Abstract
Single nucleotide polymorphisms (SNPs) are the most frequent type of genetic alterations between individuals. An SNP located within the coding sequence of a gene may lead to an amino acid substitution and in turn might alter protein function. Such a change in protein sequence could be functionally relevant and therefore might be associated with susceptibility to human diseases, such as cancer. DNA repair mechanisms are known to play an important role in cancer development, as shown in various human cancer syndromes, which arise due to mutations in DNA repair genes. This leads to the question whether subtle genetic changes such as SNPs in DNA repair genes may contribute to cancer susceptibility. In numerous epidemiological studies, efforts have been made to associate specific SNPs in DNA repair genes with altered DNA repair and cancer. The present review describes some of the common and most extensively studied SNPs in DNA repair genes and discusses whether they are functionally relevant and subsequently increase the likelihood that cancer will develop.
Similar content being viewed by others
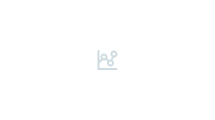
Avoid common mistakes on your manuscript.
Introduction
Cancer is a disease resulting from DNA damage caused by endogenous sources or by environmental agents. To counteract the mutagenic effects of DNA lesions, distinct pathways of DNA repair have been evolved (Friedberg et al. 2005). As DNA repair plays a critical role in protecting the human genome from mutagenic lesions, alterations in the DNA repair capacity can give rise to the development of cancer. A number of human disorders, which are characterized by a predisposition to cancer, are caused by defects in single DNA repair genes and therefore a deficiency to remove specific DNA lesions (for review see (Cleaver 2005; Hoeijmakers 2009; Köberle et al. 2005). In addition to these mutational changes, which result in human disorders, many sequence variations between individuals have been observed in human DNA repair genes (Mohrenweiser et al. 2002). Sequence variations at single nucleotides are called single nucleotide polymorphisms (SNPs). They represent the most frequent type of genetic variation between individuals. SNPs are observed with a frequency highly variable between 1 % up to 50 % in the general population. Up-to-date information about SNPs in human DNA repair genes can be found at: http://egp.gs.washington.edu, http://www.ncbi.nlm.nih.gov/SNP/ or http://snp500cancer.nci.nih.gov.
A significant number of SNPs has been identified in sequences of DNA repair genes, which do not code for a protein; the functional significance of most of these SNPs, however, has not been elucidated to date. Of those SNPs within coding sequences, some might have no effect on the protein sequence (synonymous SNPs), while others will lead to substitutions of one amino acid with another (non-synonymous SNPs). There are algorithms available to predict the impact of an amino acid substitution on protein structure and function. The sorting intolerant from tolerant program (SIFT) uses sequence alignments and is based on the assumption that an evolutionary conserved position within a protein will be intolerant to most changes while a poorly conserved position will tolerate most changes (Ng and Henikoff 2002). The Polymorphism Phenotyping program (PolyPhen) uses structural information and predicts an amino acid substitution as benign, possibly damaging or probably damaging depending on its location within the protein structure (Ramensky et al. 2002). It is estimated that up to 30 % of the repair gene SNPs might impact protein activity (Xi et al. 2004).
It is conceivable that non-synonymous SNPs may be functionally relevant by altering protein function, which could cause differences in the cellular repair capacity and hence influence the susceptibility to cancer. In addition, SNPs in DNA repair genes might also be relevant for the cure of cancer, as DNA damage induced by chemotherapeutic drugs is subjected to repair by the cellular repair machinery (Zamble and Lippard 1995). Cancer cells deficient for DNA repair have been shown to be exquisitely sensitive to chemotherapeutic treatment (Köberle et al. 1997; Usanova et al. 2010). In a vast number of publications, it has been investigated whether SNPs in different DNA repair genes are associated with cancer incidence or therapy success. In this review we will describe some of the common DNA repair gene polymorphisms, discuss their functional significance, and assess their relevance for cancer risk or treatment success.
SNPs in base excision repair genes
Base excision repair (BER) deals with small chemical base alterations, which are formed mostly endogenously due to the cellular metabolism, but can also be induced by chemicals such as alkylating agents. BER removes damaged bases by employing specific glycosylases, which recognize and remove the damaged base leading to an abasic site, which in turn is incised by an apurinic/apyrimidinic endonuclease such as APE1. The remaining sugar fragment will be removed by a phosphodiesterase or lyase, followed by either a short patch excision repair event replacing one nucleotide or by long patch repair, which replaces 2–13 nucleotides. The remaining nick is sealed by a DNA ligase to complete the repair event (Almeida and Sobol 2007; Kim and Wilson 2012; Krokan and Bjoras 2013; Lindahl and Wood 1999; Wallace 2014) (Fig. 1).
Base excision repair (BER). Specialized gylcosylases recognize and excise the damaged base (x) leading to an abasic site, which is removed by the action of an AP endonuclease such as APE1 endonuclease, followed by removal of the remaining sugar fragment by a lyase or phosphodiesterase. The resulting gap is filled by the action of DNA pol β, followed by sealing of the remaining nick by the XRCC1-ligase IIIα complex. For a more detailed model refer to (Almeida and Sobol 2007; Kim and Wilson 2012). In red: proteins encoded by OGG1 and XRCC1 for which common SNPs are described in the text
SNPs in OGG1
OGG1 is a glycosylase, which excises the common DNA base modification 8-hydroxyguanine (8-oxoG) from DNA (Lindahl 2000). 8-oxoG, the most prominent form of DNA damage induced by exposure to reactive oxygen species (ROS), is highly mutagenic and therefore a possible cause for carcinogenesis. A reduced ability to repair this lesion may therefore lead to an accumulation of mutations and hence cancer.
Four SNPs that lead to a change of amino acids have been described within the coding region of OGG1 at amino acid coding position 229, 288, 322 and 326. Of these, only Ser326Cys is a common polymorphism, with the 326Cys allele found in 32 % of individuals (Table 1). A number of studies have investigated a possible association between the 326Cys allele and cancer risk, however, with inconsistent data. For squamous cell carcinoma of the esophagus, a positive association between the 326Cys allele and cancer has been observed suggesting that the variant allele might play a role in carcinogenesis (Xing et al. 2001). In contrast, investigations with prostate cancer suggested a protective effect of the variant allele (Xu et al. 2002). Lung cancer studies have yielded mixed results. The variant allele has been associated with either no effect for lung carcinogenesis (Kohno et al. 1998; Wikman et al. 2000) or was considered a risk factor for lung cancer, especially for squamous cell carcinoma of the lung (Duan et al. 2012; Janik et al. 2011; Le Marchand et al. 2002; Sugimura et al. 1999).
It is also not known whether the amino acid change at residue 326 has any functional significance for BER. Based on in vitro data and observations in lymphocytes and tissue lysates, it has been suggested that the Ser326Cys substitution reduces the incision activity of the OGG1 protein (Janik et al. 2011; Kohno et al. 1998; Smart et al. 2006). However, other studies reported that the variant allele of OGG1 was not associated with altered OGG1 repair activity (Dherin et al. 1999; Janssen et al. 2001). By using an oligonucleotide containing a single 8-oxoG lesion (Hamann et al. 2009) we assessed the repair activity of OGG1 Ser326 and the 326Cys variant allele. We found no difference in OGG1 repair activity when we compared protein extracts of HeLa cells expressing either Ser326 or 326Cys, indicating that the SNP has no significance for the repair activity of OGG1 (Bettina M. Fischer, unpublished observations). Furthermore, no difference in cellular sensitivity was observed when we treated HeLa cells representing the different 326 alleles with the ROS producing compound menadione (Beate Köberle, unpublished observations). Altogether, based on the conflicting epidemiological data and observations concerning the functional impact, the question still remains whether the OGG1 Ser326Cys SNP is relevant to cancer risk.
SNPs in XRCC1
XRCC1 has been found to interact with numerous other BER proteins acting as a scaffolding protein (Mutamba et al. 2011; Vidal et al. 2001). Eight SNPs, which will lead to a change in amino acids, have been identified in the coding region of the XRCC1 gene, with variants Arg194Trp, Arg280His, and Arg399Gln occurring with frequencies of 10 % or more (Table 1).
SNP Arg194Trp and a possible association with lung cancer has been investigated in different studies, the results, however, are quite variable. The 194Trp allele was associated with a protective effect against lung cancer (De Ruyck et al. 2007; Yin et al. 2009), but this observation was not confirmed in other studies (Hao et al. 2006; Hung et al. 2005). Mixed results were also obtained for other cancer entities. While a protective effect of the XRCC1 variant has been reported for nasopharyngeal carcinoma (Cao et al. 2006) and squamous cell carcinoma (Kang et al. 2007), an increased risk was observed for salivary gland carcinoma (Ho et al. 2007). For alleles 280 and 399, no significant association between the variant form and risk of cancers including lung cancer (Hao et al. 2006; Hung et al. 2005; Yin et al. 2009), salivary gland carcinoma (Ho et al. 2007) and nasopharyngeal carcinoma (Cao et al. 2006) is reported, suggesting that the XRCC1 SNPs at position 280 and 399 do not play a major role for cancer risk. On the other hand, an increased cancer risk for the variant form of allele 399 was reported for squamous cell carcinoma (Kang et al. 2007) and lung cancer (De Ruyck et al. 2007; Wang et al. 2015).
Whether the described XRCC1 SNPs are functionally significant is not yet known. SIFT and PolyPhen analyses predict XPCC1 SNP Arg194Trp to be possibly damaging/intolerant, Arg280His to be benign/intolerant and Arg399Gln to be benign/tolerated (Table 1). Investigations for a possible relationship between DNA damage and XRCC1 SNPs revealed that the variant allele of Arg399Gln was associated with higher levels of DNA damage, leading to the suggestion that this SNP alters the XRCC1 protein sequence resulting in reduced DNA repair function (Lunn et al. 1999). However, to date no functional assay was used to assess base excision repair activity in relation to the variant allele of Arg399Gln.
In addition to the SNPs, which have been identified in the coding region of XRCC1 and which lead to amino acid changes, a SNP has been identified, which is located in the 5′ noncoding promoter region of the gene (Table 1) (Hao et al. 2004). The −77 T>C SNP occurs commonly enough for epidemiological studies, with a frequency of 78 % for the T allele and 22 % for the C allele. −77 T>C has been investigated for a possible link with cancer risk, and the results, however, were once again quite variable. De Ruyck et al. (2007) observed no association of the −77 C allele with carcinoma of the lung. However, Hao et al. (2004, 2006) reported that the −77 C allele increased the risk for developing cancer, such as esophageal squamous cell carcinoma and lung cancer, and explained the increased cancer risk by the findings that the change from T to C contributed to a decreased promoter activity of XRCC1 leading to a diminished expression of XRCC1. It was therefore reasoned that reduced levels of XRCC1 may affect overall BER activity and hence influence the individual’s risk to develop cancer (Hao et al. 2006).
XRCC1 and cancer treatment response
Several studies have sought to establish whether XRCC1 SNPs modulate clinical outcome, but these studies have yielded mixed results. In a couple of studies, the variant allele of Arg399Gln was associated with shorter overall survival and poor prognosis in patients suffering from advanced non-small cell lung cancer (Butkiewicz et al. 2011; Gurubhagavatula et al. 2004). This finding is in line with observations by Yarosh et al. (2005) who found that cells containing the wild-type allele at position 399 of XRCC1 were most sensitive toward a broad range of cytostatic drugs, while the variant allele conferred resistance. On the other hand, 399Gln was associated with hypersensitivity to ionizing radiation (Hu et al. 2001). Whether the SNP at position 399 might be relevant for clinical outcome is therefore not yet answered.
SNPs in poly(ADP-ribose) polymerase-1 (PARP-1)
The poly(ADP-ribose) polymerase-1 (PARP-1) is a 113-kDa nuclear protein involved in multiple cellular processes including DNA repair, genomic stability, proliferation, and cell death (Ko and Ren 2012; Langelier and Pascal 2013; Swindall et al. 2013). PARP-1 undertakes posttranslational modifications of proteins by adding poly(ADP-ribose) residues to amino acids. Various proteins involved in DNA damage repair, transcription and cell signaling are described as substrates for PARP-1 (Jungmichel et al. 2013), but the most extensively modified substrate is PARP-1 itself. Regarding DNA repair, there is strong evidence that PARP-1 participates in the repair of DNA single-strand breaks and also in the processes of BER and double-strand break (DBS) repair (Beck et al. 2014; De Vos et al. 2012). Concerning DBS repair, PARP-1 is among other a key component in an alternative non-homologous end-joining (NHEJ) pathway (A-NHEJ), which is manifested if factors of the classical NHEJ are compromised. Furthermore, PARP-1 is involved in the repair of DSBs at disrupted replication forks by competing with Ku70/ku80, resulting in DSB repair by homologous recombination (Hochegger et al. 2006; Wang et al. 2006). The degree of its participation in BER, however, is still debated (Reynolds et al. 2015; Ström et al. 2011). PARP-1 binds tightly to DNA single-strand breaks (Eustermann et al. 2011) and may also bind the single-strand break intermediate, which arises by APE1-mediated incision of abasic sites during the BER process. Following binding to single-strand breaks or single-strand break intermediates PARP-1 catalyzes the synthesis of poly(ADP-ribose) using NAD+ as a substrate. Auto-ribosylated PARP-1 may then recruit other proteins to the strand break, including the BER protein XRCC1 (Ko and Ren 2012).
