Abstract
Previous studies have suggested reduced pulmonary valve annulus (PVA) growth and progression of pulmonary outflow obstruction in fetuses with tetralogy of Fallot (TOF). The goals of this study were to (1) investigate the trajectory of PVA growth in utero, and (2) compare two methods of z-score determination for fetal and postnatal PVA size by echocardiography in order to improve prenatal counseling for patients with TOF. Fetal echocardiograms (FE) at a single institution with a diagnosis of TOF between 8/2008 and 12/2015 were retrospectively reviewed. Patients included had at least 2 FEs and 1 immediate postnatal echocardiogram (TTE). Fetal and postnatal demographic, clinical, and echocardiographic data were collected. Fetal body surface area (BSA) was calculated by estimating fetal weight and height; z-scores were determined based on fetal gestational age (GA) and BSA for both FEs and TTEs. Fetal PVA z-scores by GA or BSA were then compared to postnatal PVA z-scores by BSA. Twenty-two patients with 44 FEs and 22 TTEs were included. GA at the first FE was 23 weeks ± 3.4 and 32 weeks ± 3.1 at the second FE. There was no difference in PVA z-scores (by BSA) between the first and second FE (p = 0.34), but a decrease in PVA z-scores (by BSA) between the second FE and TTE (− 1.6 ± 0.5 vs. − 2.0 ± 0.7; p = 0.01). Repeat comparison with fetal PVA z-scores indexed to GA revealed no difference in z-scores between the first and second FE, but an increase in PVA z-scores between the second FE (by GA) and TTE (by BSA) (− 4.1 ± 1.0 vs. − 2.0 ± 0.7; p < 0.0001). The rate of PVA growth between the two FEs (23 µm/day ± 9.8) and between the second FE and TTE (28 µm/day ± 42) remained comparable (p = 0.57); however, the rate of BSA increase was greater in later gestation (9 cm2/day ± 3 vs. 20 cm2/day ± 11; p = 0.001). In patients with TOF, the rate of PVA growth appears to remain consistent through gestation; however, somatic growth rate increases in late gestation. Fetal PVA z-scores indexed to GA are thus inaccurate in predicting postnatal PVA z-scores typically indexed to BSA. This observation should be considered during prenatal consultation and delivery planning.
Similar content being viewed by others
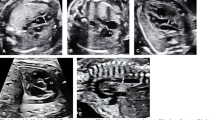
Explore related subjects
Discover the latest articles, news and stories from top researchers in related subjects.Avoid common mistakes on your manuscript.
Introduction
Tetralogy of Fallot (TOF) remains the most common form of cyanotic congenital heart disease, accounting for approximately 7–10% of all congenital cardiac malformations [1]. The diagnosis of TOF is commonly made in utero during mid-gestation with a high degree of accuracy [2, 3].
Earlier studies have suggested reduced pulmonary valve annulus (PVA) growth and progression of pulmonary outflow obstruction in fetuses with TOF [4,5,6], with implications on fetal counseling and delivery planning. As a result, an additional fetal echocardiogram (FE) in the third trimester is often performed to evaluate for progression of right ventricular outflow tract obstruction [7]. Typically, fetal z-scores are indexed to gestational age (GA), whereas postnatal z-scores are indexed to body surface area (BSA) [8, 9]; this practice difference in z-score estimation may render the fetal z-score inaccurate in predicting postnatal PVA z-scores, which play an extremely important role in determining surgical strategy [10, 11].
There is limited information on longitudinal assessment of PVA growth and the relationship of PVA to somatic growth through gestation in this patient population. This study aimed to investigate PVA growth in utero, and evaluate fetal and neonatal z-scores for PVA size indexed to both BSA and GA in order to improve prenatal counseling for patients with TOF.
Methods
Patient Population
The Lucile Packard Children’s Hospital (Stanford Children’s Health) Heart Center database was queried retrospectively to identify all fetal patients diagnosed with TOF with pulmonary stenosis (PS) between August 2008 and December 2015. Criteria for inclusion were singleton pregnancies followed longitudinally at our institution with at least 2 FEs and 1 postnatal transthoracic echocardiogram (TTE). Patients with TOF and pulmonary atresia on first FE, in utero fetal demise or termination, and those confirmed on postnatal TTE to have a diagnosis other than TOF with PS, including patients with double outlet right ventricle with subaortic ventricular septal defect (with no anterior deviation of the conal septum) and isolated valvar PS were excluded. The distinction between TOF and double outlet right ventricle was made on the basis of loss of mitral-aortic valve fibrous continuity.
