Abstract
Introduction
In this paper, we investigated whether cholecalciferol supplementation may increase the risk of stone recurrence in patients with calcium nephrolithiasis and Vitamin D deficiency.
Methods
Thirty-three stone formers (56 ± 17 years old, 12 males) with 25(OH)D < 20 ng/mL were considered. Calcium excretion and urine supersaturation with calcium oxalate (ßCaOx) and brushite (ßbsh) were evaluated, both before and after cholecalciferol supplementation. Values of ß > 1 mean supersaturation. Cholecalciferol was prescribed as oral bolus of 100,000–200,000 IU, followed by weekly (5000–10,000 IU) or monthly (25,000–50,000 IU) doses. Calcium intake varied between 800 and 1000 mg/day.
In urine, total nitrogen (TNE) was taken as an index of protein intake, sodium as a marker of dietary intake, and net acid excretion (NAE) as an index of acid–base balance.
Results
TNE, sodium, and NAE did not change during the study (p = ns). Compared to baseline values, after cholecalciferol, both serum calcium and phosphate did not vary (p = ns); 25(OH)D increased from 11.8 ± 5.5 to 40.2 ± 12.2 ng/mL (p < 0.01); 1.25(OH)2D increased from 41.6 ± 17.6 to 54 ± 16 pg/mL (p < 0.01); PTH decreased from 75 ± 27.2 to 56.7 ± 21.1 pg/mL (p < 0.01); urinary calcium increased from 2.7 ± 1.5 to 3.6 ± 1.6 mg/Kg b.w. (p < 0.01); ßbsh increased from 0.9 ± 0.7 to 1.3 ± 1.3 (p = 0.02); whereas ßCaOx varied but not significantly. Before cholecalciferol supplementation, 6/33 patients were hypercalciuric (i.e., urine Ca ≥ 4 mg/Kg b.w.) and increased to 13/33 after cholecalciferol supplementation (pX2 = 0.03).
Conclusions
Cholecalciferol supplementation may increase calcium excretion, or reveal an underlying condition of absorptive hypercalciuria. This may increase both urine supersaturation with calcium salts and stone-forming risk.
Similar content being viewed by others
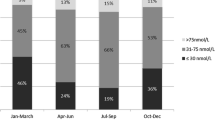
Avoid common mistakes on your manuscript.
Introduction
Over the past decade, a high prevalence of Vitamin D deficiency worldwide was reported [1], but the interpretation of data is controversial [2]. The Endocrine Society defined Vitamin D deficiency as blood levels of 25(OH)D below 20 ng/mL and vitamin D insufficiency as 25(OH)D levels of 21–29 ng/ml [3], whereas the US Institute of Medicine (now the National Academy of Sciences) considered a 25(OH)D serum level of 20 ng/mL to suffice for 97.5% of the population [4].
Elderly subjects, adults, and children with insufficient sun exposure, darker-complexion people, and patients affected by gastro-intestinal diseases with malabsorption reportedly have an increased risk for Vitamin D deficiency and insufficiency [1].
Low levels of 25(OH)D were also observed in subjects not otherwise suspected of being at risk of insufficiency or deficiency, including kidney stone formers. In these patients, some authors found serum 25(OH)D levels lower than in healthy controls [5,6,7], whereas others did not [8, 9]. Overall, stone formers may present with peculiar metabolic features, which will be briefly summarized.
In hypercalciuric patients, the intestinal absorption of calcium is basically higher than in normocalciuric ones. Nevertheless, the intestinal absorption of calcium may be inadequate to counterbalance its urinary excretion, so that negative balance may occur [10], more so in patients on a low-calcium diet [11]. Indeed, low bone mineral density (BMD) [12, 13] and increased risk of bone fracture [14, 15] were reported in these patients. Vitamin D deficiency presumably impairs the intestinal absorption of calcium and worsens low BMD.
In males with calcium nephrolithiasis, Berlin reported a positive correlation between serum 25(OH)D levels and urine calcium excretion [16], but that was not confirmed by Eisner [17]. Nguyen did not observe any association between 25(OH)D serum levels in the range of 20–100 ng/mL and the reported occurrence of kidney stones [18].
