transition layer (i.e., freezing point line) that can cause an increase in rainfall throughout all seasons.
The future extreme values of ROS runoff during winter are much different from those of the present climate (Fig. 4d). The future RV50 of the ROS runoff shows larger increases, especially in the regions where the ROS frequency is predicted to increase as the ROS events generally accelerate snowmelt (Figs. 4b) and enhance runoff (Figs. 4c). This can result in an increase in the extreme value of the total runoff (Fig. S4) from central to northern Japan. Contrarily, the decrease in the total runoff in the future RV50 (Fig. S4) for spring is associated with a decrease in future snow water equivalent (SWE) and/or early snowmelt in the previous season.
As discussed in previous studies, Indo-Pacific SST anomalies from winter to spring can affect the amount of precipitation in East Asia (Ohba and Ueda 2006) owing to the anomalous atmospheric circulations in the western Pacific (Ohba and Ueda 2009; Ohba 2013). Thus, differences in future SST warming patterns can affect the future changes in ROS. Future climate projections in d4PDF are based on six SST warming patterns (obtained from six different climate models) as the background state for boundary forcing. Since the uncertainty in future SST projections is large, it is important to understand the model’s diversity with reference to the different background SSTs. To verify the model (SST) diversity, the response of the ROS days for each model SST was evaluated (Fig. 6).
a The difference in ROS days between + 4-K future and present climate simulations using six SSTs from CMIP5 models (CCSM4, MIROC5, MPI-ESM-MR, HadGEM2-AO, GFDL-CM3, and MRI-CGCM3) during winter. b The minimum and maximum projected changes in ROS days based on the six simulations. Black dot implies the region where the snow depth in the future climate is under 10 cm
Despite the large climatological differences (Mizuta et al. 2017), the projected ROS changes are relatively comparable among the 6 SST warming patterns. There are some minor differences in spatial distribution depending on the projected SST changes (Fig. 6a). The range of the projected changes from the combinations of six projections (Fig. 6b) is an indication of the uncertainty in climate impacts, which is larger for northernmost Japan compared to the central-northern regions. For instance, the simulations from the MIROC5-SST model broadly suggest higher increases in northern Japan when compared to that of the HadGEM-SST model, which highlights the differences in projections caused by the differences in the boundary forcing. It must be noted that MIROC5 projects higher increases in temperature around Japan compared to the other models for this season (Ohba and Sugimoto 2020). This would lead to a higher rainfall frequency in MIROC5 compared to the other models.
3.2 Weather patterns leading to Rain-on-Snow events
As mentioned above, in a warming climate, the ROS events during winter significantly increased in the Hokuriku and Hokkaido regions. In this section, the impact of atmospheric circulation as a driver for the generation of the ROS events is discussed in detail. Since the winter season consists of various types of WPs, it is difficult to determine whether a given WP will produce ROS in the present- and future-climate simulations. Therefore, classifying the various types of WPs would be useful for understanding the relationship between the WPs and the ROS events. In this section, data from December 1 to February 28 (corresponding to the winter season in Japan) are presented and analyzed.
Large-scale climatological features during winter in Japan are represented in Fig. S1 (see supplemental file). It is well known that the synoptic weather conditions bringing winter precipitation (snowfall) into the inland area of mainland Japan, differ from those along the Sea of Japan and the Pacific Ocean (Akiyama 1981; Ando and Ueno 2015; Kawase et al. 2018). Statistical analyses indicated that heavy snowfall is caused by an enhanced climatological winter monsoon, where strong northerly–northwesterly winds travel from the Eurasian continent along the Sea of Japan (Akiyama 1981; Iwamoto et al. 2008; Tachibana 1995; Ueda et al. 2015; Yamazaki et al. 2019). Similarly, these occur along the Pacific Ocean when southern coastal extratropical cyclones are passing (Kawase et al. 2018; Takano 2002).