Data Collected
Fetal and postnatal demographic and clinical data were collected, including each patient’s gender, GA at the time of echocardiogram, date of birth, underlying chromosomal anomalies, and date and type of surgical repair. GA was calculated according to results of first trimester crown–rump length measurements and used for dating in subsequent FEs [12, 13]. Fetal biometric data included cardiothoracic ratio (by circumference), abdominal circumference, head circumference, biparietal diameter, and femur length. Fetal weight was calculated using the standard Hadlock formula combining abdominal circumference, femur length, and biparietal diameter [14, 15]. Femur length was used to estimate fetal length, as previously described [16]. BSA in square meters (m2) was then calculated using the Haycock formula with estimated weight and length [17].
Echocardiographic studies were performed in all patients as part of their routine pre- and postnatal evaluations. The ultrasound equipment used for the echocardiographic studies was the Sequoia C512 (Siemens Medical Solutions USA Inc., Mountain View, CA), Acuson SC2000 (Siemens Medical Solutions USA Inc., Mountain View, CA), or the Philips iE33 (Philips Medical Systems, Bothell, WA).
A single pediatric cardiologist specializing in fetal cardiology (AA) retrospectively re-measured the following fetal echocardiographic variables using the syngo Dynamics workstation (Siemens Medical Solutions USA Inc.; syngo Dynamics Solutions, Ann Arbor, MI): PVA diameter, main pulmonary artery diameter, left and right proximal pulmonary artery diameter, and aortic valve annulus diameter in the standard views (during systole from inner-edge to inner-edge) [18, 19]. Direction of flow across the ductus arteriosus, middle cerebral artery, and umbilical artery pulsatility indices were recorded. The presence of atrioventricular valve regurgitation, ventricular dysfunction, and hydrops was noted. Fetal echocardiographic characteristics were then examined in the subpopulation of neonates who required surgery at < 30 days of age.
The same pediatric cardiologist (AA), blinded to clinical outcomes, retrospectively re-measured PVA diameter, main pulmonary artery diameter, left and right proximal pulmonary artery diameter, and aortic valve annulus diameter on the postnatal TTE using the syngo Dynamics workstation (Siemens Medical Solutions USA Inc.; syngo Dynamics Solutions, Ann Arbor, MI) [8, 9].
Statistical Analysis
Descriptive statistics were calculated, with continuous data presented as mean ± standard deviation (SD), medians [range], and 95% confidence intervals (CI). Parametric testing was used to compare data with normal distributions; paired comparisons were performed using the t-test and repeated measures analysis of variance (ANOVA) with post-hoc Bonferroni correction. Non-parametric testing was used to compare data with non-normal distributions; paired comparisons were performed using the Wilcoxon signed-rank test.
Z-scores were calculated using normative data adjusted to GA and BSA for both FEs and TTEs [8, 9]. Fetal PVA z-scores based on GA or BSA were compared to postnatal PVA z-scores based on BSA. Longitudinal assessment of PVA and pulmonary artery growth was performed using the initial FE and last FE prior to delivery for each patient. Change in PVA diameter and PVA z-score over gestation were calculated using the difference between the first FE and last FE, and last FE and first postnatal TTE. The change in PVA diameter was divided by the difference in GA (in days) to obtain a rate of change in micrometers (µm)/day of gestation. Similarly, rate of BSA increase was calculated using the difference in BSA between the first FE and last FE, and last FE and first postnatal TTE divided by the difference in GA (in days) to obtain a rate of change in square centimeters (cm2)/day. Regression analysis was used to compare PVA diameter with fetal weight, femur length, abdominal circumference, and head circumference.
A second investigator (SB) performed measurements of fetal PVA size by the same methodology on a subset of 10 patients selected via random number generator in a Microsoft Excel © spreadsheet (Microsoft Corporation, Redmond, WA) to determine inter-observer variability and reproducibility.
A p value of less than 0.05 was considered statistically significant. All analyses were performed using IBM SPSS Statistics version 23.0 (IBM Corporation, Armonk, NY). The study protocol was approved by the Stanford University Institutional Review Board.
Results
Patient Population
Twenty-two fetuses diagnosed with TOF with PS met inclusion criteria (Table 1). Average GA at the first FE was 23 ± 3.4 and 32 ± 3.1 weeks at the follow-up FE prior to delivery. Initial TTE was performed at 3 ± 12.5 days of life, with 2 patients born at outside institutions and returning for subsequent care. Only 1 patient (5%) was found to have 22q11.2 deletion, and 5 patients (23%) had Trisomy 21. No fetus demonstrated ventricular dysfunction or hydrops during gestation.
