Abstract
A unique feature of leguminous plants is the establishment of symbiotic bacterial genera inside root or stem nodules that is being recently re-evaluated for investigating the micro-flora discrete to nitrogen fixation. The present research was carried out to evaluate non-rhizobial endophytes and Rhizobium from root nodules of Vigna radiata and ascertain their co-inoculation effect in pot and field conditions. Each strain displayed one or more plant growth-promoting behaviors in varying degrees. The ability to fix nitrogen was observed in all strains; however, a noticeable enhancement in nitrogen fixation was observed when all three strains were co-inoculated. All three strains were found to possess the nifH gene, which plays a key role in the nitrogen fixation process. However, only Rhizobium sp. AAU B3 also had the nodD gene present. Furthermore, combinations of all three strains produced the highest levels of phosphate solubilization, potash mobilisation, Indole Acetic Acid (IAA), and the stress-relieving enzyme 1-aminocyclopropane-1-carboxylic acid (ACC) deaminase. Interestingly, the succession of the root nodule formation within root hairs seedlings was observed under a fluorescence microscope and two NRE were found to be located inside the root nodules, indicating that they are endophytic. Additionally, a pot and field investigation revealed that the combination of chosen Rhizobium and NRE strains had a favorable impact on the growth and yield characteristics of a green gram. Selected bio-inoculants can reduce the utilization of synthetic fertilizers by 75%, which might lead to the restoration of the soil’s health. Therefore, these bio-inoculants might be explored commercially for sustainable agriculture production.
Similar content being viewed by others
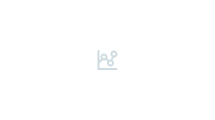
Avoid common mistakes on your manuscript.
Introduction
Farmers frequently employ more nitrogen, phosphate, or potash fertilizers, as well as other chemical fertilizers, to boost crop production [1]. In fact, the so-called “Green Revolution” of the 1960s was brought about by the development of the technology for the manufacture of chemical fertilizers in the 1930s–1950s as well as by other scientific advancements in agriculture supported by governments and businesses [2]. Around the world, the “Green Revolution” had many benefits, but over time, it also had unfavorable effects and downsides that are still noticeable today [3]. Chemical fertilizer use has a negative impact on the environment, contributing to issues such as soil acidification or salinity, deterioration of indigenous crops or microorganisms, overexploitation of genetic resources, water eutrophication, and air pollution [3,4,5,6,7]. As a consequence, it is becoming increasingly critical to boost production employing sustainable techniques by avoiding or at least minimizing the usage of chemical fertilizers. The majority of the issues brought on by the use of chemical fertilizers can be resolved using “greener” fertilizers, especially biofertilizers, which are mostly based on plant growth-promoting bacteria (PGPB). Potentially beneficial bacteria for plants possess a diverse range of characteristics that can benefit the plants. They are generally involved in nutrient acquisition via nitrogen fixation, phosphate and potash solubilization as well as production of growth hormones. IAA is involved in different plant growth and development processes such as the formation of the lateral roots and root hairs as well as increases the primary root length [8]. IAA production was reported as common in plant-associated bacteria as part of a colonization strategy that involves phytostimulation and circumvention of plant defense mechanisms [9]. The rhizobium-based bioinoculants are more frequently employed for leguminous crops than other biofertilizers due to their better ability to fix nitrogen by symbiosis.
The legume family, which includes 730 genera and more than 19,320 species, is one of the largest plant families in the world [10]. An essential pulse crop, green gram (Vigna radiata L.), is a member of the subfamily papilionaceae of the Leguminosae family. Pulses are well-known for the three “Fs”: Fodder, food, and fertilizer. In terms of nutritional value (proteins, amino acids and fibers) among pulses, green gram has been given a higher ranking next to gram and black gram [11]. In addition to its significance for agriculture, its most significant characteristic is that it contributes to 80% of biological nitrogen fixation. This is made possible by the symbiotic association that rhizobia create with root nodule bacteria.
The signaling events between symbiotic rhizobia and leguminous plants are highly explicit. The rhizobial cells secretes specified lipopolysaccharides called nod factors at the binding sites on roots of leguminous plants thereby initiating symbiosis process systematically. Highly specific nod factors and oxygen sensitive nitrogenase enzyme promotes the growth of only narrow range of microbes inside the microaerophilic nodules [12]. Although the specificity and microaerophilic conditions present inside root nodules; it is well documented and reported that the diverse group of non-rhizobial endophytes (NRE) were present inside nodules [13]. Generally, NRE gets colonized in infection thread that initiated by host specific rhizobia by breaking the host’s specificity. To study the nodule symbiosis or plant–microbe interactions use of advanced fluorescence microscopic techniques were being used by researchers world-wide. The detection of fluorescence cells of NRE isolates inside root nodule tissue confirms the accommodation of nodules by NRE bacteria strains. The genera inside root nodules may include Bacillus, Pseudomonas, Rhizobium, Xanthomonas, [14], A. rhizogenes, Phyllobacterium, Stenotrophomonas, Agrobacterium tumefacien, Enterobacteriaceae, Bacillus, Bordetella, Curtobacterium, Pantoea [15], Brevibacillus brevis, Paenibacillus sp., Pantoea agglomerans, [16] and many members of the phylum Actinobacteria including Agromyces and Microbacterium sp. [17, 18], Curtobacterium [19] and Micromonospora [20,21,22]. Although the invasive NRE inside the rood nodule has some phytobeneficial effects, the precise method by which this occurs is uncertain. In conjunction with Rhizobium sp., most NRE may help the host plants in numerous ways, including by increasing the number of nodules, encouraging aerial growth, and nutrient intake. Nonetheless, NRE does not actively contribute to the induction of root nodules.
The utilisation of PGPBs as consortia enhances not only agricultural output but also the quality of grains, fruits, and processed items from these crops [23]. Attractive from an economic and environmental standpoint, the development and deployment of single and mixed inoculants based on chosen PGPBs is now a viable option to the long-term use of synthetic fertilizers in agricultural areas. The general public has become interested in the possible application of these advantageous PGPBs in biofertilization systems. It has been demonstrated in several studies that inoculating PGPBs in consortia offers plants a variety of advantages by co-inoculating NRE and Rhizobium strains in plants [24,25,26]. The majority of this research, however, was conducted under controlled lab and greenhouse circumstances, while just a few studies being conducted in the field [23].