Figure 7 shows the WP matrix obtained from the SOM analysis. The synoptic WPs of the surface wind vectors around Japan are classified into 400 patterns presented on a 20 × 20 map. The red-blue shaded contours indicate the meridional winds. WPs featuring northerly and southerly winds are distributed near to and away from the center of the SOM, respectively. The center to right side of the SOM is covered by WPs corresponding to cold air outbreaks from the Eurasian continent. The WPs associated with cyclonic circulations are distributed in the center-left of the SOM. The relationships between WPs and ROS frequency were identified using the SOM for Region A (139° E–146° E, 41.5° N–45.6° N) and Region B (136° E–140° E, 36° N–38.5° N), which represent the Hokkaido and Hokuriku regions, respectively (Fig. 1, red boxes). Figures 8 and 9 show the regional mean values of ROS frequency at each SOM for the present-climate simulations for Regions A and B, respectively. It is evident that the occurrence of ROS events is strongly dependent on the synoptic WPs. Furthermore, a significant impact of ROS is more apparent at the center and center-left regions of the SOM in the present climate (Figs. 8a and 9a), while the ROS-related WPs are slightly different in the two regions. The dominant WPs are highlighted in Figs. 8a and 9a (see insets) corresponding to the high frequency of ROS over Japan in the present winter climate. This correlates with the cyclonic circulation patterns covering Japan which are pronounced in the west of each region.
WPs derived from the 20 × 20 SOM nonlinear classification. The classification is based on the horizontal surface wind vectors in the d4PDF-GCM for the winter season (December–January–February). The red- and blue-shaded contours indicate the northward and southward meridional winds, respectively. Four different weather patterns are enlarged as a sample of SOM nodes
Regional mean of the amount of ROS days in Region A (Hokkaido area) at each SOM node obtained from the d4PDF-RCM: a present climate simulations and b + 4-K future climate simulations. The enlarged WP is a sample of WPs related to ROS events (occurrence rate; day/day). c Difference between the + 4-K future and present climate simulations. The boxes denote the weather patterns (WP1) of the SOM nodes with the highest increase of ROS days
Regional mean of the amount of ROS days in Region B (mainly Hokuriku area in Japan) at each SOM node obtained from the d4PDF-RCM: a present climate simulations and b + 4-K future climate simulations. The enlarged WP is a sample of WPs related to ROS events (occurrence rate; day/day). c Difference between the + 4-K future and present climate simulations. The boxes denote the weather patterns (WP2 and WP3) of the SOM nodes with the highest increase and decrease of ROS days
Figures 8c and 9c show the differences in the ROS frequency between the future- and present-climate simulations. Overall, the dominant WPs are relatively similar between the present and future climate. For Region A, the overall features of the SOM for the + 4-K future-climate simulations (Fig. 8b) is almost the same as that for the present-climate simulations, while the increase in ROS is the largest in the center of the SOM (Fig. 8c). In contrast, the changes that occur in Region B (Fig. 9b) are more complex. The ROS increase in the center-right and decrease in the center-left parts of the SOM (Fig. 9c). These trends could be attributed to changes in the climatological patterns around Japan during the winter (Figs. S1). Three WPs (WP1, WP2, and WP3) are marked in Figs. 8c and 9c, which indicate relatively large changes in the occurrence of ROS. Figure 10 further highlighted these WPs.
The ROS days for a WP1, b WP2, and c WP3. These weather patterns significantly alter the total ROS days for the present climate simulations (top), + 4-K future climate simulations (middle top), and the differences between the future and present climate simulations (middle bottom). Horizontal surface wind vectors are based on the SOM analysis. The blue contour line corresponds to the freezing line (i.e., mean surface temperature is 0 °C). (bottom) Expanded zone above the freezing point from the present to future climate (pink) and sea-level pressure (SLP; black contour, 2 hPa interval) based on the present climate simulations. The regional mean values are removed to clearly show the horizontal gradient of SLP
Figure 10 shows the frequency of ROS days for WP1, WP2, and WP3 obtained for the present- and future-climate simulations and their differences. The results clearly show the impact of large-scale weather conditions on the generation of ROS events. It is evident that WPs have an impact on ROS events, and the future changes of the ROS events vary for the three WPs.