Assessment of Interval PVA Growth
The echocardiographic measurements through gestation are summarized in Table 2. Mean PVA diameter increased between the first and second FE (2.7 ± 0.9 vs. 4.1 ± 1.2 mm; p < 0.0001) and between the second FE and initial postnatal TTE (4.1 ± 1.2 vs. 5.1 ± 1.3 mm; p < 0.0001). When indexed to GA, PVA z-score did not change through gestation or postnatally; however, when indexed to BSA, PVA z-score demonstrated a decrease in z-score between the second FE and initial postnatal TTE (− 1.6 ± 0.5 vs. − 2.0 ± 0.7; p = 0.01).
Similarly, absolute main pulmonary artery diameter increased during gestation, but demonstrated a decrease in z-score by BSA between the second FE and initial postnatal TTE (− 2.0 ± 0.9 vs. − 2.7 ± 1.2; p < 0.0001).
While rate of PVA growth remained linear during gestation at 23 ± 9.8 µm/day between FEs and 28 ± 42 µm/day between the second FE and postnatal TTE (p = 0.57; Table 3; Fig. 1), BSA increased significantly in the immediate perinatal period (p = 0.001; Table 3; Fig. 1).
After excluding fetuses with Trisomy 21, results remained similar with linear PVA growth through gestation at 23 ± 1.0 µm/day between FEs and 37 ± 4.0 µm/day between the second FE and postnatal TTE (p = 0.11), but significantly increased somatic growth during later gestation (9.2 ± 3.1 cm2/day between FEs vs. 17 ± 6.2 cm2/day between second FE and postnatal TTE; p = 0.002).
Z-Scores by BSA Versus GA
At the first FE, PVA z-scores based on BSA were significantly larger than z-scores by GA (− 1.4 ± 0.4 vs. − 3.7 ± 1.0; p < 0.0001); z-scores by BSA for main (− 1.8 ± 0.8 vs. − 3.0 ± 1.1; p < 0.0001), left (p < 0.0001), and right pulmonary arteries (p < 0.0001) were also significantly larger than z-scores by GA. This finding remained true through gestation on the second FE and postnatal TTE (Table 2).
While postnatal PVA z-scores based on BSA demonstrated a marked increase from fetal z-scores by GA at the second FE (− 2.0 ± 0.7 vs. − 4.1 ± 1.0; p < 0.0001; Fig. 2a), postnatal PVA z-scores based on BSA conversely demonstrated a decrease compared to fetal z-scores by BSA at the second FE (Fig. 2b).
Similar results were obtained after exclusion of fetuses with Trisomy 21, with a marked increase in postnatal PVA z-scores by BSA compared to fetal z-scores by GA at the second FE (− 2.1 ± 0.8 vs. − 4.1 ± 1.1; p < 0.0001).
PVA Compared to Fetal Biometric Data
Figure 3 shows PVA diameter compared with fetal weight and biometric measurements. PVA diameter demonstrated a linear relationship with increasing measurements of fetal size, including fetal weight (R = 0.74, R2 = 0.54; p < 0.0001), femur length (R = 0.81, R2 = 0.65; p < 0.0001), abdominal circumference (R = 0.76, R2 = 0.57; p < 0.0001), and head circumference (R = 0.80, R2 = 0.64; p < 0.0001).
Reproducibility
Inter-observer agreement for fetal PVA between two investigators was excellent, with an interclass correlation coefficient of 0.91 (95% CI 0.47–0.98).
Neonatal Surgery at < 30 Days of Age
Five infants (23%) required neonatal surgery (< 30 days of age); none had documented chromosomal anomalies. One of five infants had an absent ductus arteriosus, two of the remaining four infants documented retrograde (left-to-right) flow across the ductus arteriosus through gestation. One of the five infants presented with discontinuous pulmonary arteries with a left pulmonary artery arising from the aorta.
Of the infants who required neonatal surgery, PVA z-scores by BSA (− 2.0 ± 0.2 vs. − 1.5 ± 0.6; p = 0.05) and GA (− 4.9 ± 0.9 vs. − 3.9 ± 0.9; p = 0.03) were significantly smaller at the last FE than those infants who did not require neonatal surgery. The ratio of absolute PVA size to aortic valve annulus size at the second FE was also significantly smaller in the group of infants requiring neonatal surgery (0.5 ± 0.1 vs. 0.6 ± 0.2; p < 0.0001). There were no statistical differences in branch pulmonary artery z-scores, aortic valve z-scores, MCA or UA pulsatility indices at the last FE between neonates who required surgery and those who did not.