There is a paucity of information, regarding the isolation of native root endogenous endophytes, their function in nutrient bioavailability, growth stimulation, and interaction with rhizobia in green gram. Additionally, despite having so high potential, green gram is frequently produced in marginal areas with few inputs, rendering it vulnerable to numerous abiotic and biotic challenges that result in significant yield losses. Environmental factors like drought, abiotic stresses and salinity in the subsoil, which can limit root growth and hinder a plant’s ability to draw moisture and nutrients from the soil.
These studies aimed to identify the key endophytic bacteria found in the root nodules of green gram plants and investigate their impact on plant nutrient acquisition. The microbiota of green gram was examined for its potential to enhance plant growth, with particular focus on utilizing nodule endophytes as a valuable resource in soil microbiology. The endophytic bacteria from the surface-sterilized root nodules of Vigna radiata were evaluated for their ability to promote plant growth and their interactions with different Rhizobium species. These NRE isolates further confirmed for their residence inside the root nodules by studying their succession of the root nodule infection thread formation within root hairs of Vigna radiata seedlings was observed under a fluorescence microscope after seed inoculation with tagged NRE isolates with 4′, 6-diamidino-2-phenylindole (DAPI). Lastly, the co-inoculation effect of Rhizobium and NRE on Vigna radiata growth was examined through pot and field study.
Materials and Methods
Bacterial Strains
The bacterial isolates Rhizobium sp. AAU B3 (GenBank® ACCN MH701891), Bacillus sp. AAU B6 (GenBank® ACCN MH701892) and Bacillus sp. AAU B12 (GenBank® ACCN MH701893) and R. selenitireducens AAU M1 were obtained from Department of Microbiology and Biofertilizer project, Anand Agricultural University, Anand [27].
Compatibility Study of Rhizobium and NRE
The Rhizobium sp. AAU B3, R. selenitireducens AAU M1 and NRE (Bacillus sp. AAU B6 and Bacillus sp. AAU B12) were cross streaked to test their compatibility with each other.
Plant Growth-Promoting Traits of Isolates
N2 Fixation
Nitrogen fixation was determined by the quantitative Micro-Kjeldahl method described by AOAC [28] and sugar utilization was estimated using Fehling’s method [29]. PCR amplification of region coding for Fe protein (component II) of the nitrogenase enzyme complex was studied as described by Dhole et al. [30]. The Fragments of nodD genes were amplified using two primer sets (NBA12- 5′GGATSGCAATCATCTAYRGMRTGG3′, NBF120- 5′GGATCRAAAGCATCCRCASTATGG3′, Y5- 5′ ATGCGKTTYARRGGMCTNGAT 3′, and Y6- 5′ CGCAWCCANATRTTYCCNGGRTC 3′) [31, 32]. The amplification was carried out as described by Laguerre et al. [32] with Mastercycler personal (Eppendorf, Germany) with the following PCR conditions: initial denaturation at 94 °C for 2 min, denaturation at 93 °C for 1 min, annealing at 55 °C for 1 min, extension at 72 °C for 2 min for 35 cycles, and final extension at 72 °C for 10 min.
Phosphate and Potassium Solubilization
Phosphate solubilization activity of all isolates individually as well as in combinations with each other was determined on Sperber’s agar plates (Glucose-10.0 g, CaCO3- 05.0 g, Yeast Extract 05.0 g, 10% K2HPO4—20 ml, MgSO4.7H2O—0.25 g, CaCl2—0.1 g, Agar—15 g, Distilled water—1000 ml, pH—7.0) by the method described by Taurian et al. [33]. Bacterial isolates were inoculated using a sterile needle under aseptic conditions and were incubated at 30 ± 2 °C for five days with observation on colony diameter and solubilization of TCP every 24 h. A clear zone around the growing colony indicated the phosphate solubilization activity.
All the isolates were spot inoculated on Aleksandrov agar [34] containing mica for testing potassium substrate solubilization. Plates were incubated at 30 + 2 °C for five days with observation on colony diameter every 24 h. Clear zone formation around the growing colony indicated potassium solubilization activity.
Phytohormones and ACC Deaminase Production
All the isolates were grown in glucose phosphate broth containing L-tryptophan (0.005 M) for 3 days at 30 + 2 °C on a shaker at 100 rpm and then centrifuged at 3000 rpm for 20 min as described by Glickmann and Dessaux, [35]. One ml supernatant was mixed with 2 ml of Salkowaski’s reagent. Un-inoculated control was kept for comparison. The intensity of pink color developed within 30 min was measured at 535 nm in UV/VIS spectrophotometer. The quantity of IAA was determined by comparison with an IAA standard curve.
Screening of bacterial isolates for ACC deaminase enzyme production was done based on their ability to use ACC as a sole nitrogen source in the minimal medium. Cultures were spot inoculated on Petri plates containing minimal medium supplemented with 3 mM ACC substrate. Plates containing minimal medium without ACC were as negative control and with (NH4)2SO4 (2.0 g l−1) as a nitrogen source served as a positive control. The plates were incubated for 3 to 4 days at 28 ± 2 °C. The growth of isolates on ACC supplemented plates was compared with positive and negative control plates. Isolates growing well on ACC plates were considered ACC deaminase enzyme producers [36].