As far as the metabolic effects of Vitamin D supplementation on both calcium absorption and excretion are concerned, the available data mostly refer to healthy subjects.
In healthy postmenopausal women without hypercalciuria, randomly assigned to receive either placebo or different daily amounts of Vitamin D (i.e., 800 IU, 2000 IU, and 4000 IU), a significant linear increase of 46Ca intestinal absorption with increasing Vitamin D dose was observed, without evidence of a threshold of calcium absorption with 25(OH)D levels ranging from 16 to 52 ng/mL [19].
Recently, Billington and co-workers demonstrated that Vitamin D supplements increase calcium excretion in normal subjects. Three different groups of both healthy men and postmenopausal women, with normal calcium intake and without Vitamin D deficiency, were assigned to receive Vitamin D either 400, or 4000, or 10,000 IU/day. Compared to the 4.3% baseline prevalence of hypercalciuria, upon supplementation prevalence increased to 17%, 22%, and 31% of patients, respectively [20].
Instead, in patients with calcium nephrolithiasis, the information about the effects of nutritional Vitamin D supplements (i.e., ergocalciferol and cholecalciferol) on both mineral metabolism and urinary stone-forming risk is today still sparse and inconclusive.
Some papers supported the hypothesis that 25(OH)D supplementation, by enhancing intestinal calcium absorption and excretion, may increase urine propensity towards calcium stone formation [6, 21], whereas others did not [22, 23]. In a large prospective study, Ferraro and co-workers did not find any significant relationship between Vitamin D intakes up to about 1300 IU/day and kidney stone formation in healthy subjects [24].
In 5110 subjects of both sexes, without history of nephrolithiasis, Malihi and co-workers reported that monthly supplementation with 100,000 IU of vitamin D, over a median of 3.3 years, was not associated with an increase in the incidence of kidney stone events compared with placebo [25].
By and large, those investigations did not provide detailed information about other potential modulators of calcium metabolism during cholecalciferol therapy, for example, serum levels of PTH and Vitamin D metabolites, markers of bone turnover, and urine excretion of sodium, nitrogen, and ash acid. Therefore, whether cholecalciferol supplementation per se significantly increases the stone-forming risk and recurrence in patients with calcium nephrolithiasis is still an open question.
This paper was aimed at evaluating in more detail the effect of cholecalciferol on both mineral metabolism and urine saturation with calcium salts in kidney stone formers, which had been treated to restore 25(OH)D levels to normal.
Patients and methods
This is a retrospective study, performed on the database of our Kidney Stone Center. In our stone-forming patients, both urinary risk profile and mineral metabolism are routinely studied by means of different sets of analyses, tailored according to individual medical history and disease severity. The most extensive pattern (“mineral metabolism” profile), including calciotropic hormones, was detailed below.
In serum: Sodium, potassium, total and ionised calcium, phosphate, chloride, magnesium, alkaline phosphatase, intact PTH, 25(OH)D, and 1.25(OH)2D.
In 24-h urine: Sodium, potassium, calcium, phosphate, chloride, magnesium, citrate, oxalate, sulphate, uric acid, ammonium, and pH.
In fasting urine: Calcium, creatinine, and deoxypyridinoline.
Among all patients studied with the “mineral metabolism” profile, in 2014 through 2017, 33 consecutive calcium stone formers, of which 12 males (aged 46 ± 22 years) and 21 females (aged 62 ± 10 years), were enrolled, according to the following criteria:
-
(a)
previous course with oral cholecalciferol, prescribed because of 25(OH)D levels lower than 20 ng/mL;
-
(b)
increase of 25(OH)D levels above 30 ng/mL upon cholecalciferol supplementation;
-
(c)
availability of the “mineral metabolism” profile both before and after cholecalciferol treatment.