PARP-1 is encoded by the human ADPRT gene. Five non-synonymous SNPs have been observed in the coding region of ADPRT, among them the common 2446T>C leading to a substitution of alanine for valine at codon 762 (Val762Ala) (Table 1) (Cottet et al. 2000). A meta-analysis based on 39 case–control studies showed no significant association of Val762Ala with overall cancer risk (Qin et al. 2014). However, subgroup analysis on cancer types revealed that the variant allele was associated with risk of esophageal squamous cell carcinoma (Hao et al. 2004), lung cancer (Zhang et al. 2005), breast cancer (Alanazi et al. 2013), and prostate and cervical cancer in Caucasians (Lockett et al. 2004; Roszak et al. 2013). When the impact of Val762Ala on PARP-1 protein function was investigated, a reduced PARP-1 enzyme activity was observed with the variant allele 762Ala (Lockett et al. 2004; Wang et al. 2007), which may be due to a change in protein structure as hypothesized by protein prediction analysis studies (Alanazi et al. 2013). For glioblastoma, on the other hand, the variant allele of PARP-1 was associated with a 20 % reduction in cancer risk (McKean-Cowdin et al. 2009) suggesting that Val762Ala may function in a cancer-specific manner.
SNPs in nucleotide excision repair genes
Nucleotide excision repair (NER) is a highly conserved repair pathway, which recognizes and removes a wide variety of mainly bulky, helix-distorting lesions induced by UV irradiation and chemical agents (Marteijn et al. 2014; Nouspikel 2009; Schärer 2013; Shuck et al. 2008; Wood 1997). Two NER sub-pathways exist: global genome NER (GG-NER) dealing with lesions in the entire genome, and transcription-coupled NER (TC-NER), which repairs damage that blocks the elongating RNA polymerase II (de Laat et al. 1999; Fousteri and Mullenders 2008). GG-NER and TC-NER differ only in the initial step of lesion detection. Following DNA damage recognition, the two sub-pathways then converge. NER has been extensively studied over the last decades and is well documented. The repair process involves the following steps: (1) recognition of the damage and incision on both sides of a lesion, (2) excision of the damaged fragment, (3) DNA synthesis to replace the excised fragment, and (4) DNA ligation (Fig. 2). In eukaryotes, more than 30 proteins are involved in NER. The core incision reaction requires the protein factors XPC-hHR23B, XPA, RPA, TFIIH, ERCC1-XPF, and XPG (Aboussekhra et al. 1995). The major roles of XPC-hHR23B, XPA, RPA, and TFIIH factors are in damage recognition and formation of an unwound intermediate structure. The heterodimer DDB is also involved in the initial damage recognition process (Wakasugi et al. 2001). The lesion containing DNA strand is then cleaved on the 3′ side by the XPG endonuclease and the 5′ side by the ERCC1-XPF endonuclease (Evans et al. 1997a; Sijbers et al. 1996). The lesion containing oligonucleotide is removed, the gap is filled by DNA repair synthesis in a reaction catalyzed by PCNA-dependent DNA polymerase δ or ε (Wood and Shivji 1997), and the patch is sealed by a XRCC1-DNA ligase IIIα complex (Marteijn et al. 2014; Moser et al. 2007). All key components of the process have been cloned in the last decades (Friedberg et al. 2005). Mutations in NER genes lead to human disorders, namely xeroderma pigmentosum (XP), Cockayne syndrome (CS), and trichothiodystrophy (TTD), of which XP is characterized by a high incidence of skin cancer (Cleaver 2005; Lehmann 2003). In addition to the mutational changes, which result in NER deficiency and hence may lead to cancer, many SNPs have been identified in NER genes (http://egp.gs.washington.edu/ner.html). Some common NER gene SNPs will now be discussed in detail.
Global genome nucleotide excision repair (NER). The damage-induced helix distortion is recognized by a complex of XPC-hHR23B and DDB1/DDB2. Transcription factor TFIIH with XPD among its subunits is recruited to the distortion together with RPA and XPA. The structure-specific endonuclease XPG cuts 3′ of the lesion, followed by a 5′ cut from ERCC1-XPF. The oligonucleotide containing the damage is removed and the resulting gap is filled in a polymerization reaction catalyzed by polymerase δ or ε. A ligase then seals the remaining nick. For a more detailed model of the mechanism of global genome NER and transcription-coupled NER, refer to (Marteijn et al. 2014; Nouspikel 2009). In red: proteins encoded by XPC, XPA, XPD, XPF and XPG for which common SNPs are described in the text
SNPs in the XPA gene
The XPA gene encodes for a 40-kDa protein, which is at the center of the NER machinery. XPA assists in damage recognition showing some preference for binding to damaged DNA, particularly when it is in a complex with RPA (Robins et al. 1991). Formation of the unwound intermediate structure, which precedes the dual incisions, is severely reduced in the absence of XPA, and neither the 3′ nor 5′ incision is formed (Evans et al. 1997b). In addition to interacting with DNA and RPA, XPA also physically interacts with TFIIH and ERCC1 (Park et al. 1995). Inactivating mutations in the XPA gene lead to severe forms of XP, with patients showing very complex clinical symptoms of an increased risk of cancer as well as neurological problems (Cleaver 2005).
Besides the XP-causing mutations, several polymorphisms are observed in the XPA gene. However, SNPs are very rare in the coding region of XPA. Three possible SNPs, which lead to amino acid changes, have been described, namely Arg228Gln, Val234Leu, and Leu252Val (Table 2) (Butkiewicz et al. 2000; Mellon et al. 2002; Mohrenweiser et al. 2002). To test whether the SNPs Arg228Gln or Val234Leu have any functional effect in human cells, Mellon et al. (2002) measured cell survival and DNA repair after UV irradiation. Expression vectors containing wild-type XPA cDNA or cDNAs representing the SNPs have been transfected into XPA-deficient XP12RO cells. The cells complemented with the variant allele of Arg228Gln or Val234Leu showed no decrease in TC-NER, GG-NER, or cellular survival after UV irradiation when compared to cells containing wild-type XPA cDNA (Mellon et al. 2002). Furthermore, when cells were treated with benzo[a]pyrene, the damage was repaired slightly more efficiently in the cells complemented with the polymorphic cDNAs (Porter et al. 2005). Taken together, the data strongly suggest that SNPs Arg228Gln and Val234Leu have no functional consequences for the cells. For Leu252Val, there is no information available regarding a possible functional effect on the XPA protein activity.
In addition, the variant alleles of Arg228Gln, Val234Leu, and Leu252Val are very rare, occurring with a frequency of ~1 % (Table 2). It therefore makes it impractical to perform epidemiological studies to investigate whether they are associated with cancer risk. For the XPA gene, only one SNP has been identified that occurs commonly enough for population-based case–control studies. This SNP is located in the 5′ noncoding region of the XPA gene. First reports claimed the SNP to be an A to G substitution located in the 5′ untranslated region at position −4 from the ATG start codon (−4A>G or A23G polymorphism) (Butkiewicz et al. 2000; Mellon et al. 2002). However, more recent analysis suggested that the major (or “wild-type” allele) at this position is the G allele. Statistics from the NIEHS SNP database and more recent published observations indicate that the G allele occurs in 62 % of individuals, while the A allele can be found in 38 % of individuals (Kiyohara and Yoshimasu 2007). Therefore, this SNP is now more commonly referred to as −4G>A or G23A polymorphism (Kiyohara and Yoshimasu 2007).
Several case–control studies have been performed to investigate whether SNP −4G>A is relevant for cancer risk, however, with conflicting results and still some confusion in the literature regarding the nature of the polymorphism. A single survey conducted by De Ruyck et al. (2007) observed no association between the −4G>A and risk of lung cancer. However, one study indicated that the A allele of −4G>A was associated with a reduced risk for lung cancer (Zienolddiny et al. 2006), while a majority of case–control studies reported a significant association of the A allele with increased lung cancer risk (Butkiewicz et al. 2004; Park et al. 2002; Popanda et al. 2004; Qian et al. 2011; Vogel et al. 2005). This observation is supported by two meta-analysis studies. Both studies describe an association between lung cancer and the presence of an A allele at −4G>A of the XPA gene, and it was concluded that the G allele at −4G>A protects against lung cancer (Kiyohara and Yoshimasu 2007; Liu et al. 2012). The reduced lung cancer risk was explained by a modulation in DNA repair, as Wu et al. (2003) reported a higher DNA repair capacity (DRC) in cells with the G allele at position −4. The data, however, should be interpreted with caution, as the difference in DRC was small. Whether the −4G>A SNP has any functional effect is therefore still not known. As the SNP is localized in the so-called Kozak consensus sequence, which is crucial for the initiation of translation (Kozak 1996), it was hypothesized that a G to A transition could influence the efficiency of translation and therefore may modulate the XPA protein level of the cells. However, no such association between the XPA −4G>A polymorphism and changes in XPA protein levels could be detected (Butkiewicz et al. 2010). In addition, to observe any effect on cellular DNA repair, the levels of the XPA protein would have to be reduced to about 10 % of that typically found in repair proficient cells (Köberle et al. 2006). Therefore, whether the −4G>A polymorphism is functionally significant still needs to be clarified.
As described above, the A allele of −4G>A has been linked to lung cancer in numerous studies. Similarly, the risk of esophageal squamous carcinoma is reported to be associated with the A allele (Guo et al. 2008), as it is also the case for gastric cancer, when combined with a polymorphism in the XPC gene (Palli et al. 2010). On the other hand, the risk of endometrial cancer was reduced when the A allele was present, but only among women who had used oral contraception (Weiss et al. 2006). For basal and squamous cell carcinoma of the skin, an increased risk was seen when the common G allele was present (Miller et al. 2006). This has been observed for colorectal cancer, breast cancer, and cancer of the head and neck (Liu et al. 2012).
Taken together, as the data of the epidemiological studies conflict with one another, we believe that the XPA −4G>A polymorphism should not be used to predict a general cancer risk but might be a useful marker for specific types of cancer.
Common SNPs in the XPD gene
The XPD gene product is one of ten subunits of the basal transcription factor TFIIH and plays an important role in both transcription and NER (Friedberg et al. 2005; Giglia-Mari et al. 2004). XPD is an 87-kDa protein with helicase activity, which unwinds DNA in the 5′–3′ direction (Coin et al. 2007; Schaeffer et al. 1994). Mutations in the XPD gene can result in a reduction of the helicase activity, which might affect NER and/or basal transcription leading to the human disorders already described (Cleaver 2005; Lehmann 2003). In addition to mutations, many SNPs have been reported within the XPD gene, most of them in noncoding intron regions. Five polymorphisms have been observed in the coding region of XPD (Broughton et al. 1996). Of those, two were found to lead to a change of amino acids (Asp312Asn and Lys751Gln), while the others have no effect on the amino acid sequence (Table 2). Variants of both Asp312Asn and Lys751Gln are common, with an allele frequency of 24 % for the Asn allele at residue 312 and 22 % for the Gln allele at residue 751. These SNPs have been investigated in numerous case–control studies for a possible link to cancer risk, however, with mixed results.
One investigation reported that for breast cancer the frequencies of the variant allele 312Asn were significantly higher in cancer patients compared to the control group (Hussien et al. 2012). Other studies, however, found no link between Asp312Asn and/or Lys751Gln variants and the incidence of breast cancer (Jorgensen et al. 2007; Kuschel et al. 2005). A meta-analysis performed on data of 40 individual studies also concluded that no clear association can be found between breast cancer and XPD SNPs, ruling out polymorphisms of XPD as a risk factor for this kind of cancer (Pabalan et al. 2010). The positive result of Hussien and co-workers might be due to the relatively small sample size of the study, which might have overestimated a possible cancer risk.
Studies of Asp312Asn or Lys751Gln have also failed to find an association with melanoma (Povey et al. 2007; Winsey et al. 2000), non-Hodgkin’s lymphoma (Worrillow et al. 2009), acute lymphoblastic leukemia (Batar et al. 2009), endometrial cancer (Weiss et al. 2006), bladder cancer (Shen et al. 2003; Stern et al. 2002), and colon cancer (Mort et al. 2003). In a recent meta-analysis, no correlation between Lys751Gln and the risk os laryngeal cancer was found (Li et al. 2016). On the other hand, XPD variants 312Asn and 751Gln have been associated with squamous cell carcinoma of head and neck (Sturgis et al. 2000), and for 312Asn a moderate risk of prostate cancer was observed (Rybicki et al. 2004). These findings, however, were not confirmed by other studies (Hu et al. 2012; Lockett et al. 2005).
The situation regarding lung cancer is similarly confusing. XPD SNPs have been most extensively studied as possible risk factors for the development of lung cancer, the studies, however, have yielded mixed results. An increased risk of lung cancer has been reported for the variant alleles of Asp312Asn or Lys751Gln (De Ruyck et al. 2007; Liang et al. 2003; Xing et al. 2002; Zienolddiny et al. 2006), with the higher risk affecting mostly light smokers and non-smokers (Butkiewicz et al. 2001; Qian et al. 2011). However, other studies report that there is no association between XPD SNPs and lung cancer (David-Beabes et al. 2001). A number of meta-analyses were done on published data from individual case–control studies about a possible link between XPD SNPs and lung cancer risk. While one of the studies suggested that the 312Asn and 751Gln variant alleles are associated with an increased risk of lung cancer (Hu et al. 2004); another meta-analysis concluded that no clear association can be found between the two XPD SNPs and lung cancer (Benhamou and Sarasin 2002). Altogether, to date it is still controversial whether there is a causal relationship between XPD SNPs and increased lung cancer risk.