Endophytic Nature Confirmation of NRE by DAPI Staining
The DAPI (Sigma Chemical Co., St Louis, MO, USA) 10 mg ml−1 stock was prepared in sterile distilled water and stored at −20 °C. Bacteria were stained according to the procedure described by Mukharjee and Ray, [37] with some modifications as follows: fresh culture (24 h after inoculation) was taken in 2 ml tubes and centrifuged at 5000 rpm for 5 min; the supernatant was discarded. The pallet was then washed with 400 µl phosphate-buffered saline (PBS) three times. Then the cells were fixed with 200 µl of 4% formaldehyde (prepared in PBS) and incubated at 28 ± 2 °C in dark for 30 min. Again, the pallet was washed with 400 µl phosphate-buffered saline (PBS) three times. Then the cells were mixed with DAPI (20 µg ml−1 working solution) and incubated in dark for 1 h. Slides were prepared and observed under Epifluorescence Microscope (Olympus CKX 41) with 100-time magnification. The tagged bacteria were inoculated by seed treatment in green gram in vitro and monitored for colonization from 7 days after inoculation (DAI). Roots from gnotobiotic seedlings were carefully removed; surface sterilized and thin Sections (50–100 mm) were prepared aseptically and were observed under Epifluorescence Microscope (Olympus CKX 41) under 100-time magnification for the presence of fluorescently tagged bacteria.
Co-inoculation Study of Rhizobium sp. and NRE in Green Gram Through Pot Study
The soil of the experimental pots was deep black loam sand with the composition of coarse sand (0.53%), fine sand (82.20%), silt (10.55%), and clay (5.12%) and locally known as “Goradu”, alluvial in origin and belongs to the order Alfisol [38]. The soil had pH 7.0 and electrical conductivity of 0.30 dSm−1 at 25 °C. The soil was well-drained and retentive of moisture having organic carbon (0.36%), available N (232.5 kg ha−1), available P (40.18 kg ha−1), and available K2O (450.0 kg ha−1). It responded well to irrigation as well as manuring and was reasonably suitable for green gram cultivation.
Seeds of green gram were surface sterilized with 70% ethanol solution for 30 s, followed by treatment with 0.1% HgCl2 for 2 min, and then washed three times with sterile distilled water for 1 min each under aseptic conditions. Seeds were then treated with individual 24-h-old isolates grown in nutrient broth at 28 ± 2 °C containing 108 cells ml−1, keeping untreated seeds as control. Treatment details were: T1- control, T2- 100% Farm Yard Manure (FYM), T3-50% FYM, T4- 100% Recommended Dose of Nitrogen Fertilizer (RDNF), T5- R. selenitireducens AAU M1, T6- Rhizobium sp. AAU B3, T7- Bacillus sp. AAU B6, T8- Bacillus sp. AAU B12, T9- T5 + T7, T10- T5 + T8, T11-T5 + T7 + T8, T12-T6 + T7, T13-T6 + T8, and T14- T6 + T7 + T8. Each treatment was repeated four times.
The percentage of germination was recorded at 10 days after sowing (DAS) and plant height after 15, 30, 45, and 60 DAS. After harvest, quantification was carried out for root and shoot length, fresh weight, dry weight, nodules per plant, bacterial count of the soil before sowing and at harvest.
The data collected on different experiments and parameters were subjected to statistical analysis using Completely Randomized Block Design (CRD). Data were subjected to analysis of variance and means and were compared by Duncan’s New Multiple Range Test (DNMRT) [39].
Co-inoculation Study of Rhizobium sp. and NRE in Green Gram Through Field Study
The effect of co-inoculation of Rhizobium and NRE on green gram growth was examined through field study through seed inoculation. The green gram seeds were treated with individual 24-h-old isolates grown in nutrient broth containing approximately 108 cells ml−1, keeping untreated seeds as control. Treatment details and observations were the same as in the pot study. Each treatment was replicated four times.
Statistical Analysis
The data collected on different experimental parameters were subjected to statistical analysis using Randomized Block Design (RBD) for field study with significance set at a probability level of 0.05. Data were subjected to analysis of variance and means compared by DNMRT [39].
Results
Plant Growth-Promoting Traits
N2 Fixation
All the 3 isolates were capable of fixation of nitrogen and the highest 54.06 mg N g−1 of glucose consumed was demonstrated by the combination of all the three isolates Rhizobium sp. AAU B3 + Bacillus sp. AAU B6 + Bacillus sp. AAU B12, followed by the combined effect of Rhizobium sp. AAU B3 and Bacillus sp. AAU B6 (47.53 mg N g−1 of glucose consumed). The lowest nitrogen fixation was observed in the case of Bacillus sp. AAU B12 (16.86 mg N g−1 of glucose consumed). Rhizobium sp. AAU B3 individually exhibited nitrogen fixation 45.20 mg N g−1 of glucose consumed.
The presence of the nifH gene was detected by PCR amplification of region encoding for Fe-protein (component II) of the nitrogenase enzyme complex. The selected primer pair selectively amplified component-II coding region (ranging from 250 to 500 bp) based upon the organism and its nif gene sequence. All the three isolates gave a single band of size −390 bp indicating these isolates were diazotrophs (Fig. 1a).
PCR Amplification of nifH (a) and nodD gene (b) observed in rhizobial and non-rhizobial isolates. nifH amplification observed in all the three isolates whereas, only Rhizobium sp. can amplify the nodD gene. (B3-Rhizobium sp. AAU B3; B6- Bacillus sp. AAU B6; B12- Bacillus sp. AAU B12 and M- 100 bp Ladder)
The nodD gene is a part of nod factors in Rhizobium which are essential for nodulation. The set of primers (NBA12 and NBF120) target the nod box regulatory elements of nodA and nodF, respectively. All the three endophytic isolates were tested for the nodD gene amplification and found that Rhizobium sp. AAU B3 gave a single band of −980 bp size (Fig. 1b). The other two isolates (Bacillus sp. AAU B6 and Bacillus sp. AAU B12) did not show any band proving that they were unable to form root nodules.
Phosphate and Potassium Solubilization
All three isolates were tested for their phosphate solubilization capacity on Sperber media containing Tri-calcium phosphate as an insoluble source. The maximum P solubilization zone was recorded for Bacillus sp. AAU B6 which was NRE strain and formed a clear zone of 1.5 mm diameter with 1.10 SI on Sperber’s agar plate (Table 1). However, when inoculated in combination with Rhizobium sp. AAUB3, both the NRE isolates demonstrated higher SI. The combination of all the three isolates, Rhizobium sp. AAU B3 + Bacillus sp. AAU B6 + Bacillus sp. AAU B12 proved to report higher SI 2.37 as compared to individual inoculants.