Patients were enrolled only if neither new prescriptions nor change in dosage of drugs potentially interfering with mineral metabolism (i.e., diuretics, Vitamin D metabolites, bisphosphonates, thyroid hormones, and steroids) had been made during the study. None was taking calcium supplements.
Oral cholecalciferol had been prescribed as an initial bolus of 100,000–200,000 IU (our standard low and high dose of repletion, respectively), followed by a dose of 5000–10,000 IU weekly, or 25,000–50,000 IU monthly (our standard low and high doses of maintenance, respectively). The highest doses were prescribed in case of 25OHD levels < 10 ng/mL; weekly or monthly maintenance doses were prescribed according to patients’ preference.
This study was divided into two phases: “Baseline”, before cholecalciferol supplementation; “Therapy”, after at least 6 months of treatment.
In both the study phases, the estimated dietary intake of calcium was of about 800–1000 mg/day, according to medical instruction, confirmed by dietary interview. Apart from calcium intake, the amount of which was estimated only from patients’ interview, dietary compliance was assayed by measuring total nitrogen, net acid, sulphate, and sodium excretions in 24-h urine, and by estimating gastro-intestinal absorption of alkali from the difference between strong cations and anions [26]. In subjects on stable dietary regimen and body weight, those urinary parameters were reported as reliable markers of dietary compliance, as detailed below.
All patients gave their informed consent to anonymously use their clinical data.
Blood and urine were assayed both in Baseline and Therapy phases. Blood was taken after an overnight fast, while 24-h urinary collection was performed using two separate tanks added with concentrated hydrochloric acid and chlorhexidine as preservatives, as detailed elsewhere [27].
Renal function was estimated by means of creatinine clearance. Main serum and urine chemistries were measured as previously described [27].
The daily amount of urinary calcium was reported in mmol/24 h, as well as normalized values, for both body weight (mg/Kg b.w.) and urinary creatinine (24 h-Ca/Cr, mg/mg). In fasting urine, calcium excretion was normalized for creatinine (fasting Ca/Cr, mg/mg).
Which level of urinary calcium should be taken as “normal” is still debated, so that some considerations are required about the definition of “hypercalciuria”. Daily excretion of urinary calcium varies in a graded fashion. Therefore, dichotomization into normal and abnormal values may not be fully appropriate, but it is essential to investigate on the relative risk of stone formation according to different levels of 24-h urine values [28]. The same holds for the investigations on the genetic basis of calcium excretion [29]. Subsequently, among the varying definitions of “Idiopathic hypercalciuria” that have been provided [10, 30], that reported as a keyword in PubMed MeSH® was used in this paper, namely, a 24-h calcium excretion ≥ 4 mg/kg body weight, in both sexes.
Plasma levels of 25(OH)D and 1.25(OH)2D were evaluated by immunometric assays on Liaison® XL (Diasorin SpA, Saluggia VC, Italy); PTH levels on Immulite 2000Xpi® (Siemens Healthcare, Milano, Italy).
Serum alkaline phosphatase (ALP) was taken as a marker of bone formation and urine deoxypyridinoline (DPD) as a marker of bone resorption.
The net variations of PTH, 25(OH)D, 1.25(OH)2D and daily urinary calcium from Baseline to Therapy phase were reported as ∆PTH (pg/mL), ∆25(OH)D (ng/mL), ∆1.25(OH)2D (pg/mL), and ∆uCa (mmol/24 h), respectively.
Concerning nutritional markers, total nitrogen excretion (TNE) was calculated as previously described [27] and was considered as an index of the whole dietary protein intake [31], whereas urinary sulphate was taken as a marker of animal protein intake [32] and urinary sodium as a marker of salt daily intake [33].
Net acid and base balances were assessed from both urinary net acid excretion (NAE, mEq/24 h) and the net gastro-intestinal absorption of alkali (GI-Alk, mEq/24 h), which were calculated according to previous papers [26, 27].
In 24-h urine, supersaturation with calcium salts, namely, calcium oxalate (ßCaOx) and calcium phosphate (ßbsh), was calculated by means of a dedicated software (Lithorisk2®), which uses results of the main urinary cations and anions. Values of ß > 1 mean supersaturation, whereas values < 1 mean undersaturation [34].