It is also questionable whether the protein changes at residues 312 and 751 have any effect on the cellular repair capacity. Based on analyses of evolutionary alignments, Clarkson and Wood predicted that it is unlikely that the two XPD SNPs will have functional consequences (Clarkson and Wood 2005). SIFT and PolyPhen predictive analyses for the two XPD SNPs also predict that both SNPs are tolerated/benign (Table 2).
Clarkson and Wood also critically evaluated a number of published studies which investigated the impact of the two common XPD SNPs at codon 312 and codon 751 on cellular DNA repair capacity but found no convincing evidence for any functional effects of the XPD SNPs on DNA repair (Clarkson and Wood 2005). Seker et al. (2001) transfected plasmids containing cDNA for XPD wild type or variants for codon 312 or 751 into a lymphoblastoid cell line from an XP-D patient and assessed the effect on the DNA repair capacity using the host cell reactivation assay. They observed that the plasmids containing the sequence variations were able to complement the mutated XPD gene and restore repair, similar to XPD wild type. Obviously, sequence variations at codon 312 and codon 751 do not alter the cellular capacity for DNA repair. This conclusion is supported by studies of Laine et al. (2007) who applied recombinant TFIIH complexes containing the XPD variants 312Asn or 751Gln in in vitro assays to directly investigate the effect of these variants on DNA repair and basal transcription. Compared to wild-type XPD, variants 312Asn or 751Gln did not show significant differences neither for their repair nor for their transcriptional activities in vitro, which is a strong indication that the two XPD SNPs at codon 312 and codon 751 do not affect the function of the protein.
In summary, based on epidemiological data and observations concerning the functional impact, it would appear that the described XPD SNPs do not constitute a major risk for developing cancer.
SNPs in NER genes XPC, XPF and XPG
Single nucleotide polymorphisms have also been identified in genes of other XP groups, such as XP-C, XP-F, and XP-G (Table 2). XPC encodes for the 106-kDa XPC protein, which is involved in the initial damage recognition step of the NER process (Batty and Wood 2000; Sugasawa et al. 1998). XPC is tightly bound to its partner hHR23B (Masutani et al. 1994). XPC-hHR23B appears to detect and bind to helical distortions caused by DNA damage, which may initiate the NER process (Batty and Wood 2000). XPC-hHR23B is also essential for the recruitment of the remaining components of the NER machinery to the damaged site (Yokoi et al. 2000). For the XPC gene, several SNPs have been identified, with three of them being commonly studied. A 83-bp insertion consisting of A and T residues together with a 5-bp deletion (GTAAC) is often observed in intron 9 of the XPC gene resulting in an intronic, biallelic poly (AT) insertion/deletion polymorphism (XPC-PAT) at this position (Khan et al. 2000) (Table 2). For squamous cell carcinoma of head and neck, it was observed that the frequency of the XPC-PAT allele was higher in patients compared to control individuals, suggesting that XPC-PAT may contribute to an increased risk of developing this kind of cancer (Shen et al. 2001). Similarly, Marin et al. (2004) reported a significantly increased risk of lung cancer when the XPC-PAT allele was present. Using host cell reactivation assays to investigate for functional significance, XPC-PAT was shown to be associated with a small variation for DNA repair capacity (Qiao et al. 2002), and the reduced repair capacity was suggested to contribute to the increased risk of lung cancer (Khan et al. 2000; Marin et al. 2004). Other studies, however, did not report a significant association between XPC-PAT and the risk of lung cancer (De Ruyck et al. 2007; Lee et al. 2005). Altogether, due to the inconsistent data, it is therefore questionable whether the XPC-PAT allele could be used as a marker for cancer development.
Among the SNPs, which have been identified in the coding sequences of XPC, two are commonly studied: Ala499Val and Lys939Gln. An increased risk for developing bladder cancer was found for variant alleles 499Val and 939Gln (Qiu et al. 2008; Rouissi et al. 2011; Sak et al. 2006; Sanyal et al. 2004). The variant 499Val was also connected to an increased risk of gall bladder cancer, while in this case the variant form of Lys939Gln does not seem to be a risk factor (Jiao et al. 2011). No significant association between 939Gln and cancer was also observed for colorectal cancer (Gil et al. 2012). Studies investigating a possible link between Lys939Gln and lung cancer risk have yielded conflicting results. On the one hand, no significant association was found for the variant allele 939Gln and lung cancer (Hu et al. 2005). On the other hand, a meta-analysis performed on Lys939Gln identified the 939Gln allele as a risk factor for lung cancer (Qiu et al. 2008).
ERCC1-XPF is the structure-specific endonuclease, which cuts the damaged DNA strand during the NER process on the 5′ side of the lesion (Sijbers et al. 1996). XPF encodes for the ~104-kDa XPF subunit, which forms a tight complex with its partner ERCC1 (Biggerstaff et al. 1993). Mutations in XPF result in xeroderma pigmentosum complementation group XP-F, which is clinically characterized by mild symptoms and a later age of onset of skin cancer (Matsumura et al. 1998). Furthermore, numerous SNPs have been identified in the XPF gene. Seven possible XPF SNPs, which lead to amino acid changes, have been observed (http://egp.gs.washington.edu/ner.html). A number of case–control studies have been performed to investigate for a possible link between XPF SNPs and different types of cancers, with most of these studies focusing on SNP Arg415Gln (Table 2). The results, however, are inconclusive. This might be explained by relatively small sample sizes of the studies, as the variant allele 415Gln is very rare (~5 %). No association between the variant form of Arg415Gln and cancer risk has been observed with laryngeal cancer (Abbasi et al. 2009), lung cancer (Hung et al. 2008) and breast cancer (Pei et al. 2014). A meta-analysis conformed that overall the risk of breast cancer is not elevated by the variant allele 415Gln but subgroup analyses by ethnicity revealed an increased risk of breast cancer in the Caucasian ethnicity (Ding et al. 2011). A meta-analysis performed on data of 43 case–control studies confirmed the lack of a significant association between XPF Arg415Gln and overall cancer risk; moreover, when analyses by cancer type, ethnicity, or sample size were preformed, no significant association with cancer risk was observed in any of the subgroups (Shi et al. 2012). Altogether, based on the data obtained to date, it would appear that XPF SNP Arg415Gln does not constitute a major risk for developing cancer.
The XPG gene encodes for a 133-kDa structure-specific endonuclease which cleaves damaged DNA ~5 nucleotides 3′ to the site of the lesion (O’Donovan et al. 1994). Mutations in the XPG gene result in the XP phenotype as well as in a phenotype that combines features of XP and CS. A number of polymorphisms have been identified in the XPG gene, including SNPs that lead to the changes Arg1009His, Arg1080Gln, and Asp1104His (Emmert et al. 2001). While variants of Arg1009His and Arg1080Gln are very rare (~1 %), Asp1104His is a common polymorphism and has been investigated in a number of population-based case–control studies. The variant allele 1104His has been associated with cancer at several sites, such as cervical carcinoma (He et al. 2008), prostate cancer (Berhane et al. 2012), lung cancer, and squamous cell carcinomas of the oropharynx, larynx, and esophagus (Cui et al. 2006; Jeon et al. 2003). Regarding breast cancer, the frequency of the variant allele was marginally but significantly increased in patients (Kumar et al. 2003). Solely for bladder cancer, the Asp1104His SNP does not seem to play a role (Rouissi et al. 2011). Altogether, based on the epidemiological observations, the XPG Asp1104His SNP might serve as a genetic marker for susceptibility to cancer.
However, it is not yet clear whether the amino acid substitution at residue 1104 directly affects the function of XPG. SIFT and PolyPhen analyses predict this SNP to be possibly damaging/intolerant (Table 2). On the other hand, no significant differences in in vivo repair kinetics for removal of UV induced lesions were found between individuals carrying different genotypes at position 1104 (Kumar et al. 2003). Therefore, as it is not yet established whether this SNP directly affects the activity of the XPG protein, no predictions about the impact on protein function should be drawn.
SNPs in NER genes and cancer treatment response
Based on the assumption that SNPs in NER genes can alter the protein activity and hence influence the capacity of nucleotide excision repair, it was hypothesized that these SNPs not only increase the susceptibility to carcinogenesis but may also affect the response to cancer treatment, as DNA lesions induced by chemotherapeutic drugs are often repaired by NER (Zamble and Lippard 1995). As discussed in “SNPs in the XPA gene” to “SNPs in NER genes XPC, XPF and XPG” section, it should be noted that there is no convincing evidence that SNPs in NER genes actually affect the NER activity. Nevertheless, there are numerous studies investigating the effect of NER gene SNPs on response to chemotherapy, however, with mixed results. In one study, the possible association between treatment response in women with advanced ovarian epithelial cancer and SNPs in the NER genes XPA, XPC, XPD and XPG was assessed (Saldivar et al. 2007). Based on the response to platinum chemotherapy, patients were classified as “responders” and “non-responders.” This study observed no statistical significant association between treatment response and NER gene SNPs, with the lack of statistical significance being explained by low sample sizes (Saldivar et al. 2007). However, the variant genotype of XPG Asp1104His appeared to negatively affect the median survival time of the ovarian cancer patients (Saldivar et al. 2007). For patients with small cell lung cancer, who were treated with cisplatin-based combination chemotherapy, no statistically significant difference in response or survival was found relating to XPD variants Asp312Asn and Lys751Gln (Ryu et al. 2004). Contradictory to these observations, another study linked the XPD variant 312Asn to a decreased overall survival in lung cancer patients treated with cisplatin or carboplatin. Based on these findings, the authors proposed to use Asp312Asn as a prognostic marker to identify lung cancer patients who might benefit from clinical trials involving novel drugs other than platinating agents (Gurubhagavatula et al. 2004). Park et al. (2001) reported that in patients with metastatic colorectal cancer the XPD variant allele of Asp312Asn was not linked to response to oxaliplatin/5-fluoruracil chemotherapy or overall survival, while the variant allele of Lys751Gln was associated with a reduced response to treatment and a significantly shorter survival suggesting that XPD Lys751Gln may be an important marker to predict the clinical outcome to chemotherapy for that specific type of cancer. For acute myeloid leukemia, it was also assumed that Lys751Gln modulates the clinical outcome (Allan et al. 2004). A recent study by Lopes-Aguiar et al. (2016) investigated the effect of SNPs in the NER genes XPC, XPD, XPF, and ERCC1 on treatment with cisplatin and reported a correlation between the variant allele of XPD Asp312Asn and reduced progression-free survival and overall survival in patients with head and neck squamous cell carcinoma. An association with response to platinum chemotherapy was also reported for the XPA SNP −4G>A, as the G allele at this position was linked to increased response to cancer treatment in patients with advanced non-small cell lung cancer (Feng et al. 2009). Taken together, even though some studies claim that SNPs in NER genes may modulate cancer treatment, no clear association between NER SNPs and cancer treatment response can be established to date. In addition as it is assumed that SNPs in NER genes can alter protein activity and might therefore lead to a reduced NER capacity, one would expect an increased response to chemotherapy with DNA-damaging agents. As the contrary has been observed in all of the studies described in this section, it is difficult to envisage a mechanism of how SNPs in NER genes might modulate cancer treatment. We therefore suggest that even though NER SNPs might be important prognostic markers to predict the efficacy of chemotherapeutic therapy they should be used with caution to predict the clinical outcome.
SNPs in double-strand break repair genes
DNA double-strand breaks (DSBs) may arise either spontaneously during replication or as a consequence of exposure of cells to DNA-damaging agents such as ionizing radiation. Unrepaired or mis-repaired DSBs are highly cytotoxic and may lead to genomic instability and cancer. Two general types of DSB repair exist: homologous recombination (HR) and non-homologous end-joining (NHEJ) (Fig. 3a, b) (Davis and Chen 2013; Haber 2000; Takata et al. 1998; Wyman and Kanaar 2006). NHEJ appears to be the major pathway for repairing DSBs, whereas HR is restricted on removal of a portion of DSBs occurring in the late S phase/G2 phase of the cell cycle (Beucher et al. 2009; Thacker 2005). Following introduction of a DSB, a cascade of reactions is triggered, leading to the recruitment of repair factors at the site of the damage. Whether HR or NHEJ is used for repair of the DSB appears to be regulated during the cell cycle (Haber 2000; Kakarougkas and Jeggo 2014).