Rhizobium sp. AAU B3 and two NRE strains (Bacillus sp. AAU B6 and Bacillus sp. AAU B12) were inoculated individually and in combinations on Aleksandrov agar containing mica as an insoluble source of potassium. All three strains when inoculated together showed significant potash solubilization. However, when all the strains inoculated in the consortium was found to report the highest potassium solubilization index (2.98) as shown in Table 1. Moreover, Rhizobium sp. AAU B3 has shown enhanced potassium solubilization in combination with both the NRE Bacillus sp.
Phytohormones and ACC Deaminase Production
The variable response was shown by strains in terms of IAA production as shown in Table 1. IAA production by the different combinations was found ranging between 97.33 and 165.50 µg ml−1. The highest IAA production 165.50 µg ml−1 was demonstrated by a consortium containing all three strains Rhizobium sp. AAU B3, Bacillus sp. AAU B6 and Bacillus sp. AAU B12, followed by the combination of Rhizobium sp. AAU B3and Bacillus sp. AAU B6 (148.17 µg ml−1). However, the lowest IAA production was demonstrated by Rhizobium sp. AAU B3 (97.33 µg ml−1). From the results, it is observed that the combination of rhizobial strain with NRE increased IAA production considerably.
All the strains grew well on positive control plates containing (NH4)2SO4 as nitrogen source and were able to grow on plates containing ACC as the sole nitrogen source and confirmed the production of ACC deaminase enzyme. However, it was observed that when all the three strains were inoculated simultaneously improved the growth on medium containing ACC as the sole nitrogen source.
Confirmation of Endophytic Nature of NRE
The succession of the infection thread formation within root hairs of green gram seedlings was observed under a fluorescence microscope (Olympus CKX 41) at 7 DAI. Figure 2A and B demonstrated the fluorescent NRE (Bacillus sp. AAU B6 and Bacillus sp. AAU B12) cells at 48 h of re-inoculation in the fresh medium after tagging with DAPI and by exciting with blue and green light. It was observed that with different wavelengths, NRE (Bacillus sp.) excited and clearly showed rod (bacilli)-shaped cells. These cells were used for surface sterilized seed inoculation to observe the colonization and infection thread development in green gram seedlings under gnotobiotic conditions.
The tagged NRE was only attached to the base of root hairs and root tips but failed to entre root hair in absence of Rhizobium sp. AAU B3. The inability of NRE (Bacillus sp. AAU B6 and Bacillus sp. AAU B12) to enter root hairs may be attributed to their inability to produce nod factors due to the absence of nodD gene which required attaching the tips of growing root hairs and signaling the plant to form nodules. Rhizobium sp. AAU B3 had nodulation and infection ability since it contains the nodD gene as shown in an earlier section. Invasion of infection thread by NRE strains is illustrated in Fig. 2C and D when co-inoculated with untagged Rhizobium sp. AAU B3. The colonization and occupation of tagged NRE strains inside the infection thread as well as other tissues were observed clearly. It was also observed that NRE bacteria being endophyte were not restricted to infection thread and dispersed in all adjusting tissues. The literature proved that the NRE enters root nodules only after the colonization of root nodules by Rhizobium. NRE isolates invaded and colonized the root nodules, accommodate inside root nodules proving the endophytic nature.
Co-inoculation Study of Rhizobium and NRE in Green Gram Through Pot Study
The effect of co-inoculation of Rhizobium sp. and NRE in green gram growth was examined through pot study and the results obtained are presented in Table 2. The germination percentage was found to be non-significant. Statistically, all the bacterial treatments increased the plant height as compared to control. The treatment T14 receiving all the three bacterial inoculants showed the highest plant height at 15 (24.40 cm) which was at par with T11, T4, T10, T12, and T13 showing 24.20, 24.00, 22.80, 22.73, and 21.73 cm, respectively. At 30, 45, and 60 DAS treatment T14 receiving all the three bacterial inoculants showed significantly the highest plant height 50.13, 57.07, and 62.93 cm, respectively which was found on par with T4 (100% RDNF), and T11 (R. selenitireducens AAU M1 + Bacillus sp. AAU B6 + Bacillus sp. AAU B12).
The highest number of root nodules were found in T14 (9.33) i.e. combination of two NRE and Rhizobium sp. strains which were at par with T9 (8), T10 (8.33), T11 (9.00), T12 (8), and T13 (8.33). Chlorophyll content was recorded at 30 DAS and the data revealed to be non-significant. Data regarding root length, fresh and dry biomass showed that T14 recorded significantly the highest root length (12.60 cm), fresh (19.23 g), and dry (12.77 g) biomass which was found at par with T4 (100% RDNF- 12.73 cm, 19.37 g, 12.87 g), and T11 (12.13 cm, 18.33 g, 12.63 g), respectively as compared to uninoculated control (8.30 cm, 9.90 g, 5.33 g). Data from the pot study revealed that the treatment T4 receiving 100% RDNF showed significantly the highest seed yield showing 3.77 g per plant which was at par with T14 showing 3.54 g per plant followed by T11 (3.44 g) per plant.
Data regarding total bacterial count and Rhizobium population shown in Fig. 3. It was revealed that the seed inoculation with bacteria had a positive effect on the total soil bacterial and Rhizobium population. A higher total count was observed in treatment T14 showing 8.94 log cfu g−1 which was found at par with T11 showing 8.91 log cfu g−1. Regarding soil Rhizobium population at harvest the highest population was found in T14 (6.04 log cfu g−1) which was on par with T2, T5, T6, T9, T10, T11, T12, and T13 showing 4.07, 5.67, 5.73, 5.83,5.86, 6.01, 5.85, and 5.86 log cfu g−1, respectively as compared to uninoculated control (3.61 log cfu g−1) and initial (3.16 log cfu g−1).
Co-inoculation Effect of Rhizobium and NRE in Green Gram Under Field Study
The effect of co-inoculation of Rhizobium and NRE in green gram in the field was recorded and presented in Table 3. Treatment T14 receiving co-inoculation of Rhizobium sp. AAU B3 + Bacillus sp. AAU B6 + Bacillus sp. AAU B12 showed the highest germination percentage (99.25) which was found to be at par with treatment T5 (98.38%), T6 (98.38%), T9 (98.50%), T10 (98.75%), T12 (98.38%), and T13 (98.88%) as compared to uninoculated control (94.44%).