Statistical analysis was performed by means of Analyse-it for Microsoft Excel®. Results were reported as mean ± standard deviation. The comparison of means was performed by Wilcoxon signed-rank test and comparison of percentages by means of Chi-square (χ2) test.
p values ≤ 0.05 were considered statistically significant.
Results
Tables 1, 2, 3 list results labelled as Baseline or Therapy, according to the phase of the study.
No variations between Baseline and Therapy were seen for creatinine clearance, body weight and nutritional markers (Table 1). The same applied for serum levels of calcium, phosphate, and alkaline phosphatase, whereas urine DPD was slightly reduced in Therapy (p = 0.02 vs Baseline) (Table 2).
Cholecalciferol supplementation was followed by a significant increase of plasma levels of both 25(OH)D and 1.25(OH)2D (p < 0.01) (Table 2). The net variations of their plasma concentrations between Baseline and Therapy phases were positively correlated, as described by the following equation:
PTH levels showed a significant reduction in Therapy compared to Baseline (p < 0.01) (Table 2). There was an inverse relationship between PTH and 25(OH)D variations, according to the following equation:
Interestingly, daily calcium excretion significantly increased in Therapy (p < 0.01 vs Baseline). Conversely, fasting Ca/Cr ratio did not change between the two study phases (Table 3).
There were no significant correlations between ∆uCa and both ∆25(OH)D and ∆1.25(OH)2D.
Urinary oxalate, phosphate, citrate, magnesium, pH, and volume remained unchanged (Table 3).
ßCaOx showed a mild, not significant, increase in Therapy (p = ns), whereas ßbsh increased significantly by of 44% (p = 0.02) (Table 3).
The incidence of hypercalciuria increased from 18.2% (6/33) to 39.4% (13/33), in Baseline vs Therapy, respectively (pX2 = 0.03). Eventually, 7 out of 13 patients who were hypercalciuric in Therapy had normocalciuria when they had vitamin D deficiency (Fig. 1).
However, even among the 20 patients who remained normocalciuric over the two phases of the study, urinary calcium increased in Therapy vs Baseline (2.5 ± 1.0 vs 1.9 ± 0.9 mg/Kg b.w.; p = 0.002) (Fig. 2). The same occurred for ßbsh (1.2 ± 1.0 vs 0.8 ± 0.7; p = 0.010).
Discussion
In stone-forming patients, some authors reported a meaningful prevalence of Vitamin D deficiency [5, 6].
On the other hand, some concern has been raised about the potentially adverse effects of Vitamin D supplementation on both the calcium excretion and the propensity to lithogenic salts crystallization in urine, so that the safety of the treatment of Vitamin D deficiency in stone-forming patients is still debated [6, 21, 22, 24, 25].
To date, nutritional Vitamin D supplementation has never been specifically discouraged in stone-forming patients with severe Vitamin D deficiency, probably due to the putative beneficial effects of Vitamin D not only on skeletal health.
The purpose of our study was to investigate whether a course of therapy with cholecalciferol, aimed at resetting low levels of circulating 25(OH)D to normal, may induce unwanted effects on the stone-forming risk in calcium nephrolithiasis.
To achieve this goal, we designed a comprehensive panel of exams including, in addition to urinary stone risk profile, calciotropic hormones, markers of bone turnover, acid–base balance, and diet-related parameters.
After cholecalciferol supplementation, the average level of 25(OH)D became about four times as high as in Baseline (Table 2). The parallel increase of plasma levels of 1.25(OH)2D, together with a highly significant correlation between the changes of 25(OH)D and 1.25(OH)2D (p < 0.01), suggests that the increased availability of calcifediol promoted the synthesis of calcitriol.
Correspondingly, the inverse linear correlation between the changes of PTH and 25(OH)D (p < 0.01) can be explained by both an indirect effect (mediated by the increased synthesis of calcitriol) and a direct activity of cholecalciferol on PTH secretion [35, 36].