Double-strand break repair. a Homologous recombination (HR). At the double-strand break a 3′ end for the Rad51, Rad52 proteins are generated by a complex of Rad50/Mre11/NBS1. Rad54, XRCC2, XRCC3, BRCA2 and Rad51 paralogs participate in the following strand invasion, branch migration and resolution of Holliday junctions. b Non-homologous end-joining (NHEJ). Non-homologous end-joining is performed by a complex of Ku70, Ku80, and DNA-PKcs, which binds to the end of a double-strand break. Ligase IV and XRCC4 complete the repair event. Other factors such as Artemis, Rad50/Mre11/NBS1 complex are also involved. In red: proteins encoded by XPCC3, BRCA2, XRCC4, XRCC5, and XRCC6 for which common SNPs are described in the text
SNPs in genes of homologous double-strand break repair
The key components of HR repair have been identified in the last decades. An important role is played by the RAD51 family of genes and Rad51-like genes, among them XRCC3 (Thacker 2005). The XRCC3 gene encodes for the 37-kDa XRCC3 protein, which is supposed to maintain chromosomal stability during the repair process (Liu et al. 1998; Pierce et al. 1999; Thacker 2005). Two SNPs, which lead to a change in amino acids, have been identified in XRCC3 (Table 3). The variant form of Thr241 Met is occurring commonly enough for population-based case–control studies. A meta-analysis of 48 case–control studies reported that the variant Thr241 Met allele is associated with a slightly increased cancer risk in general, and the effect seems to be tissue dependent (Han et al. 2006). The 241Met allele has been implicated with melanoma (Winsey et al. 2000), thyroid cancer (Sturgis et al. 2005), bladder cancer (Matullo et al. 2001a), colorectal cancer (Krupa et al. 2011), brain tumors (Custodio et al. 2012), and breast cancer (He et al. 2012). Investigation of DNA damage in lymphocytes indicated that carriers of the 241Met allele had higher DNA damage levels compared to control individuals, and it was suggested that the variant allele of Thr241Met is associated with a low DNA repair capacity (Matullo et al. 2001b). Danoy et al. (2007) performed functional analysis studies to assess whether the Thr241Met polymorphism has any effect on DNA damage induced by DNA cross-linking agents. In these experiments expression constructs containing either XRCC3 wild-type cDNA or the cDNA representing the variant allele for codon 241 were transfected into XRCC3 deficient DT40 chicken cells and the sensitivity toward cisplatin or mitomycin C was assessed using colony formation. DT40 cells were shown to be significantly impaired in their potential to perform homologous recombination (Takata et al. 2001). As DT40 cells complemented with the 241Met allele showed similar sensitivity compared to cells with wild-type cDNA, it seems that SNP Thr241Met does not have any significant effect on DNA damage repair (Danoy et al. 2007). In addition, several studies failed to find an association between variant allele 241Met and different types of cancer such as lung cancer (David-Beabes et al. 2001), colon carcinoma (Tranah et al. 2004), head and neck cancer (Gresner et al. 2012), and breast cancer (Romanowicz-Makowska et al. 2011; Silva et al. 2010). A meta-analysis performed on Thr241Met and gastric cancer observed no overall cancer risk connected to the variant allele (Fang et al. 2011). However, in a subgroup analysis based on ethnicity, the variant allele was associated with an increased cancer risk in Caucasians, but decreased cancer risk in Asians (Fang et al. 2011). Another SNP in the XRCC3 gene is Arg94His, which is very rare and the information about a possible link to cancer risk is limited. Only one study implicated the variant allele 94His with head and neck cancer (Gresner et al. 2012).
BRCA2 also functions in the HR process (Krejci et al. 2012; Venkitaraman 2002). It acts by binding to single-stranded DNA and interacting with Rad51 to stimulate strand invasion, a vital step of homologous recombination (Davies et al. 2001; Yang et al. 2002). Several SNPs leading to amino acid changes have been characterized in the BRCA2 gene, with most of them occurring very seldom (~ 1 %). In a number of epidemiological studies, some of the BRCA2 SNPs have been evaluated in relation to cancer risk. As BRCA2 is a known breast cancer susceptibility gene (Martin and Weber 2000), most studies have focused on the relevance of BRCA2 SNPs for this type of cancer, however, with contradictory results. The variant allele of Asn372His has been reported to be associated with an increased risk for breast cancer (Healey et al. 2000; Spurdle et al. 2002). A link to breast cancer has also been observed for the variant alleles of Met784Val (Ishitobi et al. 2003) and Asn991Asp (Loizidou et al. 2009). However, other studies report that there is no significant association of BRCA2 SNPs to breast cancer risk (Baynes et al. 2007; Freedman et al. 2004; Garcia-Closas et al. 2006). Numerous studies have shown that BRCA2 is implicated in hereditary breast cancer as individuals carrying mutations in BRCA2 have an increased risk for cancer development (Cavanagh and Rogers 2015; King et al. 2003). As described above the epidemiological data are variable and therefore do not allow to answer the question of whether polymorphisms in BRCA2 are also relevant for breast cancer risk. For follicular lymphoma, a subtype of non-Hodgkin’s lymphoma, BRCA2 SNP Asn372His, is implicated in cancer development (Salagovic et al. 2012). For thyroid cancer, on the other hand, neither Asn372His nor Asn991Asp had an influence on cancer development (Sturgis et al. 2005). Altogether, based on the inconsistent data, it is difficult to speculate on BRCA2 SNPs as risk factors for cancer development.
SNPs in genes of non-homologous end-joining
The second main pathway by which DSBs are repaired is by non-homologous end-joining (NHEJ), which involves the rejoining of the broken DNA ends. Several of the genes taking part in NHEJ have been identified and their protein products characterized. The process requires as a minimum the core factors Ku70, Ku80, XRCC4, DNA-PKcs, and DNA ligase IV (Fig. 3) (Davis and Chen 2013; Haber 2000). XRCC4 has been shown to interact directly with Ku70 and Ku80 and is suggested to serve as a link between Ku70/80 and ligase IV (Mari et al. 2006). Five non-synonymous SNPs have been found in the XRCC4 gene (Table 3). However, as these SNPs are rare, only limited data are available investigating for a possible relationship between SNP and cancer risk. For Ala247Ser no association between the variant allele and bladder cancer was observed (Chang et al. 2009). Similarly, a meta-analysis of published studies on lung cancer failed to find an association between this type of cancer and a number of XRCC4 SNPs (Yu et al. 2011). In addition to SNPs in the coding region of XRCC4, numerous SNPs have been identified in noncoding sequences of XRCC4 such as the promoter region and the introns. The information about these SNPs is, however, somewhat confusing as to the nature of the SNP, the exact location and the respective SNP reference. A number of SNPs are reported to be located in the promoter region of XRCC4, and it was investigated whether SNPs in the promoter region would influence the transcriptional activity and hence the susceptibility to cancer development. At position −651/−652 of the XRCC4 promoter a SNP for T/G has been reported, for which the SNP references rs6869366 or rs2075685 have been assigned. Yu et al. (2011) generated luciferase reporter gene constructs containing 1000 bp of the XRCC4 promoter region with either G or T at position −651 and reported a significantly higher reporter gene expression when T was present. As this effect has been observed in the cervical cancer cell line HeLa, the lung carcinoma cell line H1299 and the colon carcinoma cell line HCT116 the authors suggested that the G to T allele change leads to an increase in promoter activity in a non-tissue-specific manner (Yu et al. 2011). However, Shao et al. (2014) reported higher luciferase activities connected to the G allele at position −652T>G when prostate cancer cell lines were used. Therefore, neither the exact position of SNPs in the promoter region nor the effect on transcriptional activity is yet clear. Investigations for a possible link between −561/−562 SNP (rs2075685) and cancer risk also yielded conflicting data. While the T allele was associated with a higher risk of breast cancer (Fu et al. 2003) and pancreatic cancer (Shen et al. 2015), the G allele was associated with bladder cancer (Chang et al. 2009). However, a meta-analysis of data from published studies of different cancer types failed to find any association of rs2075685 with cancer risk (Shao et al. 2012/2013). Data on additional SNPs, also located in noncoding sequences of XRCC4 are similarly inconsistent, reporting a possible contribution to cancer susceptibility (Figueroa et al. 2007; Hayden et al. 2007; Shao et al. 2012/2013) or no effect on cancer risk (Chang et al. 2009; Shao et al. 2012/2013). A summary of studies investigating a possible association between XRCC4 SNPs and risk of multiple cancers is given by Wu et al. (2008).
The proteins Ku70 and Ku80 are encoded by XRCC6 and XRCC5, respectively. Regarding SNPs in XRCC6 and XRCC5 and cancer risk, only limited information is available. The variant allele of −1310 C>G, which is located in front of the promoter start of XRCC6, has been reported as a risk allele for breast cancer (Fu et al. 2003; Willems et al. 2008, 2009), while for 2099–2408 G>A of XRCC5, no significant effect on breast cancer risk was observed (Fu et al. 2003). The synonymous SNP at allele 1781 G>T of XRCC6, underlying Gly593Gly, seemed to protect against breast cancer (Willems et al. 2008) or showed no effect on cancer risk (Fu et al. 2003). In addition, rs9288516 of XRCC5 was connected to decreased risk of brain tumors (Jin et al. 2015) but increased risk of liver cancer (Li et al. 2011), while no clear association of XRCC6 SNPs and liver cancer was observed (Li et al. 2011).
Concluding remarks
A significant number of SNPs has been characterized in DNA repair genes, some of them leading to a change in the amino acid sequence of the respective protein. It therefore has been hypothesized that these so-called non-synonymous SNPs might affect the cellular DNA repair capacity and in turn might increase the likelihood that cancer will develop. A number of epidemiological studies have reported an association between specific SNPs and certain types of cancer, while other investigations failed to do so. In addition, there is little evidence that SNPs in DNA repair genes have an impact on protein function and will lead to proteins with functional deficiency. However, if a specific SNP occurs more frequently in a cancer population than in control group, this SNP may be linked to cancer risk and might be used as a genetic marker. It is, however, important to remember that a possible association between SNP and cancer risk does not imply the SNP to be functionally significant. This is illustrated by a synonymous SNP in the XPD gene, which has no effect on the protein sequence of XPD, but the variant allele at position 156 has been associated with an increased risk of bladder cancer (Shao et al. 2007). Altogether, to better understand the biological relevance of different SNPs, more functional experiments are required.