WP1 corresponds to extratropical cyclonic circulation over northeast Japan (Fig. 10a), which provides air intrusion from the south to the northeastern regions. Corresponding to the circulation, the freezing point line is slightly shifted northward compared to the climatological condition (Fig. S1a). In the present climate, cyclonic circulation can bring rainfall when the freezing point (0 °C) is exceeded in East Japan and hence, the ROS events are evident in the Tohoku region.
WP2 corresponds to extratropical cyclonic circulation over central Japan (Fig. 10b) that provides strong air intrusion from the south to central regions. This southerly wind covers all of Japan causing the freezing point line to shift towards the north. Throughout the main island of Japan, when temperatures exceed the freezing point, rainfall and snow melt occur. When a low pressure system with warm/cold front lines proceeds eastward, the warm and moist air can flow towards the lows with warm fronts. This WP often causes heavy rainfall over central Japan when the cyclonic low and related fronts pass (Ando and Ueno 2015). This phenomenon has a pronounced effect on the central to eastern part of Japan (especially for Tohoku and Hokuriku regions) for the generation of ROS events.
WP3 reflects the climatological conditions of Japan. The Japanese islands are covered by strong northwesterly winds from the Asian continent that can be enhanced by the prevalent negative zonal pressure gradient (Fig. S1). In the present climate, this WP causes snowfall (Iwamoto et al. 2008; Ueda et al. 2015; Yamazaki et al. 2019), which is characterized by an intensified wintertime zonal pressure gradient across Japan (i.e., low pressure to the east and high pressure to the west) along with enhanced cold air outbreaks (Fig. 10c). The Japan Sea polar air-mass convergence zone is found over the Sea of Japan (Kawase et al. 2016). WP3 does not lead to ROS events in the present climate.
The differences between the present- and future-climate simulations show significant changes in the frequency of ROS events in Japan (middle bottom, Fig. 10). Interestingly, the number of ROS days decreased in the mainland of Japan (WP1 and WP2), while it increased in the mountainous regions of northern central Japan (WP3) and Hokkaido (WP1 and WP2). In the future-climate projection, WP3 causes the occurrence of ROS events over the coastal plains in central-north Japan. The integration of these changes is consistent with the nonuniform future changes projected for ROS events during winter (Fig. 3c).
The changes in the ROS events can be interpreted as changes in the freezing point line corresponding to global warming. Using the WP classification, the WP of the same node can generally be recognized as having the same “dynamic” origin. Therefore, the projected differences in temperature are assumed as the differences in the “thermodynamic” effects of global warming and the frequency change in WPs represent the “dynamic” effects (Ohba 2019; Ohba and Sugimoto 2019, 2020). The spatial distribution changes in the projected freezing point line for WP1–WP3 are also shown in Fig. 10. The impact of global warming differed substantially among the WPs. The WPs showed significant changes in the regions above the freezing point (> 0 °C, pink). In the future-climate projection, the regional changes in the freezing point line owing to global warming could cause modulation from snowfall to rainfall that is coincident with the regions that have an increase in the number of ROS days (Fig. 10).
The impact of climate change on the ROS characteristics was further explored through estimations of future changes in the spatial distributions of the daily rainfall, snowmelt, and ROS-runoff for WP1–WP3 (Fig. 11). The amount of rainfall increased (Fig. 11a) with the warming. Rainfall increases corresponds to cyclonic lows in WP1 and WP2, while WP3 is caused by a transformation from snowfall to rainfall. An increase in rainfall with warming can enhance the snowmelt over the region. Indeed, snowmelt is enhanced in high elevation bands and high latitude regions while it is different among the WPs (Fig. 11b). The combination of the changes in rainfall and snowmelt can cause the enhancement of extreme runoff (Fig. 11c). The integration of these changes is highly consistent with changes in the ROS characteristics seen in Fig. 4.