In Therapy, urinary DPD was significantly lower than in Baseline (p = 0.02), thereby suggesting a reduction of bone resorption.
In patients taken as a whole, daily calcium excretion was increased by more than 30% in Therapy vs Baseline, including 20 patients who never presented hypercalciuria throughout the study (Fig. 2). The fact that the dietary-related parameters did not vary supports the contention that the above changes of calcium excretion were not secondary to changed intakes of the nutrients known to affect renal calcium handling (i.e., sodium, animal protein, ash acid, and alkali).
The daily fractional excretion of calcium (24 h-Ca/Cr) was significantly higher in Therapy compared to Baseline, whereas fasting Ca/Cr did not vary. These results are in agreement with Billington and co-workers, who also reported a discrepancy between 24-h urinary calcium excretion and fasting Ca/Cr, following the administration of different doses of Vitamin D [20]. The lack of correlations between changes of urinary calcium and both 25(OH)D and 1.25(OH)2D levels lends us to speculate that the action of Vitamin D on calciuria may not only ensue from increase in intestinal absorption of dietary calcium, but also from concurrent modulations of both parathyroid function and bone turnover [37].
In Therapy, the prevalence of patients with hypercalciuria (39.4%) was consistent with the literature reports [12, 28], unlike the low prevalence (18.2%) observed in Baseline. This suggests that, in some stone formers, an underlying absorptive hypercalciuria can be unmasked by the correction of hypovitaminosis D.
In Therapy, the significant increase of urinary calcium compared to Baseline went together with a parallel significant increase of ßbsh, to which slight increases of urinary phosphate and pH may have contributed. A small increase in ßCaOx did not reach a significance level because of tiny unexpected decrease in urine oxalate.
Our data on urine supersaturation partly confirm those of Ferroni and co-workers. By comparing patients who received 1000 IU of Vitamin D daily and those who received 50,000 IU of Vitamin D weekly, they did not find any significant variation in urine supersaturation with CaOx, whereas there was a significant increase in urine supersaturation with calcium phosphate in those given 50,000 IU of Vitamin D. Conversely, these authors did not report any significant variation in daily calcium excretion between the two groups, irrespective of the Vitamin D dosage prescribed [23].
Our retrospective study has some limitations. The relatively small sample size may have reduced the power to detect more subtle differences in our results. Dietary calcium intake was not controlled, being assessed only from dietary inquiry. Vitamin D was assayed by a fully automated chemiluminescence immunoassay, which, despite having lower accuracy by comparison with liquid chromatography–tandem mass spectrometry [38], has shown acceptable reliability for routine use.
Besides that, to the best of our knowledge and belief, this is the first study wherein changes of urine calcium upon Vitamin D supplementation were also controlled for potential interferences exerted by diet, as deducted by urinary markers of salt, protein, ash acid, and alkali metabolism.
Conclusions
In stone formers with Vitamin D deficiency, the reduced urinary excretion of calcium ensuing from lower intestinal absorption might have a favourable effect on the risk of stone formation with calcium salts. However, especially if low dietary calcium intakes concur, negative calcium balances will result in reduction of bone mineral content over time [11, 12].
Cholecalciferol supplementation, aimed at restoring physiological levels of circulating 25(OH)D, is expected to improve calcium balance and favour bone health, on one hand, but may also increase both calcium excretion and the urinary stone-forming risk, on the other hand.
Therefore, when stone formers are given cholecalciferol supplementation, urine biochemistries must be monitored and metaphylaxis carefully tailored as needed (large water intake, potassium citrate, and thiazides).