Similarly, T14 showed the highest plant height at 30 DAS (20.65 cm), 60 DAS (46.70 cm), and 80 DAS (57.00 cm) which was at par with T4 (20.13, 45.60, and 55.20 cm). Data revealed that due to co-inoculation of NRE and Rhizobium strains, numbers of root nodules per plant increased as compared to uninoculated control. T14 showed significantly the highest average root nodules per plant (13.63) as compared to uninoculated control. The information recorded from the field study revealed that the consortium of Rhizobium sp. AAU B3, Bacillus sp. AAU B6 and Bacillus sp. AAU B12 reported significantly the highest number of pods per plant (20.55) as compared to uninoculated control (12.55). Statistics regarding root length showed that bacterial inoculants as individual and in combinations had a positive influence and increased root length. T14 was found statistically the best among all the treatments exhibiting an average 12 cm root length, which was at par with T4 (100% RDNF) and T11 showing 11.15 and 10.60 cm root length, respectively. A record regarding fresh and dry biomass revealed that T14 was superior giving the highest fresh (25.45 g) and dry (16.75 g) biomass per plant, which was at par with T4 (24.18; 15.68 g) and T11 (23.91; 6.83 g), respectively. Similarly, the combined application of NRE with Rhizobium in T14 was found superior regarding 100 seed weight showing 5.35 g over uninoculated control (4.50 g) followed by T4 (100% RDNF) i.e. 5.23 g and T11 (standard Rhizobium + NRE), i.e., 5.20 g.
It was revealed that treatment receiving 100% RDNF reported the highest effect on yield of green gram showing 1584 kg ha−1 which was at par with by T14 receiving combined inoculation of Rhizobium sp. AAU B3, Bacillus sp. AAU B6 and Bacillus sp. AAU B12 showing 1572 kg ha−1and T11 receiving R. selenitireducens AAU M1 + Bacillus sp. AAU B6 + Bacillus sp. AAU B12 showing 1498 kg ha−1seed yield as compared to uninoculated control 978 kg ha−1. Moreover, total bacterial count and rhizobial count (Fig. 4) from the field study was found the highest in treatment T14 (9.07 and 6.17 log cfu g−1) which was at par with T9, T10, T11, T12, and T13 as compared to uninoculated control (7.19 log cfu g−1).
Discussion
Traditionally, root nodules were considered as an exclusive niche for the accommodation of nitrogen-fixing rhizobial bacteria only. But recently various leguminous plants are being re-evaluated for the study of free-living non-rhizobial genera unrelated to nitrogen fixation [14, 40,41,42]. It has been reported that the Vigna radiata accommodates various types of Rhizobia [43] and NRE bacterial genera including Inquilinus, Bosea, Rhodopseudomonas, Paracraurococcus, Phyllobacterium, Ochrobactrum, Starkeya, Sphingomonas, Pseudomonas, Agromyces, Microbacterium, Ornithinicoccus, Bacillus, and Paenibacillus [10, 17, 27, 30, 44].
To focus on the exact role of NRE inside the root nodule the selected three isolates were evaluated separately as well as in combination with each other. The isolates must all be compatible with one another to establish a consortium or combinations of isolates. Cross streak test was used to determine whether the isolates were compatible [9, 45]. Plant growth-promoting bacteria facilitate enhanced plant nutrient acquisition, growth hormones, and bio-control activity [46]. Rhizobium sp. are symbiotic diazotrophs involved in the reduction of atmospheric dinitrogen to ammonia inside root nodules. In the laboratory, nitrogen fixation can be measured by different techniques like Micro-Kjeldahl [47], acetylene reduction assay [47] and Radio-labeled 15N tracer technique [5]. The atmospheric nitrogen reduction involves a nitrogenase enzyme complex that contains two components (I-nitrogenase Mo-Fe protein and II- dinitrogen reductase, Fe protein). The cluster of twenty-one genes is involved in nitrogen fixation regulation at a molecular level. Among these, genes Nif DK codes for the component I and Nif H for component II. In the present investigation, the primer pairs selected to amplify the Fe–protein-coding region ranging from 250 to 500 bp. Furthermore, two sets of primers were used to amplify the nod region. The first set of primers (NBA12 and NBF120) target the nod box regulatory elements of nodA and nodF, respectively, and the amplified region included the whole of nod of 1450 bp in length. When required, a fragment internal to nodD was amplified using another set of primers (Y5 and Y6) which amplify a fragment of about 850 bp. All three isolates grew on a nitrogen-free medium and showed nitrogen fixation ability as well as amplification of the NifH gene. While the NodD region was amplified by only Rhizobium sp. confirming that Rhizobium sp. has a specific ability to develop nodules.
Next to nitrogen, phosphorus (P) and potassium (K) is the most limiting macronutrients for plant growth. Inorganic (bound, fixed, or labile) and organic (bound) forms phosphate are present in the soil. Phosphorous is constituted of nucleic acids, phospholipids and is required for plant growth and development. The bound phosphate becomes available to plants by the action of phosphate solubilizing bacteria. These microorganisms solubilize phosphate with the ability to produce and release organic acids that chelate the cations bound to phosphate, converting it into soluble forms [48]. Hence, rhizobia and NRE isolates help in P release to the plants that absorb only the soluble P like monobasic (H2PO4−) and dibasic (H2PO42−) forms. IAA phytohormone hastens the plant growth and development as it is involved in cell division, apical dominance, tissue differentiation and vascular bundle formation [49]. Phytohormone IAA, modifies a plants auxin level which ultimately leads to plant cell proliferation trough nutrient uptake and nodulation in green gram [9]. ACC deaminase is a constituent of a group of enzymes which can utilize vitamin B6 and also a part of the tryptophan synthase family. ACC deaminase converts ACC into α-ketobutyrate and ammonia [50] so serves as source of carbon and nitrogen for microorganisms. In the present study both NRE and rhizobia produced ACC deaminase, so upon inoculation positively affects the plant biochemistry and provide support to growth under stressful conditions.