4 Discussion and conclusion
This is the first study of the climatological and meteorological analysis of ROS events in East Asia. It is established that ROS events often occur from winter to spring in Japan. Projected changes to ROS characteristics (i.e., frequency, precipitation amount, and runoff) over Japan under the + 4 K global-warming projection were investigated in this study. The results of the d4PDF large-ensemble simulations suggest general increases in future ROS events in the mountainous and northern-part of Japan owing to increases in rainfall frequency during winter corresponding to the increased air temperature. They also suggest general decreases in the number of future ROS days in other regions because of decreases in snowfall in the future climate throughout the year. Future extreme runoff also tends to be enhanced for the Hokuriku and Hokkaido regions as the ROS events can enhance rainfall and accelerate snowmelt. Therefore, ROS events in these regions are enhanced in the future-climate projection in terms of intensity and frequency. These changes in ROS depend on the temperature rise, and the pattern of change may be different at different warming intensities.
The projected changes to the ROS characteristics (frequency and intensity) shown in this study are useful in evaluating detailed regional impacts on social, environmental, and ecosystem sectors throughout Japan. Overall, the results in the six simulations based on different SST warming patterns agree with each other. However, finer-scale downscaling simulations by different RCMs and from different GCMs might be useful in evaluating uncertainties in the projections.
The changes in the ROS events are especially large during winter. This study investigated the possible future change in winter ROS events and their complex linkage to synoptic WPs to gain an insight into the differences in the projected climate change impacts among synoptic WPs. The synoptic WPs, expressed by the spatial distribution of surface horizontal winds, were classified using SOM. The results indicate that the impact of synoptic WPs on ROS events in winter can be altered in the future climate situation. Different responses to varying WPs can be attributed to differences in the variation of the freezing point line that acts as the rain–snow transition layer. Thus, the spatially heterogeneous future changes in the ROS frequency could be attributed to the differences in the WP responses. Furthermore, the beneficial utility of WP comparison in terms of global warming in order to understand the future changes in the hazard map at the local scale was confirmed. The presented method is effective in analyzing the impacts of global warming, and enables an enhanced interpretability of the climate change impact assessment.
The results also illustrate that ROS events can generate floods over the mountainous regions during winter in the future. Furthermore, an increase in future extreme runoff from winter to spring owing to increased rainfall and snowmelt for the central to north-eastern regions of Japan can be expected. However, more detailed studies from a hydrological perspective are necessary to assess future occurrences of extreme compound-ROS events arising from extreme rainfall and rapid SWE loss that can lead to extreme snowmelt floods during the winter in Japan.
In contrast to the increase in ROS-floods in winter, the decrease in ROS-runoff is because of the decrease in water resources during the dry season (i.e., late spring and early summer). The hydro-resource potential could be affected by climate change because of the changes in river-flows in relation to the variations in precipitation, snowmelt, extreme weather events, and the related sediment loads in dams (Troccoli et al. 2014). As shown in this study, the management of water resources during the snowmelt season in some mid-latitude regions will be subjected to change in the future. Accurate estimation of water runoff for each river basin based on the hydrological runoff model is necessary to assess the impacts of climate change and to adequately manage the planning of dams in the future. A detailed scheme to accurately estimate the impact of winter ROS events by using a high-resolution hydrological runoff model is under investigation for the most important river-basins in Japan (Arai et al. 2020).
In this study, we studied regional climate experiments with a 20-km grid spacing, which roughly resolved the complex Japan’s mountains. The use of a 5-km resolution can better reproduce the winter precipitation (Kawase et al. 2018) compared to simulations with a 20-km grid spacing (Fig. S7), while a spatial resolution of 5 km is still insufficient to fully resolve the snowfall process in the high mountainous areas. A higher-resolution model with a 2-km grid spacing, namely the cloud-resolving or convection permitting model, would further improve the predictability of ROS events. In future studies, ensemble experiments employing the cloud-resolving model should be conducted to accurately simulate ROS events in Japan’s complex mountainous terrain. In addition, in this study, ROS events are counted in the number of days; for example, if an ROS event occurred for three consecutive days, it is counted as three events (days) that similar weather pattern governs. In other words, one limitation of this study is the increase in the number of occurrences can be due to an increase in the duration of the occurrence. The continuity of ROS events should also be investigated in the future.