Five fetuses demonstrated more than trace tricuspid regurgitation on the second FE, with 3 (60%) requiring neonatal surgery (including the patient with discontinuous pulmonary arteries). All 5 patients underwent complete surgical repair including ventricular septal defect patch closure, infundibular muscle bundle resection, and right ventricular outflow transannular patch repair.
Discussion
In our study of 22 fetuses with TOF with PS, the absolute rate of PVA growth remained linear through gestation; however, somatic growth rate increased in late gestation contributing to smaller postnatal PVA z-scores when indexing to BSA. The findings of consistent PVA growth in our study differ from previously published studies suggesting decreased PVA growth through gestation in fetuses with TOF with PS [4,5,6]; of note, no fetus in our study had progression of right ventricular outflow tract obstruction to pulmonary atresia. The exponential increase in somatic growth through late gestation is well known, with peak fetal weight gain around 36 weeks of gestation [20]; however, no previous study has taken somatic growth into account when indexing z-scores in this fetal population.
Our study highlights the discrepancy between fetal z-scores by GA and BSA throughout gestation, leading to challenges in data interpretation when comparing typical fetal z-scores by GA to postnatal z-scores indexed to BSA. Reporting fetal PVA z-scores indexed to BSA overestimates postnatal z-scores likely due to accelerated somatic growth but linear PVA growth in late gestation. However, reporting fetal PVA z-scores indexed to GA will substantially underestimate postnatal BSA-indexed z-scores, as demonstrated in Fig. 2a. This practice may lead to an exaggeration during prenatal counseling of the likelihood of requiring a transannular patch (or earlier surgery) over potential valve-sparing repair [10, 11].
Calculating fetal BSA is not typically performed as the Haycock formula requires a height and weight, which is only an estimation in fetal life [17]. However, estimations of fetal weight based on the Hadlock formula have demonstrated reliability at predicting birth weights of infants [14, 15].
As expected, infants who required neonatal surgery prior to hospital discharge (with the exclusion of the patient with discontinuous pulmonary arteries) had smaller PVA and main pulmonary artery z-scores (by BSA and GA) than those infants who did not require neonatal surgery; however, genetic anomalies and presence of a ductus arteriosus did not predict neonatal surgery. Moreover, the ratio of pulmonary to aortic valve annulus size through gestation was found to be smaller in the group of infants requiring neonatal surgery; this ratio may be useful when discussing possible postnatal trajectory. Of note, all 5 patients requiring neonatal surgery at our site underwent primary complete repair for TOF; at other institutions, neonatal intervention may instead include a modified Blalock–Taussig shunt, leaving the possibility for a pulmonary valve-sparing repair (as opposed to transannular patch) at an older age [21].
Fetal cardiologists should consider these observations when providing prenatal consultation to families of fetuses with TOF. Fetal BSA-based reference values should be established on a larger scale; however, even with limited data use in fetal imaging, z-scores indexed to BSA (rather than GA) may result in a closer approximation to postnatal PVA z-scores based on our findings. Prospective studies involving serial echocardiographic evaluation of fetuses with TOF with PS are needed to determine whether our findings remain true in a larger population and whether a future z-score model can be created to encompass both prenatal and postnatal measurements.
Limitations
This study was subject to the inherent limitations of a single-center retrospective study, and we were confined to analyzing only patients with available images. The power to detect differences in our study was also limited by our small sample size. Nevertheless, our findings lend more weight to the significant differences we did identify within the limited sample.