References
Abbasi R, Ramroth H, Becher H, Dietz A, Schmezer P, Popanda O (2009) Laryngeal cancer risk associated with smoking and alcohol consumption is modified by genetic polymorphisms in ERCC5, ERCC6 and RAD23B but not by polymorphisms in five other nucleotide excision repair genes. Int J Cancer 125:1431–1439
Aboussekhra A, Biggerstaff M, Shivji MK, Vilpo JA, Moncollin V, Podust VN, Protic M, Hubscher U, Egly JM, Wood RD (1995) Mammalian DNA nucleotide excision repair reconstituted with purified protein components. Cell 80:859–868
Alanazi M, Pathan AA, Abduljaleel Z, Shaik JP, Alabdulkarim HA, Semlali A, Bazzi MD, Parine NR (2013) Association between PARP-1 V762A polymorphism and breast cancer susceptibility in Saudi population. PLoS ONE 8:e85541
Allan JM, Smith AG, Wheatley K, Hills RK, Travis LB, Hill DA, Swirsky DM, Morgan GJ, Wild CP (2004) Genetic variation in XPD predicts treatment outcome and risk of acute myeloid leukemia following chemotherapy. Blood 104:3872–3877
Almeida KH, Sobol RW (2007) A unified view of base excision repair: lesion-dependent protein complexes regulated by post-translational modification. DNA Repair (Amst) 6:695–711
Batar B, Guven M, Baris S, Celkan T, Yildiz I (2009) DNA repair gene XPD and XRCC1 polymorphisms and the risk of childhood acute lymphoblastic leukemia. Leuk Res 33:759–763
Batty DP, Wood RD (2000) Damage recognition in nucleotide excision repair of DNA. Gene 241:193–204
Baynes C, Healey CS, Pooley KA, Scollen S, Luben RN, Thompson DJ, Pharoah PD, Easton DF, Ponder BA, Dunning AM (2007) Common variants in the ATM, BRCA1, BRCA2, CHEK2 and TP53 cancer susceptibility genes are unlikely to increase breast cancer risk. Breast Cancer Res 9:R27
Beck C, Robert I, Reina-San-Martin B, Schreiber V, Dantzer F (2014) Poly(ADP-ribose) polymerases in double-strand break repair: focus on PARP1, PARP2 and PARP3. Exp Cell Res 329:18–25
Benhamou S, Sarasin A (2002) ERCC2/XPD gene polymorphisms and cancer risk. Mutagenesis 17:463–469
Berhane N, Sobti RC, Mahdi SA (2012) DNA repair genes polymorphism (XPG and XRCC1) and association of prostate cancer in a north Indian population. Mol Biol Rep 39:2471–2479
Beucher A, Birraux J, Tchouandong L, Barton O, Shibata A, Conrad S, Goodarzi AA, Krempler A, Jeggo PA, Lobrich M (2009) ATM and Artemis promote homologous recombination of radiation-induced DNA double-strand breaks in G2. EMBO J 28:3413–3427
Biggerstaff M, Szymkowski DE, Wood RD (1993) Co-correction of the ERCC1, ERCC4 and xeroderma pigmentosum group F DNA repair defects in vitro. EMBO J 12:3685–3692
Broughton BC, Steingrimsdottir H, Lehmann AR (1996) 5 polymorphisms in the coding sequence of the xeroderma pigmentosum group D gene. Mutat Res DNA Repair 362:209–211
Butkiewicz D, Rusin M, Harris CC, Chorazy M (2000) Identification of four single nucleotide polymorphisms in DNA repair genes: XPA and XPB (ERCC3) in Polish population. Hum Mutat 15:577–578
Butkiewicz D, Rusin M, Enewold L, Shields PG, Chorazy M, Harris CC (2001) Genetic polymorphisms in DNA repair genes and risk of lung cancer. Carcinogenesis 22:593–597
Butkiewicz D, Popanda O, Risch A, Edler L, Dienemann H, Schulz V, Kayser K, Drings P, Bartsch H, Schmezer P (2004) Association between the risk for lung adenocarcinoma and a (−4) G-to-A polymorphism in the XPA gene. Cancer Epidemiol Biomark Prev 13:2242–2246
Butkiewicz D, Krzesniak M, Vaitiekunaite R, Sikora B, Bowman ED, Harris CC, Rusin M (2010) A functional analysis of G23A polymorphism and the alternative splicing in the expression of the XPA gene. Cell Mol Biol Lett 15:611–629
Butkiewicz D, Rusin M, Sikora B, Lach A, Chorazy M (2011) An association between DNA repair gene polymorphisms and survival in patients with resected non-small cell lung cancer. Mol Biol Rep 38:5231–5241
Cao Y, Miao XP, Huang MY, Deng L, Hu LF, Ernberg I, Zeng YX, Lin DX, Shao JY (2006) Polymorphisms of XRCC1 genes and risk of nasopharyngeal carcinoma in the Cantonese population. BMC Cancer 6:167
Cavanagh H, Rogers KM (2015) The role of BRCA1 and BRCA2 mutations in prostate, pancreatic and stomach cancers. Hered Cancer Clin Pract 13:16
Chang CH, Chang CL, Tsai CW, Wu HC, Chiu CF, Wang RF, Liu CS, Lin CC, Bau DT (2009) Significant association of an XRCC4 single nucleotide polymorphism with bladder cancer susceptibility in Taiwan. Anticancer Res 29:1777–1782
Clarkson SG, Wood RD (2005) Polymorphisms in the human XPD (ERCC2) gene, DNA repair capacity and cancer susceptibility: an appraisal. DNA Repair (Amst) 4:1068–1074
Cleaver JE (2005) Cancer in xeroderma pigmentosum and related disorders of DNA repair. Nat Rev Cancer 5:564–573
Coin F, Oksenych V, Egly JM (2007) Distinct roles for the XPB/p52 and XPD/p44 subcomplexes of TFIIH in damaged DNA opening during nucleotide excision repair. Mol Cell 26:245–256
Cottet F, Blanche H, Verasdonck P, Le Gall I, Schachter F, Burkle A, Muiras ML (2000) New polymorphisms in the human poly(ADP-ribose) polymerase-1 coding sequence: lack of association with longevity or with increased cellular poly(ADP-ribosyl)ation capacity. J Mol Med (Berl) 78:431–440
Cui Y, Morgenstern H, Greenland S, Tashkin DP, Mao J, Cao W, Cozen W, Mack TM, Zhang ZF (2006) Polymorphism of xeroderma pigmentosum group G and the risk of lung cancer and squamous cell carcinomas of the oropharynx, larynx and esophagus. Int J Cancer 118:714–720
Custodio AC, Almeida LO, Pinto GR, Santos MJ, Almeida JR, Clara CA, Rey JA, Casartelli C (2012) Variation in DNA repair gene XRCC3 affects susceptibility to astrocytomas and glioblastomas. Genet Mol Res 11:332–339
Danoy P, Sonoda E, Lathrop M, Takeda S, Matsuda F (2007) A naturally occurring genetic variant of human XRCC2 (R188H) confers increased resistance to cisplatin-induced DNA damage. Biochem Biophys Res Commun 352:763–768
David-Beabes GL, Lunn RM, London SJ (2001) No association between the XPD (Lys751G1n) polymorphism or the XRCC3 (Thr241 Met) polymorphism and lung cancer risk. Cancer Epidemiol Biomarkers Prev 10:911–912
Davies AA, Masson JY, McIlwraith MJ, Stasiak AZ, Stasiak A, Venkitaraman AR, West SC (2001) Role of BRCA2 in control of the RAD51 recombination and DNA repair protein. Mol Cell 7:273–282
Davis AJ, Chen DJ (2013) DNA double strand break repair via non-homologous end-joining. Transl Cancer Res 2:130–143
de Laat WL, Jaspers NGJ, Hoeijmakers JHJ (1999) Molecular mechanism of nucleotide excision repair. Genes Dev 13:768–785
De Ruyck K, Szaumkessel M, De Rudder I, Dehoorne A, Vral A, Claes K, Velghe A, Van Meerbeeck J, Thierens H (2007) Polymorphisms in base-excision repair and nucleotide-excision repair genes in relation to lung cancer risk. Mutat Res 631:101–110
De Vos M, Schreiber V, Dantzer F (2012) The diverse roles and clinical relevance of PARPs in DNA damage repair: current state of the art. Biochem Pharmacol 84:137–146
Dherin C, Radicella JP, Dizdaroglu M, Boiteux S (1999) Excision of oxidatively damaged DNA bases by the human alpha-hOgg1 protein and the polymorphic alpha-hOgg1(Ser326Cys) protein which is frequently found in human populations. Nucleic Acids Res 27:4001–4007
Ding DP, He XF, Zhang Y (2011) Lack of association between XPG Asp1104His and XPF Arg415Gln polymorphism and breast cancer risk: a meta-analysis of case-control studies. Breast Cancer Res Treat 129:203–209
Duan WX, Hua RX, Yi W, Shen LJ, Jin ZX, Zhao YH, Yi DH, Chen WS, Yu SQ (2012) The association between OGG1 Ser326Cys polymorphism and lung cancer susceptibility: a meta-analysis of 27 studies. PLoS ONE 7:e35970
Emmert S, Schneider TD, Khan SG, Kraemer KH (2001) The human XPG gene: gene architecture, alternative splicing and single nucleotide polymorphisms. Nucleic Acids Res 29:1443–1452
Eustermann S, Videler H, Yang JC, Cole PT, Gruszka D, Veprintsev D, Neuhaus D (2011) The DNA-binding domain of human PARP-1 interacts with DNA single-strand breaks as a monomer through its second zinc finger. J Mol Biol 407:149–170
Evans E, Fellows J, Coffer A, Wood RD (1997a) Open complex formation around a lesion during nucleotide excision repair provides a structure for cleavage by human XPG protein. EMBO J 16:625–638
Evans E, Moggs JG, Hwang JR, Egly J-M, Wood RD (1997b) Mechanism of open complex and dual incision formation by human nucleotide excision repair factors. EMBO J 16:6559–6573
Fang F, Wang J, Yao L, Yu XJ, Yu L (2011) Relationship between XRCC3 T241M polymorphism and gastric cancer risk: a meta-analysis. Med Oncol 28:999–1003
Feng J, Sun X, Sun N, Qin S, Li F, Cheng H, Chen B, Cao Y, Ma J, Cheng L, Lu Z, Ji J, Zhou Y (2009) XPA A23G polymorphism is associated with the elevated response to platinum-based chemotherapy in advanced non-small cell lung cancer. Acta Biochim Biophys Sin (Shanghai) 41:429–435
Figueroa JD, Malats N, Rothman N, Real FX, Silverman D, Kogevinas M, Chanock S, Yeager M, Welch R, Dosemeci M, Tardon A, Serra C, Carrato A, Garcia-Closas R, Castano-Vinyals G, Garcia-Closas M (2007) Evaluation of genetic variation in the double-strand break repair pathway and bladder cancer risk. Carcinogenesis 28:1788–1793
Fousteri M, Mullenders LH (2008) Transcription-coupled nucleotide excision repair in mammalian cells: molecular mechanisms and biological effects. Cell Res 18:73–84
Freedman ML, Penney KL, Stram DO, Le Marchand L, Hirschhorn JN, Kolonel LN, Altshuler D, Henderson BE, Haiman CA (2004) Common variation in BRCA2 and breast cancer risk: a haplotype-based analysis in the multiethnic cohort. Hum Mol Genet 13:2431–2441
Friedberg EC, Walker GC, Siede W, Wood RD, Schultz RA, Ellenberger T (2005) DNA repair and mutagenesis. ASM Press, Washington, DC
Fu YP, Yu JC, Cheng TC, Lou MA, Hsu GC, Wu CY, Chen ST, Wu HS, Wu PE, Shen CY (2003) Breast cancer risk associated with genotypic polymorphism of the nonhomologous end-joining genes: a multigenic study on cancer susceptibility. Cancer Res 63:2440–2446
Garcia-Closas M, Egan KM, Newcomb PA, Brinton LA, Titus-Ernstoff L, Chanock S, Welch R, Lissowska J, Peplonska B, Szeszenia-Dabrowska N, Zatonski W, Bardin-Mikolajczak A, Struewing JP (2006) Polymorphisms in DNA double-strand break repair genes and risk of breast cancer: two population-based studies in USA and Poland, and meta-analyses. Hum Genet 119:376–388
Giglia-Mari G, Coin F, Ranish JA, Hoogstraten D, Theil A, Wijgers N, Jaspers NG, Raams A, Argentini M, van der Spek PJ, Botta E, Stefanini M, Egly JM, Aebersold R, Hoeijmakers JH, Vermeulen W (2004) A new, tenth subunit of TFIIH is responsible for the DNA repair syndrome trichothiodystrophy group A. Nat Genet 36:714–719
Gil J, Ramsey D, Stembalska A, Karpinski P, Pesz KA, Laczmanska I, Leszczynski P, Grzebieniak Z, Sasiadek MM (2012) The C/A polymorphism in intron 11 of the XPC gene plays a crucial role in the modulation of an individual’s susceptibility to sporadic colorectal cancer. Mol Biol Rep 39:527–534
Gresner P, Gromadzinska J, Polanska K, Twardowska E, Jurewicz J, Wasowicz W (2012) Genetic variability of Xrcc3 and Rad51 modulates the risk of head and neck cancer. Gene 504:166–174
Guo W, Zhou RM, Wan LL, Wang N, Li Y, Zhang XJ, Dong XJ (2008) Polymorphisms of the DNA repair gene xeroderma pigmentosum groups A and C and risk of esophageal squamous cell carcinoma in a population of high incidence region of North China. J Cancer Res Clin Oncol 134:263–270