References
Holick MF (2017) The vitamin D deficiency pandemic: approaches for diagnosis, treatment and prevention. Rev Endocr Metab Disor 18:153–165
Manson JE, Brannon PM, Rosen CJ, Taylor CL (2016) Vitamin D deficiency—is there really a pandemic? N Engl J Med 375(19):1817–1820
Holick MF, Binkley NC, Bischoff-Ferrari HA et al (2011) Endocrine society. Evaluation, treatment, and prevention of vitamin D deficiency: an endocrine society clinical practice guideline. J Clin Endocrinol Metab 96(7):1911–1930
Ross AC, Manson JE, Abrams SA et al (2011) The 2011 report on dietary reference intakes for calcium and vitamin D from the Institute of Medicine: what clinicians need to know. J Clin Endocrinol Metab 96(1):53–58
Giron-Prieto MS, Cano-Garcia MC, Arrabal-Polo MA et al (2016) Analysis of vitamin D deficiency in calcium stone-forming patients. Int Urol Nephrol 48:1243–1246
Johri N, Jaeger P, Ferraro PM et al (2017) Vitamin D deficiency is prevalent among idiopathic stone formers, but does correction pose any risk? Urolithiasis 45(6):535–543
Ticinesi A, Nouvenne A, Ferraro PM et al (2016) Idiopathic calcium nephrolithiasis and hypovitaminosis D: a case-control study. Urology 87:40–45
Tang J, McFann KK, Chonchol MB (2012) Association between serum 25-hydroxyvitamin D and nephrolithiasis: the National Health and Nutrition Examination Survey III, 1988–1994. Nephrol Dial Transplant 27:4385–4389
Taylor EN, Hoofnagle AN, Curhan GC (2015) Calcium and phosphorus regulatory hormones and risk of incident symptomatic kidney stones. Clin J Am Soc Nephrol 10:667–675
Worcester EM, Coe FL (2008) New Insights into the pathogenesis of idiopathic hypercalciuria. Semin Nephrol 28(2):120–132
Fuss M, Pepersack T, Van Geel J et al (1990) Involvement of low-calcium diet in the reduced bone mineral content of idiopathic renal stone formers. Calcif Tissue Int 46(1):9–13
Letavernier E, Traxer O, Daudon M et al (2011) Determinants of osteopenia in male renal-stone–disease patients with idiopathic hypercalciuria. Clin J Am Soc Nephrol 6:1149–1154
Jaeger P, Lippuner K, Casez JP et al (1994) Low bone mass in idiopathic renal stone formers: magnitude and significance. J Bone Miner Res 9(10):1525–1532
Taylor EN, Feskanich D, Paik JM, Curhan GC (2016) Nephrolithiasis and risk of incident bone fracture. J Urol 195(5):1482–1486
Lauderdale DS, Thisted RA, Wen M, Favus MJ (2001) Bone mineral density and fracture among prevalent kidney stone cases in the Third National Health and Nutrition Examination Survey. J Bone Miner Res 16(10):1893–1898
Berlin T, BjorkhemI CL et al (1982) Relation between hypercalciuria and vitamin D3-status in patients with urolithiasis. Scand J Urol Nephrol 16:269–273
Eisner BH, Thavaseelan S, Sheth S et al (2012) Relationship between serum vitamin D and 24 h urine calcium in patients with nephrolithiasis. Urology 80:1007–1010
Nguyen S, Baggerly L, French C et al (2014) 25-Hydroxyvitamin D in the range of 20 to 100 ng/mL and incidence of kidney stones. Am J Public Health 104:1783–1787
Aloia JF, Dhaliwal R, Shieh A et al (2014) Vitamin D supplementation increases calcium absorption without a threshold effect. Am J Clin Nutr 99(3):624–631
Billington EO, Burt LA, Rose MS et al (2020) Safety of high-dose vitamin D supplementation: secondary analysis of a randomized controlled trial. J Clin Endocrinol Metab 105(4):1–13
Malihi Z, Wu Z, Stewart AW et al (2016) Hypercalcemia, hypercalciuria, and kidney stones in long-term studies of vitamin D supplementation: a systematic review and meta-analysis. Am J Clin Nutr 104:1039–1051
Leaf DE, Korets R, Taylor EN et al (2012) Effect of vitamin D repletion on urinary calcium excretion among kidney stone formers. Clin J Am Soc Nephrol 7(5):829–834