Abbreviations
- BSA:
-
Body surface area
- FE:
-
Fetal echocardiogram
- GA:
-
Gestational age
- PS:
-
Pulmonary stenosis
- PVA:
-
Pulmonary valve annulus
- TOF:
-
Tetralogy of Fallot
- TTE:
-
Transthoracic echocardiogram
References
Bailliard F, Anderson RH (2009) Tetralogy of Fallot. Orphanet J Rare Dis 4:2
Galindo A, Mendoza A, Arbues J, Grañeras A, Escribano D, Nieto O (2009) Conotruncal anomalies in fetal life: accuracy of diagnosis, associated defects and outcome. Eur J Obstet Gynecol Reprod Biol 146:55–60
Poon LC, Huggon IC, Zidere V, Allan LD (2007) Tetralogy of Fallot in the fetus in the current era. Ultrasound Obstet Gynecol 29:625–627
Hornberger LK, Sanders SP, Sahn DJ, Rice MJ, Spevak PJ, Benacerraf BR, McDonald RW, Colan SD (1995) In utero pulmonary artery and aortic growth and potential for progression of pulmonary outflow tract obstruction in tetralogy of Fallot. J Am Coll Cardiol 25:739–745
Allan LD, Sharland GK (1992) Prognosis in fetal tetralogy of Fallot. Pediatr Cardiol 13:1–4
Rice MJ, McDonald RW, Reller MD (1993) Progressive pulmonary stenosis in the fetus: two case reports. Am J Perinatol 10:424–427
Escribano D, Herraiz I, Granados M, Arbues J, Mendoza A, Galindo A (2011) Tetralogy of Fallot: prediction of outcome in the mid-second trimester of pregnancy. Prenat Diagn 31:1126–1133
Sluysmans T, Colan SD (2009) Structural measurements and adjustment for growth, Chap. 5. In: Lai WW, Cohen MS, Geva T, Mertens L (eds) Echocardiography in pediatric and congenital heart disease. Wiley-Blackwell, West Sussex
Colan SD (2009) Normal echocardiographic values for cardiovascular structures. In: Lai WW, Cohen MS, Geva T, Mertens L (eds) Echocardiography in pediatric and congenital heart disease. Wiley-Blackwell, West Sussex, pp 765–785
Jonas RA (2009) Early primary repair of tetralogy of Fallot. Semin Thorac Cardiovasc Surg Pediatr Card Surg Annu 1:39–47
Stewart RD, Backer CL, Young L, Mavroudis C (2005) Tetralogy of Fallot: results of a pulmonary valve-sparing strategy. Ann Thorac Surg 80:1431–1439
Doubilet PM (2014) Ultrasound evaluation of the first trimester. Radiol Clin North Am 52:1191–1199
Robinson HP, Fleming JE (1975) A critical evaluation of sonar ‘‘crown–rump length’’ measurements. Br J Obstet Gynaecol 82:702–710
Hadlock FP, Harrist RB, Carpenter RJ, Deter RL, Park SK (1984) Sonographic estimation of fetal weight: the value of femur length in addition to head and abdomen measurements. Radiology 150:535–540
Aviram A, Yogev Y, Ashwal E, Hiersch L, Hadar E, Gabbay-Benziv R (2017) Prediction of large for gestational age by various sonographic fetal weight estimation formulas-which should we use? J Perinatol 37:513–517
Vintzileos AM, Campbell WA, Neckles S, Pike CL, Nochimson DJ (1984) The ultrasound femur length as a predictor of fetal length. Obstet Gynecol 64:779–782
Haycock GB, Schwartz GJ, Wisotsky DH (1978) Geometric method for measuring body surface area: a height-weight formula validated in infants, children, and adults. J Pediatr 93:62–66
Sharland GK, Allan LD (1992) Normal fetal cardiac measurements derived by cross-sectional echocardiography. Ultrasound Obstet Gynecol 2:175–181
Achiron R, Golan-Porat N, Gabbay U, Rotstein Z, Heggesh J, Mashiach S, Lipitz S (1998) In utero ultrasonographic measurements of fetal aortic and pulmonary artery diameters during the first half of gestation. Ultrasound Obstet Gynecol 11:180–184
Brenner WE, Edelman DA, Hendricks CH (1976) A standard of fetal growth for the United States of America. Am J Obstet Gynecol 126:555–564
Nakashima K, Itatani K, Oka N, Kitamura T, Horai T, Hari Y, Miyaji K (2014) Pulmonary annulus growth after the modified Blalock-Taussig shunt in tetralogy of Fallot. Ann Thorac Surg 98:934–940
Author information
Authors and Affiliations
Corresponding author
Ethics declarations
Conflict of interest
The authors declare that they have no conflict of interest.
Ethical Approval
All procedures performed in studies involving human participants were in accordance with the ethical standards of the institutional and/or national research committee and with the 1964 Helsinki declaration and its later amendments or comparable ethical standards. This article does not contain any studies with animals performed by any of the authors.
Informed Consent
This is a retrospective data review study, hence need for consent was waived from the Institutional Review Board. Permission was granted for this retrospective data review.
Rights and permissions
About this article
Cite this article
Arunamata, A., Balasubramanian, S., Punn, R. et al. Impact of Fetal Somatic Growth on Pulmonary Valve Annulus Z-Scores During Gestation and Through Birth in Patients with Tetralogy of Fallot. Pediatr Cardiol 39, 1181–1187 (2018). https://doi.org/10.1007/s00246-018-1878-8
Received:
Accepted:
Published:
Issue Date:
DOI: https://doi.org/10.1007/s00246-018-1878-8