Gurubhagavatula S, Liu G, Park S, Zhou W, Su L, Wain JC, Lynch TJ, Neuberg DS, Christiani DC (2004) XPD and XRCC1 genetic polymorphisms are prognostic factors in advanced non-small-cell lung cancer patients treated with platinum chemotherapy. J Clin Oncol 22:2594–2601
Haber JE (2000) Partners and pathwaysrepairing a double-strand break. Trends Genet 16:259–264
Hamann I, Schwerdtle T, Hartwig A (2009) Establishment of a non-radioactive cleavage assay to assess the DNA repair capacity towards oxidatively damaged DNA in subcellular and cellular systems and the impact of copper. Mutat Res 669:122–130
Han S, Zhang HT, Wang Z, Xie Y, Tang R, Mao Y, Li Y (2006) DNA repair gene XRCC3 polymorphisms and cancer risk: a meta-analysis of 48 case-control studies. Eur J Hum Genet 14:1136–1144
Hao B, Wang H, Zhou K, Li Y, Chen X, Zhou G, Zhu Y, Miao X, Tan W, Wei Q, Lin D, He F (2004) Identification of genetic variants in base excision repair pathway and their associations with risk of esophageal squamous cell carcinoma. Cancer Res 64:4378–4384
Hao B, Miao X, Li Y, Zhang X, Sun T, Liang G, Zhao Y, Zhou Y, Wang H, Chen X, Zhang L, Tan W, Wei Q, Lin D, He F (2006) A novel T-77C polymorphism in DNA repair gene XRCC1 contributes to diminished promoter activity and increased risk of non-small cell lung cancer. Oncogene 25:3613–3620
Hayden PJ, Tewari P, Morris DW, Staines A, Crowley D, Nieters A, Becker N, de Sanjose S, Foretova L, Maynadie M, Cocco PL, Boffetta P, Brennan P, Chanock SJ, Browne PV, Lawler M (2007) Variation in DNA repair genes XRCC3, XRCC4, XRCC5 and susceptibility to myeloma. Hum Mol Genet 16:3117–3127
He X, Ye F, Zhang J, Cheng Q, Shen J, Chen H (2008) Susceptibility of XRCC3, XPD, and XPG genetic variants to cervical carcinoma. Pathobiology 75:356–363
He XF, Wei W, Su J, Yang ZX, Liu Y, Zhang Y, Ding DP, Wang W (2012) Association between the XRCC3 polymorphisms and breast cancer risk: meta-analysis based on case-control studies. Mol Biol Rep 39:5125–5134
Healey CS, Dunning AM, Teare MD, Chase D, Parker L, Burn J, Chang-Claude J, Mannermaa A, Kataja V, Huntsman DG, Pharoah PD, Luben RN, Easton DF, Ponder BA (2000) A common variant in BRCA2 is associated with both breast cancer risk and prenatal viability. Nat Genet 26:362–364
Ho T, Li G, Lu J, Zhao C, Wei Q, Sturgis EM (2007) X-ray repair cross-complementing group 1 (XRCC1) single-nucleotide polymorphisms and the risk of salivary gland carcinomas. Cancer 110:318–325
Hochegger H, Dejsuphong D, Fukushima T, Morrison C, Sonoda E, Schreiber V, Zhao GY, Saberi A, Masutani M, Adachi N, Koyama H, de Murcia G, Takeda S (2006) Parp-1 protects homologous recombination from interference by Ku and ligase IV in vertebrate cells. EMBO J 25:1305–1314
Hoeijmakers JH (2009) DNA damage, aging, and cancer. N Engl J Med 361:1475–1485
Hu JJ, Smith TR, Miller MS, Mohrenweiser HW, Golden A, Case LD (2001) Amino acid substitution variants of APE1 and XRCC1 genes associated with ionizing radiation sensitivity. Carcinogenesis 22:917–922
Hu Z, Wei Q, Wang X, Shen H (2004) DNA repair gene XPD polymorphism and lung cancer risk: a meta-analysis. Lung Cancer 46:1–10
Hu Z, Wang Y, Wang X, Liang G, Miao X, Xu Y, Tan W, Wei Q, Lin D, Shen H (2005) DNA repair gene XPC genotypes/haplotypes and risk of lung cancer in a Chinese population. Int J Cancer 115:478–483
Hu YY, Yuan H, Jiang GB, Chen N, Wen L, Leng WD, Zeng XT, Niu YM (2012) Associations between XPD Asp312Asn polymorphism and risk of head and neck cancer: a meta-analysis based on 7,122 subjects. PLoS ONE 7:e35220
Hung RJ, Brennan P, Canzian F, Szeszenia-Dabrowska N, Zaridze D, Lissowska J, Rudnai P, Fabianova E, Mates D, Foretova L, Janout V, Bencko V, Chabrier A, Borel S, Hall J, Boffetta P (2005) Large-scale investigation of base excision repair genetic polymorphisms and lung cancer risk in a multicenter study. J Natl Cancer Inst 97:567–576
Hung RJ, Christiani DC, Risch A, Popanda O, Haugen A, Zienolddiny S, Benhamou S, Bouchardy C, Lan Q, Spitz MR, Wichmann HE, LeMarchand L, Vineis P, Matullo G, Kiyohara C, Zhang ZF, Pezeshki B, Harris C, Mechanic L, Seow A, Ng DP, Szeszenia-Dabrowska N, Zaridze D, Lissowska J, Rudnai P, Fabianova E, Mates D, Foretova L, Janout V, Bencko V, Caporaso N, Chen C, Duell EJ, Goodman G, Field JK, Houlston RS, Hong YC, Landi MT, Lazarus P, Muscat J, McLaughlin J, Schwartz AG, Shen H, Stucker I, Tajima K, Matsuo K, Thun M, Yang P, Wiencke J, Andrew AS, Monnier S, Boffetta P, Brennan P (2008) International Lung Cancer Consortium: pooled analysis of sequence variants in DNA repair and cell cycle pathways. Cancer Epidemiol Biomark Prev 17:3081–3089
Hussien YM, Gharib AF, Awad HA, Karam RA, Elsawy WH (2012) Impact of DNA repair genes polymorphism (XPD and XRCC1) on the risk of breast cancer in Egyptian female patients. Mol Biol Rep 39:1895–1901
Ishitobi M, Miyoshi Y, Ando A, Hasegawa S, Egawa C, Tamaki Y, Monden M, Noguchi S (2003) Association of BRCA2 polymorphism at codon 784 (Met/Val) with breast cancer risk and prognosis. Clin Cancer Res 9:1376–1380
Janik J, Swoboda M, Janowska B, Ciesla JM, Gackowski D, Kowalewski J, Olinski R, Tudek B, Speina E (2011) 8-Oxoguanine incision activity is impaired in lung tissues of NSCLC patients with the polymorphism of OGG1 and XRCC1 genes. Mutat Res 709–710:21–31
Janssen K, Schlink K, Gotte W, Hippler B, Kaina B, Oesch F (2001) DNA repair activity of 8-oxoguanine DNA glycosylase 1 (OGG1) in human lymphocytes is not dependent on genetic polymorphism Ser326/Cys326. Mutat Res 486:207–216
Jeon HS, Kim KM, Park SH, Lee SY, Choi JE, Lee GY, Kam S, Park RW, Kim IS, Kim CH, Jung TH, Park JY (2003) Relationship between XPG codon 1104 polymorphism and risk of primary lung cancer. Carcinogenesis 24:1677–1681
Jiao X, Ren J, Chen H, Ma J, Rao S, Huang K, Wu S, Fu J, Su X, Luo C, Shi J, Broelsch CE (2011) Ala499Val (C>T) and Lys939Gln (A>C) polymorphisms of the XPC gene: their correlation with the risk of primary gallbladder adenocarcinoma—a case–control study in China. Carcinogenesis 32:496–501
Jin T, Wang Y, Li G, Du S, Yang H, Geng T, Hou P, Gong Y (2015) Analysis of difference of association between polymorphisms in the XRCC5, RPA3 and RTEL1 genes and glioma, astrocytoma and glioblastoma. Am J Cancer Res 5:2294–2300
Jorgensen TJ, Visvanathan K, Ruczinski I, Thuita L, Hoffman S, Helzlsouer KJ (2007) Breast cancer risk is not associated with polymorphic forms of xeroderma pigmentosum genes in a cohort of women from Washington County, Maryland. Breast Cancer Res Treat 101:65–71
Jungmichel S, Rosenthal F, Altmeyer M, Lukas J, Hottiger MO, Nielsen ML (2013) Proteome-wide identification of poly(ADP-ribosyl)ation targets in different genotoxic stress responses. Mol Cell 52:272–285
Kakarougkas A, Jeggo PA (2014) DNA DSB repair pathway choice: an orchestrated handover mechanism. Br J Radiol 87:20130685
Kang SY, Lee KG, Lee W, Shim JY, Ji SI, Chung KW, Chung YK, Kim NK (2007) Polymorphisms in the DNA repair gene XRCC1 associated with basal cell carcinoma and squamous cell carcinoma of the skin in a Korean population. Cancer Sci 98:716–720
Khan SG, Metter EJ, Tarone RE, Bohr VA, Grossman L, Hedayati M, Bale SJ, Emmert S, Kraemer KH (2000) A new xeroderma pigmentosum group C poly(AT) insertion/deletion polymorphism. Carcinogenesis 21:1821–1825
Kim YJ, Wilson DM 3rd (2012) Overview of base excision repair biochemistry. Curr Mol Pharmacol 5:3–13
King MC, Marks JH, Mandell JB (2003) Breast and ovarian cancer risks due to inherited mutations in BRCA1 and BRCA2. Science 302:643–646
Kiyohara C, Yoshimasu K (2007) Genetic polymorphisms in the nucleotide excision repair pathway and lung cancer risk: a meta-analysis. Int J Med Sci 4:59–71
Ko HL, Ren EC (2012) Functional aspects of PARP1 in DNA repair and transcription. Biomolecules 2:524–548
Köberle B, Grimaldi KA, Sunters A, Hartley JA, Kelland LR, Masters JR (1997) DNA repair capacity and cisplatin sensitivity of human testis tumour cells. Int J Cancer 70:551–555
Köberle B, Wittschieben J, Wood RD (2005) DNA repair and cancer. In: Knowles M, Selby PJ (eds) Cellular and molecular biology of cancer. Oxford University Press, Oxford
Köberle B, Roginskaya V, Wood RD (2006) XPA protein as a limiting factor for nucleotide excision repair and UV sensitivity in human cells. DNA Repair 5:641–648
Kohno T, Shinmura K, Tosaka M, Tani M, Kim SR, Sugimura H, Nohmi T, Kasai H, Yokota J (1998) Genetic polymorphisms and alternative splicing of the hOGG1 gene, that is involved in the repair of 8-hydroxyguanine in damaged DNA. Oncogene 16:3219–3225
Kozak M (1996) Interpreting cDNA sequences: some insights from studies on translation. Mamm Genome 7:563–574
Krejci L, Altmannova V, Spirek M, Zhao X (2012) Homologous recombination and its regulation. Nucleic Acids Res 40:5795–5818
Krokan HE, Bjoras M (2013) Base excision repair. Cold Spring Harb Perspect Biol 5:a012583
Krupa R, Sliwinski T, Wisniewska-Jarosinska M, Chojnacki J, Wasylecka M, Dziki L, Morawiec J, Blasiak J (2011) Polymorphisms in RAD51, XRCC2 and XRCC3 genes of the homologous recombination repair in colorectal cancer—a case control study. Mol Biol Rep 38:2849–2854
Kumar R, Hoglund L, Zhao C, Forsti A, Snellman E, Hemminki K (2003) Single nucleotide polymorphisms in the XPG gene: determination of role in DNA repair and breast cancer risk. Int J Cancer 103:671–675
Kuschel B, Chenevix-Trench G, Spurdle AB, Chen X, Hopper JL, Giles GG, McCredie M, Chang-Claude J, Gregory CS, Day NE, Easton DF, Ponder BA, Dunning AM, Pharoah PD (2005) Common polymorphisms in ERCC2 (xeroderma pigmentosum D) are not associated with breast cancer risk. Cancer Epidemiol Biomark Prev 14:1828–1831
Laine JP, Mocquet V, Bonfanti M, Braun C, Egly JM, Brousset P (2007) Common XPD (ERCC2) polymorphisms have no measurable effect on nucleotide excision repair and basal transcription. DNA Repair (Amst) 6:1264–1270
Langelier MF, Pascal JM (2013) PARP-1 mechanism for coupling DNA damage detection to poly(ADP-ribose) synthesis. Curr Opin Struct Biol 23:134–143
Le Marchand L, Donlon T, Lum-Jones A, Seifried A, Wilkens LR (2002) Association of the hOGG1 Ser326Cys polymorphism with lung cancer risk. Cancer Epidemiol Biomark Prev 11:409–412
Lee GY, Jang JS, Lee SY, Jeon HS, Kim KM, Choi JE, Park JM, Chae MH, Lee WK, Kam S, Kim IS, Lee JT, Jung TH, Park JY (2005) XPC polymorphisms and lung cancer risk. Int J Cancer 115:807–813
Lehmann AR (2003) DNA repair-deficient diseases, xeroderma pigmentosum, Cockayne syndrome and trichothiodystrophy. Biochimie 85:1101–1111
Li R, Yang Y, An Y, Zhou Y, Liu Y, Yu Q, Lu D, Wang H, Jin L, Zhou W, Qian J, Shugart YY (2011) Genetic polymorphisms in DNA double-strand break repair genes XRCC5, XRCC6 and susceptibility to hepatocellular carcinoma. Carcinogenesis 32:530–536
Li F, Wang J, Chen M (2016) Single nucleotide polymorphisms in DNA repair genes and the risk of laryngeal cancer: a meta-analysis. Biomed Pharmacother 78:92–100
Liang G, Xing D, Miao X, Tan W, Yu C, Lu W, Lin D (2003) Sequence variations in the DNA repair gene XPD and risk of lung cancer in a Chinese population. Int J Cancer 105:669–673
Lindahl T (2000) Suppression of spontaneous mutagenesis in human cells by DNA base excision-repair. Mutat Res Rev Mutat Res 462:129–135
Lindahl T, Wood RD (1999) Quality control by DNA repair. Science 286:1897–1905
Liu N, Lamerdin JE, Tebbs RS, Schild D, Tucker JD, Shen MR, Brookman KW, Siciliano MJ, Walter CA, Fan WF, Narayana LS, Zhou ZQ, Adamson AW, Sorensen KJ, Chen DJ, Jones NJ, Thompson LH (1998) XRCC2 and XRCC3, new human RAD51-family members, promote chromosome stability and protect against DNA cross-links and other damages. Mol Cell 1:783–793