DAPI, a fluorescent nuclear and chromosome counterstain stain that binds strongly to adenine–thymine rich regions nucleotide. It can enter trough cell membrane into live cell so being used broadly in fluorescence microscopy to observe the bacterial colonization in different plant tissues. In the current investigation, NRE isolates are endophytes that penetrate plant root tissues but are unable to produce rood nodules because they lack the genes needed for infection thread production and specificity. Therefore, Rhizobium sp. inoculation is essential when NRE are inoculated as plant growth promoting fertilizers in pot and field study.
In the present investigation, NRE were inoculated individually as well as in combination with Rhizobium to study their interaction with plants and Rhizobium. It was found that the germination, plant height, root length, number of root nodules, fresh and dry biomass were increased upon the addition of Rhizobium with NRE as compared to individual application. It is observed that the number of root nodules were increased in both pot and field study, because NRE plays a role in infection thread production due to the production of different cell wall degrading enzymes like cellulase, protease, and chitinase. The role of cellulase (CelC2), the key cell wall-degrading enzyme, in facilitating the primary infection process is reported in Rhizobium sp. [13] and degradation of pectin layers by pectate lyase favors the entry of NRE. As seen in the above study, due to enhanced nutrient acquisition, IAA production, and ACC deaminase production; the Rhizobium sp. when applied in combination with NRE bacteria in pot and field conditions, increased plant height, number of root nodules, fresh biomass, dry biomass, rhizospheric microbial count and yield [43]. The yield was significantly increased upon application of rhizobium alone or in combination with NRE. According to the observations, applying Rhizobium and NRE as a bio agent in green gram can reduce the need for chemical fertilizers by 75%. Traditionally, farmers use 100% chemical fertilizers to get the highest yield of green gram but harm soil health as well as need more investments on resources. The present study showed that farmers can reduce chemical fertilizers to 75% so that soil health also preserved and the inputs also reduced by 25%.
Conclusions
Overall, it was concluded that the diverse NRE and rhizobial type bacteria reside inside the root nodules of a green gram. The selected strains Rhizobium sp. AAU B3, Bacillus sp. AAU B6 and Bacillus sp. AAU B12 were found compatible with each other. All three strains exhibited one or more PGP traits like nitrogen fixation, phosphate, and potash solubilization, IAA, and ACC deaminase production. Selected NRE were confirmed as endophytic by viewing successful colonization inside infection thread of green gram seedlings at 7 DAI treated with DAPI stain. In addition, the consortium of selected Rhizobium and NRE strains had a positive effect on the growth and yield parameters of a green gram as ascertained through pot and field study. With the use selected bio-inoculants, the use of synthetic fertilizers can be minimized by 75% as a consequence the soil health could be restored. Thus, the selected NRE (Bacillus sp. AAU B6, Bacillus sp. AAU B12) and Rhizobium sp. AAU B3 strains could be explored as PGP bio-inoculants to improve green gram yield for sustainable agriculture.
Data Availability
All data generated or analysed during this study are included in this published article (and its supplementary information files).
References
Agriculture Nutrient Management and Fertilizer. https://www.epa.gov/agriculture/agriculture-nutrient-management-and-fertilizer. Accessed on 4 Dec 2022.
Aguilar-Rivera N, Michel-Cuello C, Cárdenas-González JF (2019) Green revolution and sustainable development. In: Leal Filho W (ed) Encyclopedia of sustainability in higher education. Springer, Cham
Eliazer Nelson ARL, Ravichandran K, Antony U (2019) The impact of the green revolution on indigenous crops of India. J Ethn Food 6(8):1–10. https://doi.org/10.1186/s42779-019-0011-9
Davies WP (2003) An historical perspective from the green revolution to the gene revolution. Nutr Rev 61(6):S124–S134. https://doi.org/10.1301/nr.2003.jun.S124-S134
Pingali PL (2012) Green revolution: impacts, limits, and the path ahead. Proc Natl Acad Sci 109(31):12302–12308. https://doi.org/10.1073/pnas.0912953109
Ilahi H, Hidayat K, Adnan M, Rehman FU, Tahir R, Saeed MS, Shah SW, Toor MD (2020) Accentuating the impact of inorganic and organic fertilizers on agriculture crop production: a review. Ind J Pure App Biosci 9:36–45. https://doi.org/10.18782/2582-2845.8546
Pahalvi HN, Rafiya L, Rashid S, Nisar B, Kamili AN (2021) Chemical fertilizers and their impact on soil health. In Microbiota Biofertilizers 2:1–20. https://doi.org/10.1007/978-3-030-61010-4_1
Dahmani MA, Desrut A, Moumen B, Verdon J, Mermouri L, Kacem M, Coutos-Thévenot P, Kaid-Harche M, Bergès T, Vriet C (2020) Unearthing the plant growth-promoting traits of Bacillus megaterium RmBm31, an endophytic bacterium isolated from root nodules of Retama monosperma. Front Plant Sci 11:124. https://doi.org/10.3389/fpls.2020.00124
Tariq M, Hameed S, Yasmeen T, Ali A (2012) Non-rhizobial bacteria for improved nodulation and grain yield of mung bean [Vigna radiata L Wilczek]. Afri J Biotech 11(84):15012–15019. https://doi.org/10.5897/AJB11.3438
Basbuga S, Basbuga S, Yayla F, Mahmoud AM, Can C (2021) Diversity of rhizobial and non-rhizobial bacteria nodulating wild ancestors of grain legume crop plants. Inter Microbiol 24(2):207–218. https://doi.org/10.1007/s10123-020-00158-6
Reorienting India’s food basket: Act on pulses now. https://www.financialexpress.com/opinion/reorienting-indias-food-basket-act-on-pulses-now/1986610/ Accessed on 4 Dec 2022.