References
Akiyama T (1981) Time and spatial variations of heavy snowfalls in the Japan Sea coastal region. Part I. Principal time and space variations of precipitation described by EOF. J Meteorol Soc Jpn 59:578–590
Ando N, Ueno K (2015) Occurrence tendency of heavy rainfall or snowfall in the inland district of Japan in winter. Seppyo 77:397–410 (in Japanese with English abstract)
Arai R, Toyoda Y, Ohba M, Sato T, Kazama S (2020) Climate change effects on snowmelt runoff in Shogawa river basin. J Jpn Soc Civ Eng Ser G (Environmental Research), in press
Bieniek PA, Bhatt US, Walsh JE, Lader R, Griffith B, Roach JK, Thoman RL (2018) Assessment of Alaska rain-on-snow events using dynamical downscaling. J Appl Meteorol Climatol 57:1847–1863
Brigode P, Mićović Z, Bernardara P, Paquet E, Garavaglia F, Gailhard J, Ribstein P (2013) Linking ENSO and heavy rainfall events over coastal British Columbia through a weather pattern classification. Hydrol Earth Syst Sci 17(4):1455–1473. https://doi.org/10.5194/hess-17-1455-2013
Cassano JJ, Uotila P, Lynch AH, Cassano EN (2007) Predicted changes in synoptic forcing of net precipitation in large Arctic river basins during the 21st century. J Geophys Res 112:G04S49. https://doi.org/10.1029/2006jg000332
Cavazos T, Comrie AC, Liverman DM (2002) Intraseasonal variability associated with wet monsoons in southeast Arizona. J Clim 15(17):2477–2490. https://doi.org/10.1175/1520-0442(2002)015<2477:ivawwm>2.0.co;2
Cohen J, Ye H, Jones J (2015) Trends and variability in rain-on-snow events. Geophys Res Lett 42:7115–7122
Endo H, Kitoh A, Mizuta R, Ishii M (2017) Future changes in precipitation extremes in East Asia and their uncertainty based on large ensemble simulations with a high-resolution AGCM. SOLA 13:7–12. https://doi.org/10.2151/sola.2017-002
Floyd W, Weiler M (2008) Measuring snow accumulation and ablation dynamics during rain-on-snow events: innovative measurement techniques. Hydrol Process 22(24):4805–4812
Freudiger D, Kohn I, Stahl K, Weiler M (2014) Large-scale analysis of changing frequencies of rain-on-snow events with flood-generation potential. Hydrol Earth Syst Sci 18(7):2695–2709
Gibson PB, Perkins-Kirkpatrick SE, Uotila P, Pepler AS, Alexander LV (2017) On the use of self-organizing maps for studying climate extremes. J Geophys Res Atmos 122(7):3891–3903. https://doi.org/10.1002/2016jd026256
Hirai M, Sakashita T, Kitagawa H, Tsuyuki T, Hosaka M, Oh'izumi M (2007) Development and validation of a new land surface model for JMA's operational global model using the CEOP observation dataset. J Meteor Soc Japan 85A:1–24
Ishii Y (2017) Research trends in snowmelt hazards due to rain-on-snow events. J Jpn Assoc Hydrol Sci 47(2):119–126. https://doi.org/10.4145/jahs.47.119(in Japanese with English abstract)
Ishii Y (2019) Snow hydrological impacts due to rain-on-snow events. Low Temp Sci 77:41–48 (in Japanese with English abstract)
Ito M, Miyoshi T, Masuyama H (2000) The characteristics of the torus self-organizing map. In: Proceedings 16th Fuzzy System Symposium Akita, Japan Society for Fuzzy and Systems, pp. 373–374.