Liu J, Zhang Z, Cao XL, Lei DP, Wang ZQ, Jin T, Pan XL (2012) XPA A23G polymorphism and susceptibility to cancer: a meta-analysis. Mol Biol Rep 39:6791–6799
Lockett KL, Hall MC, Xu J, Zheng SL, Berwick M, Chuang SC, Clark PE, Cramer SD, Lohman K, Hu JJ (2004) The ADPRT V762A genetic variant contributes to prostate cancer susceptibility and deficient enzyme function. Cancer Res 64:6344–6348
Lockett KL, Snowhite IV, Hu JJ (2005) Nucleotide-excision repair and prostate cancer risk. Cancer Lett 220:125–135
Loizidou MA, Michael T, Neuhausen SL, Newbold RF, Marcou Y, Kakouri E, Daniel M, Papadopoulos P, Malas S, Hadjisavvas A, Kyriacou K (2009) DNA-repair genetic polymorphisms and risk of breast cancer in Cyprus. Breast Cancer Res Treat 115:623–627
Lopes-Aguiar L, Costa EF, Nogueira GA, Lima TR, Visacri MB, Pincinato EC, Calonga L, Mariano FV, Altemani AM, Altemani JM, Coutinho-Camillo CM, Alves MA, Moriel P, Ramos CD, Chone CT, Lima CS (2016) XPD c.934G>A polymorphism of nucleotide excision repair pathway in outcome of head and neck squamous cell carcinoma patients treated with cisplatin chemoradiation. Oncotarget. doi:10.18632/oncotarget.7668
Lunn RM, Langlois RG, Hsieh LL, Thompson CL, Bell DA (1999) XRCC1 polymorphisms: effects on aflatoxin B1-DNA adducts and glycophorin A variant frequency. Cancer Res 59:2557–2561
Mari PO, Florea BI, Persengiev SP, Verkaik NS, Bruggenwirth HT, Modesti M, Giglia-Mari G, Bezstarosti K, Demmers JA, Luider TM, Houtsmuller AB, van Gent DC (2006) Dynamic assembly of end-joining complexes requires interaction between Ku70/80 and XRCC4. Proc Natl Acad Sci USA 103:18597–18602
Marin MS, Lopez-Cima MF, Garcia-Castro L, Pascual T, Marron MG, Tardon A (2004) Poly (AT) polymorphism in intron 11 of the XPC DNA repair gene enhances the risk of lung cancer. Cancer Epidemiol Biomark Prev 13:1788–1793
Marteijn JA, Lans H, Vermeulen W, Hoeijmakers JH (2014) Understanding nucleotide excision repair and its roles in cancer and ageing. Nat Rev Mol Cell Biol 15:465–481
Martin AM, Weber BL (2000) Genetic and hormonal risk factors in breast cancer. J Natl Cancer Inst 92:1126–1135
Masutani C, Sugasawa K, Yanagisawa J, Sonoyama T, Ui M, Enomoto T, Takio K, Tanaka K, van der Spek PJ, Bootsma D, Hoeijmakers JHJ, Hanaoka F (1994) Purification and cloning of a nucleotide excision repair complex involving the xeroderma pigmentosum group C protein and a human homologue of yeast RAD23. EMBO J 13:1831–1843
Matsumura Y, Nishigori C, Yagi T, Imamura S, Takebe H (1998) Characterization of molecular defects in xeroderma pigmentosum group F in relation to its clinically mild symptoms. Hum Mol Genet 7:969–974
Matullo G, Guarrera S, Carturan S, Peluso M, Malaveille C, Davico L, Piazza A, Vineis P (2001a) DNA repair gene polymorphisms, bulky DNA adducts in white blood cells and bladder cancer in a case-control study. Int J Cancer 92:562–567
Matullo G, Palli D, Peluso M, Guarrera S, Carturan S, Celentano E, Krogh V, Munnia A, Tumino R, Polidoro S, Piazza A, Vineis P (2001b) XRCC1, XRCC3, XPD gene polymorphisms, smoking and (32)P-DNA adducts in a sample of healthy subjects. Carcinogenesis 22:1437–1445
McKean-Cowdin R, Barnholtz-Sloan J, Inskip PD, Ruder AM, Butler M, Rajaraman P, Razavi P, Patoka J, Wiencke JK, Bondy ML, Wrensch M (2009) Associations between polymorphisms in DNA repair genes and glioblastoma. Cancer Epidemiol Biomark Prev 18:1118–1126
Mellon I, Hock T, Reid R, Porter PC, States JC (2002) Polymorphisms in the human xeroderma pigmentosum group A gene and their impact on cell survival and nucleotide excision repair. DNA Repair (Amst) 1:531–546
Miller KL, Karagas MR, Kraft P, Hunter DJ, Catalano PJ, Byler SH, Nelson HH (2006) XPA, haplotypes, and risk of basal and squamous cell carcinoma. Carcinogenesis 27:1670–1675
Mohrenweiser HW, Xi T, Vazquez-Matias J, Jones IM (2002) Identification of 127 amino acid substitution variants in screening 37 DNA repair genes in humans. Cancer Epidemiol Biomark Prev 11:1054–1064
Mort R, Mo L, McEwan C, Melton DW (2003) Lack of involvement of nucleotide excision repair gene polymorphisms in colorectal cancer. Br J Cancer 89:333–337
Moser J, Kool H, Giakzidis I, Caldecott K, Mullenders LH, Fousteri MI (2007) Sealing of chromosomal DNA nicks during nucleotide excision repair requires XRCC1 and DNA ligase III alpha in a cell-cycle-specific manner. Mol Cell 27:311–323
Mutamba JT, Svilar D, Prasongtanakij S, Wang XH, Lin YC, Dedon PC, Sobol RW, Engelward BP (2011) XRCC1 and base excision repair balance in response to nitric oxide. DNA Repair (Amst) 10:1282–1293
Ng PC, Henikoff S (2002) Accounting for human polymorphisms predicted to affect protein function. Genome Res 12:436–446
Nouspikel T (2009) DNA repair in mammalian cells: nucleotide excision repair: variations on versatility. Cell Mol Life Sci 66:994–1009
O’Donovan A, Davies AA, Moggs JG, West SC, Wood RD (1994) XPG endonuclease makes the 3′ incision in human DNA nucleotide excision repair. Nature 371:432–435
Pabalan N, Francisco-Pabalan O, Sung L, Jarjanazi H, Ozcelik H (2010) Meta-analysis of two ERCC2 (XPD) polymorphisms, Asp312Asn and Lys751Gln, in breast cancer. Breast Cancer Res Treat 124:531–541
Palli D, Polidoro S, D’Errico M, Saieva C, Guarrera S, Calcagnile AS, Sera F, Allione A, Gemma S, Zanna I, Filomena A, Testai E, Caini S, Moretti R, Gomez-Miguel MJ, Nesi G, Luzzi I, Ottini L, Masala G, Matullo G, Dogliotti E (2010) Polymorphic DNA repair and metabolic genes: a multigenic study on gastric cancer. Mutagenesis 25:569–575
Park CH, Mu D, Reardon JT, Sancar A (1995) The general transcription-repair factor TFIIH is recruited to the excision-repair complex by the XPA protein independent of the TFIIE transcription factor. J Biol Chem 270:4896–4902
Park DJ, Stoehlmacher J, Zhang W, Tsao-Wei DD, Groshen S, Lenz HJ (2001) A xeroderma pigmentosum group D gene polymorphism predicts clinical outcome to platinum-based chemotherapy in patients with advanced colorectal cancer. Cancer Res 61:8654–8658
Park JY, Lee SY, Jeon HS, Bae NC, Chae SC, Joo S, Kim CH, Park JH, Kam S, Kim IS, Jung TH (2002) Polymorphism of the DNA repair gene XRCC1 and risk of primary lung cancer. Cancer Epidemiol Biomark Prev 11:23–27
Pei XH, Yang Z, Lv XQ, Li HX (2014) Genetic variation in ERCC1 and XPF genes and breast cancer risk. Genet Mol Res 13:2259–2267
Pierce AJ, Johnson RD, Thompson LH, Jasin M (1999) XRCC3 promotes homology-directed repair of DNA damage in mammalian cells. Genes Dev 13:2633–2638
Popanda O, Schattenberg T, Phong CT, Butkiewicz D, Risch A, Edler L, Kayser K, Dienemann H, Schulz V, Drings P, Bartsch H, Schmezer P (2004) Specific combinations of DNA repair gene variants and increased risk for non-small cell lung cancer. Carcinogenesis 25:2433–2441
Porter PC, Mellon I, States JC (2005) XP-A cells complemented with Arg228Gln and Val234Leu polymorphic XPA alleles repair BPDE-induced DNA damage better than cells complemented with the wild type allele. DNA Repair (Amst) 4:341–349
Povey JE, Darakhshan F, Robertson K, Bisset Y, Mekky M, Rees J, Doherty V, Kavanagh G, Anderson N, Campbell H, MacKie RM, Melton DW (2007) DNA repair gene polymorphisms and genetic predisposition to cutaneous melanoma. Carcinogenesis 28:1087–1093
Qian B, Zhang H, Zhang L, Zhou X, Yu H, Chen K (2011) Association of genetic polymorphisms in DNA repair pathway genes with non-small cell lung cancer risk. Lung Cancer 73:138–146
Qiao Y, Spitz MR, Shen H, Guo Z, Shete S, Hedayati M, Grossman L, Mohrenweiser H, Wei Q (2002) Modulation of repair of ultraviolet damage in the host-cell reactivation assay by polymorphic XPC and XPD/ERCC2 genotypes. Carcinogenesis 23:295–299
Qin Q, Lu J, Zhu H, Xu L, Cheng H, Zhan L, Yang X, Zhang C, Sun X (2014) PARP-1 Val762Ala polymorphism and risk of cancer: a meta-analysis based on 39 case-control studies. PLoS ONE 9:e98022
Qiu L, Wang Z, Shi X (2008) Associations between XPC polymorphisms and risk of cancers: a meta-analysis. Eur J Cancer 44:2241–2253
Ramensky V, Bork P, Sunyaev S (2002) Human non-synonymous SNPs: server and survey. Nucleic Acids Res 30:3894–3900
Reynolds P, Cooper S, Lomax M, O’Neill P (2015) Disruption of PARP1 function inhibits base excision repair of a sub-set of DNA lesions. Nucleic Acids Res 43:4028–4038
Robins P, Jones CJ, Biggerstaff M, Lindahl T, Wood RD (1991) Complementation of DNA repair in xeroderma pigmentosum group A cell extracts by a protein with affinity for damaged DNA. EMBO J 10:3913–3921
Romanowicz-Makowska H, Smolarz B, Zadrozny M, Westfal B, Baszczynski J, Polac I, Sporny S (2011) Single nucleotide polymorphisms in the homologous recombination repair genes and breast cancer risk in Polish women. Tohoku J Exp Med 224:201–208
Roszak A, Lianeri M, Sowinska A, Jagodzinski PP (2013) Involvement of PARP-1 Val762Ala polymorphism in the onset of cervical cancer in caucasian women. Mol Diagn Ther 17:239–245
Rouissi K, Bahria IB, Bougatef K, Marrakchi R, Stambouli N, Hamdi K, Cherif M, Ben Slama MR, Sfaxi M, Othman FB, Chebil M, Elgaaied AB, Ouerhani S (2011) The effect of tobacco, XPC, ERCC2 and ERCC5 genetic variants in bladder cancer development. BMC Cancer 11:101
Rybicki BA, Conti DV, Moreira A, Cicek M, Casey G, Witte JS (2004) DNA repair gene XRCC1 and XPD polymorphisms and risk of prostate cancer. Cancer Epidemiol Biomarkers Prev 13:23–29
Ryu JS, Hong YC, Han HS, Lee JE, Kim S, Park YM, Kim YC, Hwang TS (2004) Association between polymorphisms of ERCC1 and XPD and survival in non-small-cell lung cancer patients treated with cisplatin combination chemotherapy. Lung Cancer 44:311–316
Sak SC, Barrett JH, Paul AB, Bishop DT, Kiltie AE (2006) Comprehensive analysis of 22 XPC polymorphisms and bladder cancer risk. Cancer Epidemiol Biomark Prev 15:2537–2541
Salagovic J, Klimcakova L, Ilencikova D, Kafkova A (2012) Association of follicular lymphoma risk with BRCA2 N372H polymorphism in Slovak population. Med Oncol 29:1173–1178
Saldivar JS, Lu KH, Liang D, Gu J, Huang M, Vlastos AT, Follen M, Wu X (2007) Moving toward individualized therapy based on NER polymorphisms that predict platinum sensitivity in ovarian cancer patients. Gynecol Oncol 107:S223–S229
Sanyal S, Festa F, Sakano S, Zhang Z, Steineck G, Norming U, Wijkstrom H, Larsson P, Kumar R, Hemminki K (2004) Polymorphisms in DNA repair and metabolic genes in bladder cancer. Carcinogenesis 25:729–734
Schaeffer L, Moncollin V, Roy R, Staub A, Mezzina M, Sarasin A, Weeda G, Hoeijmakers JHJ, Egly JM (1994) The ERCC2/DNA repair protein is associated with the class-II BTF2/TFIIH transcription factor. EMBO J 13:2388–2392
Schärer OD (2013) Nucleotide excision repair in eukaryotes. Cold Spring Harb Perspect Biol 5:a012609
Seker H, Butkiewicz D, Bowman ED, Rusin M, Hedayati M, Grossman L, Harris CC (2001) Functional significance of XPD polymorphic variants: attenuated apoptosis in human lymphoblastoid cells with the XPD 312 Asp/Asp genotype. Cancer Res 61:7430–7434
Shao J, Gu M, Xu Z, Hu Q, Qian L (2007) Polymorphisms of the DNA gene XPD and risk of bladder cancer in a Southeastern Chinese population. Cancer Genet Cytogenet 177:30–36
Shao N, Li J, Xu B, Wang Y, Lu X, Feng N (2014) Role of the functional variant (−652T>G) in the XRCC4 promoter in prostate cancer. Mol Biol Rep 41:7463–7470