Dixon R, Kahn D (2004) Genetic regulation of biological nitrogen fixation. Nat Rev Microbiol 2:621–631. https://doi.org/10.1038/nrmicro954
Pandya M, Shalini R, Kumar GN (2013) Invasion of rhizobial infection thread by non-rhizobia for colonization of Vigna radiata root nodules. FEMS Microbiol Lett. https://doi.org/10.1111/1574-6968.12245
Muresu R, Polone E, Sulas L, Baldan B, Tondello A, Delogu G, Cappuccinelli P, Alberghini S, Benhizia Y, Benhizia H, Benguedoguar A, Mori B, Calamassi R, Dazzo F, Squartini A (2008) Coexistence of predominantly nonculturable rhizobia with diverse, endophytic bacterial taxa within nodules of wild legumes. FEMS Microbiol Ecol 63:383–400. https://doi.org/10.1111/j.1574-6941.2007.00424.x
Zhao LF, Xua YJ, Lai XH (2018) Antagonistic endophytic bacteria associated with nodules of soybean (Glycine max L.) and plant growth-promoting properties. Brazilian J Microbiol 49:269–278. https://doi.org/10.1016/j.bjm.2017.06.007
Hansen BL, Pessotti RDC, Fischer MS, Collins A, El-Hifnawi L, Liu MD, Traxler MF (2020) Cooperation, competition, and specialized metabolism in a simplified root nodule microbiome. mBio 11:e01917-20. https://doi.org/10.1128/mBio.01917-20
Zakhia F, Jeder H, Willems A, Gillis M, Dreyfus B, Lajudie P (2006) Diverse bacteria associated with root nodules of spontaneous legumes in tunisia and first report for nifH-like gene within the genera Microbacterium and Starkeya. Microb Ecol 51(3):375–393. https://doi.org/10.1007/s00248-006-9025-0
Hoque MS, Broadhurst LM, Trall PH (2011) Genetic characterization of root-nodule bacteria associated with Acacia salicina and A. stenophylla (Mimosaceae) across south-eastern Australia. Int J Syst Evol Microbiol 61:299–309. https://doi.org/10.1099/ijs.0.021014-0
Sturz AV, Christie BR, Matheson BG, Nowak J (1997) Biodiversity of endophytic bacteria which colonize red clover nodules, roots, stems and foliage and their infuence on host growth. Biol Fertil Soils 25:13–19. https://doi.org/10.1007/s003740050273
Trujillo ME, Kroppenstedt RM, Schumann P, Carro L, Martinez-Molina E (2006) Micromonospora coriariae sp. nov., isolated from root nodules of Coriari amyrtifolia. Int J Syst Evol Microbiol 56:2381–2385. https://doi.org/10.1099/ijs.0.64449-0
Trujillo ME, Kroppenstedt RM, Fernandez-Molinero C, Schumann P, Martinez-Molina E (2007) Micromonospora lupini sp. nov. and Micromonospora saelicesensis sp. nov., isolated from root nodules of Lupinus angustifolius. Int J Syst Evol Microbiol 57:2799–2804. https://doi.org/10.1099/ijs.0.65192-0
Garcia LC, Martinez-Molina E, Trujillo ME (2010) Micromonosporapisi sp. nov., isolated from root nodules of Pisum sativum. Int J Syst Evol Microbiol 60:331–337. https://doi.org/10.1099/ijs.0.012708-0
Menéndez E, Paço A (2020) Is the application of plant probiotic bacterial consortia always beneficial for plants? Exploring synergies between rhizobial and non-rhizobial bacteria and their effects on agro-economically valuable crops. Life 10(3):24. https://doi.org/10.3390/life10030024
Samavat S, Samavat S, Mafakheri S, Shakouri MJ (2012) Promoting common bean growth and nitrogen fixation by the co-inoculation of Rhizobium and Pseudomonas fluorescens isolates. Bulgarian J Agr Sci 18(3):387–395
Verma JP, Yadav J, Tiwari KN (2010) Application of Rhizobium sp BHURC01 and plant growth promoting rhizobactria on nodulation, plant biomass and yields of chickpea (Cicer arietinum L). Int J Agric Res 5(3):148–56. https://doi.org/10.3923/ijar.2010.148.156
Lu J, Yang F, Wang S, Ma H, Liang J, Chen Y (2017) Co-existence of rhizobia and diverse non-rhizobial bacteria in the rhizosphere and nodules of Dalbergia odorifera seedlings inoculated with Bradyrhizobium elkanii, Rhizobium multihospitium–like and Burkholderia pyrrocinia–like strains. Front microbiol 8:2255. https://doi.org/10.3389/fmicb.2017.02255
Dhole A, Shelat H (2022) Non-rhizobial endophytes associated with nodules of vigna radiata L. and their combined activity with rhizobium sp. Curr Microbiol 79:103. https://doi.org/10.1007/s00284-022-02792-x
AOAC (1965) Official methods of analysis of the association of official agricultural chemists. 10th edn. pp 744–745.