Iwamoto K, Nakai S, Sato A (2008) Statistical analyses of snowfall distribution in the Niigata area and its relationship to the wind distribution. SOLA 4:45–48. https://doi.org/10.2151/sola.2008-012
Jeong DI, Sushama L (2018) Rain-on-snow events over North America based on two Canadian regional climate models. Climate Dyn 50:303–316
Kawase H, Murata A, Mizuta R, Sasaki H, Nosaka M, Ishii M, Takayabu I (2016) Enhancement of heavy daily snowfall in central Japan due to global warming as projected by large ensemble of regional climate simulations. Clim Change 139(2):265–278. https://doi.org/10.1007/s10584-016-1781-3
Kawase H, Sasai T, Yamazaki T, Ito R, Dairaku K, Sugimoto S, Sasaki H, Murata A, Nosaka M (2018) Characteristics of synoptic conditions for heavy snowfall in western to northeastern Japan analyzed by the 5-km regional climate ensemble experiments. J Meteorol Soc Jpn Ser II 96(2):161–178. https://doi.org/10.2151/jmsj.2018-022
Kawase H, Imada Y, Sasaki H, Nakaegawa T, Murata A, Nosaka M, Takayabu I (2019) Contribution of historical global warming to local-scale heavy precipitation in western Japan estimated by large ensemble high-resolution simulations. J Geophys Res 124:6093–6103. https://doi.org/10.1029/2018JD030155
Kohonen T (1982) Self-organized formation of topologically correct feature maps. Biol Cybern 43(1):59–69. https://doi.org/10.1007/bf00337288
McCabe GJ, Clark MP, Hay LE (2007) Rain-on-snow events in the western United States. Bull Am Meteor Soc 88:319–328
Mizuta R, Murata A, Ishii M, Shiogama H, Hibino K, Mori N, Arakawa O, Imada Y, Yoshida K, Aoyagi T, Kawase H, Mori M, Okada Y, Shimura T, Nagatomo T, Ikeda M, Endo H, Nosaka M, Arai M, Takahashi C, Tanaka K, Takemi T, Tachikawa Y, Temur K, Kamae Y, Watanabe M, Sasaki H, Kitoh A, Takayabu I, Nakakita E, Kimoto M (2017) Over 5,000 years of ensemble future climate simulations by 60-km global and 20-km regional atmospheric models. Bull Am Meteorol Soc 98(7):1383–1398. https://doi.org/10.1175/bams-d-16-0099.1
Musselman KN, Lehner F, Ikeda K, Clark MP, Prein AF, Liu C, Barlage M, Rasmussen R (2018) Projected increases and shifts in rain-on-snow flood risk over western North America. Nat Clim Change 8:808–812
Ohba M (2013) Important factors for long-term change in ENSO transitivity. Int J Climatol 33(6):1495–1509. https://doi.org/10.1002/joc.3529
Ohba M (2019) The impact of global warming on wind energy resources and ramp events in Japan. Atmosphere 10:265. https://doi.org/10.3390/atmos10050265
Ohba M, Ueda H (2006) A role of zonal gradient of SST between the Indian ocean and the western pacific in localized convection around the philippines. SOLA 2:176–179. https://doi.org/10.2151/sola.2006-045
Ohba M, Ueda H (2009) Role of nonlinear atmospheric response to SST on the asymmetric transition process of ENSO. J Clim 22(1):177–192. https://doi.org/10.1175/2008jcli2334.1
Ohba M, Sugimoto S (2019) Differences in climate change impacts between weather patterns: Possible effects on spatial heterogeneous changes in future extreme rainfall. Clim Dyn 52:4177–4191. https://doi.org/10.1007/s00382-018-4374-1
Ohba M, Sugimoto S (2020) Impacts of climate change on heavy wet snowfall in Japan. Clim Dyn 54:3151–3164. https://doi.org/10.1007/s00382-020-05163-z
Ohba M, Kadokura S, Yoshida Y, Nohara D, Toyoda Y (2015) Anomalous weather patterns in relation to heavy precipitation events in Japan during the Baiu season. J Hydrometeorol 16(2):688–701. https://doi.org/10.1175/jhm-d-14-0124.1
Ohba M, Kadokura S, Nohara D (2016a) Impacts of synoptic circulation patterns on wind power ramp events in East Japan. Renew Energy 96:591–602. https://doi.org/10.1016/j.renene.2016.05.032