Shen H, Sturgis EM, Khan SG, Qiao Y, Shahlavi T, Eicher SA, Xu Y, Wang X, Strom SS, Spitz MR, Kraemer KH, Wei Q (2001) An intronic poly (AT) polymorphism of the DNA repair gene XPC and risk of squamous cell carcinoma of the head and neck: a case–control study. Cancer Res 61:3321–3325
Shen M, Hung RJ, Brennan P, Malaveille C, Donato F, Placidi D, Carta A, Hautefeuille A, Boffetta P, Porru S (2003) Polymorphisms of the DNA repair genes XRCC1, XRCC3, XPD, interaction with environmental exposures, and bladder cancer risk in a case-control study in northern Italy. Cancer Epidemiol Biomark Prev 12:1234–1240
Shen Q, Tian Y, Li K, Jiang Q, Xue H, Yang S (2015) Association of single nucleotide polymorphisms of DNA repair gene and susceptibility to pancreatic cancer. Int J Clin Exp Pathol 8:3180–3185
Shi TY, He J, Qiu LX, Zhu ML, Wang MY, Zhou XY, Han J, Yu H, Zang RY, Wei Q (2012) Association between XPF polymorphisms and cancer risk: a meta-analysis. PLoS ONE 7:e38606
Shuck SC, Short EA, Turchi JJ (2008) Eukaryotic nucleotide excision repair: from understanding mechanisms to influencing biology. Cell Res 18:64–72
Sijbers AM, de Laat WL, Ariza RR, Biggerstaff M, Wei Y-F, Moggs JG, Carter KC, Shell BK, Evans E, de Jong MC, Rademakers S, de Rooij J, Jaspers NGJ, Hoeijmakers JHJ, Wood RD (1996) Xeroderma pigmentosum group F caused by a defect in a structure-specific DNA repair endonuclease. Cell 86:811–822
Silva SN, Tomar M, Paulo C, Gomes BC, Azevedo AP, Teixeira V, Pina JE, Rueff J, Gaspar JF (2010) Breast cancer risk and common single nucleotide polymorphisms in homologous recombination DNA repair pathway genes XRCC2, XRCC3, NBS1 and RAD51. Cancer Epidemiol 34:85–92
Smart DJ, Chipman JK, Hodges NJ (2006) Activity of OGG1 variants in the repair of pro-oxidant-induced 8-oxo-2′-deoxyguanosine. DNA Repair (Amst) 5:1337–1345
Spurdle AB, Hopper JL, Chen X, Dite GS, Cui J, McCredie MR, Giles GG, Ellis-Steinborner S, Venter DJ, Newman B, Southey MC, Chenevix-Trench G (2002) The BRCA2 372 HH genotype is associated with risk of breast cancer in Australian women under age 60 years. Cancer Epidemiol Biomark Prev 11:413–416
Stern MC, Johnson LR, Bell DA, Taylor JA (2002) XPD codon 751 polymorphism, metabolism genes, smoking, and bladder cancer risk. Cancer Epidemiol Biomark Prev 11:1004–1011
Ström CE, Johansson F, Uhlen M, Szigyarto CA, Erixon K, Helleday T (2011) Poly (ADP-ribose) polymerase (PARP) is not involved in base excision repair but PARP inhibition traps a single-strand intermediate. Nucleic Acids Res 39:3166–3175
Sturgis EM, Zheng R, Li L, Castillo EJ, Eicher SA, Chen M, Strom SS, Spitz MR, Wei Q (2000) XPD/ERCC2 polymorphisms and risk of head and neck cancer: a case–control analysis. Carcinogenesis 21:2219–2223
Sturgis EM, Zhao C, Zheng R, Wei Q (2005) Radiation response genotype and risk of differentiated thyroid cancer: a case–control analysis. Laryngoscope 115:938–945
Sugasawa K, Ng JMY, Masutani C, Iwai S, van der Spek PJ, Eker APM, Hanaoka F, Bootsma D, Hoeijmakers JHJ (1998) Xeroderma pigmentosum group C protein complex is the initiator of global genome nucleotide excision repair. Mol Cell 2:223–232
Sugimura H, Kohno T, Wakai K, Nagura K, Genka K, Igarashi H, Morris BJ, Baba S, Ohno Y, Gao C, Li Z, Wang J, Takezaki T, Tajima K, Varga T, Sawaguchi T, Lum JK, Martinson JJ, Tsugane S, Iwamasa T, Shinmura K, Yokota J (1999) hOGG1 Ser326Cys polymorphism and lung cancer susceptibility. Cancer Epidemiol Biomark Prev 8:669–674
Swindall AF, Stanley JA, Yang ES (2013) PARP-1: friend or foe of DNA damage and repair in tumorigenesis? Cancers (Basel) 5:943–958
Takata M, Sasaki MS, Sonoda E, Morrison C, Hashimoto M, Utsumi H, Yamaguchiiwai Y, Shinohara A, Takeda S (1998) Homologous recombination and nonhomologous end-joining pathways of DNA double-strand break repair have overlapping roles in the maintenance of chromosomal integrity in vertebrate cells. EMBO J 17:5497–5508
Takata M, Sasaki MS, Tachiiri S, Fukushima T, Sonoda E, Schild D, Thompson LH, Takeda S (2001) Chromosome instability and defective recombinational repair in knockout mutants of the five Rad51 paralogs. Mol Cell Biol 21:2858–2866
Thacker J (2005) The RAD51 gene family, genetic instability and cancer. Cancer Lett 219:125–135
Tranah GJ, Giovannucci E, Ma J, Fuchs C, Hankinson SE, Hunter DJ (2004) XRCC2 and XRCC3 polymorphisms are not associated with risk of colorectal adenoma. Cancer Epidemiol Biomark Prev 13:1090–1091
Usanova S, Piee-Staffa A, Sied U, Thomale J, Schneider A, Kaina B, Koberle B (2010) Cisplatin sensitivity of testis tumour cells is due to deficiency in interstrand-crosslink repair and low ERCC1-XPF expression. Mol Cancer 9:248
Venkitaraman AR (2002) Cancer susceptibility and the functions of BRCA1 and BRCA2. Cell 108:171–182
Vidal AE, Boiteux S, Hickson ID, Radicella JP (2001) XRCC1 coordinates the initial and late stages of DNA abasic site repair through protein–protein interactions. EMBO J 20:6530–6539
Vogel U, Overvad K, Wallin H, Tjonneland A, Nexo BA, Raaschou-Nielsen O (2005) Combinations of polymorphisms in XPD, XPC and XPA in relation to risk of lung cancer. Cancer Lett 222:67–74
Wakasugi M, Shimizu M, Morioka H, Linn S, Nikaido O, Matsunaga T (2001) Damaged DNA-binding protein DDB stimulates the excision of cyclobutane pyrimidine dimers in vitro in concert with XPA and replication protein A. J Biol Chem 276:15434–15440
Wallace SS (2014) Base excision repair: a critical player in many games. DNA Repair (Amst) 19:14–26
Wang M, Wu W, Rosidi B, Zhang L, Wang H, Iliakis G (2006) PARP-1 and Ku compete for repair of DNA double strand breaks by distinct NHEJ pathways. Nucleic Acids Res 34:6170–6182
Wang XG, Wang ZQ, Tong WM, Shen Y (2007) PARP1 Val762Ala polymorphism reduces enzymatic activity. Biochem Biophys Res Commun 354:122–126
Wang X, Ma KW, Zhao YG, Wang GJ, Li W (2015) XRCC1 rs25487 polymorphism is associated with lung cancer risk in epidemiologically susceptible Chinese people. Genet Mol Res 14:15530–15538
Weiss JM, Weiss NS, Ulrich CM, Doherty JA, Chen C (2006) Nucleotide excision repair genotype and the incidence of endometrial cancer: effect of other risk factors on the association. Gynecol Oncol 103:891–896
Wikman H, Risch A, Klimek F, Schmezer P, Spiegelhalder B, Dienemann H, Kayser K, Schulz V, Drings P, Bartsch H (2000) hOGG1 polymorphism and loss of heterozygosity (LOH): significance for lung cancer susceptibility in a caucasian population. Int J Cancer 88:932–937
Willems P, Claes K, Baeyens A, Vandersickel V, Werbrouck J, De Ruyck K, Poppe B, Van den Broecke R, Makar A, Marras E, Perletti G, Thierens H, Vral A (2008) Polymorphisms in nonhomologous end-joining genes associated with breast cancer risk and chromosomal radiosensitivity. Genes Chromosomes Cancer 47:137–148
Willems P, De Ruyck K, Van den Broecke R, Makar A, Perletti G, Thierens H, Vral A (2009) A polymorphism in the promoter region of Ku70/XRCC6, associated with breast cancer risk and oestrogen exposure. J Cancer Res Clin Oncol 135:1159–1168
Winsey SL, Haldar NA, Marsh HP, Bunce M, Marshall SE, Harris AL, Wojnarowska F, Welsh KI (2000) A variant within the DNA repair gene XRCC3 is associated with the development of melanoma skin cancer. Cancer Res 60:5612–5616
Wood RD (1997) Nucleotide excision repair in mammalian cells. J Biol Chem 272:23465–23468
Wood R, Shivji M (1997) Which DNA polymerases are used for DNA repair in eukaryotes? Carcinogenesis 18:605–610
Worrillow L, Roman E, Adamson PJ, Kane E, Allan JM, Lightfoot TJ (2009) Polymorphisms in the nucleotide excision repair gene ERCC2/XPD and risk of non-Hodgkin lymphoma. Cancer Epidemiol 33:257–260
Wu X, Zhao H, Wei Q, Amos CI, Zhang K, Guo Z, Qiao Y, Hong WK, Spitz MR (2003) XPA polymorphism associated with reduced lung cancer risk and a modulating effect on nucleotide excision repair capacity. Carcinogenesis 24:505–509
Wu CN, Liang SY, Tsai CW, Bau DT (2008) The role of XRCC4 in carcinogenesis and anticancer drug discovery. Recent Pat Anticancer Drug Discov 3:209–219
Wyman C, Kanaar R (2006) DNA double-strand break repair: all’s well that ends well. Annu Rev Genet 40:363–383
Xi T, Jones IM, Mohrenweiser HW (2004) Many amino acid substitution variants identified in DNA repair genes during human population screenings are predicted to impact protein function. Genomics 83:970–979
Xing DY, Tan W, Song N, Lin DX (2001) Ser326Cys polymorphism in hOGG1 gene and risk of esophageal cancer in a Chinese population. Int J Cancer 95:140–143
Xing D, Tan W, Wei Q, Lin D (2002) Polymorphisms of the DNA repair gene XPD and risk of lung cancer in a Chinese population. Lung Cancer 38:123–129
Xu J, Zheng SL, Turner A, Isaacs SD, Wiley KE, Hawkins GA, Chang BL, Bleecker ER, Walsh PC, Meyers DA, Isaacs WB (2002) Associations between hOGG1 sequence variants and prostate cancer susceptibility. Cancer Res 62:2253–2257
Yang H, Jeffrey PD, Miller J, Kinnucan E, Sun Y, Thoma NH, Zheng N, Chen PL, Lee WH, Pavletich NP (2002) BRCA2 function in DNA binding and recombination from a BRCA2-DSS1-ssDNA structure. Science 297:1837–1848
Yarosh DB, Pena A, Brown DA (2005) DNA repair gene polymorphisms affect cytotoxicity in the National Cancer Institute Human Tumour Cell Line Screening Panel. Biomarkers 10:188–202
Yin J, Vogel U, Ma Y, Qi R, Wang H (2009) Association of DNA repair gene XRCC1 and lung cancer susceptibility among nonsmoking Chinese women. Cancer Genet Cytogenet 188:26–31
Yokoi M, Masutani C, Maekawa T, Sugasawa K, Ohkuma Y, Hanaoka F (2000) The xeroderma pigmentosum group C protein complex XPC-HR23B plays an important role in the recruitment of transcription factor IIH to damaged DNA. J Biol Chem 275:9870–9875
Yu H, Zhao H, Wang LE, Han Y, Chen WV, Amos CI, Rafnar T, Sulem P, Stefansson K, Landi MT, Caporaso N, Albanes D, Thun M, McKay JD, Brennan P, Wang Y, Houlston RS, Spitz MR, Wei Q (2011) An analysis of single nucleotide polymorphisms of 125 DNA repair genes in the Texas genome-wide association study of lung cancer with a replication for the XRCC4 SNPs. DNA Repair (Amst) 10:398–407
Zamble DB, Lippard SJ (1995) Cisplatin and DNA-repair in cancer-chemotherapy. Trends Biochem Sci 20:435–439
Shao N, Jiang WY, Qiao D, Zhang, SG, Wu Y, Zhang XX, Hua LX, Ding Y, Feng NH (2012/2013) An updated meta-analysis of XRCC4 polymorphisms and cancer risk based on 31 case-control studies. Cancer Biomark 12:37–47
Zhang X, Miao X, Liang G, Hao B, Wang Y, Tan W, Li Y, Guo Y, He F, Wei Q, Lin D (2005) Polymorphisms in DNA base excision repair genes ADPRT and XRCC1 and risk of lung cancer. Cancer Res 65:722–726
Zienolddiny S, Campa D, Lind H, Ryberg D, Skaug V, Stangeland L, Phillips DH, Canzian F, Haugen A (2006) Polymorphisms of DNA repair genes and risk of non-small cell lung cancer. Carcinogenesis 27:560–567
Acknowledgments
The work was supported by Deutsche Forschungsgemeinschaft (DFG) and Exzellenzinitiative KIT.
Author information
Authors and Affiliations
Corresponding author
Ethics declarations
Conflict of interest
The authors declare that they have no conflict of interest.
Rights and permissions
About this article
Cite this article
Köberle, B., Koch, B., Fischer, B.M. et al. Single nucleotide polymorphisms in DNA repair genes and putative cancer risk. Arch Toxicol 90, 2369–2388 (2016). https://doi.org/10.1007/s00204-016-1771-2
Received:
Accepted:
Published:
Issue Date:
DOI: https://doi.org/10.1007/s00204-016-1771-2