Ferroni MC, Rycyna KJ, Averch TD, Semins MJ (2017) Vitamin D repletion in kidney stone formers: a randomized controlled trial. J Urol 197:1079–1083
Ferraro PM, Taylor EN, Gambaro G, Curhan GC (2017) Vitamin D Intake and the risk of incident kidney stones. J Urol 197:405–410
Malihi Z, Lawes CMM, Wu Z et al (2019) Monthly high-dose vitamin D supplementation does not increase kidney stone risk or serum calcium: results from a randomized controlled trial. Am J Clin Nutr 109(6):1578–1587
Oh MS (1989) A new method for estimating G-I absorption of alkali. Kidney Int 36:915–917
Vitale C, Marangella M, Cosseddu D et al (1997) Clinical and biochemical patterns of presentation in monolateral and bilateral calcium nephrolithiasis. Clin Nephrol 47(1):23–27
Curhan GC, Willett WC, Speizer FE, Stampfer MJ (2001) Twenty-four hour urine chemistries and the risk of kidney stones among women and men. Kidney Int 59(6):2290–2298
Stechman MJ, Loh NY, Thakker RV (2009) Genetic causes of hypercalciuric nephrolithiasis. Pediatr Nephrol 24(12):2321–2332
Song L, Maalouf NM (2017) 24-hour urine calcium in the evaluation and management of nephrolithiasis. JAMA 318(5):474–475
Bingham SA (2003) Urine nitrogen as a biomarker for the validation of dietary protein intake. J Nutr 133(Suppl 3):921S–924S
Magee EA, Curno R, Edmond LM, Cummings JH (2004) Contribution of dietary protein and inorganic sulfur to urinary sulfate: toward a biomarker of inorganic sulfur intake. Am J Clin Nutr 80(1):137–142
McCarron DA, Kazaks AG, Geerling JC et al (2013) Normal range of human dietary sodium intake: a perspective based on 24-Hour urinary sodium excretion worldwide. Am J Hypertens 26(10):1218–1223
Marangella M, Daniele PG, Ronzani M et al (1985) Urine saturation with calcium salts in normal subjects and idiopathic calcium stone formers estimated by an improved computer model system. Urol Res 13:189–193
Ritter CS, Armbrecht HJ, Slatopolsky E, Brown AJ (2006) 25-Hydroxyvitamin D3 suppresses PTH synthesis and secretion by bovine parathyroid cells. Kidney Int 70:654–659
Al-Aly Z, Qazi RA, González EA et al (2007) Changes in serum 25-hydroxyvitamin D and plasma intact PTH levels following treatment with ergocalciferol in patients with CKD. Am J Kidney Dis 50(1):59–68
Christakos S, Dhawan P, Porta A et al (2011) Vitamin D and intestinal calcium absorption. Mol Cell Endocrinol 347(1–2):25–29
Jones G (2015) Interpreting vitamin D assay results: proceed with Caution. Clin J Am Soc Nephrol 10(2):331–334
Author information
Authors and Affiliations
Contributions
CV: project development, data analysis, manuscript writing; MM: data analysis, manuscript editing; FB: data management; LF: data collection; AT: data collection.
Corresponding author
Ethics declarations
Conflict of interest
The authors declare that they have no conflict of interest.
Research involving human participants and/or animals
All procedures performed in studies involving human participants were in accordance with the ethical standards of the institutional and/or national research committee and with the 1964 Helsinki declaration and its later amendments or comparable ethical standards.
Informed consent
Informed consent was obtained from all individual participants involved in the study.
Additional information
Publisher's Note
Springer Nature remains neutral with regard to jurisdictional claims in published maps and institutional affiliations.
Rights and permissions
About this article
Cite this article
Vitale, C., Marangella, M., Bermond, F. et al. Metabolic effects of cholecalciferol supplementation in patients with calcium nephrolithiasis and vitamin D deficiency. World J Urol 39, 597–603 (2021). https://doi.org/10.1007/s00345-020-03222-y
Received:
Accepted:
Published:
Issue Date:
DOI: https://doi.org/10.1007/s00345-020-03222-y