Stiles HR, Peterson WH, Fred EB (1926) A rapid method for the determination of sugar in bacterial cultures. J Bacteriol 12(6):427–439. https://doi.org/10.1128/jb.12.6.427-439.1926
Dhole A, Shelat H, Vyas R, Jhala Y, Bhange M (2016) Endophytic occupation of legume root nodules by nifH positive non-rhizobia and their efficacy in groundnut (Arachis hypogaea). Annals Microbiol 66(4):1397–1407. https://doi.org/10.1007/s13213-016-1227-1
Wielbo J, Podleśna A, Kidaj D, Podleśny J, Skorupska A (2015) The diversity of pea microsymbionts in various types of soils and their effects on plant host productivity. Microbes Environ 30(3):254–261. https://doi.org/10.1264/jsme2.ME14141
Laguerre G, Nour SM, Macheret V, Sanjuan J, Drouin P, Amarger N (2001) Classification of rhizobia based on nodC and nifHgene analysis reveals a close phylogenetic relationship among Phaseolus vulgaris symbionts. Microbiology 147:981–993. https://doi.org/10.1099/00221287-147-4-981
Taurian T, María SA, Jorge GA, María LT, Liliana L, Dayana P, Fernando I, Adriana F (2010) Phosphate-solubilizing peanut associated bacteria: screening for plant growth-promoting activities. Plant Soil 329:421–431. https://doi.org/10.1007/s11104-009-0168-x
Setiawati TC, Mutmainnah L (2016) Solubilization of potassium containing mineral by microorganisms from sugarcane rhizosphere. Agric Agric Sci Procedia 9:108–117. https://doi.org/10.1016/j.aaspro.2016.02.134
Glickmann E, Dessaux Y (1995) A critical examination of the specificity of the Salkowski reagent for indolic compounds produced by phytopathogenic bacteria. Appl Environ Microbiol 61(2):793. https://doi.org/10.1128/aem.61.2.793-796.1995
Duan J, Müller KM, Charles TC, Vesely S, Glick BR (2009) 1- aminocyclopropane-1-carboxylate (ACC) deaminase genes in rhizobia from southern Saskatchewan. Microb Ecol 57(3):423–436. https://doi.org/10.1007/s00248-008-9407-6
Mukharjee C, Ray K (2015) An improved DAPI staining procedure for visualization of polyphosphate granules in cyanobacterial and microlagal cells. Protocol Exchange, Nature Publishing Group. https://doi.org/10.1038/protex.2015.066
Agro-climatic information—Anand Agricultural University. http://www.aau.in/agro-climatic-information-16. Accessed on 24 April 2016
Duncan DB (1955) Multiple range and multiple F tests. Biometrics 11:1–42. https://doi.org/10.2307/3001478
Etesami H, Adl SM (2020) Can interaction between silicon and non–rhizobial bacteria help in improving nodulation and nitrogen fixation in salinity–stressed legumes? A review. Rhizosphere 15:100229. https://doi.org/10.1016/j.rhisph.2020.100229
De Meyer SE, De Beuf K, Vekeman B, Willems A (2015) A large diversity of non-rhizobial endophytes found in legume root nodules in Flanders (Belgium). Soil Bio Biochem 83:1–11. https://doi.org/10.1016/j.soilbio.2015.01.002
Purwaningsih S, Nditasari A, Antonius S (2019) Isolation, physiological characters and effectivity of bacterial isolates of root nodules from various plants on the growth of Vigna radiata L. IOP Conf. Ser Earth Environ Sci 308:012042. https://doi.org/10.1088/1755-1315/308/1/01204
Favero VO, Carvalho RH, Motta VM, Leite AB, Coelho MR, Xavier GR, Rumjanek NG, Urquiaga S (2021) Bradyrhizobium as the only rhizobial inhabitant of mung bean (Vigna radiata) nodules in tropical soils: a strategy based on microbiome for improving biological nitrogen fixation using bio-products. Front Plant Sci 11:602645. https://doi.org/10.3389/fpls.2020.602645
Bai Y, Aoust FD, Smith BD (2002) Isolation of plant-growth promoting Bacillus strains from soybean root nodules. Can J Micro 48:230–238. https://doi.org/10.1139/w02-014
Anandaraj B, Leema RDA (2010) Studies on the influence of bio inoculants (Pseudomonas fluorescens, Rhizobium sp, Bacillus megaterium) in Green gram. J Biosci Tech 1(2):95–99
Tokgoz S, Lakshman DK, Ghozlan MH, Pinar H, Roberts DP, Mitra A (2020) Soybean nodule-associated non-rhizobial bacteria inhibit plant pathogens and induce growth promotion in tomato. Plants 9:1494. https://doi.org/10.3390/plants9111494
Subba Rao NS (2018) Soil microbiology, 5th edn. MEDTECH, A division of Scientific International Pvt. Ltd. p 217–218.
Hajjam Y, Alami IT, Udupa SM, Cherkaoui S (2016) Isolation and evaluation of phosphate solubilizing rhizobia from root nodules of faba bean (Vicia faba L) in Morocco. J Mater Environ Sci 7(11):4000–4010
Pandya M, Rajput M, Rajkumar S (2015) Exploring plant growth promoting potential of non rhizobial root nodules endophytes of V radiata. Microbiol 84(1):110–119. https://doi.org/10.1134/S0026261715010105
Chinnaswamy A, Peña TC, Stoll A, Rojo DP, Bravo J, Rincón A, Lucas MM, Pueyo JJ (2018) A nodule endophytic Bacillus megaterium strain isolated from Medicago polymorpha enhances growth, promotes nodulation by Ensifer medicae and alleviates salt stress in alfalfa plants. Ann Appl Biol 172:295–308. https://doi.org/10.1111/aab.12420
Acknowledgements
Authors are thankful to Dr. R. V. Vyas for providing facilities to carry out all the experiments at Anand Agricultural University, Anand.
Funding
The research was funded by the Department of Science and Technology via INSPIRE Fellowship.
Author information
Authors and Affiliations
Contributions
AMD: Research, Carried out experiment, Data analysis, Manuscript writing. HNS: Evolution, Statistical analysis, validation, Manuscript editing and reviewing. HKP: Molecular experimentation, Data analysis, Manuscript editing and reviewing. YKJ: Biochemical analysis, ARDRA analysis, Manuscript editing and reviewing.
Corresponding author
Ethics declarations
Conflict of interest
The authors declare that there are no conflicts of interest associated with this publication.
Ethical Approval
This research did not involve any studies with human participants or animals (vertebrates) performed by any of the authors.
Consent to Participate
N/A.
Consent for Publication
Authors approves consent for publication of the above work.
Additional information
Publisher's Note
Springer Nature remains neutral with regard to jurisdictional claims in published maps and institutional affiliations.
Rights and permissions
Springer Nature or its licensor (e.g. a society or other partner) holds exclusive rights to this article under a publishing agreement with the author(s) or other rightsholder(s); author self-archiving of the accepted manuscript version of this article is solely governed by the terms of such publishing agreement and applicable law.
About this article
Cite this article
Dhole, A.M., Shelat, H.N., Patel, H.K. et al. Evaluation of the Co-inoculation Effect of Rhizobium and Plant Growth Promoting Non-rhizobial Endophytes on Vigna radiata. Curr Microbiol 80, 167 (2023). https://doi.org/10.1007/s00284-023-03266-4
Received:
Accepted:
Published:
DOI: https://doi.org/10.1007/s00284-023-03266-4