Ohba M, Kadokura S, Nohara D, Toyoda Y (2016b) Rainfall downscaling of weekly ensemble forecasts using self-organizing maps. Tellus A Dyn Meteorol Oceanogr 68(1):29293. https://doi.org/10.3402/tellusa.v68.29293
Ohba M, Kadokura S, Nohara D (2018) Medium-range probabilistic forecasts of wind power generation and ramps in Japan based on a hybrid ensemble. Atmosphere 9(11):423. https://doi.org/10.3390/atmos9110423
Pall P, Tallaksen LM, Stordal F (2019) A Climatology of rain-on-snow events for Norway. J Clim 32:6995–7016. https://doi.org/10.1175/JCLI-D-18-0529.1
Putkonen J, Roe G (2003) Rain-on-snow events impact soil temperatures and affect ungulate survival. Geophys Res Lett. https://doi.org/10.1029/2002GL016326
Rennert KJ, Roe G, Putkonen J, Bitz CM (2009) Soil thermal and ecological impacts of rain on snow events in the circumpolar Arctic. J Clim 22(9):2302–2315
Reusch DB, Alley RB, Hewitson BC (2007) North Atlantic climate variability from a self-organizing map perspective. J Geophys Res 112:D02104. https://doi.org/10.1029/2006jd007460
Sui J, Koehler G (2001) Rain-on-snow induced flood events in Southern Germany. J Hydrol 252(1):205–220
Surfleet CG, Tullos D (2013) Variability in effect of climate change on rain-on-snow peak flow events in a temperate climate. J Hydrol 479:24–34
Tachibana Y (1995) A statistical study of the snowfall distribution on the Japan Sea side of Hokkaido and its relation to synoptic-scale and meso-scale environments. J Meteorol Soc Jpn 73:697–715
Takano I (2002) Analysis of an intense winter extratropical cyclone that advanced along the south coast of Japan. J Meteorol Soc Jpn Ser II 80(4):669–695. https://doi.org/10.2151/jmsj.80.669
Troccoli A, Dubus L, Haupt SE (eds) (2014) Weather matters for energy. Springer, New York, p 528
Ueda H, Kibe A, Saitoh M, Inoue T (2015) Snowfall variations in Japan and its linkage with tropical forcing. Int J Climatol 35(6):991–998. https://doi.org/10.1002/joc.4032
Whitaker AC, Sugiyama H (2005) Seasonal snowpack dynamics and runoff in a cool temperate forest: lysimeter experiment in Niigata, Japan. Hydrol Process 19:4179–4200
White AB, Moore BJ, Gottas DJ, Neiman PJ (2019) Winter storm conditions leading to excessive runoff above California's oroville dam during January and February 2017. Bull Amer Meteor Soc 100(1):55–70
Yamazaki A, Honda M, Kawase H (2019) Regional snowfall distributions in a Japan-Sea side area of Japan associated with jet variability and blocking. J Meteorol Soc Jpn Ser II 97(1):205–226. https://doi.org/10.2151/jmsj.2019-012
Acknowledgements
The Database for Policy Decision making for Future climate change (d4PDF), which is produced under the Program for Risk Information on Climate Change (SOUSEI Program) is used in this study. This research is partially supported by JSPS KAKENHI, grant numbers JP “17K18426” and “19H01377” and the Integrated Research Program for Advancing Climate Models (TOUGOU) Grant Number JPMXD0717935561 from the Ministry of Education, Culture, Sports, Science and Technology (MEXT), Japan.
Author information
Authors and Affiliations
Corresponding author
Additional information
Publisher's Note
Springer Nature remains neutral with regard to jurisdictional claims in published maps and institutional affiliations.
Electronic supplementary material
Below is the link to the electronic supplementary material.
Rights and permissions
About this article
Cite this article
Ohba, M., Kawase, H. Rain-on-Snow events in Japan as projected by a large ensemble of regional climate simulations. Clim Dyn 55, 2785–2800 (2020). https://doi.org/10.1007/s00382-020-05419-8
Received:
Accepted:
Published:
Issue Date:
DOI: https://doi.org/10.1007/s00382-020-05419-8